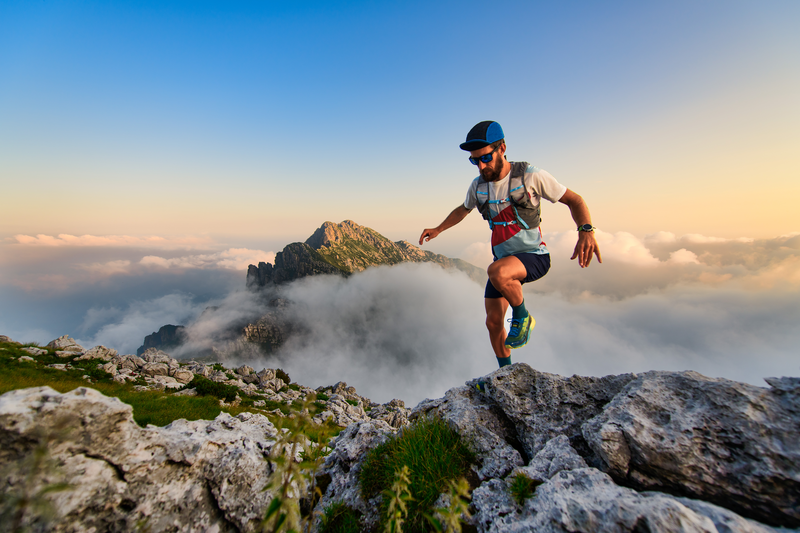
94% of researchers rate our articles as excellent or good
Learn more about the work of our research integrity team to safeguard the quality of each article we publish.
Find out more
ORIGINAL RESEARCH article
Front. Med. , 17 June 2021
Sec. Ophthalmology
Volume 8 - 2021 | https://doi.org/10.3389/fmed.2021.685032
This article is part of the Research Topic Next Therapeutic Targets in Ocular Diseases View all 16 articles
Age-related macular degeneration (AMD) is a leading cause of blindness in the elderly, and oxidative damage to retinal pigment epithelial (RPE) cells plays a major role in the pathogenesis of AMD. Exposure to high levels of atmospheric particulate matter (PM) with an aerodynamic diameter of <2.5 μm (PM2.5) causes respiratory injury, primarily due to oxidative stress. Recently, a large community-based cohort study in the UK reported a positive correlation between PM2.5 exposure and AMD. Sulforaphane (SFN), a natural isothiocyanate found in cruciferous vegetables, has known antioxidant effects. However, the protective effects of SNF in the eye, especially in the context of AMD, have not been evaluated. In the present study, we evaluated the effect of SFN against PM2.5-induced toxicity in human RPE cells (ARPE-19) and elucidated the molecular mechanism of action. Exposure to PM2.5 decreased cell viability in ARPE-19 cells in a time- and dose-dependent manner, potentially due to elevated intracellular reactive oxygen species (ROS). SFN treatment increased ARPE-19 cell viability and decreased PM2.5-induced oxidative stress in a dose-dependent manner. PM2.5-induced downregulation of serum- and glucocorticoid-inducible kinase 1 (SGK1), a cell survival factor, was recovered by SFN. PM2.5 treatment decreased the enzymatic activities of the antioxidant enzymes including superoxide dismutase and catalase, which were restored by SFN treatment. Taken together, these findings suggest that SFN effectively alleviates PM2.5-induced oxidative damage in human ARPE-19 cells via its antioxidant effects, and that SFN can potentially be used as a therapeutic agent for AMD, particularly in cases related to PM2.5 exposure.
Age-related macular degeneration (AMD) is the most devastating chorioretinal disease, and is a leading cause of blindness in the elderly population (1). The retinal pigment epithelium (RPE) is a monolayer of cells located between the retinal photoreceptors and choroid vascular bed. RPE cells support photoreceptors, which are both postmitotic and highly sensitive to environmental insults, and therefore subject to irreversible degeneration. RPE cells are continuously exposed to reactive oxygen species (ROS) due to light exposure, high retinal oxygen consumption, and abundant polyunsaturated fatty acids and photosensitizers in photoreceptors and the RPE (2). Chronic excessive ROS production and accumulation cause oxidative dysfunction in the RPE, which leads to photoreceptor loss in the advanced form of AMD, geographic atrophy (3).
Increased exposure to particulate matter (PM), especially ultrafine particles with an average aerodynamic diameter of <2.5 μm (PM2.5), has been linked to adverse health effects, such as increased risk of cardiovascular and respiratory death (4–6). PM2.5 accumulation causes oxidative stress in the body (7), which is considered to be an important molecular mechanism of PM2.5-mediated toxicity (8).
Sulforaphane (SFN) (Figure 1A) is an organosulfur compound found in cruciferous vegetables such as broccoli, Brussels sprouts, and cabbage (9). SFN has attracted particular interest as an indirect antioxidant due to its ability to induce expression of multiple endogenous antioxidant enzymes by activating nuclear factor E2-related factor-2 (Nrf2) (9). Although supplementation of antioxidant agents such as lutein and zeaxanthin has protective effects in AMD (10), the effect of SFN in AMD has not previously been evaluated. In the present study, we aimed to investigate whether SFN could alleviate PM2.5-induced oxidative stress in human retinal pigment epithelial cells (ARPE-19), and subsequently to explore the mechanisms underlying the antioxidant effects of SFN in this context.
Figure 1. Chemical structure of Sulforaphane (SFN) and effects of SFN on PM2.5-induced cell toxicity in ARPE-19 cells. (A) Chemical structure of SFN. (B) ARPE-19 cells were treated with 0.5% dimethyl sulfoxide as a control indicated as D, and 25, 50, or 100 μM SFN for 24 h, and cell viability was measured using an MTT Assay. (C) ARPE-19 cells were treated with 25, 50, or 100 μg/mL PM2.5 for 24 or 48 h, and cell viability was measured using an MTT assay. (D) ARPE-19 cells were treated with the specified concentrations of SFN or DEX (1 μM) for 6 h after 24 h PM2.5 challenge (100 μg/mL). After treatment, cell viability was determined using an MTT assay. (E) ARPE-19 cells were treated with SFN (50 μM) or DEX (1 μM) for 6 h after PM2.5 challenge (100 μg/mL) for the indicated time periods. Subsequently, LDH levels were determined using an LDH kit. Values represent the mean ± SD of three independent experiments. *p < 0.01 relative to control (C) or PM-challenged group (D,E), one-way ANOVA. #p < 0.01 relative to control (D,E), one-way ANOVA.
Diesel PM NIST 1650b (11) was purchased from Sigma-Aldrich (St. Louis, MO, USA), mixed with saline, and sonicated for 30 min to avoid agglomeration of suspended PM2.5 particles, as described previously (12). SFN and dexamethasone (DEX), a well-known anti-inflammatory drug (13) used as a positive control, were purchased from Sigma-Aldrich. All other chemicals and reagents were obtained from Sigma-Aldrich unless otherwise stated.
The human RPE cell line ARPE-19 (ATCC, Manassas, VA, USA, CLR-2302) was maintained in DMEM/F12 medium (Thermo Fisher, Waltham, MA, USA) with 10% FBS and 100 U/mL penicillin−100 μg/mL streptomycin (P/S), and passaged at a ratio of 1:2 to 1:4 using trypsin-EDTA (Thermo Fisher). Cells were grown at 37°C and 5% CO2. Cells were grown for 24 h and subsequently treated for 24 h with different concentrations of PM2.5 (25, 50, or 100 μg/mL) in the absence or presence of different concentrations of SFN (2, 5, 10, 20, or 30 μM) or DEX (1 μM).
A 3-(4,5-dimethylthiazol-2-yl)-2,5-diphenyltetrazolium bromide (MTT) assay was performed to measure cell viability as described previously (12, 14–16). The viability of treated cells was expressed as the percentage of absorbance relative to that of untreated cells, which was assumed to be 100% viability.
Apoptosis was examined using an Annexin V-FITC/PI Apoptosis Detection Kit (BD Biosciences, San Jose, CA, USA) according to the manufacturer's protocol. ARPE-19 cells were grown in a 6-well plate (2 × 105 cells/well) and treated with 100 μg/mL PM2.5 for 24 h followed by subsequent treatment with SFN for 6 h. Subsequently, cultured cells in all groups were washed twice with ice-cold PBS, resuspended in 300 μL binding buffer, and stained with 10 μL Annexin V-FITC stock and 10 μL PI in dark conditions for 20 min. Stained cells were immediately analyzed with a FACScan Calibur Flow Cytometer (BD Biosciences), and the number of apoptotic cells was calculated using CellQuest software (Becton–Dickinson, CA, USA). The results were expressed as the percentage of Annexin V-stained cells relative to control, and all experiments were performed in triplicate.
For western blot analysis, cells were first rinsed with ice-cold phosphate-buffered saline and treated with lysis buffer comprising 0.5% sodium dodecyl sulfate, 1% NP-40, 1% sodium deoxycholate, 150 mM NaCl, 50 mM Tris-HCl (pH 7.5), and protease inhibitors, as previously described (17). Protein blots were blocked with 5% bovine serum albumin BSA for 2 h and incubated with the following primary antibodies: anti-Bax (1:2000), anti-Bcl2 (1:2000), anti-SGK1 (1:1000), anti-cytochrome c (1:500), and anti-cleaved caspase-3 (1:500) (Cell Signaling Technology, Inc., Danvers, MA, USA). β-actin was used as a loading control. Subsequently, membranes were washed and incubated with horseradish peroxidase-conjugated secondary antibodies (Cell Signaling Technology, 1:5,000). Densitometry analysis was performed using the ImageJ Gel Analysis tool (NIH, Bethesda, MD, USA).
To assess the cellular toxicity of PM2.5, lactate dehydrogenase (LDH) released from cells after exposure to PM2.5 was measured. After 24 h exposure to PM2.5 (100 μg/mL), cell-free supernatant aliquots were separated and measured using a commercially available kit (Pointe Scientific, Lincoln Park, MI, USA). All samples were assayed for LDH content in duplicate using a plate reader (Tecan Austria GmbH, Grödig, Austria).
ROS production was determined using 2′, 7′-dichlorodihydrofluorescein diacetate (DCFH-DA). Cells were incubated in a 96-well plate at 2 × 105 cells/well and treated for 4, 12, 24, 48 h with different concentrations of PM2.5 (25, 50, or 100 μg/mL). And then, the media were replaced with DCFH-DA (50 μg/mL)-containing media and incubated for 30 min. Intracellular ROS levels were measured by monitoring the fluorescence generated from the oxidation product of DCFH-DA at excitation wavelengths of 485 and 535 nm.
SOD activity was measured using a SOD assay kit (Fluka). CAT activity was measured using a CAT assay kit (Sigma-Aldrich) based on the decomposition rate of the substrate hydrogen peroxide (H2O2), which was measured at 240 nm.
All experiments were performed independently at least three times, and results are expressed as mean ± standard deviation (SD). Statistical significance was analyzed using a one-way analysis of variance (ANOVA) followed by Dunnett's test, with a p-value < 0.05 considered statistically significant. SPSS for Windows version 16.0 (SPSS, Chicago, IL, USA) was used to conduct all statistical analyses.
First, we examined the potential cytotoxic effects of SFN in human ARPE-19 cells using an MTT assay. No change in cell viability occurred in cells treated with 0.5% DMSO as a control and different concentrations of SFN ranging from 25 to 100 μM for 24 h (Figure 1B). ARPE-19 cell viability decreased with PM2.5 exposure in a dose- and time-dependent manner (Figure 1C), and was recovered by post-treatment with SFN for 6 h (Figure 1D and Supplementary Figure 1). DEX, a well-known anti-inflammatory drug (13, 18), was used as a positive control. Furthermore, cellular LDH release significantly increased after 24 h exposure to PM2.5 but decreased after treatment with 30 μM SFN (Figure 1E). These results indicated that the amount of LDH released from cells treated with PM2.5 was related to cell viability, and that SFN alleviated PM2.5-induced cytotoxicity.
To further investigate the effect of SFN against PM2.5 in ARPE-19 cells, ARPE-19 cell apoptosis was assessed using flow cytometry. Exposure to 100 μg/mL PM2.5 for 24 h significantly increased late apoptosis relative to the control group, but post-treatment of ARPE-19 cells with SFN (10 and 30 μM) after PM2.5 exposure significantly decreased the PM2.5 -induced late apoptosis (Figure 2).
Figure 2. Effects of Sulforaphane (SFN) on PM2.5-induced apoptosis in ARPE-19 cells. ARPE-19 cells were treated with PM2.5 (100 μg/mL) for 24 h and subsequently treated with the specified concentrations of SFN (0–30 μM) for 6 h. Apoptosis was measured by Annexin V flow cytometry analysis. Values represent the mean ± SD of three independent experiments. *p < 0.01 relative to PM-challenged group, one-way ANOVA. #p < 0.01 relative to control, one-way ANOVA.
In light of the effects of SFN against PM2.5-induced apoptosis in ARPE-19 cells, we further investigated the effects of SFN on the levels and cleavage of apoptotic proteins, including Bax, Bcl-2, cytochrome c, and caspase-3, by western blotting. Exposure to 100 μg/mL PM2.5 (24 h) decreased Bcl-2 and increased Bax, cytochrome c, and cleaved caspase-3 (Figure 3A), which was consistent with the flow cytometry findings. However, post-treatment of ARPE-19 cells with SFN (10 and 30 μM) for 6 h dose-dependently reversed this interaction, as demonstrated by decreased Bax, cytochrome c, and cleaved caspase-3 levels and increased Bcl-2 levels (Figure 3B). Protein levels of SGK1, known as an anti-apoptotic factor (19), were also downregulated by PM2.5 treatment and recovered by SFN treatment, suggesting that SGK1 could be relevant to cell survival following PM2.5 exposure.
Figure 3. Effects of Sulforaphane (SFN) on PM2.5-induced changes in apoptosis-related protein levels. (A) ARPE-19 cells were treated with the indicated concentrations of SFN or DEX (1 μM) 24 h after PM2.5 challenge (100 μg/mL). Subsequently, western blot analysis was conducted to measure Bax, Bcl-2, SGK1, cytochrome c, and cleaved caspase-3. β-actin was used as a loading control. Representative images from each group are shown (n = 3). (B) The graphs show the densitometric intensities of each gene normalized to β-actin. n = 3 blots. *p < 0.01 relative to the control group, one-way ANOVA.
Subsequently, we determined the effects of SFN on PM2.5 induction of ROS by measuring DCFH-DA fluorescence intensity in ARPE-19 cells after exposure to 25, 50, or 100 μg/mL PM2.5 for 4, 12, 24, or 48 h. PM25 exposure increased intracellular ROS levels in a dose-dependent manner (Figure 4A). DCFH-DA fluorescence intensity peaked after 4 h exposure and then dropped to baseline levels after 24 h. Post-treatment with SFN for 6 h after 24 h PM2.5 exposure suppressed PM2.5-induced ROS in a dose-dependent manner (Figure 4B). DEX decreased ROS levels in PM2.5-treated cells (Figure 4B).
Figure 4. Effects of Sulforaphane (SFN) on PM2.5-induced ROS generation. (A) ARPE-19 cells were treated for the indicated times with the following concentrations of PM2.5: 25 μg/mL (closed circle), 50 μg/mL (closed square), or 100 μg/mL (closed triangle). Intracellular ROS levels were determined using DCFH-DA. DCFH-DA fluorescence values are expressed as the fluorescence ratio (fold) between PM2.5-treated cells and untreated control cells. (B) ARPE-19 cells were treated with the indicated concentrations of SFN or DEX (1 μM) 24 h after being challenged with PM2.5 (100 μg/mL). After treatment, ROS levels were measured. Values represent the mean ± SD of three independent experiments. *p < 0.01 relative to 0 h group (A) or PM-challenged group (B), one-way ANOVA. #p < 0.01 relative to control (B), one-way ANOVA.
The activities of SOD and CAT in ARPE-19 cells were decreased in a dose-dependent manner after 48 h exposure to PM2.5, and were recovered by post-treatment with SFN, also in a dose-dependent manner (Figure 5). These results suggested that SFN decreased PM2.5-induced oxidative stress by increasing intracellular antioxidant enzyme activity. DEX increased SOD and CAT activities under PM2.5 challenge (Figure 5).
Figure 5. Effects of Sulforaphane (SFN) and PM2.5 on antioxidant enzyme activities. ARPE-19 cells were treated with the indicated concentrations of SFN or DEX (1 μM) for 6 after 24 h PM2.5 challenge (100 μg/mL). After treatment, the activities of (A) catalase (CAT) and (B) superoxide dismutase (SOD) were measured. Values represent mean ± SD of three independent experiments. *p < 0.01 relative to PM-challenged group, one-way ANOVA. #p < 0.01 relative to control, one-way ANOVA.
A growing body of evidence supports that ROS-induced oxidative stress damages the RPE, which can eventually lead to geographic atrophy and subsequent development of AMD (20, 21). Oxidative stress results primarily from an imbalance between ROS generation and antioxidant defenses, and especially in the context of the RPE, oxidative stress increases with age, leading to photoreceptor impairment and loss (22). Thus, a balanced redox state is crucial for preventing or delaying progression of AMD and vision loss. Consistent with this hypothesis, clinical and basic research studies have demonstrated that daily dietary supplementation of natural antioxidants, such as b-carotenoid, lutein, zeaxanthin, and anthocyanins, inhibits development and progression of AMD (23, 24).
Epidemiological evidence indicates that the greatest health risks posed by environmental PM are associated with ultrafine PM (25). The PM used in the present study was <2.5 μm in diameter, which is known to exert cellular damage in the alveolar regions of the lung (25). Further, a recent study identified that PM2.5 promotes epithelial-mesenchymal transition of human RPE, which is mediated by upregulation of TGF-β-dependent nuclear transcription factors (26).
Interestingly, the relationship between air pollution and retinal structure was reported in large community-based cohort studies, collectively referred to as the UK Biobank. Higher concentrations of PM2.5 were associated decreased thickness of the ganglion cell-inner plexiform, inner nuclear, and outer plexiform + outer nuclear layers (27). Furthermore, greater exposure to PM2.5 was associated with increased incidence of self-reported AMD and decreased thickness of the RPE layer (28).
Despite evidence supporting the association between PM2.5 exposure and AMD, PM2.5 -mediated oxidative responses and the anti-oxidant effect of SFN, especially in the context of AMD, have not been thoroughly investigated. The purpose of the present study was therefore to examine the potential therapeutic effects of SFN against PM2.5-induced RPE cytotoxicity.
The cell viability assay is important in determining the cellular response to toxins, and provides information on cell death, cell survival, and metabolic activities (29). PM2.5 is believed to cause genotoxicity and cytotoxicity and suppress cell proliferation (30). The present study demonstrated that PM2.5 increased LDH released from ARPE-19 cells, suggesting that PM25 exposure decreased cell viability in a time- and dose-dependent manner.
Particles from gasoline engine exhausts filtered by a pore size of 19 μm decrease cell viability in human bronchia epithelium airway cells (31). In addition, exposure to particle suspensions significantly increases LDH levels in rat macrophages (32), which is consistent with our data. In many previous studies, the effect of improving PM2.5-caused damage such as pulmonary injury, airway inflammation, and oxidative stress was analyzed in comparison with DEX. Thus, we have scrutinized the efficacy of SFN compared to DEX (33–36). In the present study, SFN reversed PM2.5-induced cellular toxicity. Because ROS-triggered apoptosis plays a crucial role in the pathogenesis of AMD (37). Bcl-2 family proteins, including anti-apoptotic proteins, such as Bcl-2 and pro-apoptotic proteins such as Bax, are well-known regulators of apoptosis (38). Prior studies have demonstrated that increases in the Bax/Bcl-2 ratio increase the permeability of the mitochondrial membranes, which results in cytochrome c release and subsequent caspase activation (39, 40). Among activated caspases, cleaved caspase-3 serves as the central executioner in cell death in receptor- or mitochondrial-mediated apoptosis (41). The present study demonstrated that PM2.5 exposure increased Bax, cytosolic cytochrome c, and cleaved caspase-3 protein levels and decreased Bcl-2 protein levels. However, post-treatment with SNF after PM2.5 exposure effectively reversed these pro-apoptotic changes, including decreased protein levels of Bax, cytosolic cytochrome c, and cleaved caspase-3, and increased Bcl-2 levels. This suggested that elevated intracellular ROS was related to PM2.5-induced apoptosis in ARPE-19 cells. Furthermore, previous studies reported that SGK1 promotes cell survival and inhibits cell apoptosis including cardiomyocytes (42). Interestingly, expression of SGK1 was decreased in PM2.5-treated human lung alveolar epithelial cells, and overexpression of SGK1 significantly attenuated apoptosis with reduced ROS generation (19). These results were similarly shown in the present study by the SFN treatment. Thus, SFN has a therapeutic effect against PM2.5-induced apoptosis in RPE cells by regulating mechanisms upstream of caspase-3, such as antioxidant defense mechanisms.
PM2.5 is known to cause oxidative damage (43, 44). Although it is difficult to determine the contribution of PM2.5 pollutants to total oxidative burden, many studies have shown that PM2.5, metals, carbonaceous materials, and polycyclic aromatic hydrocarbons increase ROS levels (25, 45). PM2.5-induced oxidative stress and cytotoxicity are due in part to adsorption of particle transition metals and their oxidation products, which are associated with polycyclic aromatic hydrocarbons (25, 45).
Oxidative stress occurs due to an imbalance between ROS levels and the antioxidant defense mechanisms that quench ROS (46). Antioxidant defense mechanisms, which involve antioxidant enzymes such as SOD, CAT, GSH, and GPx, prevent generation of the most reactive forms of ROS, for example hydroxyl radical, preventing oxidative damage to cellular macromolecules, including DNA, proteins, and lipids (46). SOD catalyzes the dismutation of to H2O2, and CAT quenches H2O2 (47). The present study demonstrated that PM2.5 decreased SOD and CAT antioxidant enzyme activities at high concentrations (Figure 5), which is consistent with a prior report that PM impaired the antioxidant enzymatic activities of SOD, GR, CAT, and glutathione-S-transferase in human epithelial cells (48). The results of the present study demonstrated that enzymatic activities of SOD and CAT were decreased by PM exposure, and that these effects were reversed by SFN post-treatment. These results suggest that SFN has antioxidant activity against RPE exposure to PM2.5, which was recently identified as a risk factor for AMD (28).
There are several limitations in this study. First, the main limitation is the inability to determine the precise molecular mechanisms of the SFN. Intriguingly, BAK and BAX may not always be required for pro-apoptotic stimuli to promote cytochrome c release and the consequent caspase activation (49). Second, because a wide range of retinal and choroidal pathologies are also involved in AMD such as RPE-Bruch membrane thickening, drusen accumulation, reduced blood flow, photoreceptor degeneration, cofactor accumulation, and inflammatory cytokines and chemokines, our model was not able to explain all of them. Instead, our study focused on the findings that SFN alleviated PM2.5-induced RPE cell death in the aspect of oxidative stress suggesting a potential therapeutic for AMD. We will expand our study to focus on other mechanisms such as the complemental pathway (50) and to elucidate the precise molecular mechanism.
Taken together, our findings suggested that PM2.5 treatment induced oxidative stress in RPE cells, possibly by elevated intracellular ROS and/or decreasing antioxidant enzyme activity, leading to ARPE-19 cell death. Our findings suggest that PM2.5-induced oxidative stress likely exacerbates RPE dysfunction in the context of RPE, and that SFN alleviates PM2.5-induced cell death by regulating mechanisms upstream of caspase-3, such as antioxidant defense mechanisms. These findings suggest that SFN is a potential therapeutic for AMD, which is characterized in part by RPE atrophy.
The data that support the findings of this study are available from the corresponding authors upon reasonable request.
The animal study was reviewed and approved by Animal Care Committee of Kyungpook National University (2019-0104-01).
HS, WL, DP, and J-SB: design and conduct of the study, analysis and interpretation of data, writing the manuscript, and critical revision of the manuscript. All authors: collection of data and final approval of the manuscript, contributed to the article, and approved the submitted version.
J-SB was supported by a grant from the Korea Health Technology R&D Project through the Korea Health Industry Development Institute (KHIDI), funded by the Ministry of Health & Welfare, Republic of Korea (grant number: HI15C0001) and by a National Research Foundation of Korea (NRF) grant funded by the Korean government (2020R1A2C1004131, 2017M3A9G8083382, 2017R1A5A2015391). DP was financially supported by the Basic Science Research Program of the National Research Foundation of Korea (NRF), funded by the Korean government (Ministry of Science and ICT) (2019R1A2C1084371). DP was also supported by the Ministry of Science and ICT (MSIT), Korea, under the Information Technology Research Center (ITRC) support program (IITP-2021-2020-0-01808) supervised by the Institute of Information & Communications Technology Planning & Evaluation (IITP). I-KL and DP were supported by a grant of the Korea Health technology R&D Project through the Korea Health Industry Development Institute (KHIDI), funded by the Ministry of Health & Welfare, Republic of Korea (HI16C1501).
The authors declare that the research was conducted in the absence of any commercial or financial relationships that could be construed as a potential conflict of interest.
The Supplementary Material for this article can be found online at: https://www.frontiersin.org/articles/10.3389/fmed.2021.685032/full#supplementary-material
1. Gehrs KM, Anderson DH, Johnson LV, Hageman GS. Age-related macular degeneration–emerging pathogenetic and therapeutic concepts. Ann Med. (2006) 38:450–71. doi: 10.1080/07853890600946724
2. Tokarz P, Kaarniranta K, Blasiak J. Role of antioxidant enzymes and small molecular weight antioxidants in the pathogenesis of age-related macular degeneration (AMD). Biogerontology. (2013) 14:461–82. doi: 10.1007/s10522-013-9463-2
3. Mao H, Seo SJ, Biswal MR, Li H, Conners M, Nandyala A, et al. Mitochondrial oxidative stress in the retinal pigment epithelium leads to localized retinal degeneration. Invest Ophthalmol Vis Sci. (2014) 55:4613–27. doi: 10.1167/iovs.14-14633
4. Maier KL, Alessandrini F, Beck-Speier I, Hofer TP, Diabate S, Bitterle E, et al. Health effects of ambient particulate matter–biological mechanisms and inflammatory responses to in vitro and in vivo particle exposures. Inhal Toxicol. (2008) 20:319–37. doi: 10.1080/08958370701866313
5. Kim KH, Kabir E, Kabir S. A review on the human health impact of airborne particulate matter. Environ Int. (2015) 74:136–43. doi: 10.1016/j.envint.2014.10.005
6. Lee W, Ku SK, Kim JE, Cho SH, Song GY, Bae JS. Inhibitory effects of black ginseng on particulate matter-induced pulmonary injury. Am J Chin Med. (2019) 47:1237–51. doi: 10.1142/S0192415X19500630
7. Brunekreef B, Holgate ST. Air pollution and health. Lancet. (2002) 360:1233–42. doi: 10.1016/S0140-6736(02)11274-8
8. Liu CW, Lee TL, Chen YC, Liang CJ, Wang SH, Lue JH, et al. PM2.5-induced oxidative stress increases intercellular adhesion molecule-1 expression in lung epithelial cells through the IL-6/AKT/STAT3/NF-kappaB-dependent pathway. Part Fibre Toxicol. (2018) 15:4. doi: 10.1186/s12989-018-0240-x
9. Fahey JW, Talalay P. Antioxidant functions of sulforaphane: a potent inducer of Phase II detoxication enzymes. Food Chem Toxicol. (1999) 37:973–9. doi: 10.1016/S0278-6915(99)00082-4
10. Pintea A, Rugină DO, Pop R, Bunea A, Socaciu C. Xanthophylls protect against induced oxidation in cultured human retinal pigment epithelial cells. J Food Comp Anal. (2011) 24:830–6. doi: 10.1016/j.jfca.2011.03.007
11. Bergvall C, Westerholm R. Determination of dibenzopyrenes in standard reference materials (SRM) 1649a, 1650, and 2975 using ultrasonically assisted extraction and LC-GC-MS. Anal Bioanal Chem. (2006) 384:438–47. doi: 10.1007/s00216-005-0192-5
12. Lee W, Ku SK, Kim JE, Cho GE, Song GY, Bae JS. Pulmonary protective functions of rare ginsenoside Rg4 on particulate matter-induced inflammatory responses. Biotechnol Bioprocess Eng. (2019) 24:445–53. doi: 10.1007/s12257-019-0096-4
13. Mikolka P, Kosutova P, Kolomaznik M, Topercerova J, Kopincova J, Calkovska A, et al. Effect of different dosages of dexamethasone therapy on lung function and inflammation in an early phase of acute respiratory distress syndrome model. Physiol Res. (2019) 68(Suppl 3):S253–63. doi: 10.33549/physiolres.934364
14. Lee BS, Lee C, Yang S, Ku SK, Bae JS. Renal protective effects of zingerone in a mouse model of sepsis. BMB Rep. (2019) 52:271–6. doi: 10.5483/BMBRep.2019.52.4.175
15. Jeong SY, Kim M, Park EK, Kim J-S, Hahn D, Bae J-S. Inhibitory functions of novel compounds from dioscorea batatas decne peel on HMGB1-mediated septic responses. Biotechnol Bioprocess Eng. (2020) 25:1–8. doi: 10.1007/s12257-019-0382-1
16. Lee W, Lee H, Lee T, Park EK, Bae JS. Inhibitory functions of maslinic acid, a natural triterpene, on HMGB1-mediated septic responses. Phytomedicine. (2020) 69:153200. doi: 10.1016/j.phymed.2020.153200
17. Ma YH, Karunakaran T, Veeraraghavan VP, Mohan SK, Li SL. Sesame inhibits cell proliferation and induces apoptosis through inhibition of STAT-3 translocation in thyroid cancer cell lines (FTC-133). Biotechnol Bioprocess Eng. (2019) 24:646–52. doi: 10.1007/s12257-019-0151-1
18. Zhang YY, Liu MC, Fan RP, Zhou QL, Yang JP, Yang SJ, et al. Walnut protein isolates attenuate particulate matter-induced lung and cardiac injury in mice and zebra fish. Rsc Adv. (2019) 9:40736–44. doi: 10.1039/C9RA06002B
19. Li J, Zhou Q, Yang T, Li Y, Zhang Y, Wang J, et al. SGK1 inhibits PM2.5-induced apoptosis and oxidative stress in human lung alveolar epithelial A549cells. Biochem Biophys Res Commun. (2018) 496:1291–5. doi: 10.1016/j.bbrc.2018.02.002
20. Winkler BS, Boulton ME, Gottsch JD, Sternberg P. Oxidative damage and age-related macular degeneration. Mol Vis. (1999) 5:32.
21. Plafker SM, O'Mealey GB, Szweda LI. Mechanisms for countering oxidative stress and damage in retinal pigment epithelium. Int Rev Cell Mol Biol. (2012) 298:135–77. doi: 10.1016/B978-0-12-394309-5.00004-3
22. Halliwell B. Role of free radicals in the neurodegenerative diseases: therapeutic implications for antioxidant treatment. Drugs Aging. (2001) 18:685–716. doi: 10.2165/00002512-200118090-00004
23. Liu Y, Liu M, Zhang X, Chen Q, Chen H, Sun L, et al. Protective effect of fucoxanthin isolated from laminaria japonica against visible light-induced retinal damage both in vitro and in vivo. J Agric Food Chem. (2016) 64:416–24. doi: 10.1021/acs.jafc.5b05436
24. Huang WY, Wu H, Li DJ, Song JF, Xiao YD, Liu CQ, et al. Protective effects of blueberry anthocyanins against H2O2-induced oxidative injuries in human retinal pigment epithelial cells. J Agric Food Chem. (2018) 66:1638–48. doi: 10.1021/acs.jafc.7b06135
25. Jia YY, Wang Q, Liu T. Toxicity research of PM2.5 compositions in vitro. Int J Environ Res Public Health. (2017) 14:232. doi: 10.3390/ijerph14030232
26. Lee H, Hwang-Bo H, Ji SY, Kim MY, Kim SY, Park C, et al. Diesel particulate matter2.5 promotes epithelial-mesenchymal transition of human retinal pigment epithelial cells via generation of reactive oxygen species. Environ Pollut. (2020) 262:114301. doi: 10.1016/j.envpol.2020.114301
27. Chua SYL, Khawaja AP, Dick AD, Morgan J, Dhillon B, Lotery AJ, et al. Ambient air pollution associations with retinal morphology in the UK Biobank. Invest Ophthalmol Vis Sci. (2020) 61:32. doi: 10.1167/iovs.61.5.32
28. Chua SYL, Warwick A, Peto T, Balaskas K, Moore AT, Reisman C, et al. Association of ambient air pollution with age-related macular degeneration and retinal thickness in UK Biobank. Br J Ophthalmol. (2021). doi: 10.1136/bjophthalmol-2020-316218. [Epub ahead of print].
29. Zwolak I. Comparison of three different cell viability assays for evaluation of vanadyl sulphate cytotoxicity in a Chinese hamster ovary K1 cell line. Toxicol Indus Health. (2016) 32:1013–25. doi: 10.1177/0748233714544190
30. Dumax-Vorzet AF, Tate M, Walmsley R, Elder RH, Povey AC. Cytotoxicity and genotoxicity of urban particulate matter in mammalian cells. Mutagenesis. (2015) 30:621–33. doi: 10.1093/mutage/gev025
31. Bisig C, Steiner S, Comte P, Czerwinski J, Mayer A, Petri-Fink A, et al. Biological effects in lung cells in vitro of exhaust aerosols from a gasoline passenger car with and without particle filter. Emission Control Sci Technol. (2015) 1:237–46. doi: 10.1007/s40825-015-0019-6
32. Geng H, Meng Z, Zhang Q. In vitro responses of rat alveolar macrophages to particle suspensions and water-soluble components of dust storm PM(2.5). Toxicol In Vitro. (2006) 20:575–84. doi: 10.1016/j.tiv.2005.09.015
33. Pang L, Zou S, Shi Y, Mao Q, Chen Y. Apigenin attenuates PM2.5-induced airway hyperresponsiveness and inflammation by down-regulating NF-kappaB in murine model of asthma. Int J Clin Exp Pathol. (2019) 12:3700–9.
34. Lee W, Baek MC, Kim KM, Bae JS. Biapenem as a novel insight into drug repositioning against particulate matter-induced lung injury. Int J Mol Sci. (2020) 21:1462. doi: 10.3390/ijms21041462
35. Nguyen LTH, Ahn SH, Nguyen UT, Yang IJ, Shin HM. Geniposide, a principal component of gardeniae fructus, protects skin from diesel exhaust particulate matter-induced oxidative damage. Evid Based Complement Alternat Med. (2021) 2021:8847358. doi: 10.1155/2021/8847358
36. Wang YW, Wu YH, Zhang JZ, Tang JH, Fan RP, Li F, et al. Ruscogenin attenuates particulate matter-induced acute lung injury in mice via protecting pulmonary endothelial barrier and inhibiting TLR4 signaling pathway. Acta Pharmacol Sin. (2021) 42:726–34. doi: 10.1038/s41401-020-00502-6
37. Musat O, Ochinciuc U, Gutu T, Cristescu TR, Coman C. Pathophysiology and treatment of ARMD. Oftalmologia. (2012) 56:45–50.
38. Jurgensmeier JM, Xie Z, Deveraux Q, Ellerby L, Bredesen D, Reed JC. Bax directly induces release of cytochrome c from isolated mitochondria. Proc Natl Acad Sci U S A. (1998) 95:4997–5002. doi: 10.1073/pnas.95.9.4997
39. Brentnall M, Rodriguez-Menocal L, De Guevara RL, Cepero E, Boise LH. Caspase-9, caspase-3 and caspase-7 have distinct roles during intrinsic apoptosis. BMC Cell Biol. (2013) 14:32. doi: 10.1186/1471-2121-14-32
40. Lin L, Cheng K, Xie Z, Chen C, Chen L, Huang Y, et al. Purification and characterization a polysaccharide from Hedyotis diffusa and its apoptosis inducing activity toward human lung cancer cell line A549. Int J Biol Macromol. (2019) 122:64–71. doi: 10.1016/j.ijbiomac.2018.10.077
41. Yang B, Ye D, Wang Y. Caspase-3 as a therapeutic target for heart failure. Expert Opin Ther Targets. (2013) 17:255–63. doi: 10.1517/14728222.2013.745513
42. Sun N, Meng F, Xue N, Pang G, Wang Q, Ma H. Inducible miR-145 expression by HIF-1a protects cardiomyocytes against apoptosis via regulating SGK1 in simulated myocardial infarction hypoxic microenvironment. Cardiol J. (2018) 25:268–78. doi: 10.5603/CJ.a2017.0105
43. Lodovici M, Bigagli E. Oxidative stress and air pollution exposure. J Toxicol. (2011) 2011:487074. doi: 10.1155/2011/487074
44. Valavanidis A, Vlachogianni T, Fiotakis K, Loridas S. Pulmonary oxidative stress, inflammation and cancer: respirable particulate matter, fibrous dusts and ozone as major causes of lung carcinogenesis through reactive oxygen species mechanisms. Int J Environ Res Public Health. (2013) 10:3886–3907. doi: 10.3390/ijerph10093886
45. Valavanidis A, Fiotakis K, Vlachogianni T. Airborne particulate matter and human health: toxicological assessment and importance of size and composition of particles for oxidative damage and carcinogenic mechanisms. J Environ Sci Health C Environ Carcinog Ecotoxicol Rev. (2008) 26:339–62. doi: 10.1080/10590500802494538
46. Birben E, Sahiner UM, Sackesen C, Erzurum S, Kalayci O. Oxidative stress and antioxidant defense. World Allergy Organ J. (2012) 5:9–19. doi: 10.1097/WOX.0b013e3182439613
47. Ighodaro OM, Akinloye OA. First line defence antioxidants-superoxide dismutase (SOD), catalase (CAT) and glutathione peroxidase (GPX): their fundamental role in the entire antioxidant defence grid. Alex J Med. (2018) 54:287–93. doi: 10.1016/j.ajme.2017.09.001
48. Chirino YI, Sanchez-Perez Y, Osornio-Vargas AR, Morales-Barcenas R, Gutierrez-Ruiz MC, Segura-Garcia Y, et al. PM(10) impairs the antioxidant defense system and exacerbates oxidative stress driven cell death. Toxicol Lett. (2010) 193:209–16. doi: 10.1016/j.toxlet.2010.01.009
49. Galluzzi L, Vitale I, Aaronson SA, Abrams JM, Adam D, Agostinis P, et al. Molecular mechanisms of cell death: recommendations of the Nomenclature Committee on Cell Death 2018. Cell Death Differ. (2018) 25:486–541. doi: 10.1038/s41418-017-0012-4
Keywords: age-related macular degeneration, retinal pigment epithelium, oxidative stress, retina, choroid
Citation: Sim H, Lee W, Choo S, Park EK, Baek M-C, Lee I-K, Park DH and Bae J-S (2021) Sulforaphane Alleviates Particulate Matter-Induced Oxidative Stress in Human Retinal Pigment Epithelial Cells. Front. Med. 8:685032. doi: 10.3389/fmed.2021.685032
Received: 24 March 2021; Accepted: 26 May 2021;
Published: 17 June 2021.
Edited by:
Ravirajsinh Jadeja, Augusta University, United StatesReviewed by:
Agustina Alaimo, Consejo Nacional de Investigaciones Científicas y Técnicas (CONICET), ArgentinaCopyright © 2021 Sim, Lee, Choo, Park, Baek, Lee, Park and Bae. This is an open-access article distributed under the terms of the Creative Commons Attribution License (CC BY). The use, distribution or reproduction in other forums is permitted, provided the original author(s) and the copyright owner(s) are credited and that the original publication in this journal is cited, in accordance with accepted academic practice. No use, distribution or reproduction is permitted which does not comply with these terms.
*Correspondence: Dong Ho Park, RG9uZ0hvX1BhcmtAa251LmFjLmty; Jong-Sup Bae, YmFlanNAa251LmFjLmty
†These authors have contributed equally to this work
Disclaimer: All claims expressed in this article are solely those of the authors and do not necessarily represent those of their affiliated organizations, or those of the publisher, the editors and the reviewers. Any product that may be evaluated in this article or claim that may be made by its manufacturer is not guaranteed or endorsed by the publisher.
Research integrity at Frontiers
Learn more about the work of our research integrity team to safeguard the quality of each article we publish.