- 1Gastro Unit, Medical Division, Amager-Hvidovre University Hospital, Hvidovre, Denmark
- 2Department of Clinical Physiology and Nuclear Medicine 260, Center for Functional and Diagnostic Imaging and Research, Amager-Hvidovre Hospital, Hvidovre, Denmark
- 3Department of Clinical Medicine, Faculty of Health Sciences, University of Copenhagen, Copenhagen, Denmark
- 4Novo Nordisk Foundation Centre for Basic Metabolic Research, Faculty of Health Sciences, University of Copenhagen, Copenhagen, Denmark
Liver cirrhosis is a disease characterised by multiple complications and a poor prognosis. The prevalence is increasing worldwide. Chronic inflammation is ongoing in liver cirrhosis. No cure for the inflammation is available, and the current treatment of liver cirrhosis is only symptomatic. However, several different medical agents have been suggested as potential healing drugs. The majority are tested in rodents, but few human trials are effectuated. This review focuses on medical agents described in the literature with supposed alleviating and curing effects on liver cirrhosis. Twelve anti-inflammatory, five antioxidative, and three drugs with effects on gut microflora and the LPS pathway were found. Two drugs not categorised by the three former categories were found in addition. In total, 42 rodent studies and seven human trials were found. Promising effects of celecoxib, aspirin, curcumin, kahweol, pentoxifylline, diosmin, statins, emricasan, and silymarin were found in cirrhotic rodent models. Few indices of effects of etanercept, glycyrrhizin arginine salt, and mitoquinone were found. Faecal microbiota transplantation is in increasing searchlight with a supposed potential to alleviate cirrhosis. However, human trials are in demand to verify the findings in this review.
Introduction
Liver cirrhosis is a chronic disease with increasing prevalence. Its most common aetiologies are alcohol consumption, viral hepatitis, obesity, diabetes mellitus, and metabolic syndrome leading to non-alcoholic steatohepatitis as a part of non-alcoholic fatty liver disease (1). In general, liver cirrhosis results from ongoing fibrosis formation, and further progression leads to portal hypertension, hepatic encephalopathy, and an increased risk of organ failure and hepatocellular carcinoma (HCC), which is associated with high mortality (2).
Chronic inflammation in alcoholic liver disease is mediated by a direct response to alcohol and an indirect inflammatory response to gut microbiota-derived lipopolysaccharide (LPS), leading to a stronger oxidative-inflammatory response (3). With ongoing systemic inflammation, endothelial dysfunction, and fibrogenesis (4) evolve in the liver and are associated with elevated inflammatory cytokines and immune cell activation (5). The inflammation may be caused by translocation over the bowel wall of pathogens or derived pathogen-associated molecular patterns (PAMPs) and damage-associated molecular patterns (DAMPs). These are products of microbial origin produced by pathogens and not by the host. Products from apoptotic cells (6) translocate into the portal and systemic circulation via an impaired intestinal barrier. With a continuous injury, the PAMPs and DAMPs can activate hepatic stellate cells (HSC's) with unwanted adverse effects (6). The HSC's are a source of myofibroblasts and portal fibroblasts, which drive the fibrogenic process (2). When quiescent, HSC's mainly act as vitamin A reserves, but they can abundantly secrete extracellular matrix proteins and different proteinases that elicit unwanted liver architecture remodelling when activated.
Currently, there is no cure for this chronic inflammation in cirrhosis, and treatment mainly focuses on symptomatic relief. When ascites develops in the decompensated stage, diuretics, and albumin infusion improve fluid retention and circulatory function after paracentesis. Non-selective beta-blockers (NSBB's) decreases portal hypertension and are used for long-term treatment as primary and secondary prophylaxis of bleeding from oesophageal varices (7, 8). Hepatic encephalopathy can be reversed using antibiotics and lactulose, as the encephalopathy is often triggered by infections and constipation (9).
There is a reduced incidence of HCC in patients treated with NSBB's (10). The underlying mechanism might be a reduction in bacterial translocation from the gut, which may diminish the portal load of PAMP's and thus the hepatic inflammation.
As hepatic inflammation and neo-angiogenesis are critical drivers in the pathogenesis of HCC, the beta-adrenergic blockade may impede angiogenesis through inhibition of vascular endothelial growth factor production and prevent HCC (11). The preliminary studies need further scientific explorations to support this hypothesis.
Diuretics are the first choice treatment of ascites in decompensated cirrhosis (8, 12). However, side effects of diuretics include fluid- and electrolyte disturbances, dehydration, and renal impairment. Albumin infusion is used to prevent the development of hepatorenal syndrome (HRS) and relieve circulatory disturbances in decompensated cirrhosis (13, 14).
In addition to its osmotic effects, albumin has an immunomodulatory effect (14–16) that is measurable by significantly reduced interleukine-6 (IL-6) response in high dose albumin treatment (14). Thus, albumin may improve survival and prevent complications in decompensated cirrhosis, and its immunomodulatory effects require further exploration as they relate to the prevention of acute-on-chronic liver failure (ACLF).
Both inflammation and oxidative stress are considered key elements in the pathology of cirrhosis. When the liver is injured, it may increase reactive oxygen and nitrogen species (ROS, RNS). These intermediates can induce pro-fibrogenic mechanisms. The oxidative stress causes injury by an alteration of DNA, proteins and lipids, resulting in activation of the hepatic stellate cells; hence one of the triggers of fibrogenesis also elicited by inflammatory pathways. Oxidative stress and inflammation are tightly related and can create a vicious cycle to aggravate liver injuries (17).
Multiple pathways are relevant and interesting when seeking to treat liver cirrhosis. In particular, the chronic and systemic inflammatory and oxidative mechanisms that mediate several complications in cirrhosis suggest that inflammatory cascades are possible targets for the treatment of cirrhosis. The available therapy is inadequate in treating fibrogenesis and liver tissue inflammation, and novel targets and therapies are wanted.
The present review aims to evaluate possible anti-inflammatory agents as potential drug candidates that may alleviate, cure or increase survival among patients with liver cirrhosis.
Methods
A search of the literature published during the last 10 years was conducted in PubMed and Medline. Titles and abstracts were searched for the following key terms in different combinations: “cirrhosis,” “liver cirrhosis,” “cohort,” “inflammation,” “anti-inflammatory,” “chronic liver disease,” “drugs,” “targets,” “cure.” The complete search strategy is described in Supplementary Table 1. Following agreement among the authors, specific searches were then carried out that include the following terms combined with “liver cirrhosis”:
“silymarin,” “anti-TNF-α,” “curcumin,” “faecal microbiota transplantation,” “enoxaparin,” “etanercept,” “artesunate,” “celecoxib,” “aspirin,” “kahweol,” “mitoquinone,” “glycyrrhizin arginine salt,” “pentoxifylline,” “statin,” “emricasan,” “lanifibranor,” “formyl peptide receptor 2 (WKYMVm),” “tanshinone.”
Inclusion criteria for the studies were their full text being in English, and their design being clinical trials, clinical studies, comparative studies, multi-centre studies, case reports, and observational studies. Interventional studies were considered regardless of whether they had a control group or were blinded. In addition, studies evaluating the safety, efficacy, and therapeutic mechanisms of pharmacological agents with anti-inflammatory effects in humans were included, and studies evaluating inflammatory mechanisms in rodent models were also assessed to support our understanding of immunological mechanisms.
Selected studies using rodent models were considered for inclusion, as the differentiation between fibrosis and cirrhosis differs markedly from that in humans. Hence, mentioning cirrhosis was a criterion in rodent studies. Cell model studies were considered relevant only when combined with human- or rodent studies fulfilling all other search criteria.
Studies with an exclusive focus on fibrosis, steatosis, steatohepatitis, and viral hepatitis without cirrhosis were excluded.
Results
Literature searches were conducted between the 6th of March and the 16th of May 2021. The initial search strategy resulted in 57,853 general hits, which were subsequently reduced to 1,337 drug search-related hits.
After excluding duplicates and irrelevant papers and following the above-stated in- and exclusion criteria, the abstracts of 351 publications were identified and screened for studies evaluating pharmacological agents' safety, efficacy, and therapeutic mechanisms with anti-inflammatory effects in cirrhosis.
Another 16 papers were found during a manual search of reference lists and bibliographies (Figure 1, Trial flow chart). The remaining 275 papers were excluded due to their primary focus on fibrosis, with no mentioning of cirrhosis or because of a lack of investigations into inflammatory or antioxidative pathways. Seventy-six publications were considered relevant to the research question as evaluated by the authors (SM, NK, and TMK). Of these, 27 studies explored the clinical effects of the included anti-inflammatory drugs in human studies without a particular focus on the anti-inflammatory markers, and these were excluded (see Supplementary Table 2).
Forty-two studies explored potential anti-inflammatory mechanisms of drugs in animal models, and seven studies explored these same drug mechanisms in humans.
Tables 1A,B lists the included studies.
Agents were assessed according to their pathway mechanisms.
Anti-inflammatory Mediators
Twelve different anti-inflammatory mediators acting on several pathways were evaluated in 30 animal studies and five human studies. Cytokines were most often used as a marker for inflammation. Cytokines are regulatory peptides released by activated cells and act as crucial mediators in immune and inflammatory disorders. Increasing evidence support a major role for several cytokines in liver diseases (Figure 2) (66).
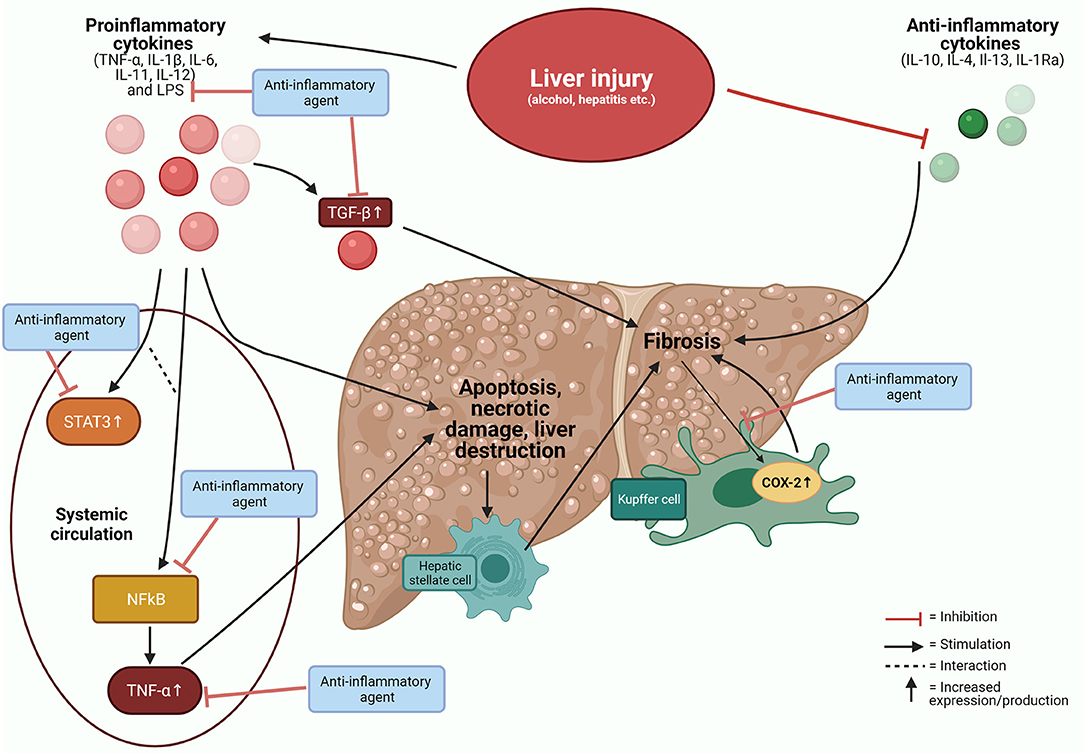
Figure 2. Cytokines in liver disease. Inflammatory responses on various liver injuries and potential targets for anti-inflammatory agents. COX-2, cyclooxygenase−2; IL, interleukin; LPS, lipopolysaccharide; NFkB, nuclear factor-kB; STAT3, signal transducer and activator 3; TGF, transforming growth factor; TNF, tumour necrosis factor.
The following agents are reported to interfere with the immune system, with potential beneficial effects:
Celecoxib (25–29, 67), aspirin (30, 31), etanercept (32), curcumin (33–39), kahweol (40, 41), pentoxifylline (42, 68), diosmin (42–44), glycyrrhizin arginine salt (45), statins (18, 19, 46–50), emricasan (20–22, 51, 69) and lanifibranor (52) and formayl receptor 2 agonist—WKYMVm (53).
In addition, 27 human studies with various methodologies investigated the mechanisms but did not report anti-inflammatory endpoints. These are listed in Supplementary Table 2.
Celecoxib
Celecoxib is a cyclooxygenase-2 (COX-2) inhibitor used in the treatment of arthritis (70, 71). COX-2, an enzyme expressed due to inflammation, is increased in inflammatory, vascular endothelial lining, and expressed by Kupffer cells in the cirrhotic liver (72, 73). Celecoxib has anti-inflammatory effects to relieve cirrhosis complications and reduce portal hypertension in several rat studies (25–29, 67) (Supplementary Table 3a). Celecoxib was administered to prevent cirrhosis in experimental animal models where cirrhosis was induced by peritoneal injections of thioacetamide (TAA). However, no human studies with anti-inflammatory endpoints have been carried out with celecoxib.
Aspirin
Aspirin is a COX-inhibitor that acts on the nuclear factor kappa B (NFkB), which transcript adhesion molecules in endothelial cells and vascular smooth muscle cells, which affect macrophage and T lymphocyte adherence (30). In addition, aspirin may enhance interferon-α-induced growth inhibition and apoptosis in HCC (74), but few studies have investigated aspirin as a single treatment for cirrhosis.
In rats with TAA-induced cirrhosis, aspirin markedly reduces fibrogenesis with a macroscopic and histologic improvement of the liver tissue compared to controls (30, 31).
While biomarkers of inflammation were not assessed in these studies (30), serum bilirubin levels were significantly lower in the aspirin-treated cirrhotic rats than in the untreated cirrhotic rats (31).
So far, no human studies of aspirin as a treatment of inflammation have investigated specific molecular or anti-inflammatory biomarkers or inflammation cascades. Clinical endpoints investigated are listed in Supplementary Table 2 (75–77).
Etanercept
TNF-α is a cytokine produced in immune cells, and it is shown that hepatic signalling through the TNF-R1-receptor is essential for liver regeneration (78). TNF-α activates the NFkB-pathway, which mediates protective and anti-apoptotic effects but also initiates transcription of inflammatory mediator genes (79). However, TNF-α is also a proinflammatory mediator capable of inducing apoptosis and liver destruction (79). Etanercept is a TNF-α antagonist and an approved drug for autoimmune diseases, e.g., inflammatory bowel disease and rheumatoid arthritis. A single rat study (32) found that TNF-α levels were neutralised combined with a significantly lower expression of TNF-R1 by etanercept exposure. Surprisingly, a retrospective study of patients with various immune-related diseases receiving TNF-α-inhibitors found an increased hazard ratio for developing cirrhosis. Immunological pathways were not further assessed, and its endpoints are listed in Supplementary Table 2 (80).
Curcumin
Curcumin is a derivative of turmeric, which appears to have anti-inflammatory, antioxidant, plus anticarcinogenic effects (81).
Curcumin has an increasing effect on the signalling molecules nuclear factor erythroid 2-related factor 2 (Nrf-2), Nrf2-NFkB (measured as mRNA) and protein expression (34), and a decrease in the cytokines TGF-1β and TNF-α and in IL-10 (33). In addition, curcumin reduces alanine aminotransferase (ALT) levels in hamsters and rats with CCl4-induced cirrhosis (33, 35).
Curcumin may also reduce the expression of α-smooth muscle actin (α-SMA), a phenotypic marker of HSC-activation, and COX-2 combined with lactulose (35). In addition curcumin increases SIRT3, a sirtuin with a pivotal role in fatty acid oxidation and reduction of cellular reactive oxygen species (ROS) in the liver, which is decreased in cirrhotic rats (36). Furthermore, mRNA expression of the signalling molecules AMPK, CPT-1A, IDH2, and MnSOD was increased by curcumin, indicating reduced oxidative stress.
Curcumin was found to reduce protein expressions of eNOS, COX-2, VEGF, p-VEGFR2, and p-Erk in cirrhotic rats (37). The impact of curcumin on inflammatory markers was investigated by Cai et al. (38) and by Hernández-Aquino et al. (39) (Supplementary Table 3b). Curcumin appeared to decrease the levels of LPS-TLR4-related downstream inflammatory cytokines in the liver, specifically, TNF-α, IL-1β, IL-6, and CINC-1/IL-8. The decreases were mediated by decreased LPS levels and innate inflammation in the curcumin-treated group, not due to decreased LPS absorption but enhanced LPS clearance and detoxification in the liver (38). In addition, restoration of MMP-9, MMP-2, MMP-13m NFkB, IL-1, IL-10, TGF-β, connective tissue growth factor (CTGF), collagen, α-SMA, and Smad3+7 was also induced by curcumin as well as a decrement in activated hepatic stellate cells (39).
Overall, curcumin seems to decrease inflammatory responses, ameliorate fibrosis and portal hypertension, and attenuate splanchnic hyperdynamic circulation at least partly by inducing vasoconstriction through inhibition of eNOS and decreasing mesenteric angiogenesis via VEGF blockade.
In humans, a recent double-blind placebo-controlled trial demonstrated the effects of curcumin vs. placebo on disease severity in cirrhosis. However, specific anti-inflammatory effects have not been addressed in humans, see Supplementary Table 2 (82).
Kahweol
The LPS-induced inflammatory response is a crucial driver in systemic inflammation, most likely caused by bacterial translocation of PAMPs and DAMPs from the gut into blood circulation (6). The LPS signal transducer activates NFkB. Signal transducer and activator 3 (STAT3) is another transcriptional factor involved in the NFkB-pathway. Inhibition of these two factors could reduce the inflammatory responses (83).
Kahweol is a coffee-specific compound of coffee beans that exhibits anticarcinogenic, anti-tumour progressive, and anti-inflammatory properties (84), probably via affection of the NFkB and STAT3 signalling.
Seo et al. investigated kahweol's antifibrotic and anti-inflammatory effect on mouse liver Kupffer cells and hepatocytes in vitro (40). Kahweol was found to limit the production of IL-1α, IL-1β, IL-6, and TNF-α were reduced. Furthermore, this inhibitory effect was associated with the downregulation of LPS-stimulated phosphor-NFkB and STAT3.
Arauz et al. (41) investigated the effects of coffee in rats with TAA-induced cirrhosis. Coffee prevented a weight loss and limited the increase of ALT, alkaline phosphatase (ALP), and gamma-glutamyl transpeptidase observed in cirrhotic controls. In addition, significant amelioration of the cytokines TGF-β, CTGF, IL-10, and MMP-13 and α-SMA spikes evolved when coffee was administered to the rats. Thus, the action mechanisms are probably associated with antioxidant properties, mainly with coffee's ability to block the elevation of the profibrogenic cytokine (TGF-β) and the downstream effector CTGF.
No intervention studies in humans have yet explored the anti-inflammatory effects of coffee derivatives in patients with cirrhosis. However, one epidemiologic study is listed in Supplementary Table 2.
Pentoxifylline and Diosmin
Pentoxifylline is a non-selective phosphodiesterase inhibitor, which exhibits vasodilator activity on peripheral hepatic blood vessels (85). In addition, it exerts an anti-inflammatory regulation by affecting TGF-β- and tissue inhibitor metalloproteinase-1 (TIMP-1) expressions. Beneficial effects in humans with advanced liver disease have been described (68), and regulating effects of the hepatic stellate cell activity, is suggested to be related to the Hedgehog signalling pathway (86).
Diosmin is a natural flavone reported to prevent hepatic injury through inhibition of NFkB activation (42, 87). In bile duct ligated(BDL)-induced cirrhotic rats, pentoxifylline and diosmin have increased survival (42). A healing effect on the fibrotic markers HYP and TGF-β, and the oxidative markers malondialdehyde (MDA), SOD (superoxide dismutase), glutathione reductase (GSH), and nicotinamide adenine dinucleotide phosphate (NADPH) oxidase activity favoured both diosmin and pentoxifylline; see Supplementary Table 3c.
Diosmin has been investigated as a single treatment and in combination with sildenafil (43). Findings about its anti-inflammatory and antioxidant effects are described in Supplementary Table 3c. A rodent study (44) confirmed findings of regulation of TNF-α and NFkB activation in rats treated with ethanol and diosmin concomitant for 4 weeks. TNF-α was significantly elevated by ethanol and remitted by the concomitant addition of diosmin. NFkB was investigated by immunohistochemical staining, and the expression was markedly suppressed in diosmin-treated rat groups.
In a clinical trial, 329 patients with cirrhosis were randomised to treatment with pentoxifylline or placebo for 6 months (68). Pentoxifylline lowered complication rates of bacterial infections, renal insufficiency, hepatic encephalopathy, and gastrointestinal bleeding. Higher TNF-α baseline levels were associated with the development of complications in the absence of pentoxifylline.
Glycyrrhizin Arginine Salt
Glycyrrhizin is the primary active constituent of liquorice root. Liquorice has anti-inflammatory, spasmolytic, laxative, anti-depressive, anti-ulcer, and anti-diabetic effects (88). The statement is supported by a rodent study in which glycyrrhizin combined with arginine seems to protect against hyperammonaemia and hepatic encephalopathy (45).
The therapeutic effects of glycyrrhizin and arginine also change cytokine levels (TGF-β1 and TNF-α), antibodies against matrix metalloproteinases, and biochemical markers of liver function. In this study, significant changes were found to recommend glycyrrhizin arginine salt treatment. Results for its anti-inflammatory effects are listed in Supplementary Table 3d.
Statins
Statins have proved antioxidative, antiproliferative, and anti-inflammatory properties and a capacity to improve endothelial function and stimulate neoangiogenesis (89, 90). Statins decrease leukocyte adhesion to endothelial and epithelial cells by inhibiting expression and binding of the integrin LFA-1 and the intercellular adhesion molecule 1 (ICAM-1). Statins also decrease NFkB production, and hence the release of proinflammatory cytokines such as TNF-α and IL-6. This chain of action blocks critical proteins required to form lipid rafts and immune cell activation and growth. They also reduce the levels of oxidative stress (91).
Studies in rats with experimental-induced cirrhosis have shown that statins may prevent LPS-induced ACLF-derived complications and prolong survival. Moreover, statins increase the hepatic sinusoidal function, protect against endothelial dysfunction and the harmful effects of hypovolemic insults. Finally, statins normalise inflammatory markers during critical events such as ACLF and hypovolemia (46, 47) (see Supplementary Table 3e). A significant effect of simvastatin, when combined with bone-marrow-derived mesenchymal stem cells, has been demonstrated with amelioration of fibrosis (50) (see Supplementary Table 3e). Atorvastatin also reduces portal pressure in CCl4-cirrhotic rats (48). In human cirrhotic liver samples, Sonic hedgehog (Shh) and Glioma-associated oncogene family zinc finger-2 (Gli-2) mRNA levels, as well as protein expressions, increases (48). Atorvastatin treatment significantly downregulated the hedgehog components Shh and Gli-2 in the BDL and CCl4-cirrhotic models. Likewise, mRNA levels of α-SMA, collagen-1, and vimentin decreased after atorvastatin treatment.
In contrast, one rat study did not find a significant amelioration of cirrhosis on treatment with atorvastatin or rosuvastatin (49).
Simvastatin may increase the hepato-splanchnic output of nitric oxide products in patients with cirrhosis, thereby improving portal hypertension (18).
Randomised clinical trials have investigated beneficial effects on clinical outcomes such as liver function, rebleeding from oesophageal varices, and survival (92–94), and one ongoing multi-centre trial is also prospectively investigating the potential reduction of hepatic decompensation (19). However, no human studies have explored anti-inflammatory mechanisms in detail; hence, the therapeutic effects of statins are not yet completely understood [for clinical endpoints in human studies (90, 92–104) see Supplementary Table 2].
Emricasan
Emricasan is an oral pan-caspase inhibitor with alleviating impact on apoptosis, inflammation, and fibrosis in animal models of liver injury.
Studies have demonstrated how emricasan can inhibit hepatic cell death with reductions in caspase-3-activity in CCl4-cirrhotic rats, while reducing portal hypertension and hepatic microvascular dysfunction in rats with advanced cirrhosis is also described (51). In addition, three human studies have explored the clinical effects of emricasan (20–22).
Frenette et al. (20) administered emricasan 25 mg in a randomised, double-blinded, placebo-controlled trial. Emricasan reduced cleaved keratin-18 (a marker of apoptosis) relative to placebo, although insignificant, but caspase 3/7 and flCK-18 levels reduced significantly. Garcia-Tsao et al. (21) likewise found reductions in cleaved cytokeratin 18 and caspase-3/7 after 28 days of treatment with emricasan in 22 cirrhotic patients. Supplementary Table 3 lists anti-inflammatory marker results for the rodent studies.
A recent clinical trial concerning NASH-related cirrhosis by the same authors (22) showed the same biomarker patterns described above. However, the clinical effects on liver biochemistry and portal hypertension were not observed in patients with decompensated NASH-related cirrhosis (69) (see Supplementary Table 2).
Lanifibranor
Peroxisome proliferator-activated receptors (PPARs) are present in mammals in three isoforms, and all isoforms have a role in maintaining liver function (105). The pan-PPAR agonist lanifibranor has shown potential to alleviate models of mild liver injury and non-alcoholic fatty liver disease. We found one study of the effects of lanifibranor on cirrhotic rats and on cirrhotic human hepatic cells in vitro. Lanifibranor ameliorated fibrosis and portal hypertension in the rats in addition to significant anti-inflammatory effects (Supplementary Table 3f) and showed promising results in human hepatic cells. However, no human clinical trials were found.
Formyl Peptide Receptor 2 Agonist—(WKYMVm)
Hexapeptide WKYMVm (Trp-Lys-Tyr-Met-Val-D-Met) is a ligand of the formyl peptide receptor 2. It exhibits anti-inflammatory and angiogenic properties in multiple disease models. The WKYMVm peptide improves vascular remodelling and inhibits fibrosis in a rat model of hepatic failure (53) (See Supplementary Table 3g). Furthermore, WKYMVm enhances hepatic function by upregulating the expression of hepatic function markers. These data suggest that the WKYMVm peptide modulates liver function and vascular regeneration in rodent hepatic failure. No human trials are described.
Antioxidants
Seventeen animal studies investigated the effects of five different antioxidant mediators, three of which were investigated in human studies with clinical endpoints): Curcumin (33–38), pentoxifylline (42, 68), and diosmin (42–44) are described above. Mitoquinone (54, 55) and silymarin (56–60, 106) are described below.
Antioxidant agents seem to attenuate hepatic fibrosis in rodent models (107). The mechanism is partly due to the influence on the activation of hepatic stellate cells, which induce extracellular matrix deposition (Figure 3). Several agents have supposed antioxidant effects demonstrated by measurements of reactants as MDA, NADPH oxidase, Nrf2, Keap 1, NFkB, and IkB. NADPH oxidase is highly expressed by Kupffer cells and generates high amounts of ROS during early liver injury. In addition, hepatic stellate cells also seem to express NADPH—generating ROS, which mediates fibrogenic factors (107).
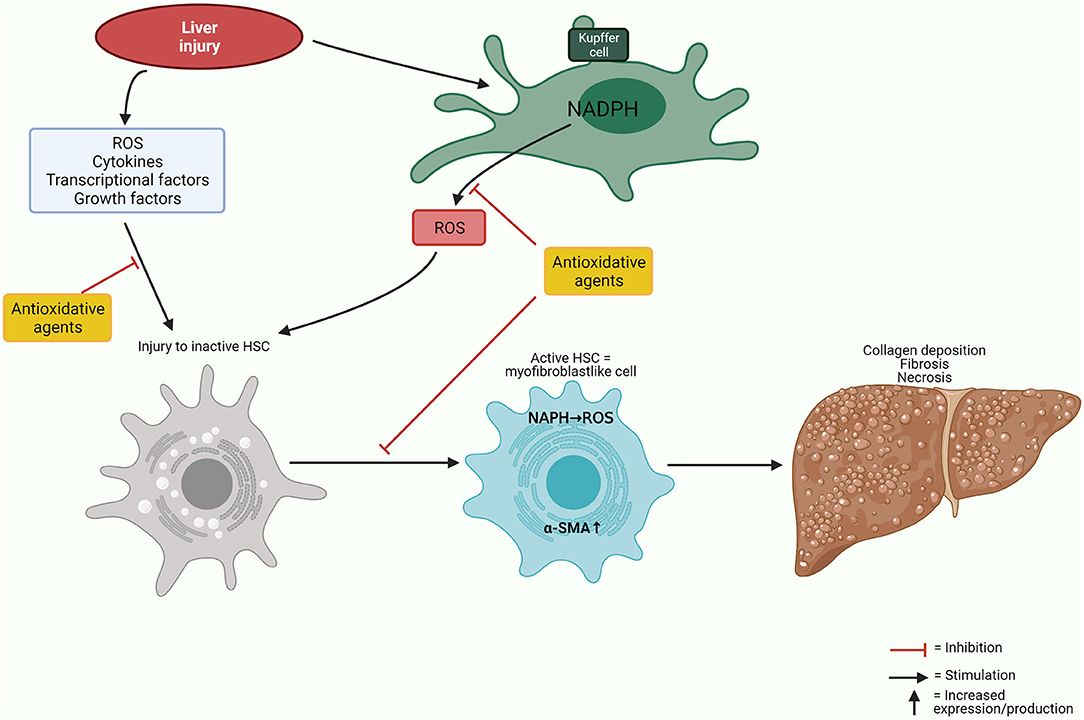
Figure 3. Antioxidative mechanisms. Simplified illustration of oxidative and antioxidative mechanisms affecting liver cells. α-SMA, α-smooth muscle actin; HSC, hepatic stellate cell; NADPH, nicotinamide adenine dinucleotide phosphate; ROS, reactive oxygen species.
Mitoquinone
Mitochondrial dysfunction appears to play a crucial role in the development and progression of liver cirrhosis. Cirrhotic livers exhibit increased ROS produced by mitochondria. Mitoquinone is a mitochondria-targeted antioxidant, which might relieve the damaging effects of ROS within cirrhotic livers (54, 55). Two rat studies have assessed the effects of treatment with mitoquinone. Thus, Vilaseca et al. (54) found a relieving effect on portal hypertension in rats, as well as on fibrosis and oxidative markers.
Mitochondrial superoxide content was significantly higher in hepatic stellate cells and hepatocytes from cirrhotic rats but not in sinusoidal- or Kupffer cells compared to non-cirrhotic rats, and this effect appeared to be dose-dependent. Similar effects on HSC activity were found in human liver cells exposed to mitoquinone.
Mitoquinone also reduced oxidative stress and reduced portal pressure and intrahepatic vascular resistance in rats with CCl4-induced cirrhosis. Mitoquinone resulted in a significant reduction in hepatic fibrosis, which points to a potential clinical value of this drug. The activity of the hepatic stellate cells was assessed by the expression of profibrogenic genes and α-SMA, and both markers reduced significantly in cirrhotic rats.
Finally, inflammatory markers in in vivo models were measured, and iNOS, IL-6, and IL-1β were all reduced significantly.
In another study of BDL rats, Turkseven et al. (55) also investigated the effects of mitoquinone. Treatment with mitoquinone prevented inflammation, hepatocyte necrosis, and progression of fibrosis. Initially, bile-duct ligation of the rats led to increased gene expression (Qr-PCR) of inflammatory and oxidant markers, and these responses were reduced by mitoquinone. Collagen type col1α1, TGF-β, TNF-α, IL-6, IL-1β, and levels of circulatory TNF-α were all reduced. Furthermore, mitoquinone reduced the protein carbonylation, an indicator of irreversible oxidative protein modification, in cirrhotic rats. Mitoquinone normalised the gene expression of the mitochondrial antioxidant Mn-SOD, Cu/ZnSOD, and catalase impaired by cirrhosis.
Parkin protein expression in mitochondria is an indicator of the removal of dysfunctional mitochondria by autophagy. Parkin protein expression decreases in cirrhosis but increases in rats treated with mitoquinone (55).
Mitoquinone seems to possess both anti-inflammatory and antioxidant effects in human cells and rodent models, but no human studies have yet verified these effects.
Silymarin
Silymarin is an extract of the plant Silybum marianum (milk thistle), the main compound being silybin. Silymarin has a low bioavailability and lack solubility in water. Silybin acts by turning off proinflammatory signals derived from NFkB-activation (which is involved in the induction of TNF-α, IL-1, IL-6, and GM-CSF) and induces apoptosis. Silymarin's antioxidant activity is related to its free radical-scavenging and lipid peroxidation inhibition, as demonstrated in vivo and in vitro (108).
We identified five rodent studies that have assessed the effects of silymarin in cirrhosis models.
Ali et al. (58) investigated the modulatory effects of curcumin, silybin-phytosome, and alpha-R-lipoic acid in rats with TAA-induced cirrhosis. TAA was given at the same time as the intervention. Glutathione depletion, collagen deposition, matrix metalloproteinase-2 activity, TGF-β1 levels and heat shock protein-47 gene expressions- all factors believed to be involved in the development of cirrhosis-, were partially blocked by the combination therapy with curcumin, silybin-phytosome, and alpha-R-lipoic acid. Thus, therapy increased ROS generation and inhibited the activation of hepatic stellate cells, thereby preventing liver cirrhosis.
Zaidi et al. (56) also evaluated the effects of silymarin on rats with TAA-induced cirrhosis. Antioxidant activity was reduced as superoxide dismutase (SOD) and GSH were low, and MDA (measure of lipid peroxidation) and catalase were increased before treatment was initiated. Conversely, silymarin restored SOD and GSH, MDA, and catalase activity.
A possible synergistic effect between silymarin and lactulose has been investigated in a cirrhotic rat model (57) with no significant difference found between treatment groups according to their necro-inflammatory scores.
A combination of silymarin and the amino acid taurine was assessed in a study on CCl4-induced cirrhosis (59). Silymarin alone and silymarin with taurine restored the TBARS (thiobarbituric acid reactive substances) levels, and the combination treatment significantly reduced NO levels and NOS activity. However, activities of SOD, glutathione peroxidase (GPx), and glutathione reductase (GR) increased significantly in all treatment groups. In addition, glutathione-S-transferase (GST) and reduced glutathione (GSH) increased in rats treated with silymarin alone or the combination treatment.
The cytokines TNF-α, TGF-β1, IL-6, and the proteins leptin and resistin elevated in the cirrhotic model, while adiponectin reduced. All three treatments reduced TGF-β1, IL-6, and leptin, but only taurine and the combination taurine and silymarin reduced TNF-α and resistin.
Finally, a combination of bone-marrow-derived stromal cells and silymarin ameliorated liver tissue damage in a CCl4-cirrhotic rat model through immunoregulatory activities. However, antioxidative markers were not investigated in this study (60).
Only a single cohort study of silymarin has been carried out in humans. Fathalah et al. (106) investigated the effects of high-dose silymarin in decompensated chronic hepatitis C virus (HCV)-cirrhotic patients. The main results improved biochemical liver parameters and Child-Pugh score; however, no oxidative markers were investigated (see Supplementary Table 2).
Gut Microflora and the LPS Pathway
Gut dysbiosis with translocation of bacteria and the bacterial product might play a role in the development of complications of cirrhosis. Alleviation of the dysbiosis in the gut flora of patients with cirrhosis, and the effects on LPS, supports that counteracting anti-inflammatory mechanisms are beneficial in cirrhosis.
Our search resulted in four animal studies, two human studies reporting effects on inflammatory markers, and two human studies where only clinical endpoints were considered. Thus, we found three different gut microbial modulation therapies, namely Faecal microbiota transplantation (23, 24, 62, 109), kahweol (40, 41) as described earlier, and artesunate (63).
Faecal Microbiota Transplantation (FMT)
Among pertinent mechanisms, an increase in LPS leads to hepatocyte damage, which stimulates hepatic macrophages and increases the release of IL-1, IL-6, and TNF-α. Several pathways are involved in the promotion or counteraction of chronic inflammation (40). For example, kahweol affects the LPS pathway in the gut resulting in anti-inflammatory effects (described above). Liu et al. explored the effects faecal microbiota transplantation (FMT) from humans to germ-free and conventional mice (62). They found reduced neuroinflammation and microglial activation and dysbiosis 15 days after FMT exposure, whereas liver inflammation was unaffected. Higher degrees of neuroinflammation in mice regardless of their cirrhosis state was found with faecal microbial colonisation from humans with cirrhosis as compared with mice exposed to colonisation from healthy humans. Bajaj et al. investigated the safety of FMT capsules in patients with cirrhosis and recurrent HE in a Phase 1 randomised, placebo-controlled trial (23). FMT improved duodenal mucosal diversity, dysbiosis, and expression of duodenal antimicrobial peptide (AMP) and reduced lipopolysaccharide-binding protein (LBP). Subsequently, a trial was constructed based on the same cohorts elucidating the effects on inflammatory markers: IL-6 and LPS-binding protein, and bile acids in serum (24). Four weeks of FMT decreased levels of serum IL-6 and LBP compared to the placebo group. In the FMT group, greater deconjugation and secondary bile acid formation were found. In an ongoing study, Woodhouse et al. (109) currently assess if FMT in patients with advanced cirrhosis is effective, feasible, and safe.
Artesunate
Artesunate is an extract of the Chinese herb “Artemisia annua,” which has historically been used as an antimalarial drug. It is assumed to affect the pathological bacterial translocation (63), which is thought to be the key driver of spontaneous infection in patients with cirrhosis (110). For example, prophylactic antibacterial treatment is often indicated in patients with ascites and risk of spontaneous bacterial peritonitis. A single study assessed the effects of artesunate in rats with CCl4-induced cirrhosis (63). Thus, artesunate decreased IL-6 and TNF-α in the cirrhotic liver at week 4, 6, and 8, indicating an effect on inflammatory responses. Microbial diversity in the artesunate group, as compared to controls was increased at week 4 and reduced at weeks 6 and 8. Bacterial genomic DNA products reappeared in rats treated with artesunate after 4 weeks, unlike in the cirrhotic rats not treated with artesunate. No bacteria were detected in the blood in either group. Thus, artesunate decreased the occurrence of bacterial translocation significantly.
No human studies have yet been conducted, but the primary impression of the effects of artesunate is promising according to alleviating the inflammatory factors and the dysbiosis of gut microbiota in cirrhosis.
Deactivation of Hepatic Stellate Cells
Several of the therapeutic agents described are hypothesised to have multiple impacts on different homeostatic and pathophysiological pathways. For example, enoxaparin (30, 31, 64, 65, 111) and tanshinone (61) may have effects that have not yet been classified as direct antifibrotic mechanisms and do not fit into a concrete anti-inflammatory or antioxidative mode of action.
Enoxaparin
Enoxaparin has both anticoagulant and antithrombotic effects (112). A rat experimental study observed a reduction of proliferation and activation of hepatic stellate cells (30). In addition, it has been demonstrated that patients with cirrhosis more frequently exhibit a prothrombotic state than a hypocoagulative state (113, 114).
Enoxaparin has been proven to reduce portal pressure in cirrhotic rats, implying effects beyond the anti-thrombotic (65). Short-term treatment of cirrhotic rats with enoxaparin showed a significant reduction of superoxide content, α-SMA, and mRNA of pro-collagen I and liver fibrosis. In addition, the oxidative stress levels were lower, and fibrosis reduced by 25% after enoxaparin treatment.
However, Fortea et al. (64) did not find an amelioration of fibrosis, biochemical parameters, hepatic endothelial dysfunction, or portal hypertension after enoxaparin treatment of cirrhotic rats. On the contrary, the therapeutic dose of enoxaparin did decrease survival in rats with CCl4-induced cirrhosis. Enoxaparin as a preventive therapy for portal venous thrombosis in patients with Child-Pugh B-C cirrhosis has been suggested by the authors of one study (111), favouring enoxaparin compared to no treatment. It was found safe and preventive for thrombosis for 34 patients treated for 48 weeks. In addition, the frequency of decompensation reduced, and survival increased in the enoxaparin group.
Tanshinone
Salvia miltiorrhiza (S. miltiorrhiza) is a Chinese herb comprising multiple compounds. Tanshinone is extracted from S. miltiorrhiza and is described as a natural antioxidant with hepatoprotective, antifibrotic, and anticancerogenic effects. In addition, it is supposed to induce stem cell proliferation and differentiation (115). A single rat study investigated the effects in a cirrhotic model (61), where tanshinone improved the histological injury, serological tests, and increased expression of markers indicating newly proliferated stem cells. These effects appeared to be caused by promoting proliferation and differentiation of endogenous liver stem cells. No human trials were found.
Discussion
In the present review, we have identified and explored possible anti-inflammatory and antioxidant agents as potential drug candidates to interfere with the fibrogenesis processes and thereby alleviate the development and perpetuation of complications of cirrhosis. In cirrhotic rodent models we have found promising indices of beneficial anti-inflammatory and antioxidative effects of the COX-2 inhibitor celecoxib, aspirin, curcumin, kahweol, pentoxifylline, diosmin, statins, emricasan, and silymarin. Few indices of effects of etanercept, glycyrrhizin arginine salt, and mitoquinone were found. In addition, FMT is a growing field with the potential to alleviate cirrhosis by a beneficial regulation of the gut flora.
The main limitation of the present review is the lack of human studies assessing anti-inflammatory agents in cirrhosis. The lack makes it difficult to assess the clinical efficacy of the agents discussed and to compare the effects between different studies. The literature offers many experimental studies in rodents where multiple beneficial effects on cirrhosis regarding fibrosis are reported (see Supplementary Table 3). However, extrapolating experimental animal studies into a clinically relevant setting is problematic. Nevertheless, several different agents were identified, and many of these showed potential curing or relieving mechanisms and effects in the applied models. However, most of the rodent studies are not comparable since especially the method of inducing cirrhosis differs. The procedures, the duration, and the timing of adding the experimental agents differ vividly.
There are important conflicting results for enoxaparin, statins, and etanercept. In particular, one human study investigating etanercept found significant harmful effects (80). One rat study of statins (49) and enoxaparin (64) showed no intervention effects. Curcumin seems promising in both rodent and human clinical trials. Anti-inflammatory and antifibrotic effects, as well as a positive impact on portal pressure and hemodynamic in rodents combined with favourable clinical outcome in cirrhotic patients, indicates a convincing potential (33–38, 82). Curcumin also has potential effects on quality of life (116). Silymarin is also studied in a different setting and shows potential anti-inflammatory and antioxidative effects in rodents and clinical improvement in patients. Emricasan showed promising alleviating effects in both rodent and human studies on several parameters. Promising clinical effects (20–22) are shown, but only caspase and flCK-18 are measured as anti-inflammatory markers. Hence, the mechanisms are still largely unexplored, and future studies with inflammatory and clinical endpoints are warranted.
Several retrospective studies have suggested that statins can improve mortality in cirrhosis (90, 95, 103). Clinical studies of the anti-portal hypertensive effects of statins have provided encouraging results in recently published trials (93, 97, 102, 117), while other studies have reported a reduced risk of decompensation and death (89, 90, 92, 94), and that statins might reduce the risk of infections in patients with cirrhosis (96). One study also described improved survival during infections among cirrhotic patients undergoing continuing treatment with statins (104). Human studies exploring anti-inflammatory mechanisms in cirrhosis should be highly encouraged.
The anti-inflammatory effects of statins seem to be steps ahead of other agents concerning testing in human trials, mainly due to the similar effect of statins on portal hypertension (93, 97, 102, 117). Novel research implies the anti-inflammatory effects of statins as key drives in lowering the portal pressure, but the immunological impact needs further exploration in humans (89, 91).
Dysbiosis in the gut flora is suspected as a precipitating factor in cirrhotic patients with infections who need antibiotic treatment. FMT could prove to be a valuable “post-treatment” after antibiotic exposure, restoring the potentially harmful effect antibiotics have on microbial diversity and function (118). Initial studies have focused on safety and organ function outcomes in cirrhosis (23), relevant in a complex disease entity. Studies on FMT without prior antibiotics are needed to assess the impact of gut dysbiosis and the inhibition or alleviation of inflammation in decompensated cirrhosis. Future studies are awaited (109) in the search for preventive and treating agents in cirrhosis.
Very few side effects are reported for the main part of the described agents, and safety deserves a primary focus in future investigations of anti-inflammatory agents. Only two safety studies regarding celecoxib are conducted (119, 120).
Real-life clinical trials exploring anti-inflammatory interventions and their safety in patients with liver cirrhosis are notably missing in the literature. Furthermore, data on the combination of anti-inflammatory or antioxidative markers and clinical outcomes are scarce. Human randomised clinical trials, preferably placebo-controlled, are the next step toward clinical application of anti-inflammatory agents. As most inflammation markers are easy to sample by blood tests, this should be possible and feasible to add to clinical protocols.
Autologous macrophage therapy is another promising treatment that seems safe (121); the effects of which are yet to be fully investigated. This is also the case for other autologous cell transplantation, such as mesenchymal stromal cell therapy (122).
In conclusion, we recommend further study of the inflammatory, oxidative, microbiological, and immunological mechanisms and pathways responsible for disease progression in cirrhosis. Known and novel compounds with potential healing effects in cirrhosis are identified and require further exploration. In general, the literature encloses very few human clinical trials on the aspects, and the need for studies is growing. Future studies should include anti-inflammatory biomarkers and clinical endpoints in combination to assess potential immunological agents in the treatment of cirrhosis.
Data Availability Statement
The original contributions presented in the study are included in the article/Supplementary Material, further inquiries can be directed to the corresponding author/s.
Author Contributions
TK, NK, and SM contributed to conception and design of the review. TK performed the literature searches and wrote the first draught of the manuscript. NK and TK assessed the literature searches. All authors critically revised the litterature and contributed to manuscript revision, read, and approved the submitted version.
Conflict of Interest
The authors declare that the research was conducted in the absence of any commercial or financial relationships that could be construed as a potential conflict of interest.
Publisher's Note
All claims expressed in this article are solely those of the authors and do not necessarily represent those of their affiliated organizations, or those of the publisher, the editors and the reviewers. Any product that may be evaluated in this article, or claim that may be made by its manufacturer, is not guaranteed or endorsed by the publisher.
Supplementary Material
The Supplementary Material for this article can be found online at: https://www.frontiersin.org/articles/10.3389/fmed.2021.718896/full#supplementary-material
Abbreviations
ACLF, acute on chronic liver failure; α-SMA, α-smooth muscle actin; ALT, alanine aminotransferase; ALP, alkaline phosphatase; AMP, antimicrobial peptide; AST, aspartate aminotransferase; BDL, bile duct ligation; CCl4, carbon tetrachloride; cCK18, cleaved cytokeratine-18; COX, cyclooxygenase; CTGF, connective tissue growth factor; eNOS, endothelial nitric oxide synthase; flCK18, full-length cytokeratine 18; FMT, faecal microbiota transplantation; GPx, glutathione peroxidase; GR, glutathione reductase; GSH, glutathione reductase/reduced glutathione; GST, glutathione-S-transferase; HBV, Hepatitis B virus; HCC, hepatocellular carcinoma; HCV, Hepatitis C virus; HE, hepatic encephalopathy; HRS, hepatorenal syndrome; HSC, hepatic stellate cells; HVPG, hepatic venous pressure gradient; HYP, hydroxyproline; ICAM-1, intercellular adhesion molecule 1; IL-6, interleukine-6; Keap-1, Kelch-like ECH-associated protein 1; KLF2, Krüppel-like factor 2; LDL, low density lipoprotein; LFA-1, lymphocyte function-associated antigen 1; LPS, lipopolysaccharide; MDA, malondialdehyde, measure of lipid peroxidation; MSCs, mesenchymal stem cells; NADPH, nicotinamide adenine dinucleotide phosphate; NFkB, nuclear factor-kB; NO, nitrogen oxide; NOS, nitrogen oxygen synthase; Nrf2, Nuclear factor erythroid 2-realted factor 2; PARs, protease activated receptors; PDGF, platelet derived growth factor; PPARα, peroxisome proliferator-activated receptor-α; RCT, randomised controlled trial; ROS, reactive oxygen species; SBP, spontaneous bacterial peritonitis; SOD, superoxide dismutase; STAT3, signal transducer and activator 3; Supp., supplementary; TAA, thioacetamide; TBARS, thiobarbituric acid reactive substances; TGF-β, transforming growth factor-β; TNF-α, tumour necrosis factor-α; VEGF, vascular endothelial growth factor; WKYMVm, a hexapeptide.
References
1. Smith A, Baumgartner K, Bositis C. Cirrhosis: diagnosis and management. Am Fam Physician. (2019) 100:759–70.
2. Zhang CY, Yuan WG, He P, Lei JH, Wang CX. Liver fibrosis and hepatic stellate cells: etiology, pathological hallmarks and therapeutic targets. World J Gastroenterol. (2016) 22:10512–22. doi: 10.3748/wjg.v22.i48.10512
3. Park BJ, Lee YJ, Lee HR. Chronic liver inflammation: clinical implications beyond alcoholic liver disease. World J Gastroenterol. (2014) 20:2168–75. doi: 10.3748/wjg.v20.i9.2168
4. Albillos A, Lario M, Álvarez-Mon M. Cirrhosis-associated immune dysfunction: distinctive features and clinical relevance. J Hepatol. (2014) 61:1385–96. doi: 10.1016/j.jhep.2014.08.010
5. Koyama Y, Brenner DA. Liver inflammation and fibrosis. J Clin Invest. (2017) 127:55–64. doi: 10.1172/JCI88881
6. Simbrunner B, Mandorfer M, Trauner M, Reiberger T. Gut-liver axis signaling in portal hypertension. World J Gastroenterol. (2019) 25:5897–917. doi: 10.3748/wjg.v25.i39.5897
7. Reiberger T, Mandorfer M. Beta adrenergic blockade and decompensated cirrhosis. J Hepatol. (2017) 66:849–59. doi: 10.1016/j.jhep.2016.11.001
8. Kockerling D, Nathwani R, Forlano R, Manousou P, Mullish BH, Dhar A. Current and future pharmacological therapies for managing cirrhosis and its complications. World J Gastroenterol. (2019) 25:888–908. doi: 10.3748/wjg.v25.i8.888
9. Angeli P, Bernardi M, Villanueva C, Francoz C, Mookerjee RP, Trebicka J, et al. EASL clinical practice guidelines for the management of patients with decompensated cirrhosis. J Hepatol. (2018) 69:406–60. doi: 10.1016/j.jhep.2018.03.024
10. Thiele M, Albillos A, Abazi R, Wiest R, Gluud LL, Krag A. Non-selective beta-blockers may reduce risk of hepatocellular carcinoma: a meta-analysis of randomized trials. Liver Int. (2015) 35:2009–16. doi: 10.1111/liv.12782
11. Thiele M, Wiest R, Gluud LL, Albillos A, Krag A. Can non-selective beta-blockers prevent hepatocellular carcinoma in patients with cirrhosis? Med Hypotheses. (2013) 81:871–4. doi: 10.1016/j.mehy.2013.08.026
12. Pose E, Cardenas A. Translating our current understanding of ascites management into new therapies for patients with cirrhosis and fluid retention. Dig Dis. (2017) 35:402–10. doi: 10.1159/000456595
13. Caraceni P, Riggio O, Angeli P, Alessandria C, Neri S, Foschi FG, et al. Long-term albumin administration in decompensated cirrhosis (ANSWER): an open-label randomised trial. Lancet. (2018) 391:2417–29. doi: 10.1016/S0140-6736(18)30840-7
14. Fernández J, Clària J, Amorós A, Aguilar F, Castro M, Casulleras M, et al. Effects of albumin treatment on systemic and portal hemodynamics and systemic inflammation in patients with decompensated cirrhosis. Gastroenterology. (2019) 157:149–62. doi: 10.1053/j.gastro.2019.03.021
15. Wilde B, Katsounas A. Immune dysfunction and albumin-related immunity in liver cirrhosis. Mediators Inflamm. (2019) 2019:7537649. doi: 10.1155/2019/7537649
16. Bernardi M, Angeli P, Claria J, Moreau R, Gines P, Jalan R, et al. Albumin in decompensated cirrhosis: new concepts and perspectives. Gut. (2020) 69:1127–38. doi: 10.1136/gutjnl-2019-318843
17. Li S, Hong M, Tan HY, Wang N, Feng Y. Insights into the role and interdependence of oxidative stress and inflammation in liver diseases. Oxid Med Cell Longev. (2016) 2016:4234061. doi: 10.1155/2016/4234061
18. Zafra C, Abraldes JG, Turnes J, Berzigotti A, Fernández M, García-Pagán JC, et al. Simvastatin enhances hepatic nitric oxide production and decreases the hepatic vascular tone in patients with cirrhosis. Gastroenterology. (2004) 126:749–55. doi: 10.1053/j.gastro.2003.12.007
19. Kaplan DE, Mehta R, Garcia-Tsao G, Albrecht J, Aytaman A, Baffy G, et al. SACRED: Effect of simvastatin on hepatic decompensation and death in subjects with high-risk compensated cirrhosis: statins and cirrhosis: reducing events of decompensation. Contemp Clin Trials. (2021) 104:106367. doi: 10.1016/j.cct.2021.106367
20. Frenette CT, Morelli G, Shiffman ML, Frederick RT, Rubin RA, Fallon MB, et al. Emricasan improves liver function in patients with cirrhosis and high model for end-stage liver disease scores compared with placebo. Clin Gastroenterol Hepatol. (2019) 17:774–83.e4. doi: 10.1016/j.cgh.2018.06.012
21. Garcia-Tsao G, Fuchs M, Shiffman M, Borg BB, Pyrsopoulos N, Shetty K, et al. Emricasan (IDN-6556) lowers portal pressure in patients with compensated cirrhosis and severe portal hypertension. Hepatology. (2019) 69:717–28. doi: 10.1002/hep.30199
22. Garcia-Tsao G, Bosch J, Kayali Z, Harrison SA, Abdelmalek MF, Lawitz E, et al. Randomized placebo-controlled trial of emricasan for non-alcoholic steatohepatitis-related cirrhosis with severe portal hypertension. J Hepatol. (2020) 72:885–95. doi: 10.1016/j.jhep.2019.12.010
23. Bajaj JS, Salzman NH, Acharya C, Sterling RK, White MB, Gavis EA, et al. Fecal microbial transplant capsules are safe in hepatic encephalopathy: a phase 1, randomized, placebo-controlled trial. Hepatology. (2019) 70:1690–703. doi: 10.1002/hep.30690
24. Bajaj JS, Salzman N, Acharya C, Takei H, Kakiyama G, Fagan A, et al. Microbial functional change is linked with clinical outcomes after capsular fecal transplant in cirrhosis. JCI Insight. (2019) 4:e133410. doi: 10.1172/jci.insight.133410
25. Gao JH, Wen SL, Tong H, Wang CH, Yang WJ, Tang SH, et al. Inhibition of cyclooxygenase-2 alleviates liver cirrhosis via improvement of the dysfunctional gut-liver axis in rats. Am J Physiol - Gastrointest Liver Physiol. (2016) 310:G962–72. doi: 10.1152/ajpgi.00428.2015
26. Gao JH, Wen SL, Yang WJ, Lu YY, Tong H, Huang ZY, et al. Celecoxib ameliorates portal hypertension of the cirrhotic rats through the dual inhibitory effects on the intrahepatic fibrosis and angiogenesis. PLoS ONE. (2013) 8:e0146617. doi: 10.1371/journal.pone.0069309
27. Wen SL, Gao JH, Yang WJ, Lu YY, Tong H, Huang ZY, et al. Celecoxib attenuates hepatic cirrhosis through inhibition of epithelial-to-mesenchymal transition of hepatocytes. J Gastroenterol Hepatol. (2014) 29:1932–42. doi: 10.1111/jgh.12641
28. Su W, Tai Y, Tang SH, Ye YT, Zhao C, Gao JH, et al. Celecoxib attenuates hepatocyte apoptosis by inhibiting endoplasmic reticulum stress in thioacetamide-induced cirrhotic rats. World J Gastroenterol. (2020) 26:4094–107. doi: 10.3748/wjg.v26.i28.4094
29. Tang S, Huang Z, Jiang J, Gao J, Zhao C, Tai Y, et al. Celecoxib ameliorates liver cirrhosis via reducing inflammation and oxidative stress along spleen-liver axis in rats. Life Sci. (2021) 272:119203. doi: 10.1016/j.lfs.2021.119203
30. Li CJ, Yang ZH, Shi XL, Liu DL. Effects of aspirin and enoxaparin in a rat model of liver fibrosis. World J Gastroenterol. (2017) 23:6412–9. doi: 10.3748/wjg.v23.i35.6412
31. Assy N, Hussein O, Khalil A, Luder A, Szvalb S, Paizi M, et al. The beneficial effect of aspirin and enoxaparin on fibrosis progression and regenerative activity in a rat model of cirrhosis. Dig Dis Sci. (2007) 52:1187–93. doi: 10.1007/s10620-006-9595-1
32. Abdul-Hamid M, Ahmed RR, Moustafa N, Nady R. The antifibrogenic effect of etanercept on development of liver cirrhosis induced by thioacetamide in rats. Ultrastruct Pathol. (2017) 41:23–35. doi: 10.1080/01913123.2016.1256361
33. Abo-Zaid MA, Shaheen ES, Ismail AH. Immunomodulatory effect of curcumin on hepatic cirrhosis in experimental rats. J Food Biochem. (2020) 44:e13219. doi: 10.1111/jfbc.13219
34. Macías-Pérez JR, Vázquez-López BJ, Muñoz-Ortega MH, Aldaba-Muruato LR, Martínez-Hernández SL, Sánchez-Alemán E, et al. Curcumin and α/β-Adrenergic antagonists cotreatment reverse liver cirrhosis in hamsters: Participation of Nrf-2 and NF-κB. J Immunol Res. (2019) 2019:3019794. doi: 10.1155/2019/3019794
35. Kyung EJ, Kim HB, Hwang ES, Lee S, Choi BK, Kim JW, et al. Evaluation of hepatoprotective effect of curcumin on liver cirrhosis using a combination of biochemical analysis and magnetic resonance-based electrical conductivity imaging. Mediators Inflamm. (2018) 2018:5491797. doi: 10.1155/2018/5491797
36. Chenari S, Safari F, Moradi A. Curcumin enhances liver SIRT3 expression in the rat model of cirrhosis. Iran J Basic Med Sci. (2017) 20:1306–11. doi: 10.22038/IJBMS.2017.9609
37. Hsu SJ, Lee JY, Lin TY, Hsieh YH, Huang HC, Lee FY, et al. The beneficial effects of curcumin in cirrhotic rats with portal hypertension. Biosci Rep. (2017) 37:BSR20171015. doi: 10.1042/BSR20171015
38. Cai Y, Lu D, Zou Y, Zhou C, Liu H, Tu C, et al. Curcumin protects against intestinal origin endotoxemia in rat liver cirrhosis by targeting PCSK9. J Food Sci. (2017) 82:772–80. doi: 10.1111/1750-3841.13647
39. Hernández-Aquino E, Quezada-Ramírez MA, Silva-Olivares A, Ramos-Tovar E, Flores-Beltrán RE, Segovia J, et al. Curcumin downregulates Smad pathways and reduces hepatic stellate cells activation in experimental fibrosis. Ann Hepatol. (2020) 19:497–506. doi: 10.1016/j.aohep.2020.05.006
40. Seo HY, Kim MK, Lee SH, Hwang JS, Park KG, Jang BK. Kahweol ameliorates the liver inflammation through the inhibition of NF-κB and STAT3 activation in primary kupffer cells and primary hepatocytes. Nutrients. (2018) 10:863. doi: 10.3390/nu10070863
41. Arauz J, Moreno MG, Cortés-Reynosa P, Salazar EP, Muriel P. Coffee attenuates fibrosis by decreasing the expression of TGF-β and CTGF in a murine model of liver damage. J Appl Toxicol. (2013) 33:970–9. doi: 10.1002/jat.2788
42. Ali FEM, Bakr AG, Abo-youssef AM, Azouz AA, Hemeida RAM. Targeting Keap-1/Nrf-2 pathway and cytoglobin as a potential protective mechanism of diosmin and pentoxifylline against cholestatic liver cirrhosis. Life Sci. (2018) 207:50–60. doi: 10.1016/j.lfs.2018.05.048
43. Ali FEM, Azouz AA, Bakr AG, Abo-youssef AM, Hemeida RAM. Hepatoprotective effects of diosmin and/or sildenafil against cholestatic liver cirrhosis: the role of Keap-1/Nrf-2 and P38-MAPK/NF-κB/iNOS signaling pathway. Food Chem Toxicol. (2018) 120:294–304. doi: 10.1016/j.fct.2018.07.027
44. Tahir M, Rehman MU, Lateef A, Khan R, Khan AQ, Qamar W, et al. Diosmin protects against ethanol-induced hepatic injury via alleviation of inflammation and regulation of TNF-α and NF-κB activation. Alcohol. (2013) 47:131–9. doi: 10.1016/j.alcohol.2012.12.010
45. Zhang H, Lin Y, Zhen Y, Hu G, Meng X, Li X, et al. Therapeutic effect of glycyrrhizin arginine salt on rat cholestatic cirrhosis and its mechanism. Am J Chin Med. (2018) 46:1111–27. doi: 10.1142/S0192415X18500581
46. Tripathi DM, Vilaseca M, Lafoz E, Garcia-Calderó H, Viegas Haute G, Fernández-Iglesias A, et al. Simvastatin prevents progression of acute on chronic liver failure in rats with cirrhosis and portal hypertension. Gastroenterology. (2018) 155:1564–77. doi: 10.1053/j.gastro.2018.07.022
47. Meireles CZ, Pasarin M, Lozano JJ, García-Calderó H, Gracia-Sancho J, García-Pagán JC, et al. Simvastatin attenuates liver injury in rodents with biliary cirrhosis submitted to hemorrhage/resuscitation. Shock. (2017) 47:370–7. doi: 10.1097/SHK.0000000000000734
48. Uschner FE, Ranabhat G, Choi SS, Granzow M, Klein S, Schierwagen R, et al. Statins activate the canonical hedgehog-signaling and aggravate non-cirrhotic portal hypertension, but inhibit the non-canonical hedgehog signaling and cirrhotic portal hypertension. Sci Rep. (2015) 5:14573. doi: 10.1038/srep14573
49. Shirin H, Sharvit E, Aeed H, Gavish D, Bruck R. Atorvastatin and rosuvastatin do not prevent thioacetamide induced liver cirrhosis in rats. World J Gastroenterol. (2013) 19:241–8. doi: 10.3748/wjg.v19.i2.241
50. Jang YO, Kim SH, Cho MY, Kim KS, Park KS, Cha SK, et al. Synergistic effects of simvastatin and bone marrow-derived mesenchymal stem cells on hepatic fibrosis. Biochem Biophys Res Commun. (2018) 497:264–71. doi: 10.1016/j.bbrc.2018.02.067
51. Gracia-Sancho J, Manicardi N, Ortega-Ribera M, Maeso-Díaz R, Guixé-Muntet S, Fernández-Iglesias A, et al. Emricasan ameliorates portal hypertension and liver fibrosis in cirrhotic rats through a hepatocyte-mediated paracrine mechanism. Hepatol Commun. (2019) 3:987–1000. doi: 10.1002/hep4.1360
52. Boyer-Diaz Z, Aristu-Zabalza P, Andrés-Rozas M, Robert C, Ortega-Ribera M, Fernández-Iglesias A, et al. Pan-PPAR agonist lanifibranor improves portal hypertension and hepatic fibrosis in experimental advanced chronic liver disease. J Hepatol. (2021) 74:1188–99. doi: 10.1016/j.jhep.2020.11.045
53. Jun JH, Park SY, Park S, Park HJ, Kim JY, Park GT, et al. Formyl peptide receptor 2 alleviates hepatic fibrosis in liver cirrhosis by vascular remodeling. Int J Mol Sci. (2021) 22:1–4. doi: 10.3390/ijms22042107
54. Vilaseca M, García-Calderó H, Lafoz E, Ruart M, López-Sanjurjo CI, Murphy MP, et al. Mitochondria-targeted antioxidant mitoquinone deactivates human and rat hepatic stellate cells and reduces portal hypertension in cirrhotic rats. Liver Int. (2017) 37:1002–12. doi: 10.1111/liv.13436
55. Turkseven S, Bolognesi M, Brocca A, Pesce P, Angeli P, Di Pascoli M. Mitochondria-targeted antioxidant mitoquinone attenuates liver inflammation and fibrosis in cirrhotic rats. Am J Physiol - Gastrointest Liver Physiol. (2020) 318:G298–304. doi: 10.1152/ajpgi.00135.2019
56. Zaidi SNF, Mahboob T. Prevention of liver cirrhosis by Silymarin. Pak J Pharm Sci. (2017) 30:1203–11.
57. Ghobadi Pour M, Mirazi N, Alaei H, Moradkhani S, Rajaei Z, Monsef Esfahani A. Effects of lactulose and silymarin on liver enzymes in cirrhotic rats. Can J Physiol Pharmacol. (2017) 95:522–9. doi: 10.1139/cjpp-2016-0454
58. Ali SO, Darwish HAEM, Ismail NAEF. Modulatory effects of curcumin, silybin-phytosome and alpha-R-lipoic acid against thioacetamide-induced liver cirrhosis in rats. Chem Biol Interact. (2014) 216:26–33. doi: 10.1016/j.cbi.2014.03.009
59. Abdel-Moneim AM, Al-Kahtani MA, El-Kersh MA, Al-Omair MA. Free radical-scavenging, anti-inflammatory/anti-fibrotic and hepatoprotective actions of taurine and silymarin against CCl4 induced rat liver damage. PLoS ONE. (2015) 10:e0144509. doi: 10.1371/journal.pone.0144509
60. Aithal AP, Bairy LK, Seetharam RN, Kumar N. Hepatoprotective effect of bone marrow-derived mesenchymal stromal cells in CCl4-induced liver cirrhosis. 3 Biotech. (2021) 11:107. doi: 10.1007/s13205-021-02640-y
61. Yang N, Chen H, Gao Y, Zhang S, Lin Q, Ji X, et al. Tanshinone IIA exerts therapeutic effects by acting on endogenous stem cells in rats with liver cirrhosis. Biomed Pharmacother. (2020) 132:110815. doi: 10.1016/j.biopha.2020.110815
62. Liu R, Kang JD, Sartor RB, Sikaroodi M, Fagan A, Gavis EA, et al. Neuroinflammation in murine cirrhosis is dependent on the gut microbiome and is attenuated by fecal transplant. Hepatology. (2020) 71:611–26. doi: 10.1002/hep.30827
63. Chen YX, Lai LN, Zhang HY, Bi YH, Meng L, Li XJ, et al. Effect of artesunate supplementation on bacterial translocation and dysbiosis of gut microbiota in rats with liver cirrhosis. World J Gastroenterol. (2016) 22:2949–59. doi: 10.3748/wjg.v22.i10.2949
64. Fortea JI, Zipprich A, Fernandez-Mena C, Puerto M, Bosoi CR, Almagro J, et al. Enoxaparin does not ameliorate liver fibrosis or portal hypertension in rats with advanced cirrhosis. Liver Int. (2018) 38:102–12. doi: 10.1111/liv.13510
65. Cerini F, Vilaseca M, Lafoz E, García-Irigoyen O, García-Calderó H, Tripathi DM, et al. Enoxaparin reduces hepatic vascular resistance and portal pressure in cirrhotic rats. J Hepatol. (2016) 64:834–42. doi: 10.1016/j.jhep.2015.12.003
66. Tilg H. Cytokines and liver diseases. Can J Gastroenterol. (2001) 15:661–8. doi: 10.1155/2001/746736
67. Gao JH, Wen SL, Feng S, Yang WJ, Lu YY, Tong H, et al. Celecoxib and octreotide synergistically ameliorate portal hypertension via inhibition of angiogenesis in cirrhotic rats. Angiogenesis. (2016) 19:501–11. doi: 10.1007/s10456-016-9522-9
68. Lebrec D, Thabut D, Oberti F, Perarnau JM, Condat B, Barraud H, et al. Pentoxifylline does not decrease short-term mortality but does reduce complications in patients with advanced cirrhosis. Gastroenterology. (2010) 138:1755–62. doi: 10.1053/j.gastro.2010.01.040
69. Frenette C, Kayali Z, Mena E, Mantry PS, Lucas KJ, Neff G, et al. Emricasan to prevent new decompensation in patients with NASH-related decompensated cirrhosis. J Hepatol. (2021) 74:274–82. doi: 10.1016/j.jhep.2020.09.029
70. Krasselt M, Baerwald C. Celecoxib for the treatment of musculoskeletal arthritis. Expert Opin Pharmacother. (2019) 20:1689–702. doi: 10.1080/14656566.2019.1645123
71. Puljak L, Marin A, Vrdoljak D, Markotic F, Utrobicic A, Tugwell P. Celecoxib for osteoarthritis. Cochrane Database Syst Rev. (2017) 2017:CD009865. doi: 10.1002/14651858.CD009865.pub2
72. Mohammed NA, El-Aleem SA, El-Hafiz HA, McMahon RFT. Distribution of constitutive (COX-1) and inducible (COX-2) cyclooxygenase in postviral human liver cirrhosis: a possible role for COX-2 in the pathogenesis of liver cirrhosis. J Clin Pathol. (2004) 57:350–4. doi: 10.1136/jcp.2003.012120
73. El-Bassiouny A, Zoheiry MMK, Nosseir MMF, El-Ahwany EG, Ibrahim RA, El-Bassiouni NEI. Expression of cyclooxygenase-2 and transforming growth factor-beta 1 in HCV-induced chronic liver disease and hepatocellular carcinoma. MedGenMed. (2007) 9:45.
74. Li T, Dong ZR, Guo ZY, Wang CH, Tang ZY, Qu SF, et al. Aspirin enhances IFN-α-induced growth inhibition and apoptosis of hepatocellular carcinoma via JAK1/STAT1 pathway. Cancer Gene Ther. (2013) 20:366–74. doi: 10.1038/cgt.2013.29
75. Jiang ZG, Feldbrügge L, Tapper EB, Popov Y, Ghaziani T, Afdhal N, et al. Aspirin use is associated with lower indices of liver fibrosis among adults in the United States. Aliment Pharmacol Ther. (2016) 43:734–43. doi: 10.1111/apt.13515
76. Shin S, Lee SH, Lee M, Kim JH, Lee W, Lee HW, et al. Aspirin and the risk of hepatocellular carcinoma development in patients with alcoholic cirrhosis. Medicine. (2020) 99:e19008. doi: 10.1097/MD.0000000000019008
77. Lee TY, Hsu YC, Tseng HC, Yu SH, Lin JT, Wu MS, et al. Association of daily aspirin therapy with risk of hepatocellular carcinoma in patients with chronic hepatitis B. JAMA Intern Med. (2019) 179:633–40. doi: 10.1001/jamainternmed.2018.8342
78. Böhm F, Köhler UA, Speicher T, Werner S. Regulation of liver regeneration by growth factors and cytokines. EMBO Mol Med. (2010) 2:294–305. doi: 10.1002/emmm.201000085
79. Baeck C, Tacke F. Balance of inflammatory pathways and interplay of immune cells in the liver during homeostasis and injury. EXCLI J. (2014) 13:67–81.
80. Tang KT, Dufour JF, Chen PH, Hernaez R, Hutfless S. Antitumour necrosis factor-α agents and development of new-onset cirrhosis or non-alcoholic fatty liver disease: a retrospective cohort. BMJ Open Gastroenterol. (2020) 7:e000349. doi: 10.1136/bmjgast-2019-000349
81. Qiu P, Sun J, Man S, Yang H, Ma L, Yu P, et al. Curcumin attenuates N-Nitrosodiethylamine-induced liver injury in mice by utilizing the method of metabonomics. J Agric Food Chem. (2017) 65:2000–7. doi: 10.1021/acs.jafc.6b04797
82. Nouri-Vaskeh M, Malek Mahdavi A, Afshan H, Alizadeh L, Zarei M. Effect of curcumin supplementation on disease severity in patients with liver cirrhosis: a randomized controlled trial. Phyther Res. (2020) 34:1446–54. doi: 10.1002/ptr.6620
83. He G, Karin M. NF-κB and STAT3- key players in liver inflammation and cancer. Cell Res. (2011) 21:159–68. doi: 10.1038/cr.2010.183
84. Cárdenas C, Quesada AR, Medina MA. Anti-angiogenic and anti-inflammatory properties of kahweol, a coffee diterpene. PLoS ONE. (2011) 6:e23407. doi: 10.1371/annotation/38262cc6-07cc-4074-8ce7-2181d4d0fbdc
85. Bektas S, Karakaya K, Can M, Bahadir B, Guven B, Erdogan N, et al. The effects of tadalafil and pentoxifylline on apoptosis and nitric oxide synthase in liver ischemia/reperfusion injury. Kaohsiung J Med Sci. (2016) 32:339–47. doi: 10.1016/j.kjms.2016.05.005
86. Li H, Hua J, Guo CX, Wang WX, Wang BJ, Yang DL, et al. Pentoxifylline inhibits liver fibrosis via hedgehog signaling pathway. J Huazhong Univ Sci Technol - Med Sci. (2016) 36:372–6. doi: 10.1007/s11596-016-1594-7
87. Zheng Y, Zhang R, Shi W, Li L, Liu H, Chen Z, et al. Metabolism and pharmacological activities of the natural health-benefiting compound diosmin. Food Funct. (2020) 11:8472–92. doi: 10.1039/D0FO01598A
88. Dastagir G, Rizvi MA. Review Glycyrrhiza glabra L. (Liquorice). Pak J Pharm Sci. (2016) 29:1727–33.
89. Tsochatzis EA, Bosch J. Statins in cirrhosis—ready for prime time. Hepatology. (2017) 66:697–9. doi: 10.1002/hep.29277
90. Bang UC, Benfield T, Bendtsen F. Reduced risk of decompensation and death associated with use of statins in patients with alcoholic cirrhosis. a nationwide case-cohort study. Aliment Pharmacol Ther. (2017) 46:673–80. doi: 10.1111/apt.14243
91. Pose E, Trebicka J, Mookerjee RP, Angeli P, Ginès P. Statins: old drugs as new therapy for liver diseases? J Hepatol. (2019) 70:194–202. doi: 10.1016/j.jhep.2018.07.019
92. Pollo-Flores P, Soldan M, Santos UC, Kunz DG, Mattos DE, da Silva AC, et al. Three months of simvastatin therapy vs. placebo for severe portal hypertension in cirrhosis: a randomized controlled trial. Dig Liver Dis. (2015) 47:957–63. doi: 10.1016/j.dld.2015.07.156
93. Abraldes JG, Villanueva C, Aracil C, Turnes J, Hernandez-Guerra M, Genesca J, et al. Addition of simvastatin to standard therapy for the prevention of variceal rebleeding does not reduce rebleeding but increases survival in patients with cirrhosis. Gastroenterology. (2016) 150:1160–70.e3. doi: 10.1053/j.gastro.2016.01.004
94. Abraldes JG, Albillos A, Bañares R, Turnes J, González R, García-Pagán JC, et al. Simvastatin lowers portal pressure in patients with cirrhosis and portal hypertension: a randomized controlled trial. Gastroenterology. (2009) 136:1651–8. doi: 10.1053/j.gastro.2009.01.043
95. Huang YW, Lee CL, Yang SS, Fu SC, Chen YY, Wang TC, et al. Statins reduce the risk of cirrhosis and its decompensation in chronic hepatitis b patients: a nationwide cohort study. Am J Gastroenterol. (2016) 111:976–85. doi: 10.1038/ajg.2016.179
96. Motzkus-Feagans C, Pakyz AL, Ratliff SM, Bajaj JS, Lapane KL. Statin use and infections in veterans with cirrhosis. Aliment Pharmacol Ther. (2013) 38:611–8. doi: 10.1111/apt.12430
97. Bishnu S, Ahammed SKM, Sarkar A, Hembram J, Chatterjee S, Das K, et al. Effects of atorvastatin on portal hemodynamics and clinical outcomes in patients with cirrhosis with portal hypertension: a proof-of-concept study. Eur J Gastroenterol Hepatol. (2018) 30:54–9. doi: 10.1097/MEG.0000000000001006
98. Kumar S, Grace ND, Qamar AA. Statin use in patients with cirrhosis: a retrospective cohort study. Dig Dis Sci. (2014) 59:1958–65. doi: 10.1007/s10620-014-3179-2
99. Simon TG, Bonilla H, Yan P, Chung RT, Butt AA. Atorvastatin and fluvastatin are associated with dose-dependent reductions in cirrhosis and hepatocellular carcinoma, among patients with hepatitis C virus: results from ERCHIVES. Hepatology. (2016) 64:47–57. doi: 10.1002/hep.28506
100. Mohanty A, Tate JP, Garcia-Tsao G. Statins are associated with a decreased risk of decompensation and death in veterans with hepatitis c-related compensated cirrhosis. Gastroenterology. (2016) 150:430–40.e1. doi: 10.1053/j.gastro.2015.10.007
101. Chang FM, Wang YP, Lang HC, Tsai CF, Hou MC, Lee FY, et al. Statins decrease the risk of decompensation in hepatitis B virus– and hepatitis C virus–related cirrhosis: a population-based study. Hepatology. (2017) 66:896–907. doi: 10.1002/hep.29172
102. Abd-Elsalam S, Elwan N, Salah R, Hamisa M, Shady E, Hawash N. Evaluation of portal pressure by doppler ultrasound in patients with cirrhosis before and after simvastatin administration - a randomized controlled trial. F1000Research. (2018) 7:256. doi: 10.12688/f1000research.13915.1
103. Kaplan DE, Serper MA, Mehta R, Fox R, John B, Aytaman A, et al. Effects of hypercholesterolemia and statin exposure on survival in a large national cohort of patients with cirrhosis. Gastroenterology. (2019) 156:1693–706.e12. doi: 10.1053/j.gastro.2019.01.026
104. Hung TH, Tsai CC, Lee HF. Statin use in cirrhotic patients with infectious diseases: a population-based study. PLoS ONE. (2019) 14:e0215839. doi: 10.1371/journal.pone.0215839
105. Liss KHH, Finck BN. PPARs and nonalcoholic fatty liver disease. Biochimie. (2017) 136:65–74. doi: 10.1016/j.biochi.2016.11.009
106. Fathalah WF, Aziz MAA, Soud NHA El, Raziky MES El. High dose of silymarin in patients with decompensated liver disease: a randomized controlled trial. J Interf Cytokine Res. (2017) 37:480–7. doi: 10.1089/jir.2017.0051
107. Bataller R, Schwabe RF, Choi YH, Yang L, Paik YH, Lindquist J, et al. NADPH oxidase signal transduces angiotensin II in hepatic stellate cells and is critical in hepatic fibrosis. J Clin Invest. (2003) 112:1383–94. doi: 10.1172/JCI18212
108. Federico A, Dallio M, Loguercio C. Silymarin/Silybin and chronic liver disease: a marriage of many years. Molecules. (2017) 22:191. doi: 10.3390/molecules22020191
109. Woodhouse CA, Patel VC, Goldenberg S, Sanchez-Fueyo A, China L, O'Brien A, et al. PROFIT, a PROspective, randomised placebo controlled feasibility trial of faecal microbiota transplantation in cirrhosis: study protocol for a single-blinded trial. BMJ Open. (2019) 9:e023518. doi: 10.1136/bmjopen-2018-023518
110. Cirera I, Martin Bauer T, Miguel N, Vila J, Grande L, Taurá P, et al. Bacterial translocation of enteric organisms in patients with cirrhosis. J Hepatol. (2001) 34:32–7. doi: 10.1016/S0168-8278(00)00013-1
111. Villa E, Cammà C, Marietta M, Luongo M, Critelli R, Colopi S, et al. Enoxaparin prevents portal vein thrombosis and liver decompensation in patients with advanced cirrhosis. Gastroenterology. (2012) 143:1253–60.e4. doi: 10.1053/j.gastro.2012.07.018
112. Frizelle S, Schwarz J, Huber SA, Leslie K. Evaluation of the effects of low molecular weight heparin on inflammation and collagen deposition in chronic coxsackievirus B3-induced myocarditis in A/J mice. Am J Pathol. (1992) 141:203–9
113. Tripodi A, Primignani M, Chantarangkul V, Dell'Era A, Clerici M, de Franchis R, et al. An imbalance of pro- vs anti-coagulation factors in plasma from patients with cirrhosis. Gastroenterology. (2009) 137:2105–11. doi: 10.1053/j.gastro.2009.08.045
114. Lisman T, Leebeek FWG. Hemostatic alterations in liver disease: a review on pathophysiology, clinical consequences, and treatment. Dig Surg. (2007) 24:250–8. doi: 10.1159/000103655
115. Shi MJ, Dong BS, Yang WN, Su SB, Zhang H. Preventive and therapeutic role of Tanshinone IIA in hepatology. Biomed Pharmacother. (2019) 112:108676. doi: 10.1016/j.biopha.2019.108676
116. Nouri-Vaskeh M, Afshan H, Malek Mahdavi A, Alizadeh L, Fan X, Zarei M. Curcumin ameliorates health-related quality of life in patients with liver cirrhosis: a randomized, double-blind placebo-controlled trial. Complement Ther Med. (2020) 49:102351. doi: 10.1016/j.ctim.2020.102351
117. Pose E, Napoleone L, Amin A, Campion D, Jimenez C, Piano S, et al. Safety of two different doses of simvastatin plus rifaximin in decompensated cirrhosis (LIVERHOPE-SAFETY): a randomised, double-blind, placebo-controlled, phase 2 trial. Lancet Gastroenterol Hepatol. (2020) 5:31–41. doi: 10.1016/S2468-1253(19)30320-6
118. Bajaj JS, Kakiyama G, Savidge T, Takei H, Kassam ZA, Fagan A, et al. Antibiotic-associated disruption of microbiota composition and function in cirrhosis is restored by fecal transplant. Hepatology. (2018) 68:1549–58. doi: 10.1002/hep.30037
119. Clària J, Kent JD, López-Parra M, Escolar G, Ruiz-del-Arbol L, Ginès P, et al. Effects of celecoxib and naproxen on renal function in nonazotemic patients with cirrhosis and ascites. Hepatology. (2005) 41:579–87. doi: 10.1002/hep.20595
120. Guevara M, Abecasis R, Terg R. Effect of celecoxib on renal function in cirrhotic patients with ascites. a pilot study. Scand J Gastroenterol. (2004) 39:385–6. doi: 10.1080/00365520310008205
121. Moroni F, Dwyer BJ, Graham C, Pass C, Bailey L, Ritchie L, et al. Safety profile of autologous macrophage therapy for liver cirrhosis. Nat Med. (2019) 25:1560–5. doi: 10.1038/s41591-019-0599-8
Keywords: liver cirrhosis, inflammation, treatment, cytokines, anti-oxidation, cirrhosis models
Citation: Kronborg TM, Ytting H, Hobolth L, Møller S and Kimer N (2021) Novel Anti-inflammatory Treatments in Cirrhosis. A Literature-Based Study. Front. Med. 8:718896. doi: 10.3389/fmed.2021.718896
Received: 01 June 2021; Accepted: 26 August 2021;
Published: 23 September 2021.
Edited by:
Gianfranco Danilo Alpini, Indiana University, United StatesReviewed by:
Nazri Mustaffa, Universiti Sains Malaysia (USM), MalaysiaNan Wu, Indiana University, United States
Lixian Chen, Indiana University, United States
Copyright © 2021 Kronborg, Ytting, Hobolth, Møller and Kimer. This is an open-access article distributed under the terms of the Creative Commons Attribution License (CC BY). The use, distribution or reproduction in other forums is permitted, provided the original author(s) and the copyright owner(s) are credited and that the original publication in this journal is cited, in accordance with accepted academic practice. No use, distribution or reproduction is permitted which does not comply with these terms.
*Correspondence: Thit Mynster Kronborg, thit.mynster.kronborg@regionh.dk