- 1Department of Medical Science, Faculty of Medicine, University of Brasilia, Brasilia, Brazil
- 2Faculty of Medicine, CESMAC University Center, Maceio, Brazil
- 3Department of Health Science, School of Medicine, University Center of Brasilia (UniCEUB), Brasilia, Brazil
- 4Laboratory of Protein Chemistry and Biochemistry, Department of Cell Biology, Institute of Biological Sciences, University of Brasilia, Brasilia, Brazil
- 5Faculty of Medicine, Federal University of Goias, Goiania, Brazil
Age-related macular degeneration (AMD) is among the world's leading causes of blindness. In its neovascular form (nAMD), around 25% of patients present further anatomical and visual deterioration due to persistence of neovascular activity, despite gold-standard treatment protocols using intravitreal anti-VEGF medications. Thus, to comprehend, the molecular pathways that drive choroidal neoangiogenesis, associated with the vascular endothelial growth factor (VEGF), are important steps to elucidate the mechanistic events underneath the disease development. This is a pilot study, a prospective, translational experiment, in a real-life context aiming to evaluate the protein profiles of the aqueous humor of 15 patients divided into three groups: group 1, composed of patients with nAMD, who demonstrated a good response to anti-VEGF intravitreal injections during follow-up (good responsive); group 2, composed of patients with anti-VEGF-resistant nAMD, who demonstrated choroidal neovascularization activity during follow-up (poor/non-responsive); and group 3, composed of control patients without systemic diseases or signs of retinopathy. For proteomic characterization of the groups, mass spectrometry (label-free LC-MS/MS) was used. A total of 2,336 proteins were identified, of which 185 were distinctly regulated and allowed the differentiation of the clinical conditions analyzed. Among those, 39 proteins, including some novel ones, were analyzed as potential disease effectors through their pathophysiological implications in lipid metabolism, oxidative stress, complement system, inflammatory pathways, and angiogenesis. So, this study suggests the participation of other promising biomarkers in neovascular AMD, in addition to the known VEGF.
Introduction
Age-related macular degeneration (AMD) is expected to affect 288 million people in 2040 (1) and currently represents the primary cause of severe visual loss in patients over 50 years of age in industrialized countries (1–8) with its incidence quadrupling every decade of life (9, 10). Progressive visual impairment in AMD occurs due to degeneration of the photoreceptors and retinal pigment epithelium (RPE) or when new blood vessels form in the center of the retina (6, 11–13). The main symptoms are blurred, distorted, or absent central vision, and patients have problems in reading, driving, and recognizing faces (5, 8, 14).
The most aggressive form of the disease is the neovascular age-related macular degeneration (nAMD), for which an antiangiogenic (anti-VEGF) therapy is considered the gold-standard treatment (15–17). Although anti-VEGF therapy with bevacizumab, ranibizumab, and aflibercept proved important efficacious in the majority of patients, deterioration of visual acuity and persistence of the disease (choroidal neovascularization activity) are observed in about 25% of treated patients (18). These patients represent patients with poor/non-responsive nAMD to anti-VEGF group. Additionally, their definitions are based on morphological and functional criteria or combination of both (18).
Genomics provides important diagnostic information for monogenic diseases and helps to elucidate the risk of developing others. On the other hand, proteomics allows the analysis of the posttranslation profile of numerous diseases, which becomes a strategic tool to assess the severity of the disease and to identify therapeutic targets (19).
The protein global profile of neovascular AMD is not fully defined (20). This study was designed to obtain new information on its pathophysiology, staging (phenotypes), and relative resistance to standard therapy, corroborated with the identification of possible early diagnosis biomarkers and new therapeutic targets, even if as adjuvants.
Patients and Methods
Study Design
It is characterized as a pilot study, a prospective and translational experiment in a real-life context. The initial sampling effort, a number of individuals who were invited to participate in the research, was 250 enrolled patients, of which 30 met the inclusion and exclusion criteria (Figure 1). It was previously defined that each group would have five patients with aqueous humor samples collected at the Brazilian Vision Center (Brasília, Brazil), from January 17 to July 18. In the follow-up of these patients, the response to 06-month intravitreal injections of anti-VEGF was analyzed for retinography and spectral-domain optical coherence tomography (OCT) (Figure 2). Due to the response to anti-VEGF therapy, the first 5 that presented a good response to treatment formed group 1 (good responsive), the first 5 that presented poor or non-response formed group 2 (poor/non-responsive). Group 3 (control group) was composed of 5 patients with cataracts, without systemic diseases or signs of retinopathy (Table 1).
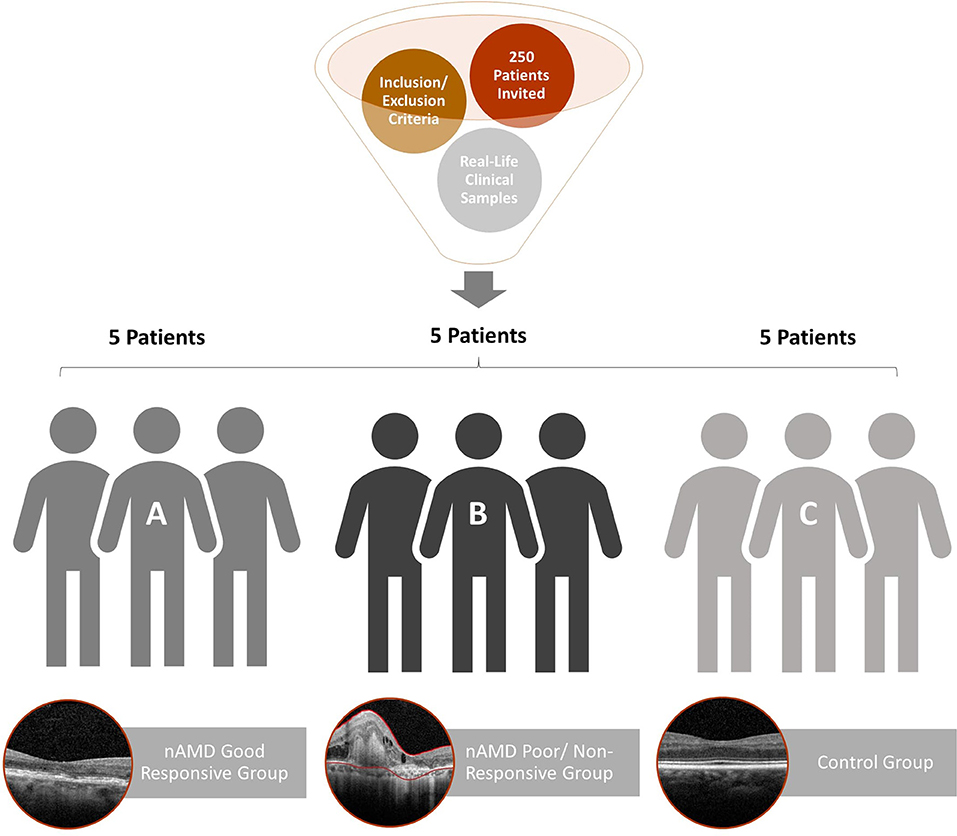
Figure 1. Flowchart of patient recruitment and exclusion for aqueous humor proteome analysis. The 15 patients selected were separated into three groups: (A) good response to anti-VEGF therapy (nAMD good responsive); (B) resistance to anti-VEGF therapy (nAMD poor/non-responsive); (C) control patients without systemic diseases or signs of retinopathy.
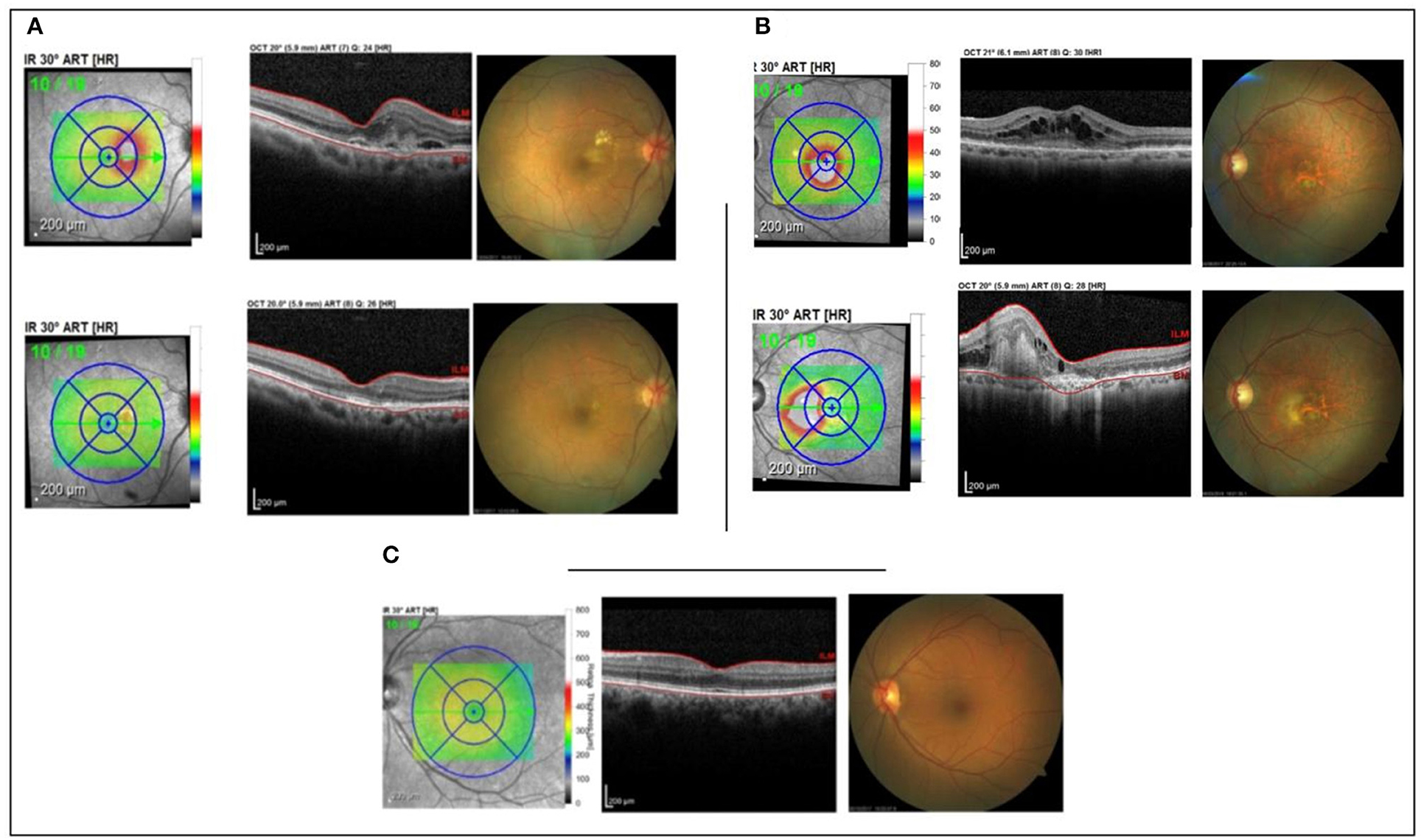
Figure 2. Patient's OCT. (A) good responsive group; (B) poor/non-responsive group; (C) control group.
Ethics Statement
This research was approved by the Institution's Research Ethics Committee (CAAE 64921317.1.0000.5667–Platafoma Brazil). Written informed consent was obtained from the individuals for the publication of any potentially identifiable images or data included in this article.
Inclusion Criteria
These included patients with AMD without other ocular and systemic comorbidities. The patients who were included in this real-life study were classified according to the functional (visual acuity) and morphological (presence/absence of subretinal fluid, intraretinal fluid, intra-retinal cysts, and increase in central retinal thickness) criteria, evaluated by clinical examination and by spectral domain OCT [Amoaku et al. (21), Supplementary Table S1]. Follow-up was at least 6-month injections of anti-VEGF (ranibizumab 0.5 mg/0.05 ml or aflibercept 2 mg/0.05 ml). The best-corrected visual acuity (MAVC) was between 20/25 and 20/400 according to the Snellen table.
Exclusion Criteria
These included patients who presented signs of retinopathy other than AMD. Patients with senile cataract (physiological lens aging) within the control group, who showed any signs of evident retinopathy, were excluded. All patients with a history of intraocular surgery other than anti-VEGF injections in the 6 months prior to the start of the research were excluded.
Sample Collection
The sample was 0.05 ml of aqueous humor collected by anterior chamber paracentesis, using an ultrafine 30-G disposable syringe needle, before the application of anti-VEGF, performed by an experienced surgeon, in an operating room.
Sample Preparation
Samples were diluted in 0.02M TEAB solution and sonicated with 40% intensity in 3 cycles of 10 s each using a Tip Sonicador Q125 (QSonica). Protein concentrates were quantified using Qubit™ method (Invitrogen). Aliquots containing 10 μg of protein were submitted to trypsin in-solution digestion, and the resulting peptide samples were desalted using homemade C18 reverse-phase microcolumns (22). The peptide concentration was also determined using Qubit™ protein assay.
Mass Spectrometry
The LC-MS/MS analyses were performed on a Dionex Ultimate 3000 RSLCnano UPLC system (Thermo Fisher Scientific) coupled to an LTQ-Orbitrap Elite mass spectrometer (Thermo Fisher Scientific). Aliquots containing 1 μg of peptides were injected into a homemade precolumn (100 μm internal diameter x 3 cm long) with spherical silica particles coated with 5 μm C18 Reprosil-Pur and 120Å pores to remove salt residues. Subsequently, these peptides were fractionated in a homemade analytical column (75 μm internal diameter x 24 cm long) with 3 μm C18 Reprosil-Pur particles and 120Å pores. The linear elution gradient between solvents A (0.1% formic acid in water) and B (0.1% formic acid in acetonitrile) was composed of 2 to 35% B over 155 min. Mass spectra were acquired in positive mode with data-dependent MS/MS spectra acquisition (DDA). In MS1, high-resolution spectra (120000 FWHM) between 300 and 1,650 m/z were obtained in the Orbitrap analyzer. Each scan was followed by MS2 in the ion trap analyzer of the 20 most intense ions above the required minimum signal of 3,000 by the collision-induced dissociation (CID) method. The reanalysis of already fragmented ions was inhibited by dynamic exclusion, thus favoring the identification of less abundant peptides.
Data Analysis
Spectra were processed by Progenesis QI software (http://www.nonlinear.com/progenesis/qi) (Nonlinear Dynamics ©), where they were first submitted to the chromatographic retention time alignment and then quantified according to the area of integrated intensity of the peaks recovered from the extracted ion chromatogram (XIC). Statistically significant events (ANOVA p ≤ 0.05) had their MS2 spectra exported for the identification on the Peaks® Studio 7.0 platform (http://www.bioinfor.com/peaks-studio) (Bioinformatics Solutions, Inc.) obeying the following parameters: proteins with a minimum of 2 peptides, homo sapiens taxonomy, UniProtKB database–SWISS-PROT/TrEMBL (April/2019), MS1 accuracy of 10 ppm; MS2 accuracy of 0.5 Da; up to two missed cleavage sites; carbamidomethylation of cysteines as fixed modification; methionine oxidation as a variable modification. Number and groups of proteins and number of peptides were filtered with false-discovery rate (FDR) < 0.01 before the identification list was exported to Progenesis QI for analysis of variance (p ≤ 0.05), PCA and dendogram. MetaboAnalyst 4.0® (http://www.metaboanalyst.ca) (23) and String (https://string-db.org/) (24) platforms completed the data refinement based on the experimental design. To unify gene representations and the attributes of the various gene products, the proteins identified in this study were submitted to gene ontology (GO term) for molecular function, biological process, and analysis of cellular components.
Results
In the aqueous humor samples analyzed, a total of 2,336 proteins were identified, using an FDR 1% of which 185 were statistically significant (ANOVA p < 0.05) and allowed the distinction between the three groups analyzed in this study (Figure 3). Thirty-nine proteins were correlated with visual function or with metabolic pathways directly or indirectly linked to choroidal neoangiogenesis, an essential condition for neovascular AMD (Table 2). Additionally, according to the String program pattern, most of the selected proteins play important roles at a functional protein-protein interaction network (Figure 4). Using supervised multivariate analysis, as partial least squares discriminant analysis (PLS-DA), it was possible to obtain the variable importance projection scores (VIP scores) and identify the 30 proteins which are most discriminants within each scenario (Figure 5).
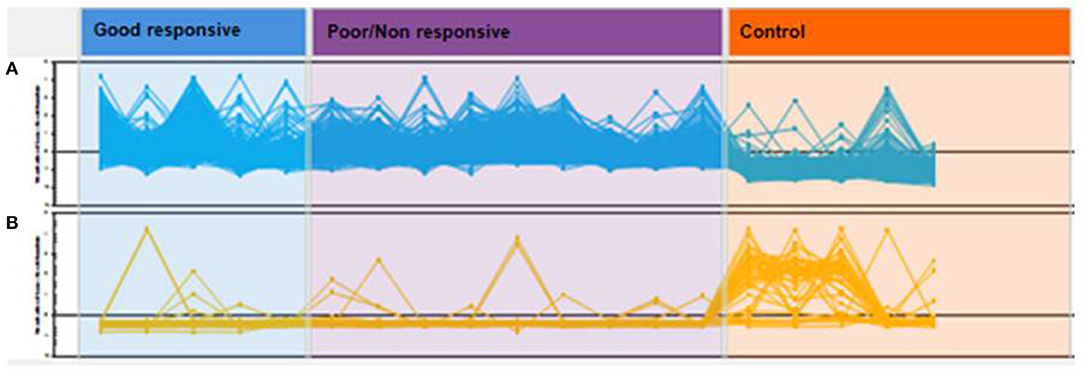
Figure 3. Hierarchic cluster analyses (HCA) of global protein profiles. Each line represents a protein and each peak or valley, its abundance per patient. Proteins in (A) have a predominance of positive regulation in cases of good responsive nAMD and poor/non-responsive nAMD with the predominance of negative regulation in controls. In (B), the opposite relationship is observed.
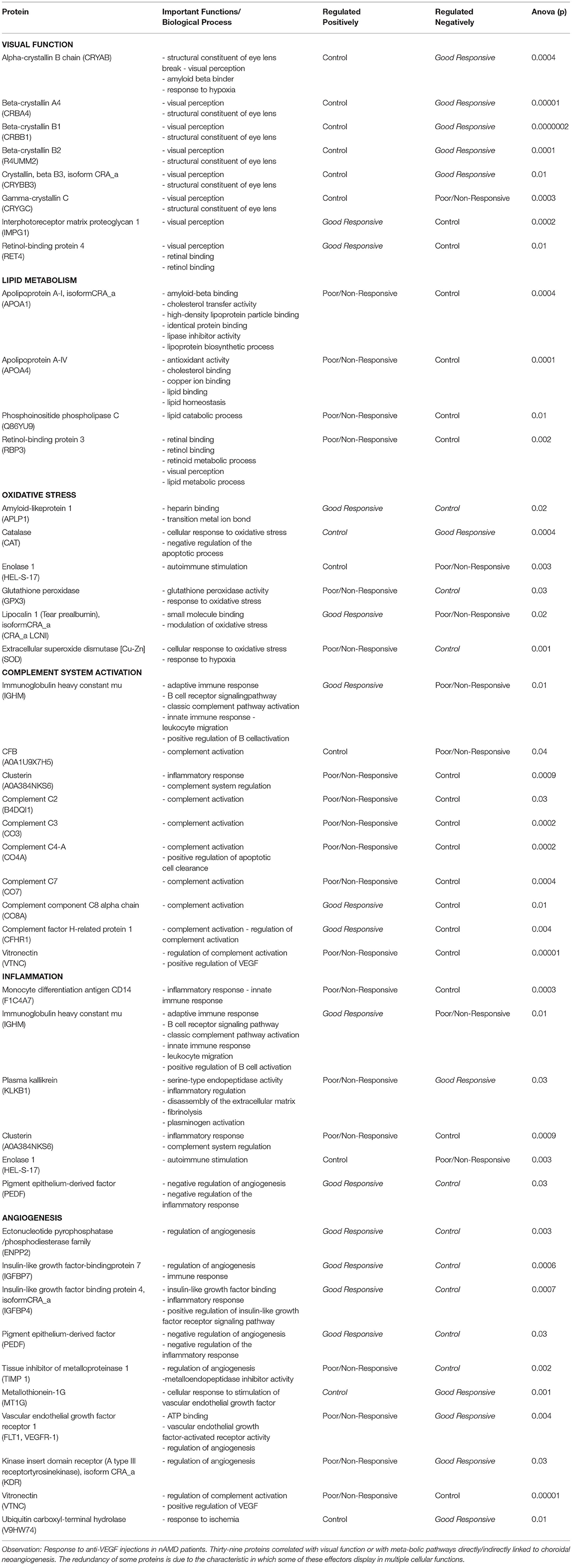
Table 2. Selected discriminant proteins by metabolic function related to the pathophysiology of AMD.
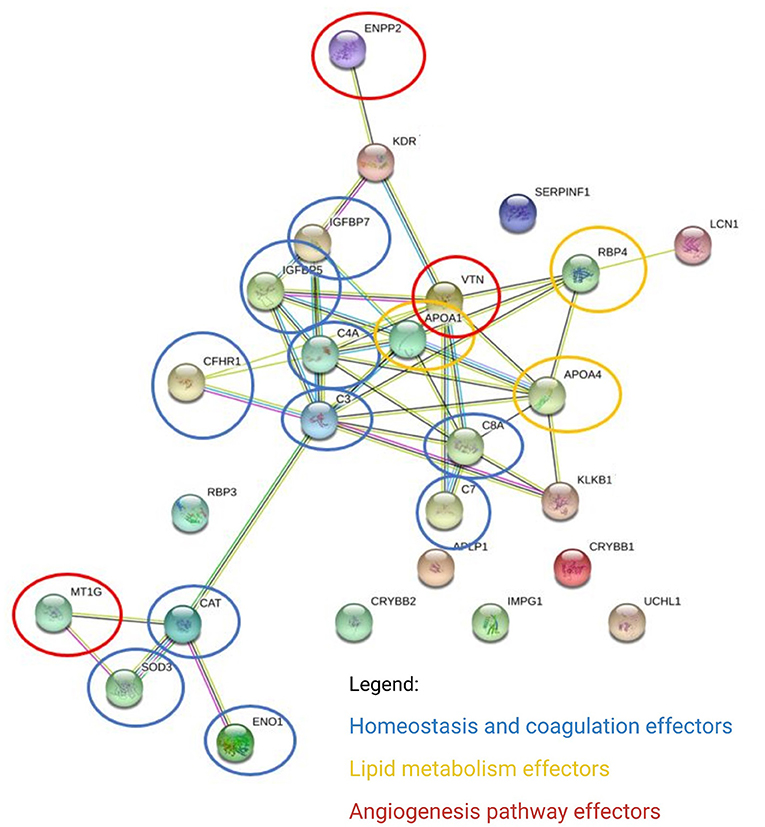
Figure 4. Differentially expressed proteins converge on main pathways by biological processes. The links between proteins represent interactions, according to the pattern of the String program (protein-protein interaction network enriched with functional analysis). ENPP2, ectonucleotide pyrophosphatase/ phosphodiesterase family member 2; KDR, kinase insert domain receptor (A type III receptor tyrosine kinase), isoform CRA_a; IGFBP7, insulin-like growth factor-binding protein 7; IGFBP5, insulin-like growth factor-binding protein 5; CFHR1, complement factor H-related protein 1; C4A, complement C4-A; APOA1, apolipoprotein A-I, isoform CRA_a; VTNC, vitronectin; C3, tetranectin; C8A, complement component C8 alpha-chain; APOA4, apolipoprotein A-IV; RBP4, retinol-binding protein 4; SERPINF1, pigment epithelium-derived factor; LCN1, lipocalin 1 (tear prealbumin), isoform CRA_a; C7, complement component C7; APCP1, amyloid-like protein 1; CRYBB2, beta-crystallin B2; IMPG1, interphotoreceptor matrix proteoglycan 1; CRYBB1, beta-crystallin B1; UCHL1, ubiquitin carboxy-terminal hydrolase; KLKB1, plasma kallikrein; RBP3, retinol-binding protein 3; CAT, cathepsin D; MT1G, metallothionein 1G; SOD3, extracellular superoxide dismutase [Cu-Zn]; ENO1, enolase 1 (alpha), isoform CRA_a.
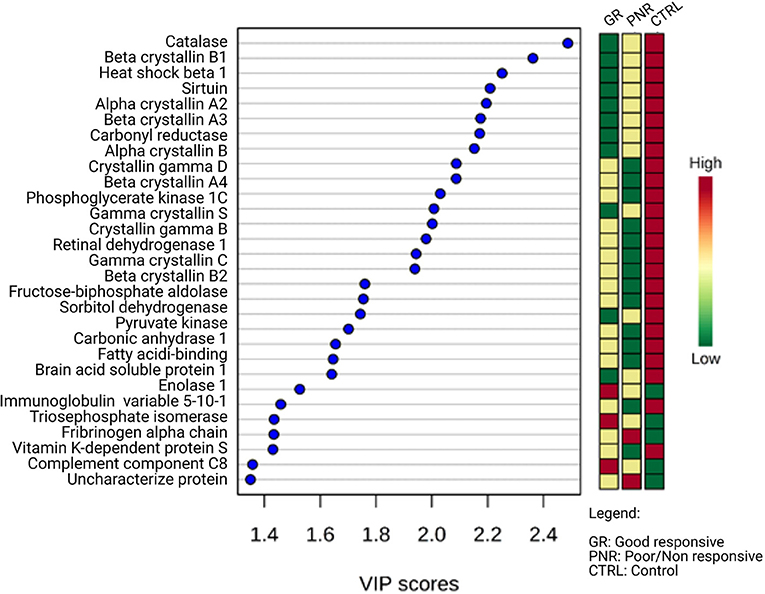
Figure 5. Multivariate analyses by VIP scores of regulated proteins in aqueous humor of patients with good responsive nAMD, poor/non-responsive nAMD, and control patients (PLS-DA imp. features). Among the 30 regulated proteins with the highest VIP score, 25 have negative regulation in pathological scenarios. The colored boxes on the right indicate the relative intensity of each protein in the respective scenarios.
A posteriori statistical power analysis demonstrated that five patients per group were sufficient to achieve statistical robustness, with all samples having a statistical power > 0.8 (86.4%), which means that the experimental design allowed 84% of the data to present statistical power >0.8.
Discussion
In addition to the vascular endothelial growth factor (VEGF) pathway, some other pathophysiogenic pathways in nAMD are described in the literature, and choroidal neoangiogenesis has been already correlated with oxidative stress, complement system activation, immune system activation, and lipid metabolism (25–28). This study was able to identify 39 proteins related to visual function or to at least one alternative pathophysiological pathway of neovascular AMD (Table 2).
Drusen, one of the main signs of AMD, correspond to an accumulation of cellular debris, including proteins, minerals, and lipids, between Bruch's membrane and the RPE. Thompson et al. (29) proposed a new mechanism for drusen formation and growth. They confirmed the presence of calcium phosphate (hydroxyapatite, HAp) in small hollow spheres containing cholesterol in all sub-RPE deposits in the macula and in the periphery. Using immunohistochemistry, they showed that numerous proteins coated those small spheres, such as complement factor H, vitronectin (VTNC), and beta-amyloid. Lipids and lipoproteins that contain cholesterol, which is produced and secreted at least in part by the RPE (30), are recruited and retained in the aged Bruch's membrane (31, 32), which in turn, in a self-directed oligomerization process, facilitates additional depositions that ultimately lead to drusen formation and growth (33). Studies have also established analogies between the oxidation of lipoproteins in Bruch's membrane and atherosclerosis (33–37).
Drusen function as a barrier between the choriocapillary layer and the photoreceptors, limiting the oxygenation and nutritional support of cones and rods and generating local hypoxia, a fundamental agent in the pathophysiogenesis of AMD (38).
Physiopathological Pathways Described for AMD
Visual Function
Crystallins, the main components of the lens, also act in the regulation of astrocytes, the remodeling of retinal vessels (39), the regulation of apoptosis (40), and the depuration of the external segments of photoreceptors by the RPE (41). In neovascular AMD, some serum markers (42) and numerous autoantibodies and immune complexes against α- and β-crystallins have been demonstrated in the retina and choroid (43). The α-crystallins are expressed in the cytosol and mitochondria of RPE cells, protecting them from oxidative stress. They function as angiogenic and VEGF modulators. Chaperone proteins derived from α-crystallins function as inhibitors of oxidation-induced cell death (44). The β-crystallin proteins and interphotoreceptor matrix proteoglycan 1 (IMPG1), reported in this study and involved in visual perception, allowed the grouping of biological replicates and the distinction between the three research groups: good responsive to anti-VEGF, poor/non-responsive to anti-VEGF, and control. The literature lacks studies that establish a consistent correlation of these proteins with the pathophysiogenesis of AMD. Retinol-binding protein 4 (RBP-4) not only has GO term annotations for its involvement in visual perception and the metabolism of retinol, but it has been described as a potential target in treating atrophic AMD and Stargardt's disease (45).
Lipid Metabolism
There are in the literature reports which demonstrate that lipid accumulation in the formation of drusen can be considered as an early event in the pathophysiogenesis of AMD (46). Thus, in senile patients, oxidized lipoproteins and lipids can be considered as triggers of AMD (8, 33, 34).
This study reported numerous proteins frequently associated with lipoprotein particles, including complement factor H (CFHR1), amyloid-similar protein 1 (APLP1), and VTNC, which were highlighted by Thompson et al. (29) as having roles in the pathophysiogenesis of AMD. Moreover, it was possible to demonstrate that the A-I and A-IV apolipoprotein isoforms were upregulated in patients with poor/non-responsive AMD, thus corroborating the results of Levy et al. (47) who proved that apolipoprotein is a factor that promotes the survival of subretinal mononuclear phagocytes and therefore stimulates chronic inflammation in AMD. Phosphoinositide phospholipase C (Q86YU9) and retinol-binding protein 3 (RBP-3), which are related to lipid metabolism, were reported in the analyzed samples, and they were upregulated in the poor/non-responsive group and downregulated in the control group. Morohoshi et al. (48) identified antiretinal antibodies against RBP-3 in patients with neovascular AMD. Recent studies in the literature demonstrate the potential strategic importance of other antibodies, such as anti-HtrA1, in the therapy of AMD geographic atrophy (49).
Oxidative Stress
The association between oxidative stress and age-related conditions has been extensively documented, as in Alzheimer's disease, Parkinson's disease, atherosclerosis, certain types of cancer, and AMD (50–52). For comparison, the retina has the highest oxygen consumption per gram of tissue in the human body, and along with the RPE, it is extremely susceptible to damage because of oxidative stress (53). When the antioxidant capacity of the eye is exceeded, a large number of oxidized proteins, lipids, and inflammation-related factors are formed, which cause drusen to appear (54). The heterophagy of photoreceptors by RPE cells is a constant source of polyunsaturated fatty acids, thus generating an environment rich in reactive oxygen species (ROS) (55) that can induce the oxidative modification of phospholipids. ROS exposure induces immune recognition with inflammatory damage, primarily through complement activation (53, 56).
This study identified several proteins related to redox imbalance as amyloid protein (APLP1), catalase (CAT), enolase (HEL-S-17), glutathione peroxidase (GPx), lipocalins (CRAa LCNI), and superoxide dismutase (SOD) regulated within the biological scenarios, which allowed distinct protein clusters in all three phenotypes. A correlation was observed between these proteins' GO annotations and functional changes caused by oxidative stress and present in pathophysiology of AMD.
The retina contains a considerable number of antioxidant agents in photoreceptors and the RPE, primarily in the central portion of the retina, including SOD, GPx, and CAT (57). Increased concentrations of SOD and GPx were reported in the anti-VEGF poor/non-responsive group and CAT was upregulated in the control group. That is, catalase, as one of the most important antioxidant enzymes, was reduced in patients with AMD in this research, a condition compatible with the literature whose CAT deficiency or malfunction is related to many degenerative diseases associated with age, such as diabetes mellitus, vitiligo, cardiovascular diseases, Wilson's disease, hypertension, anemia, some dermatological disorders, Alzheimer's disease, bipolar disorder, and schizophrenia (58). SOD and GPx are produced in an oxidative injured environment, whereas CAT is a physiological constituent of the cell.
Amyloid protein, known to be present in Alzheimer's disease (59), is another important component of drusen (60, 61), and in this study, it was reported to be downregulated in the control group (patients without AMD). Lipocalin 1 (LCN1), which has already been characterized as a protector against the oxidative stress, potentially because of lipid peroxidation products, was reported in this study to be upregulated in patients with good responsive AMD and downregulated in patients with anti-VEGF poor/non-responsive.
Enolase (HEL-S-17) is a key enzyme of the glycolytic pathway that is reported in AMD geographic and neovascular atrophies (48, 62, 63), and has GO annotations correlating it with oxidative stress. Enolase was reported to be upregulated in the control group and downregulated in the anti-VEGF poor/non-responsive group. Several researchers have described autoimmune stimulation of enolase, and its serum measurement is a potential staging or, even, a prognostic tool for AMD. Adamus et al. (62) have analyzed antiretinal autoantibodies against enolase at different stages of AMD.
Complement System
Over the years, photoreceptors and RPE cells are exposed to numerous innate immune activators. Therefore, a strict regulation of immunity is essential to prevent harmful inflammatory events. However, the deregulation of the complement system can potentially lead to chronic inflammation of the eye. One of the first indications that the complement system is involved in the progression of AMD was the identification of complement by-products (factor H) in drusen (64). In this study, an increase in C2, C3, C4, and C7 was reported in patients with poor/non-responsive AMD, in accordance with the literature (25, 65, 66). Factor C8 was reported to be upregulated in the group of good responsive AMD. As reported by Lu et al. (66), complement factor B (CFB) was reported in a higher concentration in control patients, functioning as a preventive factor for AMD.
Clusterin (CLU), according to the observed GO annotations, is involved in inflammatory processes and in the complement cascade. However, it can play a protective role in the response to the redox (oxidative stress) environment of human RPE cells, contributing to cell survival using PI3K/Akt, an intracellular signaling pathway with an important role in the regulation of the cell cycle. Therefore, CLU can be considered as a preventive factor for AMD. It was annotated in a relatively greater concentration in patients with poor/non-responsive AMD in this study and which could demonstrate that this pathway remains active in an attempt to restore local homeostasis. Similar to APLP1, CLU has been described in the pathophysiogenesis of Alzheimer's disease (67). The heavy chain of immunoglobulin Mu (IGHM), in turn, despite GO annotations correlating it with the immune response and complement activation, was a marker found in this study, although no association with AMD has been described in the literature until nowadays.
However, VTNC, which has been associated with lipoprotein particles, has GO annotations within extracellular matrix (ECM) constituents, upregulation of VEGF (angiogenesis), cell adhesion, and complement regulation. It is also considered one of the main proteins involved in the formation of drusen (68).
Numerous studies confirm the close relationship of the complement system with the progression of retinal degeneration (69). However, the adaptive immune response that leads to the activation and regulation of inflammatory cytokines of B and T cells has not yet been fully elucidated (70, 71).
Inflammation
The heavy chain of IGHM, CLU, and enolase (HEL-S-17) are some of the proteins marked in this study that are potentially involved in inflammatory pathways and are linked to other pathways of neovascular AMD. The CD14 monocyte differentiation antigen (F1C4A7) and plasma kallikrein (KLKB1) were reported to have different regulation levels throughout the groups analyzed in this study. Most significantly, they were upregulated in the poor/non-responsive AMD group. However, the literature lacks studies that show a direct correlation of these proteins with AMD.
Guo et al. (72) and Ali et al. (73) inferred a correlation between CD14 antigen with apolipoprotein E (APOE), the main lipoprotein found in the retina (72–74), which plays a crucial role in local lipid transport (47). Thus, the CD14 antigen can also potentially participate in lipid metabolism in the pathogenesis of AMD.
Angiogenesis
The impairment of RPE function is an early and crucial event that influences and drives choroidal neovascularization (75, 76). The pigment epithelium-derived factor (PEDF) participates in the suppression of angiogenesis and negatively regulates the inflammatory response. It was found to be negatively regulated in the control group (cataract) in this study, precisely in an environment with reduced need for inflammatory and angiogenesis inhibiting factors.
Insulin-like growth factor-binding protein 7 (IGFBP-7) and PEDF are inhibitors of angiogenesis and were upregulated in patients with neovascular AMD. Ectonucleotide pyrophosphatase (ENPP2) was upregulated in the good responsive group, but despite having a GO annotation correlating it with the neoangiogenesis, scarce literature correlates this protein with AMD.
The growth of choroidal neovascularization through the Bruch's membrane is stimulated by producing metalloproteinases (MMPs), which are enzymes that degrade ECM proteins (75). Tissue inhibitors of metalloproteinases (TIMPs) also play an important role in the degradation of ECM components or in the accumulation of deposits in the Bruch's membrane (BM) (77). By inhibiting the migration of microvascular endothelial cells, or function as competitive inhibitors of the binding site between VEGF and VEGF receptor 2, TIMP-1 act as angiogenesis inhibitors (76–78). Recent studies demonstrate significant differences in plasma concentrations of MMP-9, TIMP-1, and TIMP-3 proteins in patients with AMD: higher levels of TIMP-1 and MMP-9 in patients with geographic atrophy (GA) and lower levels of TIMP-3 and lower TIMP-3/MMP-2 ratios in patients with CNV (79). In this particular experimental study, TIMP-1 was reported to be upregulated in the aqueous humor of patients in the poor/non-responsive group, a finding that is still poorly defined in the literature.
Metallothionein 1G (MT1G) is a cysteine-rich, low molecular-weight metalloprotein. In mammals, it plays multiple roles, such as the detoxification of heavy metals, the regulation of essential metal homeostasis, and the protection against oxidative stress. This protein was upregulated in this study's control group, in agreement with the literature, which correlates it with retinal neuroprotection (80).
The phosphorylation of protein kinases is an important mechanism for endothelial cell proliferation and differentiation because the activity of tyrosine kinase receptors is essential for angiogenesis (81). The tyrosine kinase receptor (KDR) and VEGF receptor are the key factors in the pathogenesis of choroidal neovascularization in AMD (82–84). In this study, a type-III tyrosine KDR and vascular endothelial growth factor receptor 1 (VEGFR1) were reported to be upregulated in the poor/non-responsive group and downregulated in the good responsive group.
The ubiquitin–proteosome system is one of the primary components which is responsible for the degradation of proteins that are damaged or unnecessary to cellular metabolism and are produced by heterophagy of the external segments of photoreceptors (85). Ubiquitin carboxy-terminal hydrolase (UCH) was reported to be upregulated in the control group and downregulated in the good responsive group. This finding contradicts those described by Gleen et al. (86).
Sequential Analysis in Patients With AMD: Resistance to the Gold-Standard Treatment
Excluding the control group, proteins with statistical significance and discriminatory capability as possible biomarkers were identified among the groups of patients with AMD. Two proteins, –LCN1 and the heavy chain of IGHM, were upregulated in the good responsive group and downregulated in the anti-VEGF poor/non-responsive group. On the other hand, for three other proteins, plasma kallikrein (KLKB1), kinase insert domain receptor (KDR), and vascular endothelial growth factor receptor 1 (VEGFR-1), the opposite has been observed.
Indeed, LCN1 and the heavy chain of IGHM were upregulated in the treatment-naive group and downregulated in the poor/non-responsive group. Specifically due to LCN1, its correlation with AMD is consistently established in the literature. According to Ghosh et al. (87), LCN1 acts as a marker of early AMD, particularly when lysosome-mediated clearance is compromised in the RPE, which contributes to the induction of a chronic inflammatory response. This hypothesis had been demonstrated in an animal AMD model (88). There are few studies on the recent literature regarding the relationship between the heavy chain of IGHM and ophthalmological diseases. However, researchers have already demonstrated the association between this protein and rare presentations of hematological diseases (benign monoclonal gammopathy and agammaglobulinemia 1) (89, 90).
For the three proteins that were upregulated in the poor/non-responsive group and downregulated in the good responsive group, the literature shows that one of them (plasma kallikrein, KLKB1) is directly linked to inflammatory processes and two of them (kinase insert domain receptor, KDR and vascular endothelial growth factor receptor 1, VEGFR-1) are linked to vascular and endothelial growth events. By characterizing a gradual loss of drug efficacy (anti-VEGF) over time, during the gold-standard therapy for neovascular AMD, some researchers introduced a new concept of treatment-resistance in the context of AMD. Tolerance to medication is characterized by a slow loss of efficacy over time (91). The effect of the drug can be improved if the dosage is increased or if the drug is administered at shorter intervals. On the other hand, the efficacy is not restored if anti-VEGF treatment is stopped temporarily (91). In this research, this difference in the number of receptors in poor/non-responsive patients not only might explain the difficulty in controlling the subretinal neovascular membrane regime, but also may corroborate the explanation regarding the pathological tolerance that some patients develop to the gold-standard treatment of AMD. Since VEGFR-1 is upregulated in patients of the poor/non-responsive group, this feature may explain, at least in part, the persistence of disease activity, even with the usage of anti-VEGF in patients with AMD.
Among the 39 proteins selected in this study, the most promising were those with upregulated and higher relative intensity (VIP score >1), feasible access to serum matrix, and relevant metabolic importance in choroidal neovascularization impaired pathways.
By their importance in the visual function, the crystalline α-, β-, and γ- (such as the α-crystalline, CRYAB; β-crystalline 1, CRBB1; and γ-crystalline C, CRYGC) stand out. In the lipid metabolism pathway, important in early diagnosis, apolipoprotein A-I isoform CRA_A (APOA1), which is correlated with the formation of drusen, appears with high discriminant, along with phosphoinositide phospholipase C (PLC), which features the greatest fold change, and retinol-binding protein 3 (RBP3), for its potential in serum documentation by self-antibodies.
In the oxidative stress pathway, CAT is the protein with the highest relative intensity and the highest discriminatory capability within the phenotypic conditions (VIP score). In the complement activation pathway, this study corroborates with the descriptions in the literature, with the groups of patients with AMD showing, in general, a relative increase compared with the control group. All proteins described in this study and involved in this specific pathway may be potential biomarker candidates.
In the inflammatory pathway, enolase 1 (HEL-S-17) is noteworthy, because it is presented within the 30 proteins with the highest VIP score. Finally, in the angiogenic pathway, VEGFR 1 is highlighted as an important effector, and plays a core role in the choroidal neovascularization pathophysiology. Although, there are discrepancies with the literature data, tissue inhibitor of metalloproteins 1 (TIMP 1) is displayed at the VIP score chart. Nonetheless, further studies are the requirement to a deeper understanding of this effector.
Although under treatment, an important percentage of the population with nAMD remains with the disease inactivity. This aspect requires a deeper understanding of alternative pathways for choroidal neoangiogenesis. Nowadays, high-performance analytical experiments pursue diagnostic and therapeutic means through additional pathways in the pathophysiogenesis of nAMD. In this study, the proteins selected may contribute as potential biomarkers for early diagnosis and even new adjuvant therapeutic targets.
A more expanded research analysis should be performed as well, and validation studies in large cohorts are still required to assess the diagnostic and prognostic capability of the protein classes selected herein.
Data Availability Statement
The datasets for this study can be found in the Repository MassIVE (https://massive.ucsd.edu/ProteoSAFe/dataset.jsp?task=852e34f8818041e3a434132163460c89).
Ethics Statement
The studies involving human participants were reviewed and approved by Ethics Committee: Plataforma Brasil/Hospital Oftalmológico De Brasília (under the number CAAE 64921317.1.0000.5667). The patients provided their written informed consent to participate in this study.
Author Contributions
BC has contributed to conceptualizing, drafting, writing, experiment analysis, funding, and final revision of the manuscript. FC has organized the data and tables. RO has involved in experiment and data analysis, organizing figures and reference revision. ON and CR have contributed on funding and final revision. WF has performed experiment and data analysis. MS has contributed on funding and infrastructure. MÁ has contributed to conceptualizing, infrastructure, mentoring, and final revision. AM has contributed to conceptualizing, drafting, experiment and data analysis, writing, mentoring, final revision, and supervision of the manuscript. All authors contributed to the article and approved the submitted version.
Conflict of Interest
The authors declare that the research was conducted in the absence of any commercial or financial relationships that could be construed as a potential conflict of interest.
Publisher's Note
All claims expressed in this article are solely those of the authors and do not necessarily represent those of their affiliated organizations, or those of the publisher, the editors and the reviewers. Any product that may be evaluated in this article, or claim that may be made by its manufacturer, is not guaranteed or endorsed by the publisher.
Acknowledgments
The authors acknowledge the Graduate Program of Medical Sciences of the Faculty of Medicine of the University of Brasília, the Laboratory of Protein Chemistry and Biochemistry (UnB), and the Brazilian Center of Vision-CBV/DF, Brazil, especially to Fabrício Tadeu Borges, MD.
Supplementary Material
The Supplementary Material for this article can be found online at: https://www.frontiersin.org/articles/10.3389/fmed.2021.692272/full#supplementary-material
References
1. Wong WL, Su X, Li X, Cheung CMG, Klein R, Cheng CY, et al. Global prevalence of age-related macular degeneration and disease burden projection for 2020 and 2040: a systematic review and meta-analysis. Lancet Glob Heal. (2014) 2:e106–16. doi: 10.1016/S2214-109X(13)70145-1
2. Ávila MP de, Garcia JM, Isaac DLC. Degeneração macular relacionada à idade: presente e futuro e-OftalmoCBO. Rev Digit Oftalmol. (2015) 1:1–9. doi: 10.17545/e-oftalmo.cbo/2015.18
3. Brandl C, Stark KJ, Wintergerst M, Heinemann M, Heid IM, Finger RP. Epidemiologie der altersbedingten Makuladegeneration [Epidemiology of age-related macular degeneration]. Der Ophthalmol. (2016) 113:735–45. doi: 10.1007/s00347-016-0341-6
4. Rudnicka AR, Jarrar Z, Wormald R, Cook DG, Fletcher A, Owen CG. Age and gender variations in age-related macular degeneration prevalence in populations of European ancestry: a meta-analysis. Ophthalmology. (2012) 119:571–80. doi: 10.1016/j.ophtha.2011.09.027
5. Schramm EC, Clark SJ, Triebwasser MP, Raychaudhuri S, Seddon JM, Atkinson JP. Genetic variants in the complement system predisposing to age-related macular degeneration: a review. Mol Immunol. (2014) 61:118–25. doi: 10.1016/j.molimm.2014.06.032
6. Sun X, Yang S, Zhao J. Resistance to anti-VEGF therapy in neovascular age-related macular degeneration: a comprehensive review. Drug Des Devel Ther. (2016) 1857–67. doi: 10.2147/DDDT.S97653
7. Lynch AM, Wagner BD, Palestine AG, Janjic N, Patnaik JL, Mathias MT, et al. Plasma biomarkers of reticular pseudodrusen and the risk of progression to advanced age-related macular degeneration. Transl Vis Sci Technol. (2020) 9:1–13. doi: 10.1167/tvst.9.10.12
8. Fleckenstein M, Keenan TDL, Guymer RH, Chakravarthy U, Schmitz-Valckenberg S, Klaver CC, et al. Age-related macular degeneration. Nat Rev Dis Prim. (2021) 7:31. doi: 10.1038/s41572-021-00265-2
9. Rudnicka AR, Kapetanakis V V., Jarrar Z, Wathern AK, Wormald R, Fletcher AE, et al. Incidence of late-stage age-related macular degeneration in American whites: systematic review and meta-analysis. Am J Ophthalmol. (2015) 83–93. doi: 10.1016/j.ajo.2015.04.003
10. Zhou H, Zhang H, Yu A, Xie J. Association between sunlight exposure and risk of age-related macular degeneration: a meta-analysis. BMC Ophthalmol. (2018) 18:1–8. doi: 10.1186/s12886-018-1004-y
11. Lores-Padín A, Fernández B, Álvarez L, González-Iglesias H, Lengyel I, Pereiro R. Multiplex bioimaging of proteins-related to neurodegenerative diseases in eye sections by laser ablation-Inductively coupled plasma–Mass spectrometry using metal nanoclusters as labels. Talanta. (2021) 221:121489. doi: 10.1016/j.talanta.2020.121489
12. Jensen EG, Jakobsen TS, Thiel S, Askou AL, Corydon TJ. Associations between the complement system and choroidal neovascularization in wet age-related macular degeneration. Int J Mol Sci. (2020) 21:1–28. doi: 10.3390/ijms21249752
13. Pugazhendhi A, Hubbell M, Jairam P, Ambati B. Neovascular macular degeneration: a review of etiology, risk factors, and recent advances in research and therapy. Int J Mol Sci. (2021) 22:1–25. doi: 10.3390/ijms22031170
14. Pool FM, Kiel C, Serrano L, Luthert PJ. Repository of proposed pathways and protein–protein interaction networks in age-related macular degeneration. NPJ Aging Mech Dis. (2020) 6:1–11. doi: 10.1038/s41514-019-0039-5
15. Chakravarthy U, Harding SP, Rogers CA, Downes SM, Lotery AJ, Wordsworth S, et al. Ranibizumab versus bevacizumab to treat neovascular age-related macular degeneration: one-year findings from the IVAN randomized trial. Ophthalmology. (2012) 119:1399–411. doi: 10.1016/j.ophtha.2012.04.015
16. Gao Y, Yu T, Zhang Y, Dang G. Anti-VEGF monotherapy versus photodynamic therapy and anti-VEGF combination treatment for neovascular age-related macular degeneration: a meta-analysis. Investig Opthalmology Vis Sci. (2018) 59:4307. doi: 10.1167/iovs.17-23747
17. Heier JS, Brown DM, Chong V, Korobelnik JF, Kaiser PK, Nguyen QD, et al. Intravitreal aflibercept (VEGF trap-eye) in wet age-related macular degeneration. Ophthalmology. (2012) 119:2537–48. doi: 10.1016/j.ophtha.2012.09.006
18. Ehlken C, Jungmann S, Böhringer D, Agostini HT, Junker B, Pielen A. Switch of anti-VEGF agents is an option for nonresponders in the treatment of AMD. Eye. (2014) 28:538–45. doi: 10.1038/eye.2014.64
19. de Carvalho JRL, Park KS, Saraiva FP, Tsang SH, Mahajan VB, Cabral T. Proteomic biomarkers in vitreoretinal disease. In: Precision Medicine for Investigators, Practitioners and Providers. Elsevier. p. 247–54.
20. Campochiaro PA. Molecular pathogenesis of retinal and choroidal vascular diseases. Prog Retin Eye Res. (2015) 49:67–81. doi: 10.1016/j.preteyeres.2015.06.002
21. Amoaku WM, Chakravarthy U, Gale R, Gavin M, Ghanchi F, Gibson J, et al. Defining response to anti-VEGF therapies in neovascular AMD. Eye. (2015) 29:721–31. doi: 10.1038/eye.2015.48
22. Rappsilber J, Mann M, Ishihama Y. Protocol for micro-purification, enrichment, pre-fractionation and storage of peptides for proteomics using StageTips. Nat Protoc. (2007) 2:1896–906. doi: 10.1038/nprot.2007.261
23. Chong J, Soufan O, Li C, Caraus I, Li S, Bourque G, et al. MetaboAnalyst 40: towards more transparent and integrative metabolomics analysis. Nucleic Acids Res. (2018) 46:W486–94. doi: 10.1093/nar/gky310
24. Szklarczyk D, Morris JH, Cook H, Kuhn M, Wyder S, Simonovic M, et al. The STRING database in 2017: quality-controlled protein-protein association networks, made broadly accessible. Nucleic Acids Res. (2017) 45:D362–8. doi: 10.1093/nar/gkw937
25. Volz C, Pauly D. Antibody therapies and their challenges in the treatment of age-related macular degeneration. Eur J Pharm Biopharm. (2015) 95:158–72. doi: 10.1016/j.ejpb.2015.02.020
26. Rezar-Dreindl S, Sacu S, Eibenberger K, Pollreisz A, Bühl W, Georgopoulos M, et al. The intraocular cytokine profile and therapeutic response in persistent neovascular age-related macular degeneration. Investig Ophthalmol Vis Sci. (2016) 57:4144–50. doi: 10.1167/iovs.16-19772
27. Ambati J, Atkinson JP, Gelfand BD. Immunology of age-related macular degeneration. Nat Rev Immunol. (2013) 13:438–51. doi: 10.1038/nri3459
28. Mantel I, Borgo A, Guidotti J, Forestier E, Kirsch O, Derradji Y, et al. Molecular biomarkers of neovascular age-related macular degeneration with incomplete response to anti-vascular endothelial growth factor treatment. Front Pharmacol. (2020) 11:1–14. doi: 10.3389/fphar.2020.594087
29. Thompson RB, Reffatto V, Bundy JG, Kortvely E, Flinn JM, Lanzirotti A, et al. Identification of hydroxyapatite spherules provides new insight into subretinal pigment epithelial deposit formation in the aging eye. Proc Natl Acad Sci. (2015) 112:1565–70. doi: 10.1073/pnas.1413347112
30. Johnson LV, Forest DL, Banna CD, Radeke CM, Maloney MA, Hu J, et al. Cell culture model that mimics drusen formation and triggers complement activation associated with age-related macular degeneration. Proc Natl Acad Sci U S A. (2011) 108:18277–82. doi: 10.1073/pnas.1109703108
31. Rudolf M, Curcio CA. Esterified cholesterol is highly localized to Bruch's membrane, as revealed by lipid histochemistry in wholemounts of human choroid. J Histochem Cytochem. (2009) 57:731–9. doi: 10.1369/jhc.2009.953448
32. Curcio CA, Johnson M, Huang J-D, Rudolf M. Aging, age-related macular degeneration, and the response-to-retention of apolipoprotein B-containing lipoproteins. Prog Retin Eye Res. (2009) 28:393–422. doi: 10.1016/j.preteyeres.2009.08.001
33. Jun S, Datta S, Wang L, Pegany R, Cano M, Handa JT. The impact of lipids, lipid oxidation, and inflammation on AMD, and the potential role of miRNAs on lipid metabolism in the RPE. Exp Eye Res. (2019) 181:346–55. doi: 10.1016/j.exer.2018.09.023
34. Handa JT, Cano M, Wang L, Datta S, Liu T. Lipids, oxidized lipids, oxidation-specific epitopes, and age-related macular degeneration. Biochim Biophys Acta-Mol Cell Biol Lipids. (2017) 1862:430–40. doi: 10.1016/j.bbalip.2016.07.013
35. Hewitt AT, Nakazawa K, Newsome DA. Analysis of newly synthesized Bruch's membrane proteoglycans. Invest Ophthalmol Vis Sci. (1989) 30:478–86.
36. Zarbin MA. Current concepts in the pathogenesis of age-related macular degeneration. Arch Ophthalmol. (2004) 122:598. doi: 10.1001/archopht.122.4.598
37. Newsome DA, Hewitt AT, Huh W, Robey PG, Hassell JR. Detection of specific extracellular matrix molecules in drusen, Bruch's membrane, and ciliary body. Am J Ophthalmol. (1987) 104:373–81. doi: 10.1016/0002-9394(87)90227-3
38. de Carlo TE, Romano A, Waheed NK, Duker JS. A review of optical coherence tomography angiography (OCTA). Int J Retin Vitr. (2015) 1:5. doi: 10.1186/s40942-015-0005-8
39. Sinha D, Klise A, Sergeev Y, Hose S, Bhutto IA, Hackler L, et al. βA3/A1-crystallin in astroglial cells regulates retinal vascular remodeling during development. Mol Cell Neurosci. (2008) 37:85–95. doi: 10.1016/j.mcn.2007.08.016
40. Ma B, Sen T, Asnaghi L, Valapala M, Yang F, Hose S, et al. βA3/A1-Crystallin controls anoikis-mediated cell death in astrocytes by modulating PI3K/AKT/mTOR and ERK survival pathways through the PKD/Bit1-signaling axis. Cell Death Dis. (2011) 2:1–12. doi: 10.1038/cddis.2011.100
41. Valapala M, Wilson C, Hose S, Bhutto IA, Grebe R, Dong A, et al. Lysosomal-mediated waste clearance in retinal pigment epithelial cells is regulated by CRYBA1/βA3/A1-crystallin via V-ATPase-MTORC1 signaling. Autophagy. (2014) 10:480–96. doi: 10.4161/auto.27292
42. Lynch AM, Wagner BD, Weiss SJ, Wall KM, Palestine AG, Mathias MT, et al. Proteomic profiles in advanced age-related macular degeneration using an aptamer-based proteomic technology. Transl Vis Sci Technol. (2019) 8:14. doi: 10.1167/tvst.8.1.14
43. Morohoshi K, Patel N, Ohbayashi M, Chong V, Grossniklaus HE, Bird AC, et al. Serum autoantibody biomarkers for age-related macular degeneration and possible regulators of neovascularization. Exp Mol Pathol. (2012) 92:64–73. doi: 10.1016/j.yexmp.2011.09.017
44. Kannan R, Sreekumar PG, Hinton DR. Alpha crystallins in the retinal pigment epithelium and implications for the pathogenesis and treatment of age-related macular degeneration. Biochim Biophys Acta-Gen Subj. (2016) 1860:258–68. doi: 10.1016/j.bbagen.2015.05.016
45. Cioffi CL, Racz B, Freeman EE, Conlon MP, Chen P, Stafford DG, et al. Bicyclic [330]-Octahydrocyclopenta[c]pyrrolo antagonists of retinol binding protein 4: potential treatment of atrophic age-related macular degeneration and stargardt disease. J Med Chem. (2015) 58:5863–88. doi: 10.1021/acs.jmedchem.5b00423
46. Curcio CA, Presley JB, Malek G, Medeiros NE, Avery DV, Kruth HS. Esterified and unesterified cholesterol in drusen and basal deposits of eyes with age-related maculopathy. Exp Eye Res. (2005) 81:731–41. doi: 10.1016/j.exer.2005.04.012
47. Levy O, Calippe B, Lavalette S, Hu SJ, Raoul W, Dominguez E, et al. Apolipoprotein E promotes subretinal mononuclear phagocyte survival and chronic inflammation in age-related macular degeneration. EMBO Mol Med. (2015) 7:211–26. doi: 10.15252/emmm.201404524
48. Morohoshi K, Ohbayashi M, Patel N, Chong V, Bird AC, Ono SJ. Identification of anti-retinal antibodies in patients with age-related macular degeneration. Exp Mol Pathol. (2012) 93:193–9. doi: 10.1016/j.yexmp.2012.03.007
49. Tom I, Pham VC, Katschke KJ Li W, Liang W, Gutierrez J. Development of a therapeutic anti-HtrA1 antibody and the identification of DKK3 as a pharmacodynamic biomarker in geographic atrophy. Proc Natl Acad Sci. (2020) 117:9952–63. doi: 10.1073/pnas.1917608117
50. Dong A, Xie B, Shen J, Yoshida T, Yokoi K, Hackett SF, et al. Oxidative stress promotes ocular neovascularization. J Cell Physiol. (2009) 219:544–52. doi: 10.1002/jcp.21698
51. Dentchev T, Milam AH, Lee VM-Y, Trojanowski JQ, Dunaief JL. Amyloid-beta is found in drusen from some age-related macular degeneration retinas, but not in drusen from normal retinas. Mol Vis. (2003) 9:184–90. doi: 10.1016/S0002-9394(03)00804-3
52. Hollyfield JG, Bonilha VL, Rayborn ME, Yang X, Shadrach KG, Lu L, et al. Oxidative damage-induced inflammation initiates age-related macular degeneration. Nat Med. (2008) 14:194–8. doi: 10.1038/nm1709
53. Hernández-Zimbrón LF, Zamora-Alvarado R, Ochoa-De la Paz L, Velez-Montoya R, Zenteno E, Gulias-Cañizo R, et al. Age-related macular degeneration: new paradigms for treatment and management of AMD. Oxid Med Cell Longev. (2018) 2018:1–14. doi: 10.1155/2018/8374647
54. Blasiak J. Implications of altered iron homeostasis for age-related macular degeneration. Front Biosci. (2011) 16:1551–9. doi: 10.2741/3804
55. Catala A. Lipid peroxidation of membrane phospholipids in the vertebrate retina. Front Biosci. (2011) S3:131. doi: 10.2741/s131
56. Shaw PX, Zhang L, Zhang M, Du H, Zhao L, Lee C, et al. Complement factor H genotypes impact risk of age-related macular degeneration by interaction with oxidized phospholipids. Proc Natl Acad Sci. (2012) 109:13757–62. doi: 10.1073/pnas.1121309109
57. Mrowicka M, Mrowicki J, Szaflik JP, Szaflik M, Ulinska M, Szaflik J, et al. Analysis of antioxidative factors related to AMD risk development in the polish patients. Acta Ophthalmol. (2017) 95:530–6. doi: 10.1111/aos.13289
58. Nandi A, Yan L-J, Jana CK, Das N. Role of catalase in oxidative stress- and age-associated degenerative diseases. Oxid Med Cell Longev. (2019) 2019:1–19. doi: 10.1155/2019/9613090
59. Kasim JK, Kavianinia I, Harris PWR, Brimble MA. Three decades of amyloid beta synthesis: challenges and advances. Front Chem. (2019) 7:1–25. doi: 10.3389/fchem.2019.00472
60. Lee CS, Larson EB, Gibbons LE, Lee AY, McCurry SM, Bowen JD, et al. Associations between recent and established ophthalmic conditions and risk of Alzheimer's disease. Alzheimer's Dement. (2019) 15:34–41. doi: 10.1016/j.jalz.2018.06.2856
61. Ohno-Matsui K. Parallel findings in age-related macular degeneration and Alzheimer's disease. Prog Retin Eye Res. (2011) 30:217–38. doi: 10.1016/j.preteyeres.2011.02.004
62. Adamus G, Chew EY, Ferris FL, Klein ML. Prevalence of anti-retinal autoantibodies in different stages of age-related macular degeneration. BMC Ophthalmol. (2014) 14:154. doi: 10.1186/1471-2415-14-154
63. Joachim SC, Bruns K, Lackner KJ, Pfeiffer N, Grus FH. Analysis of IgG antibody patterns against retinal antigens and antibodies to α-crystallin, GFAP, and α-enolase in sera of patients with “wet” age-related macular degeneration. Graefe's Arch Clin Exp Ophthalmol. (2007) 245:619–26. doi: 10.1007/s00417-006-0429-9
64. Hageman G. An integrated hypothesis that considers drusen as biomarkers of immune-mediated processes at the RPE-Bruch's membrane interface in aging and age-related macular degeneration. Prog Retin Eye Res. (2001) 20:705–32. doi: 10.1016/S1350-9462(01)00010-6
65. Grassmann F, Cantsilieris S, Schulz-Kuhnt AS, White SJ, Richardson AJ, Hewitt AW, et al. Multiallelic copy number variation in the complement component 4A (C4A) gene is associated with late-stage age-related macular degeneration (AMD). J Neuroinflammation. (2016) 13:1–9. doi: 10.1186/s12974-016-0548-0
66. Lu F, Liu S, Hao Q, Liu L, Zhang J, Chen X, et al. Association between complement factor C2/C3/CFB/CFH polymorphisms and age-related macular degeneration: a meta-analysis. Genet Test Mol Biomarkers. (2018) 22:526–40. doi: 10.1089/gtmb.2018.0110
67. Dhiman K, Blennow K, Zetterberg H, Martins RN, Gupta VB. Cerebrospinal fluid biomarkers for understanding multiple aspects of Alzheimer's disease pathogenesis. Cell Mol Life Sci. (2019) 76:1833–63. doi: 10.1007/s00018-019-03040-5
68. Wang L, Clark ME, Crossman DK, Kojima K, Messinger JD, Mobley JA, et al. Abundant lipid and protein components of drusen. PLoS ONE. (2010) 5:1–12. doi: 10.1371/journal.pone.0010329
69. Ma W, Zhao L, Wong WT. Microglia in the outer retina and their relevance to pathogenesis of age-related macular degeneration. Adv Exp Med Biol. 723:37–42. doi: 10.1007/978-1-4614-0631-0_6
70. Liu B, Wei L, Meyerle C, Tuo J, Sen HN Li Z, Chakrabarty S, et al. Complement component C5a promotes expression of IL-22 and IL-17 from human T cells and its implication in age-related macular degeneration. J Transl Med. (2011) 9:111. doi: 10.1186/1479-5876-9-111
71. Camelo S. Potential sources and roles of adaptive immunity in age-related macular degeneration: shall we rename AMD into autoimmune macular disease? Autoimmune Dis. (2014) 2014:1–11. doi: 10.1155/2014/532487
72. Guo L, LaDu MJ, Van Eldik LJ. A dual role for apolipoprotein e in neuroinflammation: anti- and pro-inflammatory activity. J Mol Neurosci. (2004) 23:205–12. doi: 10.1385/JMN:23:3:205
73. Ali K, Middleton M, Puré E, Rader DJ. Apolipoprotein E suppresses the type I inflammatory response in vivo. Circ Res. (2005) 97:922–7. doi: 10.1161/01.RES.0000187467.67684.43
74. Anderson DH, Ozaki S, Nealon M, Neitz J, Mullins RF, Hageman GS, et al. Local cellular sources of apolipoprotein E in the human retina and retinal pigmented epithelium: implications for the process of drusen formation. Am J Ophthalmol. (2001) 131:767–81. doi: 10.1016/S0002-9394(00)00961-2
75. Farnoodian M, Sorenson C, Sheibani N. Negative regulators of angiogenesis, ocular vascular homeostasis, and pathogenesis and treatment of exudative AMD. J Ophthalmic Vis Res. (2018) 13:470. doi: 10.4103/jovr.jovr_67_18
76. Qi JH, Ebrahem Q, Moore N, Murphy G, Claesson-Welsh L, Bond M, et al. novel function for tissue inhibitor of metalloproteinases-3 (TIMP3): inhibition of angiogenesis by blockage of VEGF binding to VEGF receptor-2. Nat Med. (2003) 9:407–15. doi: 10.1038/nm846
77. García-Onrubia L, Valentín-Bravo FJ, Coco-Martin RM, González-Sarmiento R, Pastor JC, Usategui-Martín R, et al. Matrix metalloproteinases in age-related macular degeneration (AMD). Int J Mol Sci. (2020) 21:5934. doi: 10.3390/ijms21165934
78. Akahane T, Akahane M, Shah A, Connor CM, Thorgeirsson UP. TIMP-1 inhibits microvascular endothelial cell migration by MMP-dependent and MMP-independent mechanisms. Exp Cell Res. (2004) 301:158–67. doi: 10.1016/j.yexcr.2004.08.002
79. Nielsen MK, Subhi Y, Molbech CR, Nilsson LL, Nissen MH, Sørensen TL. Imbalances in tissue inhibitors of metalloproteinases differentiate choroidal neovascularization from geographic atrophy. Acta Ophthalmol. (2019) 97:84–90. doi: 10.1111/aos.13894
80. Ito Y, Tanaka H, Hara H. The potential roles of metallothionein as a therapeutic target for cerebral ischemia and retinal diseases. Curr Pharm Biotechnol. (2013) 14:400–7. doi: 10.2174/1389201011314040003
81. Ng DS, Yip YW, Bakthavatsalam M, Chen LJ, Ng TK, Lai TY, et al. Elevated angiopoietin 2 in aqueous of patients with neovascular age related macular degeneration correlates with disease severity at presentation. Sci Rep. (2017) 7:45081. doi: 10.1038/srep45081
82. Robinson GS, Ju M, Shih S-C, Xu X, McMahon G, Caldwell RB, et al. Nonvascular role for vascular endothelial growth factor (VEGF): VEGFR-1 and VEGFR-2 activity is critical for neural retinal development. FASEB J. (2001) 15:1215–7. doi: 10.1096/fj.00-0598fje
83. Fruttiger M. Development of the mouse retinal vasculature: angiogenesis versus vasculogenesis. Invest Ophthalmol Vis Sci. (2002) 43:522–7.
84. Rahimi N. Vascular endothelial growth factor receptors: molecular mechanisms of activation and therapeutic potentials. Exp Eye Res. (2006) 83:1005–16. doi: 10.1016/j.exer.2006.03.019
85. Blasiak J, Pawlowska E, Szczepanska J, Kaarniranta K. Interplay between autophagy and the ubiquitin-proteasome system and its role in the pathogenesis of age-related macular degeneration. Int J Mol Sci. (2019) 20:210. doi: 10.3390/ijms20010210
86. Glenn J V, Mahaffy H, Dasari S, Oliver M, Chen M, Boulton ME, et al. Proteomic profiling of human retinal pigment epithelium exposed to an advanced glycation-modified substrate. Graefe's Arch Clin Exp Ophthalmol. (2012) 250:349–59. doi: 10.1007/s00417-011-1856-9
87. Ghosh S, Shang P, Yazdankhah M, Bhutto I, Hose S, Montezuma SR, et al. Activating the AKT2-nuclear factor-κB-lipocalin-2 axis elicits an inflammatory response in age-related macular degeneration. J Pathol. (2017) 241:583–8. doi: 10.1002/path.4870
88. Valapala M, Edwards M, Hose S, Grebe R, Bhutto IA, Cano M, et al. Increased lipocalin-2 in the retinal pigment epithelium of cryba1 cKO mice is associated with a chronic inflammatory response. Aging Cell. (2014) 13:1091–4. doi: 10.1111/acel.12274
89. Wahner-Roedler DL, Kyle RA. μ-heavy chain disease: presentation as a benign monoclonal gammopathy. Am J Hematol. (1992) 40:56–60. doi: 10.1002/ajh.2830400112
90. Yel L, Minegishi Y, Coustan-Smith E, Buckley RH, Trübel H, Pachman LM, et al. Mutations in the mu heavy-chain gene in patients with agammaglobulinemia. N Engl J Med. (1996) 335:1486–93. doi: 10.1056/NEJM199611143352003
Keywords: AMD (age-related macular degeneration), resistance, proteomics, mass spectrometry (MS), biomarkers, choroidal neo vascularization
Citation: Coronado BNL, da Cunha FBS, de Oliveira RM, Nóbrega OdT, Ricart CAO, Fontes W, de Sousa MV, de Ávila MP and Martins AMA (2022) Novel Possible Protein Targets in Neovascular Age-Related Macular Degeneration: A Pilot Study Experiment. Front. Med. 8:692272. doi: 10.3389/fmed.2021.692272
Received: 08 April 2021; Accepted: 14 December 2021;
Published: 27 January 2022.
Edited by:
Jodhbir Mehta, Singapore National Eye Center, SingaporeReviewed by:
Jaya P. Gnana-Prakasam, Saint Louis University, United StatesFolami Powell, Augusta University, United States
Copyright © 2022 Coronado, da Cunha, de Oliveira, Nóbrega, Ricart, Fontes, de Sousa, de Ávila and Martins. This is an open-access article distributed under the terms of the Creative Commons Attribution License (CC BY). The use, distribution or reproduction in other forums is permitted, provided the original author(s) and the copyright owner(s) are credited and that the original publication in this journal is cited, in accordance with accepted academic practice. No use, distribution or reproduction is permitted which does not comply with these terms.
*Correspondence: Bruno Nobre Lins Coronado, YnJ1bm9ub2JyZWxpbnNAZ21haWwuY29t; Aline Maria Araújo Martins, YWxpbjMubTRydGluc0BnbWFpbC5jb20=