- 1Laboratory SUBATECH, CNRS/IN2P3, IMT Atlantique, Université de Nantes, Nantes, France
- 2GIP ARRONAX, Saint Herblain, France
The pair of copper radionuclides 64Cu/67Cu (T1/2 = 12. 7 h/61.8 h) allows, respectively, PET imaging and targeted beta therapy. An analysis of the different production routes of 67Cu with charged particles was performed and the reaction 70Zn(d,x) route was identified as a promising one. It may allow the production of 67Cu without 64Cu. The production cross section has been measured up to 28.7 MeV. Measurements were done using the well-known stacked-foils technique using 97.5% enriched 70Zn homemade electroplated targets. These measurements complement at higher incident energies the only set of data available in nuclear databases. The results show that using a 26 MeV deuteron beam and a highly enriched 70Zn target, it is possible to produce high purity 67Cu comparable to that obtained using photoproduction. This production route can be of interest for future linear accelerators under development where mA deuteron beams can be available if adequate targetry is developed.
Introduction
67Cu (T1/2 = 61.8 h) is a radionuclide with physical properties convenient for therapeutic use as targeted radiotherapy. It is a beta-emitter with a maximum energy of 561 keV, which corresponds to an electron path of about 3 mm in water (1). Its energy range is comparable to that of the 177Lu currently used in targeted radiotherapy (2). 67Cu emits also photons of 184.6 keV (3) which offers the possibility of carrying out SPECT imaging. It can be used either prior the treatment as an imaging agent or during therapy to monitor the diffusion and distribution of the 67Cu radiolabelled radiopharmaceuticals.
To select the best production route both cross section data associated to the production of 67Cu and to contaminants are of primary importance. Among contaminants, coproduced copper isotopes are of great concern, as they cannot be removed from the final product by chemical separation. Especially 64Cu, with its 12.7 h half-life, will have an impact on the specific activity. It is then interesting to look at production routes that reduce or exclude the co-production of 64Cu, even if its real impact on the patient and staff needs to be studied and clarified.
The most cited production route for 67Cu production uses enriched 68Zn target bombarded by high-energy protons (4–7). Large quantities can be produced but it is not possible to limit 64Cu co-production. In the 90's and 2000's, the 64Ni(α,p)67Cu reaction with an 64Ni enriched target and an alpha beam was also studied. Experimental cross sections for this reaction are known with a maximum cross section value around 35 mb at 22 MeV (7, 8). The threshold energy for the production of 64Cu through 64Ni(α,n+t) is equal to 23.7 MeV. Using the very high enrichment level of 64Ni available, there is a possibility to produce 67Cu free of 64Cu by limiting the beam energy below this latter value. However, alpha beams are poorly available and thermal constrains associated to high intensity alpha beam are very penalizing.
An alternative consists to use an enriched 70Zn target bombarded with either protons or deuterons. In this case, the production of 64Cu can be limited by an appropriate choice of the beam energy and high target enrichment. As an example, the 70Zn(d,x)64Cu reaction threshold is 26.4 MeV whereas that for 67Cu production is 0 MeV (see Supplementary Table 1). 70Zn(p,α)67Cu reaction cross section reaches a maximum of 15 mb at 15 MeV (7, 9) whereas the available data for 70Zn(d,x)67Cu (10) show that the reaction cross section maximum is higher, even if the exact value is not known as this data set do not cover the whole energy range of interest.
In this work, we have measured 70Zn(d,x)67Cu production cross section up to 28.7 MeV in order to determine the position and value of the maximum. Production cross sections of contaminants have been also extracted. Using these new data, we were able to determine production yields and, with the help of TALYS 1.9 calculations (12), the expected specific activity of the final product.
Materials and Methods
Production cross sections for the 70Zn(d,x)67Cu reaction was measured using the stacked-foils activation method (4, 13–16). A series of six irradiations, spread for over 7 months, was carried out at the GIP ARRONAX C70 cyclotron, Saint-Herblain, France. In our experiments, a stack was made of two patterns each composed of a 10 μm thick enriched 70Zn (97.5% purity) electroplated on a 25 μm Ni foil (99.9% purity) followed by an aluminum foil (10 μm, 99.0% purity). Their thicknesses were determined assuming homogeneity by weighing and performing surface calculation with a high definition scanner. The obtained values are reported in Supplementary Table 2. Aluminum is used as a foil to catch recoil nuclei. The stack was placed inside a dedicated vacuum chamber positioned at the end of the AX beam line. It contains an instrumented Faraday cup used to determine the particle flux going through the stack. We limited the total thickness of the stack to prevent a large geometrical straggling that will result on an increased uncertainty on the flux measurement. A Ti foil, having an area equivalent to the 70Zn deposit, was added between the Ni and Al foil of the second pattern to obtain a second independent flux value by measuring the production cross section associated to natTi(d,x)48V. This reaction is well-known and is used as a reference (17) (monitor) to make sure everything went well during our experiments. After irradiation which stands for 1 h with an average current of ~50 nA, activities of each thin foils were measured using gamma spectroscopy (HPGe). The well-known activation formula was used to calculate the cross section values.
Gamma analyses were carried out using the FitzPeaks software (18). Spectra were recorded in a suitable geometry calibrated in energy and efficiency with standard 57Co, 60Co, and 152Eu sources from LEA-CERCA (France). The full widths at half maximum were 1.05 keV at 122 keV (57Co) and 1.97 keV at 1,332 keV (60Co). No activity was measured for recoil nuclei on catching foils. The 48V activity was measured only after full 48Sc decay, 3 weeks after End Of Beam (EOB).
The energy loss of the particles passing through the stack has been calculated from the equations of Ziegler et al., using their SRIM-2013 software (19). The energies are calculated in the middle of the foils and are shown in Supplementary Table 2.
Chemical preparations and electroplating were made on site using enriched 70Zn metallic powder from Trace Sciences International. The enrichment level of 70Zn was 97.5%, 68Zn 2.2%, 67Zn 0.1%, 66Zn 0.1% and 64Zn 0.1%. All solutions were freshly prepared with ultra-pure water treated with Milipore Milli Q system. The metallic powder was dissolved in diluted sulfuric acid (1 M) to obtain zinc sulfate, then evaporated to dryness and rinsed twice with ultra-pure water. For each preparation, pH was adjusted to two by addition of sulfuric acid. The electroplating was carried out in a simple homemade three-electrode Teflon cell. The counter electrode was made of platinum and an Ag-|AgCl|Cl− (saturated KCl) electrode was used as reference and was connected to the cell. The deposition area was delimited during electroplating using a silicon gasket and corresponds to 4 cm2. Electroplating was performed by using the VoltaLab050 potentiostat. The deposition was obtained by applying a constant current density of −20 mA/cm2. During plating, the temperature was kept constant at 30°C and the solution was stirred at 300 rpm for homogenization purpose. To reach a thickness of 10 μm, a deposition time of 30 min was necessary.
The presence of 68Zn (2.2%) in the target material implies potential contamination, which is taken into account during the analysis. Indeed, the interaction of deuterons on 68Zn can produce 67Ga (Ethreshold = 14.6 MeV) whereas 67Ga is not produced in our energy range by deuteron interactions with 70Zn (Ethreshold = 30.8 MeV). 67Ga decays to 67Zn as 67Cu leading to common gamma rays during both decays, fortunately with different intensities. As an example, the 184 keV gamma ray corresponds to an intensity of 48.7% in 67Cu decay whereas it is only 21.41% for 67Ga decay (3). The same holds for the 300 keV gamma line which intensity is 0.797% for 67Cu and 16.64% for 67Ga. Therefore, we used this property to discriminate production of 67Cu and 67Ga. This is based on a set of equations (1−3) involving the number of gamma collected at 184 keV and 300 keV. These equations relate to the total number of gammas collected, NTOT, from a gamma peak to the number of gamma collected from each contributor.
Equations can be written as:
Where i corresponds to a specific radionuclide, × to a given gamma line, ε to the detector efficiency at this energy, I to the intensity of the gamma emission, λ to the radioactive constant and tLT to the acquisition time. Expressed in terms of activity of each radionuclide, the system of equations is written as follows:
The activities of 67Cu and 67Ga are determined from equations in (3). The uncertainties associated with this activity calculation have been established according to the following equation:
Where y represents the different parameters involved in each equation (3).
Results and Discussion
In these experiments, excitation functions up to 28.7 MeV were measured for 67Cu and 67Ga from the zinc deposit whereas 61Cu, 55, 56, 57, 58Co were extracted from the Ni backing and 48V from the Ti foil. All nuclear reactions involved are reported in the Supplementary Table 3 as well as gamma lines used for the analysis of each radionuclide. Our results are displayed on Figures 1–3, in values reported in Table 1. The simulation code of nuclear reactions TALYS 1.9 was used to extend the study in particular on the stable (65Cu) nucleus production. This is the reason why, in addition to our data points, we have displayed on our figures the values obtained with TALYS 1.9 (12). Reactions on the Ni support and on the Ti foil are monitor reactions for which reference data exist at IAEA (17). Through these monitor cross section values, we can control the measurement of the particle flux and consequently the correct execution of the experiment.
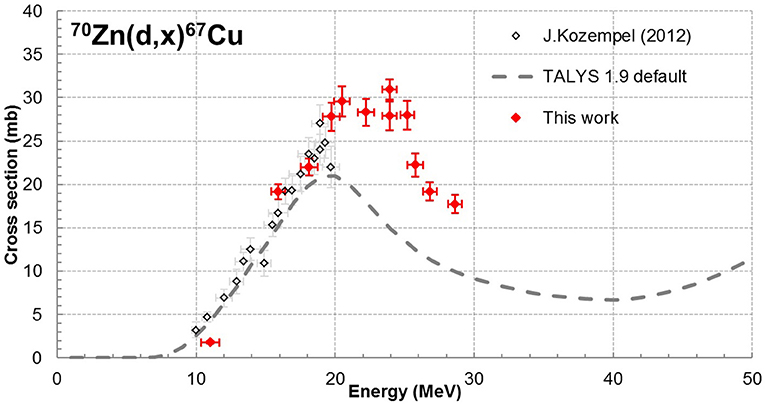
Figure 1. Cross sections of reaction 70Zn(d,x)67Cu (10).
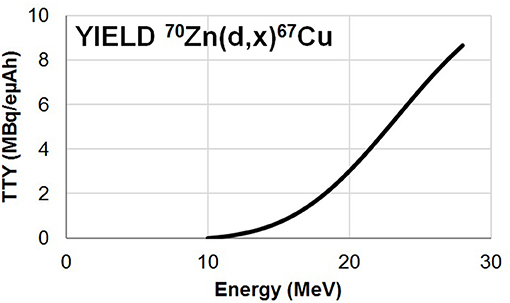
Figure 2. Thick Target Yield curve for the 70Zn(d,x)67Cu reaction (11).
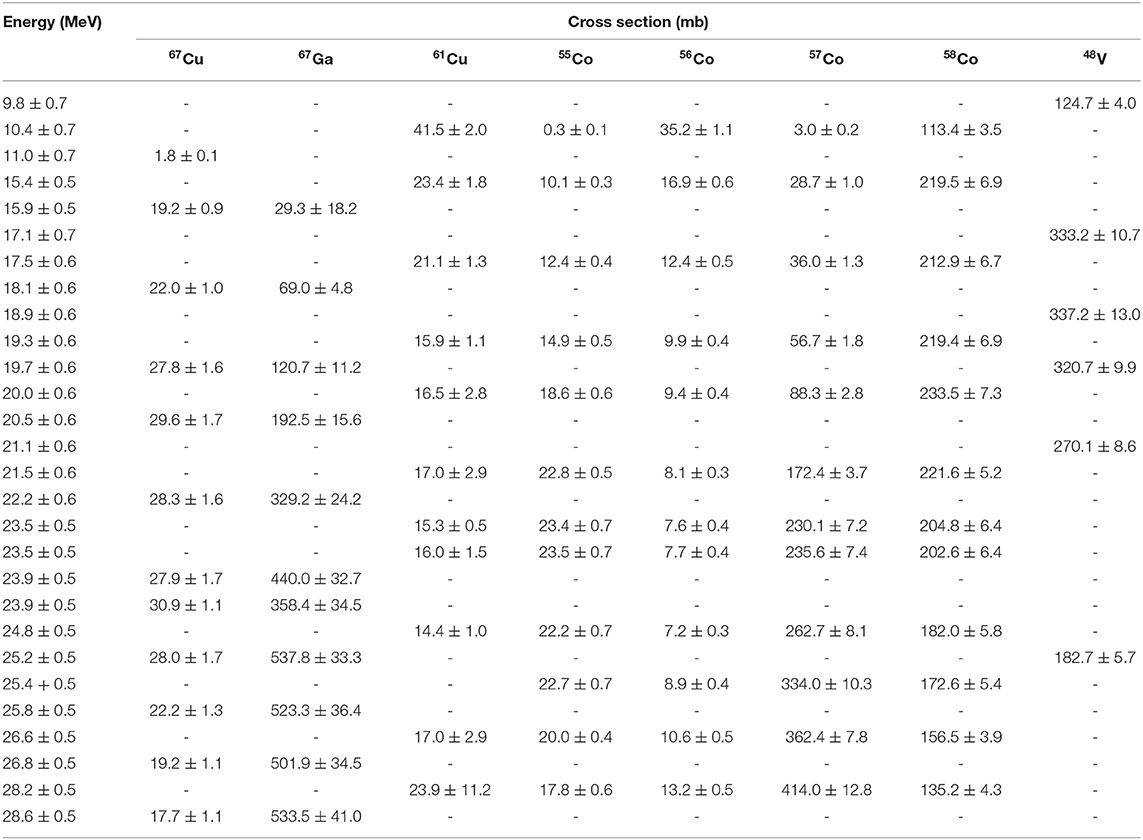
Table 1. Cross section values measured in this study for reactions having taken place in zinc, nickel, and titanium.
The natTi(d,x)48V Reaction
48V (T1/2 = 15.973 d) is produced by the natTi(d,x)48V reaction. The foil has been cut and positioned to have the same surface area as the 70Zn deposit. If the entire beam does not pass through the deposit because it is too wide, this will also be the case for the titanium foil. In this case, the extracted values will not be in agreement with the monitor cross section.
During the irradiation of a titanium foil, not only 48V is produced but also 48Sc which decay to the same daughter nucleus than 48V. To get rid of 48Sc, we let it decay, at least 19 days, until the vast majority of the 48Sc disintegrates. The results are presented in Supplementary Figure 1.
These data are in agreement with experimental values available in the literature (20–25). The agreement of these data shows that the foils and deposits were crossed by the entire beam. The agreement is generally good with the cross section recommended by the IAEA (13). In agreement with experimental data in the literature, our points indicate a peak around 19 MeV which is not described by the IAEA curve.
The natNi(d,x)61Cu Reaction
The 61Cu (T1/2 = 3.339 h) is produced by the natNi(d,x) reaction. The gamma emissions used for activity measurement are 282.956 keV (12.2%), 373.050 keV (2.15%), 588.605 keV (1.17%), 656.008 keV (10.77%), 908.631 keV (1.102%), and 1185.234 keV (3.75%). Ni is the backing of the Zn target. This reaction is a monitor reaction for which the IAEA proposes a reference curve. Our data are presented in Supplementary Figure 2. One data point shows large error bar. This is due to a late counting that induces a lack of statistics. However, our data are in good agreement with experimental data available in the literature (20–22, 26–31) and with the IAEA curve (17). This confirms that the experiment was well-controlled.
The 70Zn(d,x)67Cu Reaction
67Cu (T1/2 = 61.83 h) cross sections were determined from gamma emissions at 184.6 keV (48.7%) and 300.2 keV (0.797%) and Equation 3. The production contribution of 67Cu from 68Zn (present at 2.2%) is not taken into account as it is expected to be negligible [of the order of 1 mb in the model calculation TALYS 1.9 (12)]. Our data are presented in Figure 1.
Our data complement the data already presented in the literature (10) to higher incident energies. They are in good agreement with Kozempel et al. (10) and allow determining the energy of the maximum of the cross section near 23 MeV and its value around 30 mb. This information will allow to more precisely defining optimal beam parameters for 67Cu production using a deuteron beam and a 70Zn target.
The TALYS 1.9 simulation code was used with its default set of parameters to calculate the cross section of the 70Zn(d,x)67Cu reaction. The code calculation is not able to describe the data. There is a slight shift toward lower energies of the maximum and the data are underestimated by the code calculation.
Using our dataset and that of Kozempel et al., we have performed a 67Cu thick target yield calculation (TTY) according to (32). In the formula (5), σ is the production cross section, H is the enrichment and the purity of the foil, Na is the Avogadro's number, λ is the decay constant of the radioisotope, Z is the charge of the fully ionized projectile, e is the elementary charge, M is the atomic mass of the target, Emax and Emin are the maximal and minimal energy of the projectile penetrating the target and dE/dx is the stopping power of the projectile in the irradiated target. The result is plotted on Figure 2 as a function of the incident deuteron energy.
We can clearly see that the yield increases more rapidly around the maximum as expected. Taking into account the threshold of 26.4 MeV associated to the production of 64Cu, the shape of the 67Cu cross section and the high price of 70Zn, the preferred energy range for production through this route is 16–26 MeV. This energy range corresponds to a 70Zn thickness of 576 μm.
By setting the beam intensity at 1 μA, 1 h of irradiation and a target purity of 97.5%, the estimated activity produced over the 16–26 MeV energy range is 6.2 MBq. This result is higher than that of Hosseini et al. (33) which corresponds to model calculations. The main difference comes from the cross section values used in this study that are lower than those experimental ones.
Interesting information is related to the expected specific activity of the final product. To determine the contribution of each copper isotope, experimental data were used for 67Cu and TALYS 1.9 calculations using default parameters for the other isotopes.
With 80 μA and 40 h of irradiation, the expected activity of 67Cu EOB is 16.4 GBq and the 67Cu represents only 35.77% of the total copper activity due to the production of short-lived 66, 68, 69Cu (T1/2 : 5.12 min; 0.515 min; 2.85 min). However, by waiting 70 min after irradiation for decays, the activity of 67Cu reaches 99.99% of the total copper activity and, at this time, the specific activity is 1.87 × 103 MBq/nmol or 2.79 × 104 GBq/mg. This specific activity value is very close to the theoretical maximum (2.80 × 104 GBq/mg). This small difference is due to the production of 65Cu.
Using an enriched target such as the one used in this study (97.5%), 67Cu represents 99.99% of the copper activity after 121 h of decay and the specific activity is 2.52 × 104 GBq/mg (99.00% reached for 15 h of decay). This is due to the production of 64Cu in a thick target containing a non-negligible proportion of 68Zn. Using a higher enrichment will reduce the impact of other copper isotopes and especially 64Cu. However, during this 15 h decay time, the copper extraction chemistry can be performed as well as the sample delivery.
The 68Zn(d,x)67Ga Reaction
In our experimental condition, 67Ga (T1/2 = 78.3 h) is produced only from the residual amount of 68Zn through 68Zn(d,3n) reaction. Indeed, the energy threshold for the production of 67Ga using 70Zn is equal to 30.77 MeV. As 67Ga decays to the same daughter nuclide as 67Cu, its contribution in the spectra was extracted from equations (3) using gamma emissions of 184.6 keV (21.41%) and 300.2 keV (16.64%). Cross sections data for the 68Zn(d,3n)67Ga reaction are shown in Figure 3 as red dots. There is no data for this reaction in the literature. The only possibility is to compare to calculated values using the TALYS 1.9 code with the default set of parameters (dashed line). The amplitude of the cross section is compatible with the data. Additional data at higher energies will help to constrain the theoretical models contained in the simulation code. The cross section is relatively high which implies, despite a 68Zn concentration of 2.2%, a non-negligible activity production of 67Ga. The percentage of 67Ga in the total 67Cu+67Ga activity EOB varies from 2.4% to 31.8% with the minimum at 15.9 MeV and the maximum at 26.8 MeV which follow the minimum and maximum of the cross section curve, Figure 3.
The 64Cu Production
The 64Cu (T1/2 = 12.701 h) emits a gamma of 1345.77 keV at 0.475% during its beta+ decay to 61.5%. Due to the low emission intensity, it was not detected. Moreover, its production is possible on several isotopes present in the target (68Zn and 70Zn) and with nickel support (62Ni and 64Ni) which do not allow unambiguous identification of its origin. Therefore, the calculation of the cross section of a specific reaction could not be done. So, no cross section values for 64Cu are presented.
Discussion
In this work, we have determined the 67Cu production cross section associated to the use of a deuteron beam impinging an enriched 70Zn target. This production route is of great interest as it limits strongly the production of 64Cu that is directly linked to the level of 68Zn impurity in the target. In our study, data up to 28.7 MeV have been obtained using the stacked-foils technique. Beam intensity has been obtained using an instrumented Faraday cup. Cross sections for the following monitor reaction natTi(d,x)48V, natNi(d,x)56Co, natNi(d,x)56Co, and natNi(d,x)61Cu have been extracted from the target backing and the Ti monitor foil. These experimental values are in agreement with datasets available in the literature indicating that the experiment was well-controlled. Our new data on 70Zn(d,x)67Cu allows to clearly identifying the maximum of the cross section around 30 mb for an incident energy of 23 MeV. Based on these data, we propose to use a deuteron beam of 26 MeV and a target of 576 μm (leading to outgoing deuteron energy of 16 MeV) as optimum irradiation parameters. This leads to a production yield of 6.4 MBq/μA/h and allows the production of 16.4 GBq with a specific activity of 2.79 × 104 GBq/mg for an irradiation of 40 h with an intensity of 80 μA followed by a decay period of 70 min and with a 100% enriched 70Zn target. These amounts of 67Cu activity produced with high specific activity especially without the presence of 64Cu are suitable for clinical studies. This makes the 70Zn(d,x) an attractive production route for 67Cu. It can become the production route of choice only if the use of linear accelerators such as SPIRAL2 (34) or SARAF (11) is set-up that will provide beam intensities in the mA range and if adequate targetry is developed.
Data Availability Statement
The raw data supporting the conclusions of this article will be made available by the authors, without undue reservation.
Author Contributions
EN: main contributor (experiment, analyses, and article writing). AG: experiment and article review. FH: project leader and article review. TS: experiment, target manufacturing, and article review. All authors contributed to the article and approved the submitted version.
Funding
The cyclotron ARRONAX was supported by CNRS, Inserm, INCa, the Nantes University, the Regional Council of Pays de la Loire, local authorities, the French government and the European Union. This work has been, in part, supported by a Grant from the French National Agency for Research called Investissements d'Avenir, Equipex Arronax-Plus noANR-11-EQPX-0004, Labex IRON noANR-11-LABX-18-01 and ISITE NExT no ANR-16-IDEX-007.
Conflict of Interest
The authors declare that the research was conducted in the absence of any commercial or financial relationships that could be construed as a potential conflict of interest.
Supplementary Material
The Supplementary Material for this article can be found online at: https://www.frontiersin.org/articles/10.3389/fmed.2021.674617/full#supplementary-material
References
1. NIST (National Institute of Standards and Technology) Software online. Stopping Powers and Ranges for Electrons (estar). Available online at: https://physics.nist.gov/PhysRefData/Star/Text/ESTAR.html.
2. Bailly C, Vidal A, Bonnemaire C, Krarber-Bodéré F, Chérel M, Pallardy A, et al. Potential for nuclear medicine therapy for gliobastoma treatment. Front Pharmacol. (2019) 10:772. doi: 10.3389/fphar.2019.00772
3. Chart of Nuclides from National Nuclear Data Center (NNDC). Database NuDat 2.8. Available online at: https://www.nndc.bnl.gov/nudat2/
4. Pupillo G, Sounalet T, Michel N, Mou L, Esposito J, Haddad F. New production cross sections for the theranostic radionuclide 67Cu. Nucl Instrum Methods Phys Res Sect B Beam Interact Mater Atoms. (2018) 415:41–47. doi: 10.1016/j.nimb.2017.10.022
5. Szelecsényi F, Steyn GF, Dolley SG, Kovács Z, Vermeulen C, van der Walt NT. Investigation of the 2009 68Zn(p:2p)67Cu nuclear reaction: new measurements up to 40 MeV and compilation up to 100 MeV. Nucl Instrum Methods Phys Res Sect B Beam Interact Mater Atoms. (2009) 267:1877–81. doi: 10.1016/j.nimb.2009.03.097
6. Stoll T, Kastleiner S, Shubin YN, Coenen HH, Qaim SM. Excitation functions of proton induced reactions on 68Zn from threshold up to 71 MeV, with specific reference to the production of 67Cu. Radiochim Acta. (2002) 90:309–13. doi: 10.1524/ract.2002.90.6.309
7. Levkovski V. Cross Sections of Medium Mass Nuclide Activation (A=40-100) by Medium Energy Protons and Alpha Particles (E=10-50 MeV). Inter-Vesi, Moscow: USSR (1991).
8. Skakun Y, Qaim SM. Excitation function of the 64Ni(α,p)67Cu reaction for production of 67Cu. Appl Radiat Isot. (2004) 60:33–9. doi: 10.1016/j.apradiso.2003.09.003
9. Kastleiner S, Coenen HH, Qaim SM. Possibility of production of 67Cu at a small-sized cyclotron via the (p,a)-reaction on enriched 70Zn”, Radiochim. Acta. (1999) 84:107–10. doi: 10.1524/ract.1999.84.2.107
10. Kozempel J, Abbas K, Simonelli F, Bulgheroni A, Holzwarth U, Gibson N. Preparation of 67Cu via deuteron irradiation of 70Zn”, Radiochim. Acta. (2012) 100:419–23. doi: 10.1524/ract.2012.1939
11. Pichoff N, Bredy P, Ferrand G, Girardot PF, Gougnaud F, Jacquemet M, et al. The SARAF-LINAC Project for SARAF-PHASE. 2. In: 6th International Particle Accelerator Conference (IPAC2015). Richmond (2015). p. 3 683–5.
12. Koning A, Hilaire S, Goriely S. Open Souce Software GPL license TALYS-1.9. Petten: NRG Petten; Bruyeres-le-Chatel: CEA-Bruyeres-le-Chatel; Brussels: University of Brussels; Vienna: International Atomic Energy Agency (2017).
13. Garrido E, Duchemin C, Guertin A, Haddad F, Michel N, Metivier V. New excitation functions for proton induced reactions on natural titanium, nickel and copper up to 70 MeV. NIMBB. (2016) 383:191–212. doi: 10.1016/j.nimb.2016.07.011
14. C Duchemin, Guertin A, Haddad F, Michel N, Metivier V. Production of scandium-44m and scandium-44g with deuterons on calcium-44: cross section measurements and production yield. Phys Med Biol. (2015) 60:6847. doi: 10.1088/0031-9155/60/17/6847
15. Duchemin C, A Guertin, Haddad F, Michel N, Metivier V. Cross section measurements of deuteron induced nuclear reactions on natural tungsten up to 34 MeV. Appl Rad Isotopes. (2015) 97:52–8. doi: 10.1016/j.apradiso.2014.12.011
16. Sitarz M, Nigron E, Guertin A, Haddad F, Matulewicz T. New cross sections for natMo(a,x) reaction and medical 97Ru production estimations with radionuclide yield calculator. Instruments. (2019) 3:7. doi: 10.3390/instruments3010007
17. Hermanne A, Ignatyuk AV, Capote R, Carlson BV, Engle JW, Kellett MA, et al. Reference cross sections for charged-particle monitor reactions. Nucl Data Sheets. (2018) 148:338–2. doi: 10.1016/j.nds.2018.02.009
18. Fitzgerald J, Servives's JC. Software Fitzpeaks Gamma Analysis Software, Software version 3.66. Oxfordshire.
19. Ziegler JF, Biersack JP, Ziegler, MSoftware D. SRIM: The Stopping and Range of Ions in Matter. SRIM Company (2008).
20. Takács S, Sonck M, Scholten B, Hermanne A, Tárkányi F. Excitation functions of deuteron induced nuclear reactions on (nat)Ti up to 20 MeV for monitoring deuteron beams. Appl Radia. Isot. (1997) 48:657–65. doi: 10.1016/S0969-8043(97)00001-8
21. Takács S, et al. New cross-sections and intercomparison of deuteron monitor reactions on Al, Ti, Fe, Ni and Cu. Nucl Instru Methods Phys Res Sect B Beam Interact Mater Atoms. (2001) 174:235–58. doi: 10.1016/S0168-583X(00)00589-9
22. Takács S, Tárkányi F, Király B, Hermanne A, Sonck M. Evaluated activation cross sections of longer-lived radionuclides produced by deuteron induced reactions on natural nickel. Nucl Instru Methods Phys Res Sect B Beam Interact Mater Atoms. (2007) 260:495–507. doi: 10.1016/j.nimb.2006.11.136
23. Gagnon K, Avila-Rodriguez MA, Wilson J, McQuarrie SA. Experimental deuteron cross section measurements using single natural titanium foils from 3 to 9 MeV with special reference to the production of 47V and 51Ti. Nucl Instru Methods Phys Res Sect B Beam Interact Mater Atoms. (2010) 268:1392–98. doi: 10.1016/j.nimb.2010.01.025
24. Khandaker MU, Haba H, Kanaya J, Otuka N. Excitation functions of (d,x) nuclear reactions on natural titanium up to 24 MeV. Nucl Instru Methods Phys Res Sect B Beam Interact Mater Atoms 2013) 296:14–21. doi: 10.1016/j.nimb.2012.12.003
25. Khandaker MU, Haba H, Murakami M, Otuka N. Production cross-sections of long-lived radionuclides in deuteron-induced reactions on natural zinc up to 23 MeV. Nucl Instru Methods Phys Res Sect B Beam Interact Mater Atoms. (2015) 346:8–16. doi: 10.1016/j.nimb.2015.01.011
26. Zweit J, Smith AM, Downey S, Sharma HL. Excitation functions for deuteron induced reactions in natural nickel: production of no-carrier-added 64Cu from enriched 64Ni targets for positron emission tomography. Int J Radiat Appl Instrum Part. (1991) 42:193–7. doi: 10.1016/0883-2889(91)90073-A
27. Hermanne A, Tárkányi F, Takács S, Kovalev SF, Ignatyuk A. Activation cross sections of the 64Ni(d,2n) reaction for the production of the medical radionuclide 64Cu. Nucl Instru Methods Phys Res Sect B Beam Interact Mater Atoms. (2007) 258:308–12. doi: 10.1016/j.nimb.2007.02.071
28. Ochiai K, Nakao M, Kubota N, Sato S, Yamauchi M, Ishioka NH, et al. Deuteron induced activation cross section measurement for IFMIF. In: ND 2007, International Conference on Nuclear Data for Science and Technology. Nice (2008). p. 3–6. doi: 10.1051/ndata:07663
29. Hermanne A, Takács S, Adam-Rebeles R, Tárkányi F, Takács MP. New measurements and evaluation of database for deuteron induced reaction on Ni up to 50 MeV. Nucl Instru Methods Phys Res Sect B Beam Interact Mater Atoms. (2013) 299:8–23. doi: 10.1016/j.nimb.2013.01.005
30. Avrigeanu M, Šimečková E, Fischer U, Mrázek J, Novak J, Štefánik M, et al. Deuteron-induced reactions on Ni isotopes up to 60MeV. Phys Rev C. (2016) 94:1–16. doi: 10.1103/PhysRevC.94.014606
31. Usman AR, Khandaker MU, Haba H, Murakami M, Otuka N. Measurements of deuteron-induced reaction cross-sections on natural nickel up to 24 MeV. Nucl Instru Methods Phys Res Sect B Beam Interact Mater Atoms. (2016) 368:112–9. doi: 10.1016/j.nimb.2015.10.077
33. Hosseini SF, Aboudzadeh M, Sadeghi M, Teymourlouy AA, Rostampour M. Assessment and estimation of 67Cu production yield via deuteron induced reactions on natZn and 70Zn. Appl. Rad. Isotopes. (2017) 127:137–41. doi: 10.1016/j.apradiso.2017.05.024
Keywords: 67Cu, production, theranostic, cross section, deuteron reactions, accelerators
Citation: Nigron E, Guertin A, Haddad F and Sounalet T (2021) Is 70Zn(d,x)67Cu the Best Way to Produce 67Cu for Medical Applications? Front. Med. 8:674617. doi: 10.3389/fmed.2021.674617
Received: 01 March 2021; Accepted: 14 June 2021;
Published: 05 July 2021.
Edited by:
Thierry Stora, European Organization for Nuclear Research (CERN), SwitzerlandReviewed by:
Roger Schibli, Paul Scherrer Institut (PSI), SwitzerlandMoumita Maiti, Indian Institute of Technology Roorkee, India
Copyright © 2021 Nigron, Guertin, Haddad and Sounalet. This is an open-access article distributed under the terms of the Creative Commons Attribution License (CC BY). The use, distribution or reproduction in other forums is permitted, provided the original author(s) and the copyright owner(s) are credited and that the original publication in this journal is cited, in accordance with accepted academic practice. No use, distribution or reproduction is permitted which does not comply with these terms.
*Correspondence: Etienne Nigron, nigron@subatech.in2p3.fr; Ferid Haddad, haddad@subatech.in2p3.fr