- 1Translational Research Center for Gastrointestinal Disorders (TARGID), Lab for Intestinal Neuro-Immune Interaction, Department of Chronic Diseases, Metabolism and Ageing, Katholieke Universiteit Leuven - University of Leuven, Leuven, Belgium
- 2Translational Research Center for Gastrointestinal Disorders (TARGID), Lab for Mucosal Immunology, Department of Chronic Diseases, Metabolism and Ageing, Katholieke Universiteit Leuven - University of Leuven, Leuven, Belgium
- 3Department of Pathology, Universitair Ziekenhuis Leuven, Leuven, Belgium
Background: We previously showed increased susceptibility to dextran sulfate sodium (DSS)-induced colitis in vagotomized mice. Here, we evaluated whether vagus nerve stimulation (VNS) is able to reduce the severity of DSS colitis and aimed to unravel the mechanism involved.
Methods: Colitis was induced in wild type mice by 2.5% DSS administration in drinking water for 5 days. VNS (5 Hz, 1 ms, 1 mA) was applied 1 day prior to and after 4 days of DSS administration to evaluate changes in epithelial integrity and inflammatory response, respectively. Epithelial integrity was assessed using TUNEL and Ki67 staining. Monocytes, immature and mature macrophages were sorted from colonic samples and gene expression levels of pro-inflammatory cytokines were studied.
Results: VNS applied prior to DSS administration (i.e., prophylactic VNS) reduced disease activity index (VNS 0.8 ± 0.6 vs. sham 2.8 ± 0.7, p < 0.001, n = 5) and tended to improve histology score. Prophylactic VNS significantly increased epithelial cell proliferation and diminished apoptosis compared to sham stimulation. VNS applied at day 4 during DSS administration (i.e., therapeutic VNS) decreased the influx of monocytes, monocyte-derived macrophages and neutrophils, and significantly reduced pro-inflammatory cytokine expression (i.e., Tnfα and Cxcl1) in immature macrophages compared to sham stimulation.
Conclusions: A single period of VNS applied prior to DSS exposure reduced DSS-induced colitis by an improvement in epithelial integrity. On the other hand, VNS applied during the inflammatory phase of DSS colitis reduced cytokine expression in immature macrophages. Our data further underscores the potential of VNS as novel therapeutic approach for inflammatory bowel diseases.
Introduction
More than 2 decades ago, the vagus nerve was proposed as an important regulator of the immune system. Electrical and pharmacological activation of the vagus nerve indeed improved survival in a model of sepsis by reducing TNFα production of splenic macrophages (Mφ) (1). We further extended the therapeutic potential of the so-called cholinergic anti-inflammatory pathway (CAIP) to the gut by showing that cervical and abdominal VNS dampens muscularis macrophage (MMφ) activation and attenuates surgery-induced intestinal inflammation (2–4). Similar to the spleen, the alpha 7 nicotinic acetylcholine receptor (α7nAChR) was identified as the target receptor of the “gastrointestinal CAIP”, suggesting that acetylcholine (ACh) released from the vagus nerve inhibits MMφ (2, 3, 5, 6). To date, electrical stimulation of the cervical vagus nerve is already used as therapeutic tool for refractory epilepsy and treatment-resistant depression (7), while its applicability in inflammatory disorders including rheumatoid arthritis (8) and inflammatory bowel diseases (IBD) (9) is currently under investigation.
IBD, comprising Crohn's disease and ulcerative colitis, consists of chronic inflammatory disorders of the gastrointestinal tract whose prevalence has dramatically increased over the past decade. IBD is the second most common chronic inflammatory disease worldwide after rheumatoid arthritis, affecting millions of people mainly in industrialized countries. Although the exact etiology is unknown, immunological, microbial, environmental, nutritional, and genetic factors are proposed to contribute to the pathogenesis and severity of IBD (10–13). To date, the clinical management has significantly improved with the introduction of biologicals, yet a significant proportion of patients remains refractory to these compounds. Based on its anti-inflammatory properties, electrical and pharmacological activation of the vagal anti-inflammatory pathway has been studied in preclinical models of IBD. Bonaz and colleagues demonstrated that 5 days of VNS successfully prevented body weight loss and improved colonic mucosal damage in a rat model of 2,4,6-trinitrobenzenesulfonic acid (TNBS) colitis (14). This effect was associated with a decrease in TNFα, IL-1β, and IL-6 levels through inhibition of the JAK2/STAT3 signaling pathway (2). In line, pharmacological activation of the CAIP was also obtained by blockade of acetylcholine esterase (AChE). In dextran sulfate sodium (DSS)-induced colitis, central activation of the vagus nerve by galantamine, an AChE inhibitor, reduced the severity of DSS-induced colitis in mice (15). Conversely, vagotomy increased the susceptibility to develop DSS colitis, impairing the ability to develop oral tolerance (16–18). However, the target population of VNS in colitis remains largely understudied.
During DSS-induced colitis, upon 3 days of DSS administration, changes in the epithelial barrier occur: increased levels of pro-inflammatory cytokines, such as TNFα, IL-1β, and IL-8 lead to a decreased barrier function inducing reorganization of tight junction proteins. These changes will ultimately trigger the immune response contributing the propagation of intestinal inflammation. Recently, Asano et al. (19) elegantly identified the kinetics of the inflammatory response triggered by DSS. In more detail, the toxic effect of DSS to the colonic epithelium induced erosions and apoptosis that eventually increased colonic epithelial permeability. In this study, it was demonstrated that a specific subset of Mφ, carrying CD169 on their surface, is strategically localized closely to the blood vessels and distant from the epithelial barrier. This unusual localization confers to the role of CD169+ Mφ as sentinels of the immune system in the gut, due to their ability to produce and directly release high levels of the chemoattractant CCL8, a key regulator of mucosal inflammation, into the blood stream.
Based on this study, we hypothesized that VNS reduces the severity of DSS-induced colitis not only by its anti-inflammatory properties, but also by reducing epithelial damage and/or increasing barrier resistance against DSS. Indeed, increased intestinal permeability caused by DSS allows increased entrance of antigens, resulting in an amplification of the inflammatory response in IBD (20, 21). Of interest, in a thermal injury model, post injury VNS preserves intestinal architecture by preventing epithelial barrier breakdown and reducing inflammation (22). Moreover, nicotine administration increased enteric glial cell activation, which improved intestinal epithelial barrier function in an in vitro model of intestinal injury (23).
Based on the above, this study was designed to evaluate the effect of VNS in DSS-induced colitis focusing on its effect on epithelial integrity in the early phase of the disease (d2), and on intestinal inflammation in a later phase of colitis (d5).
Materials and Methods
Experimental Animals
Ten to 14 weeks old wild-type female C57BL/6J mice were bred and housed at the KU Leuven specific pathogen-free animal facility under environmentally controlled conditions (12 h-12 h dark-light cycle; 20–22°C, 55% humidity). Mice were provided with commercially available chow (Ssniff R/M-H; ssniff Spezialdiäten GmbH, Soest, Germany) and water ad libitum. All animals were treated carefully in strict accordance with the ethical guidelines and all experimental procedures were approved by the Animal Care and Animal Experiments Committee of KU Leuven (P002/2014; P003/2014; Leuven, Belgium). All surgeries were performed under anesthesia and all efforts were made to minimize suffering.
Electrical Stimulation of the Vagus Nerve
Anesthetized (2.5% isoflurane) (ISO-VET, Eurovet NV/SA, Heusden-Zolder, Belgium) mice underwent VNS as previously described (24). In more detail, a midline cervical incision was made, and the subcutaneous tissue was dissected and retracted laterally. The mandibular salivary glands were bluntly separated and retracted laterally. The right cervical vagus nerve was isolated from the carotid artery and stimulated electrically using a bipolar platinum electrode (Bilaney, Dusseldorf, Germany). Electrical stimuli consisted of square pulses (5 Hz, 1 mA, 1 ms for 5 min) (Keithley Instruments, Cleveland, Ohio) (25). Sham-operated mice were handled similarly, but the vagus nerve was not dissected from the carotid artery to avoid mechanical stimulation. Mice were kept on a heating pad to recover from surgery with ad libitum access to food and water. Depending on the timing of VNS application, the mice were divided into two groups: the first group of mice underwent VNS or sham-stimulation 1 day prior to DSS administration (prophylactic effect), while the second group underwent VNS or sham-stimulation after 4 days of DSS administration (therapeutic effect).
DSS-Induced Colitis
Colitis was induced in wild-type C57BL/6J mice by administration of 2.5% DSS (TbB Consultancy AB, Uppsala, Sweden) in drinking water for 5 days followed by normal drinking water until day 14. When VNS was studied as a prophylactic treatment, VNS and sham-stimulated mice were exposed to 2.5% DSS for 2 days and sacrificed at day 2. To study the therapeutic effect of VNS during ongoing inflammation, VNS and sham-stimulated mice were exposed to 2.5% DSS for 5 days and sacrificed at day 5 by CO2 overdose. The colon and spleen were dissected and blood was taken via cardiac puncture for further investigation.
Quantification of Disease Activity
Daily clinical assessment of DSS-treated animals included measurement of a validated clinical disease activity index (DAI), calculated using the following parameters: stool consistency (0–4), presence or absence of fecal blood (0–4) (Hemoccult, Beckman Coulter, Pasadena, USA), and weight loss (0–4) (26). After assessment of DAI at day 14, mice were sacrificed by CO2 overdose, and the colon was dissected for further investigation.
Immunohistochemistry
One part of colon was immediately fixed in 4% buffered paraformaldehyde, dehydrated in 100% ethanol and embedded in paraffin. Five μm thin sections were cut. To determine the degree of colonic damage and inflammation, sections were stained with hematoxylin and eosin (H&E) and were histologically examined by a pathologist in a blinded fashion using a validated scoring system (Table 1) (27). The total colonic score was calculated as the sum of the two parameters per section (Table 1).
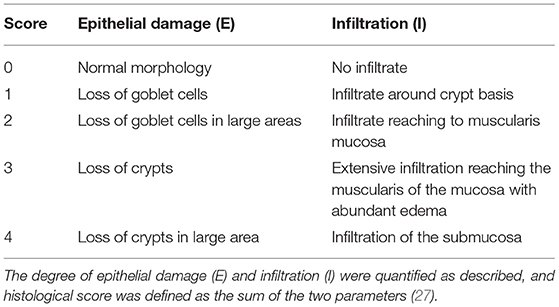
Table 1. Histological scoring using Ito et al. (27).
In addition, other colonic sections were stained with Ki67 to detect epithelial proliferation. To this end, sections were rehydrated and blocked with 5% H2O2 and ABC Kits (Vector Laboratories, California, USA) to reduce non-specific signal from endogenous peroxidase. The slides were then incubated overnight with a rabbit polyclonal anti-Ki67 antibody (1:500, Abcam, Cambridge, UK). Cells immunoreactive to Ki67 were detected using VECTASTAIN ABC streptavidin-HRP system (Vector Laboratories, California, USA). Next, these sections were counterstained with hematoxylin, dehydrated and mounted. The number of Ki-67-positive cells was counted in 10 randomly chosen representative high-power fields. All stained sections were imaged with a microscope (BX 41 Olympus, Aartselaar, Belgium) connected to a camera (SC30 Olympus, Tokyo, Japan) and Cell∧F software (Olympus, Tokyo, Japan).
Immunofluorescence
TUNEL staining was performed on colonic sections using the Click-iT TUNEL Alexa Fluor 488 Imaging Assay kit (ThermoFischer Scientific, Waltham, USA) according to the manufacturer's instructions. Samples were evaluated using confocal microscopy (Zeiss 880, ZEN software, Zeiss, Germany). The number of TUNEL-positive cells was counted in 10 randomly chosen representative high-power fields and images were acquired using a 20X objective and 40X objective.
Isolation of Leukocytes From Blood, Spleen and Colon, and Flow Cytometry
Blood was collected by cardiac puncture. Red blood cells were lysed with homemade lysis buffer (EDTA 68.5 μM, NaHCO3 5.95 mM, NH4Cl 77.57 mM dissolved in sterile water) after which the sample was centrifuged at 2,000 g for 10 min. Splenocytes were obtained from homogenized spleen in phosphate-buffered saline 1X (PBS) (Lonza, Verviers, Belgium) + 1% fetal bovine serum (FBS) (Nuaillé, France). Red blood cells were lysed with homemade lysis buffer. Cell suspensions were centrifuged at 4°C, filtered with a 70 μm cell strainer and counted. Lamina propria cell suspensions were prepared from the colon lamina propria as previously described (28).
All single cell suspensions were blocked with rat anti-mouse CD16/CD32 antibodies (BD Pharmigen, Franklin Lakes, USA) (1:200) for 12 min and then incubated with the following fluorophore-conjugated antibodies at recommended dilutions for 1 h at 4°C: CD11b (M1/70; BD Biosciences), SiglecF (1RNM44N, eBioscience), Ly6G (1A8, BD Biosciences), Ly6C (AL-21, BD Biosciences), MHCII (M5/114.15.2, eBioscience), CD169 (SER4, eBioscience) to sort lamina propria monocytes (CD11b+SiglecF−Ly6G−Ly6C+MHCII−), immature Mφ (CD11b+SiglecF−Ly6G−Ly6C+ MHCII+) and mature Mφ [CD11b+ SiglecF−Ly6G−Ly6C−MHCII+ (CD169− or CD169+)]. Samples were gated as indicated in Supplementary Figure 1. Dead cells were excluded using DAPI (ThermoFischer Scientific, Waltham, USA) and wash steps were performed in FACS buffer (PBS, 2% FBS and 0.78 mM EDTA). Cells were analyzed on a BD Canto HTS (BD Biosciences, Franklin Lakes, USA). Data was analyzed using FlowJo software (Tree Star Inc., Ashland, USA). FACS sorting experiments for gene expression analyses were performed on a BD Aria III (BD Biosciences, Franklin Lakes, USA) up to >95% purity. These cells were immediately sorted in RLT plus lysis buffer (QIAGEN, Hilden, Germany) containing β-mercaptoethanol and placed on dry ice.
RNA Extraction and Gene Expression
RNA extraction was performed using RNeasy Micro Kit (Qiagen, Hilden, Germany) following the manufacturer's instructions. Total RNA was retro-transcribed into complementary cDNA using qScript cDNA SuperMix (Quanta Biosciences, Gaithersburg, USA) according to the manufacturer's instructions and gene expression was assayed by quantitative reverse transcription PCR. Quantitative real-time transcription polymerase chain reactions were performed in duplicate with the FastStart SYBR Green Master mix (Roche, Basel, Switzerland) using the LightCycler® 96 (Roche, Basel, Switzerland). The expression levels of the genes of interest were normalized to the expression levels of the reference gene rpl32. The mean relative gene expression was calculated using the 2−ΔΔCT method. Primer sequences used are listed in Supplementary Table 1.
Statistical Analyses
The number of animals used per group was powered based on previous experimental results and observed variability. Normality and the presence of outliers were determined via the Kolmogorov–Smirnov test and Grubbs' testing, respectively, prior to further statistical analysis. Two-way ANOVA test with Bonferroni correction was performed to assess the effect of VNS on body weight loss and DAI. To compare differences in gene expression, histological score and flow cytometry data between sham- and VNS-treated animals, an unpaired t-test or Mann-Whitney test was done depending on normality. To compare differences between naïve, sham- and VNS-stimulated mice in flow cytometry data, we performed one-way ANOVA with Sidak correction. Data are expressed as mean ± standard error of mean (SEM) or median ± interquartile range depending on normality. Probability level of P < 0.05 was considered statistically significant. Statistical analysis was performed with GraphPad Prism Software (GraphPad Prism, La Jolla, USA).
Results
VNS Prior to DSS Exposure Improves Colitis by Reducing Apoptosis and Promoting Epithelial Cell Proliferation
First, we evaluated if prophylactic VNS reduced the severity of DSS-induced colitis (Figure 1A). We observed that a single application of VNS 1 day prior to the induction of DSS-induced colitis (day−1) caused a significant drop in the percentage of body weight in VNS-treated animals compared to the sham-operated animals, an effect most likely caused by a combination of surgical pain and a decreased appetite (29) following the VNS procedure (data not shown). This initial body weight drop in the VNS-treated mice prevented us to observe the true beneficial effect of VNS on body weight (Figure 1B). Nevertheless, we observed that prophylactic VNS significantly improved DAI compared to sham-operated mice (Figure 1C). Moreover, VNS-treated mice tended to improve histology score compared to the sham-operated mice (Figure 1D).
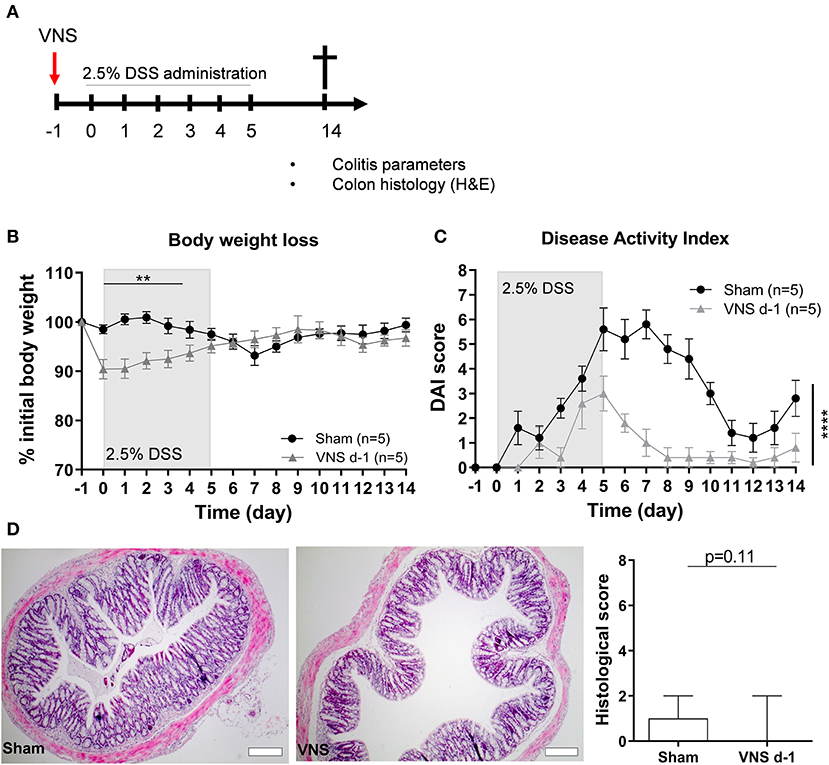
Figure 1. Vagus nerve stimulation prior to DSS exposure improves colitis. (A) Wild type mice were stimulated at day−1 and exposed to 2.5% DSS in the drinking water for 5 days, colitis severity was assessed daily. Mice were sacrificed at day 14 and tissue samples were collected for different analysis. Body weight (B) and disease activity index (C) were assessed daily. (B,C) Data are expressed as mean ± SEM as determined by the repeated-measures two-way ANOVA test. **p < 0.01; ****p < 0.0001; (n = 5 mice per group) (D) Representative H&E stainings of colonic sections from control sham- and VNS-stimulated mice. Scale bars are 200 μm. Bar graphs represent histological injury score of colonic samples of sham- and VNS-treated mice 14 days post colitis induction. Data are expressed as median ± interquartile range as determined by the Mann- Whitney test (n = 5–6 mice per group). (A–D) shows representative experiment with at least 1 similar replicate.
As the effect of VNS only lasts 48 h (30) and intestinal inflammation is triggered from day 3 following DSS exposure (19), we hypothesized that the beneficial effect of VNS was mediated by improved resistance of the epithelium toward the cytotoxic effect of DSS. Hence, to investigate this hypothesis, mice received VNS prior to DSS and were sacrificed at day 2 (Figure 2A). To confirm that no immune activation was present at day 2, pro-inflammatory cytokine and chemokine expression was assessed in sorted monocytes and immature Mφ and the presence of different leukocyte population was determined using flow cytometry in naïve, sham-operated and VNS-treated mice. As shown in Figure 2B, no significant upregulation could be identified in both VNS-treated and sham-operated mice compared to naïve mice (monocyte Il6 sham- vs. VNS-treated mice p = 0.09; Cxcl1 p = 0.5; immature Mφ Il6 sham- vs. VNS-treated mice p = 0.3; Cxcl1 p = 0.8). In addition, there was no significant increase in the percentage of eosinophils, neutrophils and monocytes compared to naïve mice (Figure 2C).
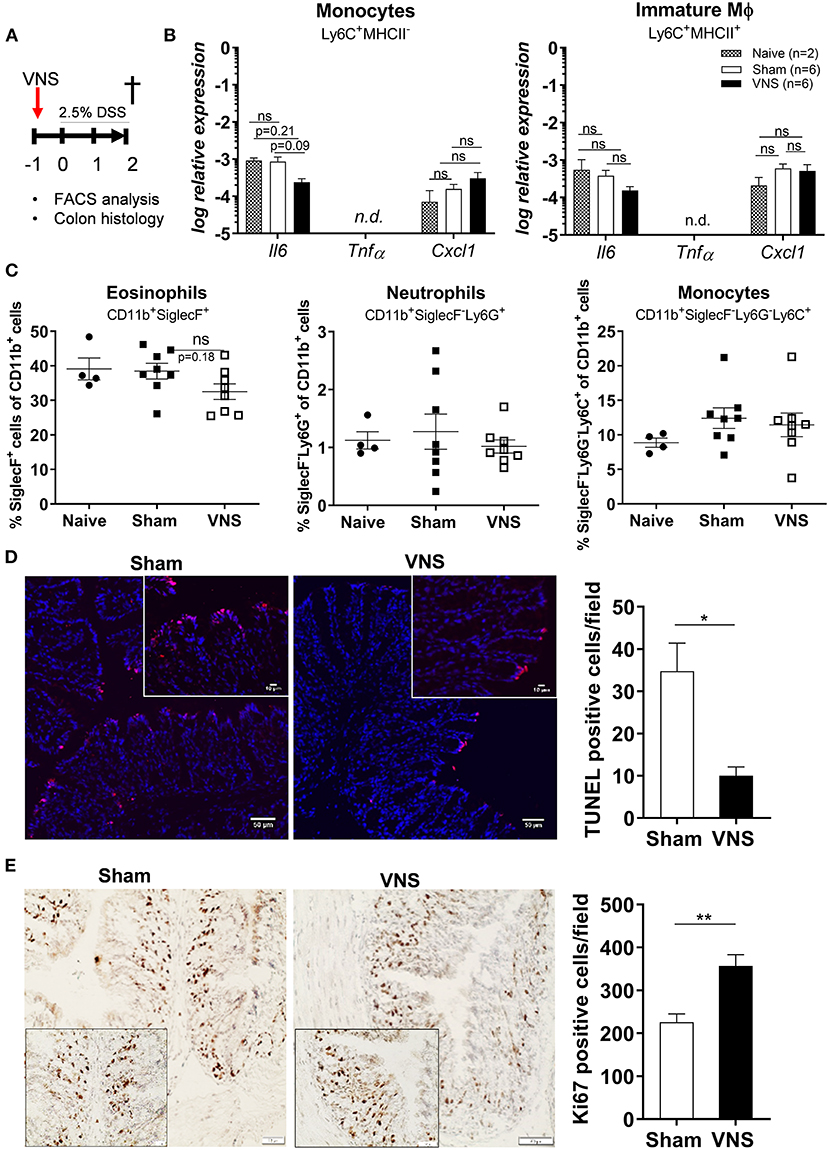
Figure 2. Vagus nerve stimulation tightens the epithelial barrier by reducing apoptosis and promoting proliferation. (A) Wild type mice were stimulated at day−1 and exposed to 2.5% DSS in the drinking water for 2 days, colitis severity was assessed daily. Mice were sacrificed at day 2 and tissue samples were collected for different analysis. (B) Pro-inflammatory cytokine and chemokine expression was quantified in sorted colonic monocytes and immature macrophages (Mφ) isolated from sham and VNS-treated mice at day 2. Data are expressed as mean ± SEM as determined by one-way ANOVA with multiple testing. *p < 0.05; ns, not significant; n.d., not detected; (naïve mice n = 2; sham-operated mice n = 6; VNS-treated mice n = 6). (C) Percentage of eosinophils, neutrophils, monocytes and CD169+ Mφ in lamina propria of sham and VNS-treated mice at day 2. Data are expressed as mean ± SEM as determined by the one-way ANOVA with multiple testing. **p < 0.01 (naïve mice n = 4; sham-operated mice n = 8; VNS-treated mice n = 8). (D,E) Representative images of colonic sections stained for TUNEL (D) and Ki67 (E) from sham- and VNS-stimulated mice and their relative cell count. Scale bars are 50 μm and the scale bar of the inlet is 10 μm. Bar graphs represent TUNEL-positive cells/field (D) and Ki67-positive cells/field (E) of sham- and VNS-treated mice 2 days post colitis induction. (D,E) Data are expressed as mean ± SEM as determined by the unpaired t-test (n = 5–6 mice per group).
Next, we evaluated epithelial damage and integrity by assessing the number of apoptotic cells (Figure 2D) and epithelial cell proliferation using TUNEL and Ki-67 stainings, respectively (Figure 2E). Of interest, VNS significantly reduced the number of apoptotic cells and increased Ki-67-positive cells compared to sham-stimulated mice.
Altogether, these data suggest that in the early phase of DSS-induced colitis, when no inflammatory response is present yet, VNS treatment has a beneficial effect on the disease course, most likely by promoting epithelial proliferation and decreasing apoptosis leading to increased resistance of the epithelium against the toxic effect of DSS.
VNS Treatment During DSS-Induced Colitis Improves Body Weight and Disease Activity
In the second part of this study, we aimed to evaluate the effect of VNS on the immune system during the inflammatory response. Mice received VNS or sham stimulation after 4 days of DSS exposure (Figure 3A). As shown in Figure 3B, VNS successfully prevented body weight loss compared to sham-operated mice. In line, VNS-treated mice showed a significant improvement of DAI compared to sham-operated mice (Figure 3C). Of note, VNS-treated mice only tended to have a lower histological score compared to sham-stimulated mice (Figure 3D). These findings show that VNS applied during DSS colitis is able to ameliorate the course of the disease leading to a faster recovery from colitis.
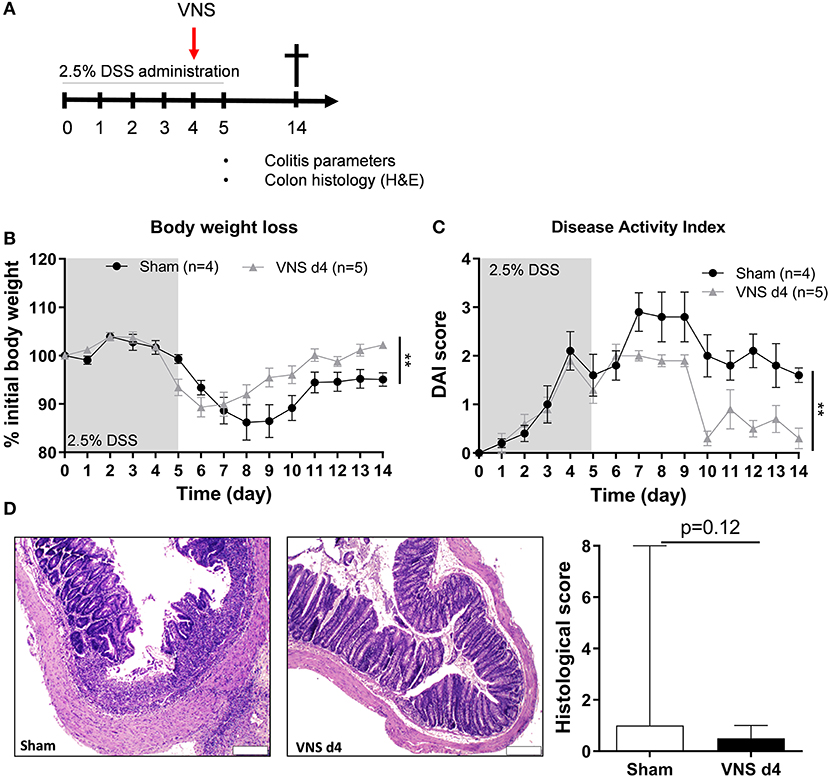
Figure 3. Vagus nerve stimulation during DSS colitis improves body weight and disease activity. (A) Wild type mice were stimulated at day 4 and exposed to 2.5% DSS in the drinking water for 5 days, colitis severity was assessed daily. Mice were sacrificed at day 14 and tissue samples were collected for different analysis. Body weight (B) and disease activity index (C) were assessed daily. Data are expressed as mean ± SEM as determined by the repeated-measures two-way ANOVA test. **p < 0.01; (sham-operated mice n = 4; VNS-treated mice n = 5). (D) Representative images of colonic sections stained with hematoxylin and eosin (H&E) from sham- and VNS-stimulated mice. Scale bars are 200 μm. Bar graphs represent histological injury score of colonic samples of sham- and VNS-treated mice 14 days post colitis induction. Data are expressed as median ± interquartile range as determined by the Mann- Whitney test (sham-operated mice n = 10; VNS-treated mice n = 7). (A–D) shows representative experiment with at least 1 similar replicate.
VNS During DSS-Induced Colitis Controls Innate Immune Cell Influx Into Inflamed Colon Tissue and Dampens the Immune Response
Next, we aimed to study the effect of VNS on the immune response in more detail and to characterize the target cell(s) population affected by VNS. Therefore, neutrophils, monocytes, eosinophil, immature Mφ and mature CD169+ and CD169− Mφ from the colonic lamina propria of day 4 VNS- and sham-stimulated mice were studied and characterized by flow cytometry (Supplementary Figure 1 and Figures 4A,B). As shown in Figure 4B, VNS-treated mice showed a decreased number of eosinophils, neutrophils, monocytes, immature Mφ and mature CD169− Mφ in the lamina propria compared to sham-operated mice. CD169+ Mφ were however not significantly decreased. Similar to whole colon tissue, immature Mφ of VNS-treated mice showed decreased gene expression of Tnfα and Cxcl1 compared to sham-operated mice (Figures 4C,D), while VNS did not affect the pro- and anti-inflammatory cytokine expression in the CD169+ Mφ population. Previous studies also found that electrical VNS dampened leukocyte trafficking (31). To this end, we examined if VNS altered the expression of surface markers associated with migration such as CD11b in the spleen and blood. Interestingly, VNS-treated mice had a reduced percentage and mean fluorescent intensity (MFI) of CD11b+ cells and monocytes in the spleen compared to sham-operated animals (Figure 4E), while the percentage and MFI of CD11b+ cells and monocytes remained unchanged in blood (Figure 4F). These observations suggest that a reduction in surface CD11b levels by VNS might contribute to the observed reduction in inflammatory cells in the intestine. Altogether, these data demonstrate that VNS modulates the inflammatory response during colitis by reducing the influx of inflammatory cells, such as eosinophils, neutrophils, incoming monocytes, and immature Mφ.
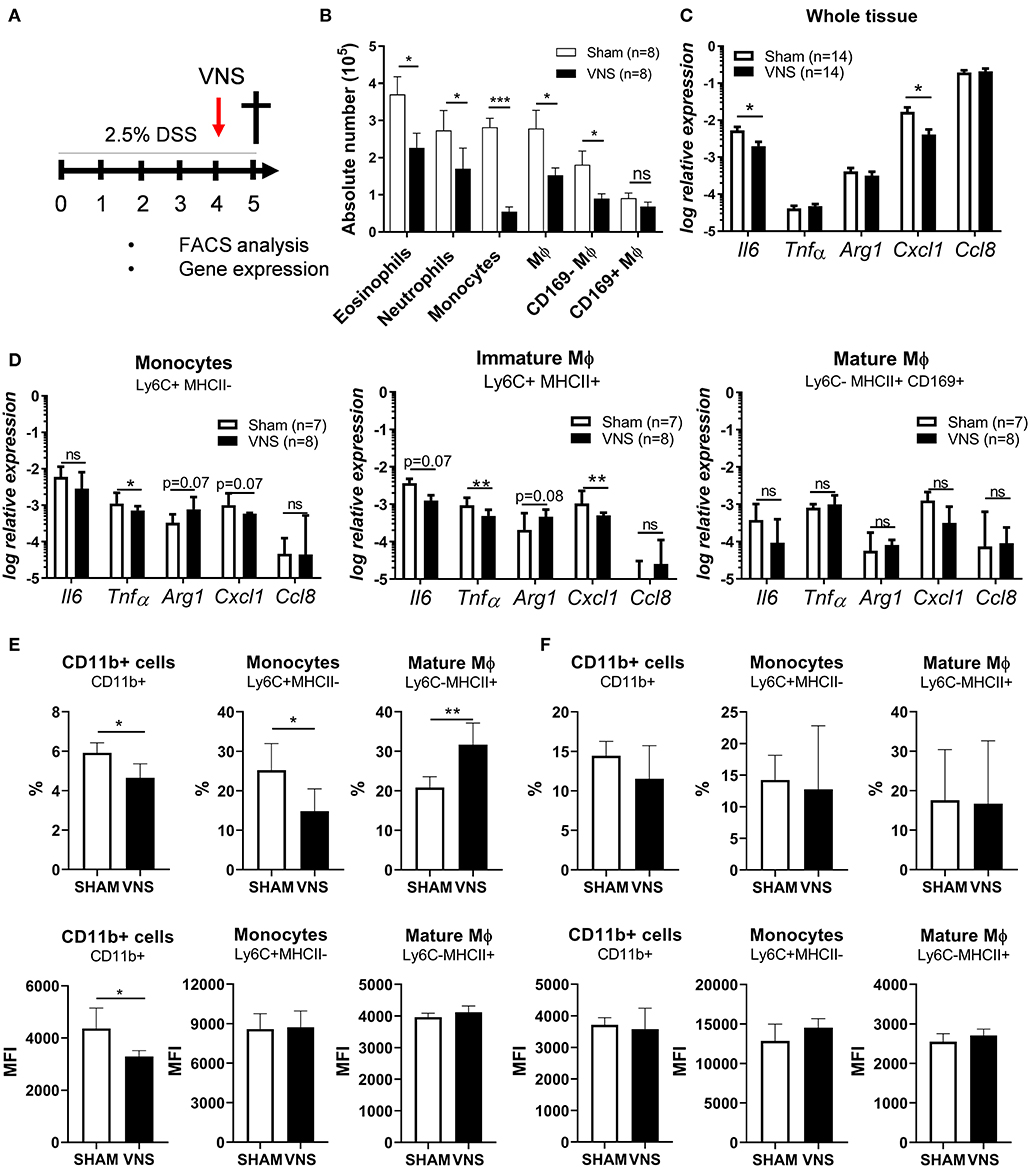
Figure 4. Vagus nerve stimulation affects monocyte recruitment and dampens cytokine production by immature macrophages (Mϕ) during the acute phase of colitis. (A) Wild type mice were stimulated at day 4 and exposed to 2.5% DSS in the drinking water for 5 days, colitis severity was assessed daily. Mice were sacrificed at day 5 and tissue samples were collected for different analysis. (B) Absolute numbers of eosinophils (SiglecF+CD11b+), neutrophils (Ly6G+CD11b+), monocytes (Ly6C+MHCII−) and mature macrophages (Mφ; Ly6C−MHCII+, MCHII+) CD169+ or CD169− Mφ among CD11b+ cells from colon of sham- and VNS-treated mice 1 day post VNS. Data are expressed as mean ± SEM as determined by the unpaired t-test from two independent experiments. ns, not significant; *p < 0.05, **p < 0.01, ***p < 0.001; (n = 8 mice per group). (C,D) Pro-inflammatory cytokine and chemokine expression was quantified in whole tissue (C); sorted colonic monocytes, immature Mφ and mature CD169+ Mφ (D) isolated from sham and VNS-treated mice at day 5. Data are expressed as median ± interquartile range as determined by the Mann-Whitney test from two independent experiments. *p < 0.05, **p < 0.01; ns, not significant; (n = 7–14 mice per group). Data representative of two independent experiments. (E,F) The percentage and MFI of splenic (E) and blood (F) CD11b+ cells, monocytes (Ly6C+MHCII−) and mature Mφ (Mφ; Ly6C−MHCII+, MCHII+) from sham and VNS-treated mice at day 5. Data are expressed as mean ± SEM as determined by unpaired t-test. *p < 0.05, **p < 0.01 (sham-operated mice n = 5; VNS-treated mice n = 5).
Discussion
In the past decades, preclinical studies have demonstrated the beneficial effect of the CAIP in several inflammatory disorders. In the present study, we investigated the prophylactic and therapeutic effect of VNS in a model of DSS-induced colitis. Our results showed that prophylactic VNS was associated with lowered DAI compared to sham-operated animals. These improved clinical parameters are most probably due to the maintenance of the intestinal barrier by the induction of epithelial cell proliferation and decreased cell apoptosis. Of note, prophylactic VNS leads to significant body weight loss compared to the sham-operated animals, an effect most likely due to decreased appetite following the VNS surgical procedure rendering it difficult to appreciate the true beneficial effect of prophylactic VNS in the early disease course of DSS-induced colitis. Nevertheless, we found that therapeutic VNS also decreased DAI and tended to reduce histological score compared to sham-operated animals, which is due to a decreased cytokine expression in incoming monocytes and differentiating Mφ, an effect associated with reduced influx of immune cells into the gut. These observations highlight the fact that VNS should be further investigated as a possible prophylaxis of IBD flares as well as therapeutic treatment during active IBD.
Based on a previous study by Asano et al. (19), we hypothesized that VNS might act through two distinct mechanisms. We proposed that during the initial phase of the disease (i.e., first 48 h), VNS improved the maintenance of intestinal epithelial barrier integrity, which is vital to limit bacterial translocation across the membrane and to prevent a subsequent systemic inflammatory response. Altered intestinal barrier integrity is not only associated with major trauma and septic states, but it is also integral in many intestinal diseases including IBD, stress-induced conditions such as irritable bowel syndrome, food allergy, and other intestinal infections (32). During these pathological conditions, mechanisms such as tight junction rearrangements, decreased mitochondrial function, increased apoptosis, and diminished proliferation of the epithelium can all contribute to barrier disruption (33). In the present study, we showed that prophylactic treatment with VNS significantly increased epithelial cell proliferation and diminished apoptosis compared to sham stimulation. These findings are in line with several previous studies evaluating the effect of VNS on gut barrier function. For instance, in a model of skin burn injury, VNS prevented intestinal barrier injury by decreasing intestinal levels of TNF-α, a phenomenon associated with modulation of the intestinal tight junction proteins, MLCK and MLC, and known to decrease intestinal epithelial permeability (22, 34). Similarly, VNS protects tight junction expression and barrier function in endotoxemic mice and gut ischemia by suppressing NF-kB translocation and downregulating MLCK (33, 35). In our study, no inflammation could be demonstrated after 2 days of DSS treatment, excluding the possibility that a reduction in the activation of the NF-kB pathway and the release of TNF-α or IL-8 can explain the improvement in barrier function. Of interest, however, VNS was previously reported to reduce the amount of intracellular mucus in the tracheal goblet cells of guinea pigs, suggesting increased mucus secretion (36). To what extent increased mucus production contributes to the increased protection against DSS observed in our study remains to be investigated. Of note, however, we showed that VNS applied prior to DSS administration reduces apoptosis and promotes proliferation of intestinal epithelial cells, leading to a milder development of colitis. We speculate that these mechanisms contribute to a better protection against the noxious effect of DSS and may be of interest to prevent disease recurrence in IBD patients, although more experiments are required to further explore this hypothesis.
To investigate if VNS is also effective in reducing active disease, we evaluated the effect of VNS during ongoing DSS colitis. Of interest, we showed that VNS applied on day 4 of DSS administration improved disease outcome, associated with reduced body weight loss and a tendency to restore the normal colonic architecture of the intestine. Importantly, through flow cytometric analysis we observed a significant decrease in the influx of innate immune cells, in particular monocytes, after VNS compared to sham-stimulated mice. As CCL8 produced by CD169+ Mφ has been proposed to be detrimental in attracting monocytes and pro-inflammatory Mφ, we focused on this cell population as potential target of VNS. However, no effect of VNS could be detected on CCL8 expression by CD169+ Mφ arguing against the hypothesis that a reduction of CCL8 production by CD169+ Mφ with subsequent prevention of influx of monocytes and pro-inflammatory Mφ explains the anti-inflammatory effect of VNS in DSS-induced colitis. Instead, we observed decreased expression of the pro-inflammatory cytokines Tnfα and Cxcl1 in Ly6C+ MHCII+ immature Mφ, indicating that these immune cells represent the potential target cells of VNS. The degree of reduction in gene expression was however rather limited suggesting that additional mechanisms might be responsible for the significant reduction in incoming immune cells. Of interest, VNS was also shown to reduce leukocyte trafficking via down-regulation of CD11b, a β2-integrin involved in cell adhesion and leukocyte chemotaxis, a mechanism that takes place in the spleen (31). Alternatively, previous studies have proposed that the anti-inflammatory effect of VNS in experimental DNBS colitis is mediated by altered dendritic—T cell interaction in the spleen (37). This mechanism is however unlikely to explain our findings as DSS colitis is a T cell-independent model of colitis. So clearly, more experiments are required to further explore the underlying mechanisms of the anti-inflammatory effect of VNS in DSS colitis.
In summary, our results show that VNS can improve the maintenance of the intestinal epithelial barrier by promoting epithelial cell proliferation and decreasing cell apoptosis. Secondly, VNS decreases cytokine expression in incoming monocytes and Ly6C+ MHCII+ Mφ, an effect associated with a reduction in influx of immune cells. Our preclinical findings underscore that VNS might be an interesting therapeutic approach to treat inflammatory disorders such as IBD. With this study, we shed new light on the mechanism of action of VNS and its cellular target, however, the exact molecular mechanisms still need to be further investigated.
Data Availability Statement
The raw data supporting the conclusions of this article will be made available by the authors, without undue reservation.
Ethics Statement
The animal study was reviewed and approved by Animal Care and Animal Experiments Committee of KU Leuven.
Author Contributions
EM, NS, GM, and GB planned and designed experiments. MS, JA-L, MF, MD, VS, GD, GG, and GM performed or supervised the experiments. EM, NS, and GB reviewed data and wrote the manuscript. All authors corrected and approved the final version of the manuscript.
Funding
This work was supported by the European Research Council (ERC) Advanced Grant (ERC-340101-Cholstim) to GB. GB was also supported by Flanders Fund for Innovation by Science and Technology (IWT-TBM; 110699), Research Foundation—Flanders (FWO): Odysseus programme (G.0905.07), and two FWO grants (G.0566.12N and G.0890.18N). NS (12V3619N), PG-P, JA-L (12X9820N), and GG were supported by a postdoctoral research fellowship of FWO. MS was supported by a PhD fellowship of FWO. GM was supported by an FWO grant (G.0D83.17N) and by KU Leuven grants (ZKD2906-C14/17/097 and ZKC9531-C12/15/016).
Conflict of Interest
The authors declare that the research was conducted in the absence of any commercial or financial relationships that could be construed as a potential conflict of interest.
Acknowledgments
The authors would like to thank I. Appeltans for her excellent technical assistance. Tissue imaging was performed at the Cell Imaging Core (KU Leuven, Belgium). Cell sorting and flow cytometry was done at the FACS core facility (KU Leuven, Belgium).
Supplementary Material
The Supplementary Material for this article can be found online at: https://www.frontiersin.org/articles/10.3389/fmed.2021.694268/full#supplementary-material
References
1. Borovikova LV, Ivanova S, Nardi D, Zhang M, Yang H, Ombrellino M, et al. Role of vagus nerve signaling in CNI-1493-mediated suppression of acute inflammation. Autonom Neurosci Basic Clin. 85:141–7 (2000). doi: 10.1016/S1566-0702(00)00233-2
2. de Jonge WJ, van der Zanden EP, The FO, Bijlsma MF, van Westerloo DJ, Bennink RJ. Stimulation of the vagus nerve attenuates macrophage activation by activating the Jak2-STAT3 signaling pathway. Nat Immunol. (2005) 6:844–51. doi: 10.1038/ni1229
3. Matteoli G, Gomez-Pinilla PJ, Nemethova A, Di Giovangiulio M, Cailotto C, van Bree SH, et al. A distinct vagal anti-inflammatory pathway modulates intestinal muscularis resident macrophages independent of the spleen. Gut. (2013) 1136:gutjnl-2013–304676. doi: 10.1136/gutjnl-2013-304676
4. Stakenborg N, Wolthuis AM, Gomez-Pinilla PJ, Farro G, Di Giovangiulio M, Bosmans G, et al. Abdominal vagus nerve stimulation as a new therapeutic approach to prevent postoperative ileus. Neurogastroenterol Motil. (2017) 2017:13075. doi: 10.1111/nmo.13075
5. The FO, Boeckxstaens GE, Snoek SA, Cash JL, Bennink R, Larosa GJ. Activation of the cholinergic anti-inflammatory pathway ameliorates postoperative ileus in mice. Gastroenterology. (2007) 133:1219–28. doi: 10.1053/j.gastro.2007.07.022
6. Wang H, Yu M, Ochani M, Amella CA, Tanovic M, Susarla S, et al. Nicotinic acetylcholine receptor alpha7 subunit is an essential regulator of inflammation. Nature. (2003) 421:384–8. doi: 10.1038/nature01339
7. Stakenborg N, Di Giovangiulio M, Boeckxstaens GE, Matteoli G. The versatile role of the vagus neve in the gastrointestinal tract. EMJ Gastroenterol. (2013) 1:106–14. Available online at: https://www.emjreviews.com/gastroenterology/article/the-versatile-role-of-the-vagus-nerve-in-the-gastrointestinal-tract/
8. Koopman FA, Chavan SS, Miljko S, Grazio S, Sokolovic S, Schuurman PR, et al. Vagus nerve stimulation inhibits cytokine production and attenuates disease severity in rheumatoid arthritis. Proc Natl Acad Sci USA. (2016) 113:8284–9. doi: 10.1073/pnas.1605635113
9. Bonaz B, Sinniger V, Hoffmann D, Clarencon D, Mathieu N, Dantzer C, et al. Chronic vagus nerve stimulation in Crohn's disease: a 6-month follow-up pilot study. Neurogastroenterol Motil. (2016) 2016:12792. doi: 10.1111/nmo.12792
10. Cohen LJ, Cho JH, Gevers D, Chu H. Genetic factors and the intestinal microbiome guide development of microbe-based therapies for inflammatory bowel diseases. Gastroenterology. (2019) 3:17. doi: 10.1053/j.gastro.2019.03.017
11. Dalal SR, Chang EB. The microbial basis of inflammatory bowel diseases. J Clin Invest. (2014) 124:4190–6. doi: 10.1172/JCI72330
12. Di Giovangiulio M, Verheijden S, Bosmans G, Stakenborg N, Boeckxstaens GE, Matteoli G. The neuromodulation of the intestinal immune system and its relevance in inflammatory bowel disease. Front Immunol. (2015) 6:590. doi: 10.3389/fimmu.2015.00590
13. Meroni E, Stakenborg N, Viola MF, Boeckxstaens GE. Intestinal macrophages and their interaction with the enteric nervous system in health and inflammatory bowel disease. Acta Physiol. (2019) 225:e13163. doi: 10.1111/apha.13163
14. Meregnani J, Clarencon D, Vivier M, Peinnequin A, Mouret C, Sinniger V, et al. Anti-inflammatory effect of vagus nerve stimulation in a rat model of inflammatory bowel disease. Autonom. Neurosci. Basic Clin. (2011) 160:82–9. doi: 10.1016/j.autneu.2010.10.007
15. Ji H, Rabbi MF, Labis B, Pavlov VA, Tracey KJ, Ghia JE. Central cholinergic activation of a vagus nerve-to-spleen circuit alleviates experimental colitis. Mucosal Immunol. (2014) 7:335–47. doi: 10.1038/mi.2013.52
16. Di Giovangiulio M, Bosmans G, Meroni E, Stakenborg N, Florens M, Farro G, et al. Vagotomy affects the development of oral tolerance and increases susceptibility to develop colitis independently of the alpha-7 nicotinic receptor. Mol Med. (2016) 22:62. doi: 10.2119/molmed.2016.00062
17. Ghia JE, Blennerhassett P, El-Sharkawy RT, Collins SM. The protective effect of the vagus nerve in a murine model of chronic relapsing colitis. Am J Physiol Gastrointest Liver Physiol. (2007) 293:G711–8. doi: 10.1152/ajpgi.00240.2007
18. Ghia JE, Blennerhassett P, Kumar-Ondiveeran H, Verdu EF, Collins SM. The vagus nerve: a tonic inhibitory influence associated with inflammatory bowel disease in a murine model. Gastroenterology. (2006) 131:1122–30. doi: 10.1053/j.gastro.2006.08.016
19. Asano K, Takahashi N, Ushiki M, Monya M, Aihara F, Kuboki E, et al. Intestinal CD169(+) macrophages initiate mucosal inflammation by secreting CCL8 that recruits inflammatory monocytes. Nat Commun. (2015) 6:7802. doi: 10.1038/ncomms8802
20. Arrieta MC, Madsen K, Doyle J, Meddings J. Reducing small intestinal permeability attenuates colitis in the IL10 gene-deficient mouse. Gut. (2009) 58:41–8. doi: 10.1136/gut.2008.150888
21. Turner JR. Intestinal mucosal barrier function in health and disease. Nat Rev Immunol. (2009) 9:799–809. doi: 10.1038/nri2653
22. Krzyzaniak M, Peterson C, Loomis W, Hageny AM, Wolf P, Reys L, et al. (2011). Postinjury vagal nerve stimulation protects against intestinal epithelial barrier breakdown. J Trauma 70:1168–75. doi: 10.1097/TA.0b013e318216f754
23. Cheadle GA, Costantini TW, Bansal V, Eliceiri BP, Coimbra R. Cholinergic signaling in the gut: a novel mechanism of barrier protection through activation of enteric glia cells. Surg Infect. (2014) 15:387–93. doi: 10.1089/sur.2013.103
24. Stakenborg N, Labeeuw E, Gomez-Pinilla PJ, De Schepper S, Aerts R, Goverse G, et al. Preoperative administration of the 5-HT4 receptor agonist prucalopride reduces intestinal inflammation and shortens postoperative ileus via cholinergic enteric neurons. Gut. (2018) 2018:317263. doi: 10.1136/gutjnl-2018-317263
25. Bosmans G, Appeltans I, Stakenborg N, Gomez-Pinilla PJ, Florens MV, Aguilera-Lizarraga J, et al. Vagus Nerve Stimulation dampens intestinal inflammation in a murine model of experimental Food Allergy. Allergy. (2019) 2019:13790. doi: 10.1111/all.13790
26. Cooper HS, Murthy SN, Shah RS, Sedergran DJ. Clinicopathologic study of dextran sulfate sodium experimental murine colitis. Lab Invest. (1993) 69:238–49.
27. Ito R, Shin-Ya M, Kishida T, Urano A, Takada R, Sakagami J, et al. Interferon-gamma is causatively involved in experimental inflammatory bowel disease in mice. Clin Exp Immunol. (2006) 146:330–8. doi: 10.1111/j.1365-2249.2006.03214.x
28. De Schepper S, Verheijden S, Aguilera-Lizarraga J, Viola MF, Boesmans W, Stakenborg N, et al. Self-maintaining gut macrophages are essential for intestinal homeostasis. Cell. (2018) 175:400–15 e413. doi: 10.1016/j.cell.2018.07.048
29. Val-Laillet D, Biraben A, Randuineau G, Malbert CH. Chronic vagus nerve stimulation decreased weight gain, food consumption and sweet craving in adult obese minipigs. Appetite. (2010) 55:245–52. doi: 10.1016/j.appet.2010.06.008
30. Olofsson PS, Levine YA, Caravaca A, Chavan SS, Pavlov VA, Faltys M, et al. Single-pulse and unidirectional electrical activation of the cervical vagus nerve reduces tumor necrosis factor in endotoxemia. Bioelectron Med. (2015) 2:37–42. doi: 10.15424/bioelectronmed.2015.00006
31. Huston JM, Rosas-Ballina M, Xue X, Dowling O, Ochani K, Ochani M, et al. Cholinergic neural signals to the spleen down-regulate leukocyte trafficking via CD11b. J Immunol. (2009) 183:552–9. doi: 10.4049/jimmunol.0802684
32. Schulzke JD, Bojarski C, Zeissig S, Heller F, Gitter AH, Fromm M. Disrupted barrier function through epithelial cell apoptosis. Ann Ny Acad Sci. (2006) 1072:288–99. doi: 10.1196/annals.1326.027
33. Hu S, Du MH, Luo HM, Wang H, Lv Y, Ma L, et al. Electroacupuncture at Zusanli (ST36) prevents intestinal barrier and remote organ dysfunction following Gut Ischemia through activating the cholinergic anti-inflammatory-dependent mechanism. Evid Based Complement Alternat Med. (2013) 2013:592127. doi: 10.1155/2013/592127
34. Costantini TW, Bansal V, Peterson CY, Loomis WH, Putnam JG, Rankin F, et al. Efferent vagal nerve stimulation attenuates gut barrier injury after burn: modulation of intestinal occludin expression. J Trauma. (2010) 68:1349–54. doi: 10.1097/TA.0b013e3181dccea0
35. Zhou H, Liang H, Li ZF, Xiang H, Liu W, Li JG. Vagus nerve stimulation attenuates intestinal epithelial tight junctions disruption in endotoxemic mice through alpha7 nicotinic acetylcholine receptors. Shock. (2013) 40:144–51. doi: 10.1097/SHK.0b013e318299e9c0
36. Kaser A, Zeissig S, Blumberg RS. Inflammatory bowel disease. Annu Rev Immunol. (2010) 28:573–621. doi: 10.1146/annurev-immunol-030409-101225
Keywords: vagus nerve stimulation, dextran sodium sulfate, inflammatory bowel diseases, macrophage - cell, epithelial barrier
Citation: Meroni E, Stakenborg N, Gomez-Pinilla PJ, Stakenborg M, Aguilera-Lizarraga J, Florens M, Delfini M, de Simone V, De Hertogh G, Goverse G, Matteoli G and Boeckxstaens GE (2021) Vagus Nerve Stimulation Promotes Epithelial Proliferation and Controls Colon Monocyte Infiltration During DSS-Induced Colitis. Front. Med. 8:694268. doi: 10.3389/fmed.2021.694268
Received: 12 April 2021; Accepted: 07 June 2021;
Published: 07 July 2021.
Edited by:
Mohammad Bashashati, Texas Tech University Health Sciences Center, United StatesReviewed by:
Marta Castro, University of Zaragoza, SpainNicolas Cenac, INSERM U1220 Institut de Recherche en Santé Digestive, France
Copyright © 2021 Meroni, Stakenborg, Gomez-Pinilla, Stakenborg, Aguilera-Lizarraga, Florens, Delfini, de Simone, De Hertogh, Goverse, Matteoli and Boeckxstaens. This is an open-access article distributed under the terms of the Creative Commons Attribution License (CC BY). The use, distribution or reproduction in other forums is permitted, provided the original author(s) and the copyright owner(s) are credited and that the original publication in this journal is cited, in accordance with accepted academic practice. No use, distribution or reproduction is permitted which does not comply with these terms.
*Correspondence: Guy E. Boeckxstaens, Z3V5LmJvZWNreHN0YWVucyYjeDAwMDQwO2t1bGV1dmVuLmJl
†These authors have contributed equally to this work