- 1Department of Medical and Surgical Sciences, Magna Graecia University, Catanzaro, Italy
- 2Geriatrics Division, Azienda Ospedaliero-Universitaria Mater Domini, Catanzaro, Italy
- 3Geriatrics Division, Azienda Ospedaliera Annunziata, Cosenza, Italy
- 4Department of Clinical and Molecular Medicine, La Sapienza University, Rome, Italy
Background: Obstructive sleep apnea syndrome (OSAS) is an independent risk factor for cardiovascular morbidity and mortality, and it has a detrimental effect on renal function. Obesity is the major risk factor for OSAS, and represents a risk factor for chronic kidney disease. Continuous positive airway pressure (CPAP) is the suggested therapy for moderate-to-severe OSAS. We designed this study to evaluate the effect of CPAP on estimated glomerular filtration rate (e-GFR) in a cohort of obese patients with moderate-to-severe OSAS and normal renal function.
Methods: We enrolled 198 obese subjects, divided into two groups (OSAS+ and OSAS–), on the basis of cardiorespiratory monitoring; mild OSAS patients (n = 33) were excluded from the study, thus the analyses were conducted on 165 patients. Comparisons between groups were made by Student t-test or χ2 test as appropriate. Linear regression analyses were used to assess the relationship between baseline e-GFR and different covariates and, in the OSAS+ group, between Δe-GFR and different covariates. A multivariate regression analysis was performed to determinate the independent predictor of the Δe-GFR.
Results: OSAS+ subjects showed significantly increased values of systolic blood pressure, HOMA, pulse wave velocity, high-sensitivity C reactive protein and uric acid compared with OSAS– group. OSAS+ group showed significantly lower values of e-GFR and increased values of microalbuminuria. At linear regression analysis e-GFR resulted significantly and inversely related to AHI in the whole study population and in the two groups. After 6 months of CPAP therapy, OSAS+ subjects showed an improvement in respiratory parameters, as well as a significant increase in e-GFR values (104.2 + 19.0 vs. 84.0 + 13.1 ml/min/1.73 m2, P < 0.0001). At multiple regression analysis, Δ apnea/hypopnea index (AHIa) resulted the main independent predictor of Δe-GFR explaining 22% of its variation.
Conclusions: Obese OSAS patients show significantly lower values of e-GFR, even if in the normal range, compared with obese non-OSAS subjects. After 6 months of CPAP, e-GFR significantly improved (+20 ml/min/1.73 m2) and ΔAHIa resulted the most important independent predictor of Δe-GFR.
Introduction
In the last years obstructive sleep apnea syndrome (OSAS) has been considered as an independent risk factor for cardiovascular (CV) morbidity and mortality, similarly to other classical risk factors such as obesity, diabetes mellitus (DM), and hypertension (1). Even if the pathogenetic mechanisms underlying the association between OSAS and CV diseases have been not completely elucidated, hypoxia-induced endothelial dysfunction (2, 3), insulin resistance (4), and sympathetic-related CV hemodynamics (5, 6) seem to play a pivotal role.
Renal function decline, evaluated by estimated glomerular filtration rate (e-GFR), is associated with increased CV morbidity and mortality in the general population as well as in various settings of patients (7–9), and mild-to-moderate renal insufficiency has emerged as a major public and clinical health problem. It has been hypothesized that chronic kidney disease (CKD) shares common pathogenetic mechanisms with OSAS, such as increased oxidative stress, metabolic alterations and sympathetic activation, which could explain the link between OSAS and renal function decline (10). Moreover, secondary analyses of intervention studies conducted in both hypertensive patients (11) and selected patients at high CV risk (12) have shown that classical CV risk factors such as hypertension, DM, hyperlipidemia, smoking, and overweight/obesity represent major correlates of renal dysfunction.
However, the relationship between OSAS and renal function decline needs to be further elucidated; it is well-known that OSAS is highly prevalent among individuals with end-stage renal disease (ESRD) (13) and that patients with early stages of CKD show an increased risk of OSAS compared to subjects with normal renal function (14), but the cause-effect link between these conditions has not yet been clearly explained. In fact, there is also evidence that ESRD may induce a progression of CKD, thus indicating a bidirectional relationship between the conditions (15).
On the other hand, several data showed that obesity, the main risk factor for OSAS, is also associated with dysregulation of renal function, such as glomerular hyperfiltration and increased albuminuria, thus representing a risk factor for CKD and its progression to kidney failure (16–18).
Currently, the gold-standard treatment of moderate-severe OSAS is continuous positive airway pressure (CPAP), regardless of body weight (19). This therapy has shown a reduction/disappearance of nocturnal hypoxic episodes which, in turn, determinates the improvement of several hemodynamic and cardiometabolic parameters.
Previous studies investigating the effect of CPAP treatment on renal function were conducted in patients with CKD (20) or in non-obese OSAS patients with normal renal function (21) but, to our knowledge, no data exist in obese OSAS subjects with normal renal function. Thus, we designed this study to evaluate the effect of CPAP therapy on renal function in a cohort of well-characterized obese patients with moderate-to-severe OSAS and preserved renal function (e-GFR > 60 ml/min/1.73 m2).
Materials and Methods
Study Population
We recruited 198 obese Caucasian outpatients (body mass index, BMI >30 Kg/m2; 110 males and 55 females; mean age 55.3 ± 11.0 years) referred to the Sleep Breathing Disorders Clinic of the University Hospital of Catanzaro, who underwent cardiorespiratory monitoring (CRM) during sleep for reported daytime sleepiness and persistent (>6 months) snoring. None of the patients had history or clinical evidence of angina, myocardial infarction, valvular heart disease, arrhythmias, peripheral vascular disease, arterial hypertension, DM, coagulopathy or any disease predisposing to vasculitis or Raynaud's phenomenon, liver and respiratory disease, lung failure, CKD (defined for e-GFR values <60 ml/min/1.7 m2) or urinary tract infections. At the time of the first evaluation, we collected medical history and performed physical examination evaluating body weight, height and BMI, waist and neck circumference (NC), determination of arterial stiffness, routine blood tests, and 75-g oral glucose tolerance test (OGTT) after 12 h fasting, 0 and 120 min of sampling for plasma glucose and insulin determination. According with clinical guidelines for OSAS management (22), all subjects participating to the study received general education about the importance of weight loss, sleep position, alcohol avoidance and risk factors modifications. Subjects who were diagnosed with moderate-to-severe OSAS were prescribed with CPAP therapy. In accordance with the aim of the study, OSAS patients treated with CPAP were specifically re-evaluated for respiratory parameters, renal function and CV risk factors 6 months after the beginning of CPAP therapy; after the observation period, appropriate anti-hypertensive and/or insulin-sensitizers medications were prescribed, when needed. At the end of the follow-up period, all subjects whose BMI remained in the obesity range were prescribed with a hypocaloric diet with a caloric deficit of 500 kcal/die, in order to achieve a BMI of 25 kg/m2 or less. The local Ethics Committee (Calabria Centro) approved the protocol (approval number 2,012.63) and informed written consent was obtained from all participants. All the investigations were performed in accordance with the principles of the Declaration of Helsinki.
Laboratory Determinations
All laboratory measurements were performed after a fast of at least 12 h. Plasma glucose was determined immediately by the glucose oxidase method (Glucose Analyzer, Beckman Coulter S.p.A., Milan, Italy). Triglyceride and total, low-density lipoprotein (LDL), and high-density lipoprotein (HDL) cholesterol concentrations were measured by enzymatic methods (Roche Diagnostics GmbH, Mannheim, Germany). Plasma concentrations of insulin were determined by chemiluminescence test (Roche Diagnostics). Insulin resistance was determined by homeostasis model assessment (HOMA) index, calculated according with the following equation:
(23)
that is strongly related to the euglycemic clamp, which represents the gold standard test for measuring insulin sensitivity. Creatinine measurements and uric acid (UA) were performed at baseline by use of the Jaffe methodology and the uricase peroxidase (uricase/POD; Boehringer Mannheim, Mannheim, Germany) method implemented in an autoanalyzer. Values of e-GFR (mL/min/1.73 m2) were calculated with the equation proposed by investigators in the Chronic Kidney Disease Epidemiology (CKD-EPI) Collaboration (24). This equation was developed from a cohort of patients that included both healthy individuals and individuals with chronic kidney disease and that was much larger than the Modification of Diet in Renal Disease study. We preferred this equation because it is more accurate in subjects with GFR > 60 mL/min/1.73 m2, which our patients were expected to have given the inclusion criteria. Total serum insulin-like growth factor-1 (IGF-1) concentrations were determined by chemiluminescent immunoassay (Nichols Advantage, Nichols Institute Diagnostic, San Juan Capistrano, CA). High sensitivity C-reactive protein (hs-CRP) levels were measured by an automated instrument (CardioPhaseH hsCRP, Siemens, Italy).
Cardio-Respiratory Monitoring
To confirm the diagnosis of OSAS, patients underwent a CRM during sleep (Compumedics, Somtè), with continuous non-invasive recordings of respiratory pressure and flow, thoracic-abdominal motion, oxygen saturation, ECG and body position. Results from the sleep studies were analyzed by a trained technician using the recommendations of the American Academy of Sleep Medicine (AASM) (25). The severity classification of OSAS was based on the apnea/hypopnea index (AHI): mild (5 < AHI < 15), moderate (15 < AHI < 30), and severe (AHI > 30). According with current clinical recommendations (22), patients with moderate or severe OSAS were prescribed with nocturnal CPAP with an automated device (ResMed AirSenseTM10 AutoSet/Elite- AutoSet TM CS-A). Moreover, all patients received counseling on sleep hygiene. CPAP has been titrated for three nights. The definitive value of CPAP is the amount of pressure that eliminates events in ~95% of the total sleep time (95th centile). Patients have been assessed monthly for compliance and effectiveness of the intervention. Compliance to the CPAP was measured using the data from the CPAP card; a patient was considered to be compliant when using CPAP at least 4 h/night (average) in at least 70% of nights. CPAP was considered effective if the AHI falled below 5/h.
Blood Pressure Measurement
Blood pressure (BP) measurements readings of clinic BP were obtained in the non-dominant arm of the seated patient, after 5 min of quiet rest, with a mercury sphygmomanometer. Systolic and diastolic BP was recorded at the first appearance (phase I) and disappearance (phase V) of Korotkoff sounds. Baseline BP values were the average of the last two of the three consecutive measurements obtained at intervals of 3 min (26).
Arterial Stiffness
These measurements were obtained by a validated system (SphygmocorTM; AtCor Medical, Sydney, Australia) that employs high-fidelity applanation tonometry (Millar) and appropriate computer software for the analysis of pressure wave (SphygmocorTM). Aortic pulse wave velocity (PWV) was determined from carotid and femoral pressure wave forms. Carotid to femoral transit time (DT) was computed from the foot-to-foot time difference between carotid and femoral waveforms. The distance between the surface markings of the sternal notch and femoral artery was used to estimate the path length between the carotid and femoral arteries (L), and PWV computed as L/DT.
Statistical Analysis
The analysis was performed on 165 subjects, and results were expressed as mean ± SD or as percent frequency. Comparisons between groups were made by paired or unpaired Student t-test or χ2 test as appropriate. Patients were divided in two groups: OSAS + (AHI>15) and OSAS– (AHI < 5). Linear regression analysis was used to assess the relationship between baseline e-GFR and different covariates in all population and in the groups separately. Thus, the same analysis was performed in the OSAS+ to evaluate the major determinant of the e-GFR variation (Δe-GFR), before and after 6 months of CPAP, considering the variation (Δ) of the examined covariates. After this, a multivariate regression analysis was performed to determinate the independent predictor of the Δe-GFR. A value of P < 0.05 was considered statistically significant. All calculations were made with a standard statistical package (SPSS for Windows version 16.0).
Results
From an initial cohort of 198 obese subjects, 33 were excluded because they were diagnosed with mild OSAS (5 < AHI <15); baseline anthropometric, clinical and humoral characteristics of these patients are described in the Supplementary Material. The remaining 165 patients were divided into two groups (OSAS– n = 100, and OSAS+ n = 65) on the basis of CRM results. Baseline anthropometric, clinical, and humoral characteristics of the whole study population and of the two groups separately are listed in Table 1. There were no significant differences between the two groups with respect to mean age, gender, BMI, waist, DBP, total and LDL-cholesterol. As expected, patients with OSAS showed significantly increased values of AHI, oxygen desaturation index (ODI), cumulative sleep time percentage with oxyhemoglobin saturation <90% (TC90), and NC; furthermore, OSAS+ patients also showed higher values of SBP, hs-CRP, UA, triglycerides, fasting plasma glucose, 2 h-postload plasma glucose, HOMA and PWV, while IGF-1 and HDL-cholesterol were significantly lower in this group. Of interest, OSAS+ patients showed significantly higher values of microalbuminuria (Table 1) and significantly lower e-GFR values (84.0 ± 13.1 vs. 114.5 ± 12.8 mg/dl/1.73 m2, P < 0.0001) compared with OSAS– group (Figure 1).
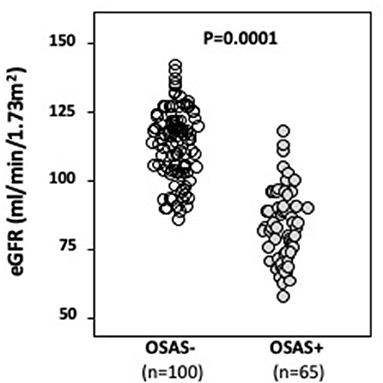
Figure 1. Mean e-GFR baseline values in OSAS– and OSAS+ groups. In this figure, we graphically reported mean baseline e-GFR values of both OSAS– and OSAS+ groups. As indicated, OSAS+ subjects showed statistically significant lower baseline e-GFR values when compared to OSAS– subjects.
At linear regression analysis (Table 2) e-GFR was significantly and inversely associated with AHI (r = −0.667, P < 0.0001), ODI (−0.510, P < 0.0001), TC90 (−0.639, P < 0.0001), microalbuminuria (−0.457, P < 0.0001), SBP (−0.225, P = 0.002), UA (r = −0.208, P = 0.004), hs-CRP (r = −0.197, P = 0.006), triglycerides (r = −0.150, P = 0.027), while it was directly associated with HDL-cholesterol (r = 0.147, P = 0.029) and IGF-1 (r = 0.200, P = 0.005) in the whole study population. In OSAS– group e-GFR was significantly related to hs-CRP (r = 0.292, P = 0.002) and DBP (r = 0.189, P = 0.030), while it was inversely related to AHI (r = −0.297, P < 0.001). In OSAS+ patients, e-GFR was significantly and inversely related only with AHI (r = −0.319, P = 0.005) (Figure 2) and microalbuminuria (r = −0.217, P = 0.041).
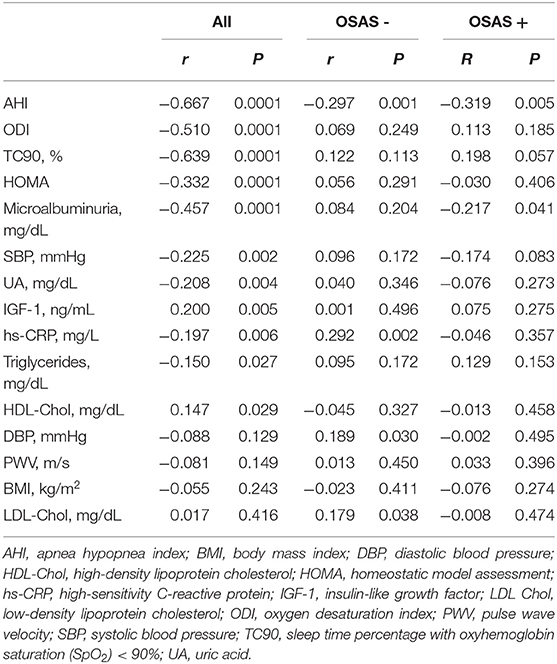
Table 2. Univariate relationship between e-GFR and different covariates in the whole study population and in the two groups separately.
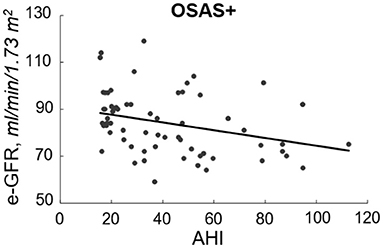
Figure 2. Inverse relationship between e-GFR and AHI in OSAS+ group. In this figure, we reported the inverse relationship between e-GFR and AHI in subjects with OSAS.
Table 3 shows the clinical and biochemical characteristics of OSAS+ patients at baseline and after CPAP therapy. After 6 months, these subjects presented significantly lower values of BMI, waist, AHI, ODI, TC90, hs-CRP, UA, microalbuminuria, HOMA and PWV. Of interest, we observed a significant increase of e-GFR values (104.2 ± 19.0 vs. 84.0 ± 13.1 ml/min/1.73 m2, P < 0.0001) after 6 months of CPAP therapy (Figure 3); in two subjects in the OSAS+ group we detected an almost doubled value of e-GFR (from 80 to 160 ml/min/1.73 m2) at the end of the follow-up period, to be intended as a hyperfiltration due to a weight gain >5% from the basal value. Subsequently, in the same group, a linear regression analysis was performed between Δe-GFR, as dependent variable, Δ of different covariates and baseline e-GFR. As shown in Table 4, Δe-GFR was significantly and inversely correlated with ΔAHI (r = −0.482, P < 0.0001) and e-GFR at baseline (r = −0.543, P = 0.0001). Then, we performed a multiple regression analysis (Table 5) including (Model A) and excluding (Model B) baseline e-GFR from the model. We observed that Δe-GFR was influenced by e-GFR at baseline, that explained 28.4% of its variation, and by ΔAHI that determined another 9.5% of its variation. In the second model, ΔAHI resulted the main independent predictor of Δe-GFR explaining 22% of its variation, followed by ΔTC90 that explained another 5.1% of Δe-GFR variation.
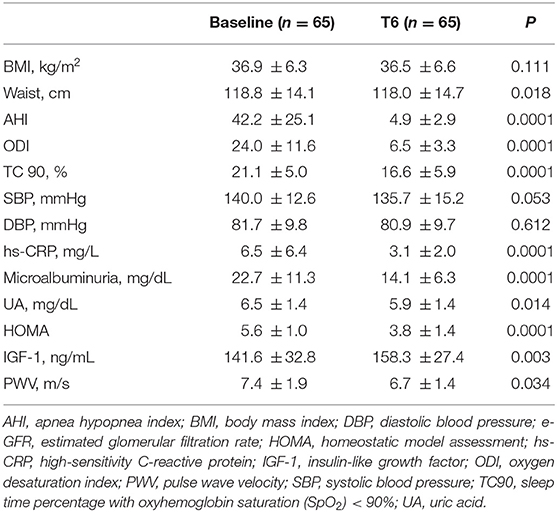
Table 3. Anthropometric, hemodynamic and humoral characteristics of OSAS+ patients at baseline and after 6 months of CPAP therapy.
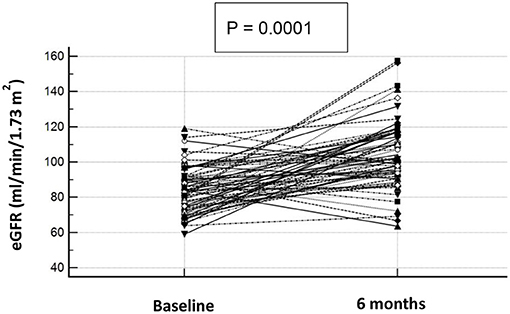
Figure 3. e-GFR baseline and after 6 months of CPAP therapy in OSAS+ subjects. Graphical representation of e-GFR variation after 6 months of CPAP therapy in OSAS+ subjects. Each dot represents a single subject.
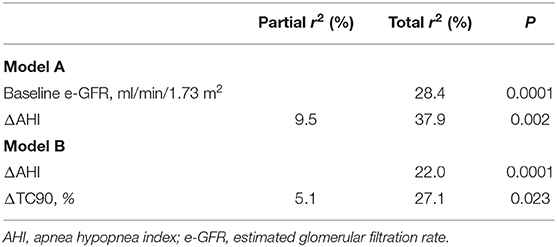
Table 5. Multiple linear regression models for Δe-GFR with (model A) and without (model B) baseline e-GFR.
Discussion
The most important finding obtained in this study, conducted in a population of obese patients with normal renal function, is that those with moderate-to-severe OSAS showed lower—even if in the normal range—baseline e-GFR values compared to subjects without OSAS; moreover, 6 months treatment with CPAP was effective in improve e-GFR by 20 ml/min/1.73 m2. Clinically relevant, ΔAHI resulted the most important independent predictor of Δe-GFR, allowing to affirm that the improvement of nocturnal respiratory function is the main determinant of e-GFR increase in this particular setting of patients. In fact, ΔAHI -or the resolution of apneas during CPAP-, and ΔTC90 -or the improvement of oxyhemoglobin saturation during sleep-, represent the main predictors of Δe-GFR, independently from metabolic, hormonal, and inflammatory status. These findings are consistent with other published literature, which identifies the resolution of chronic intermittent hypoxic episodes during sleep as the major pathophysiological mechanism of the improvement of endothelial glomerular function (10, 27, 28). Our data are in agreement with previous literature but it appears evident that there are other pathophysiological OSAS-related mechanisms responsible of renal function impairment in this setting of patients. Our hypothesis is that arterial stiffness, in addition to the inflammatory status characterizing obese patients, may negatively influence intraglomerular hemodynamic. In fact, arterial stiffness represents an independent predictor of cardiovascular, cerebral and renal morbidity (29); mineral metabolism disturbances, vascular calcifications, formation of advanced glycation end-products, and acute and chronic volume overload, occurring in ESRD and CKD patients, have been proposed as the main pathogenetic mechanisms linking arterial stiffness to renal disease (30). In our study population, there were not significant differences in PWV (a surrogate marker of arterial stiffness) between OSAS– and OSAS+ patients, despite significantly higher SBP values in the last group. Of clinical importance, PWV was significantly lower after 6 months of CPAP therapy, leading to hypothesize a positive impact of reduced arterial stiffness on renal hemodynamic. Moreover, it is well-established that CPAP therapy reduces CV risk in OSAS patients (19, 31) through an improvement in the insulin-resistant status. In addition, in a group of subjects affected by OSAS and CKD stage III-IV, CPAP therapy was effective in reducing proteinuria and increasing e-GFR after a year of treatment (32). Other studies have demonstrated that CPAP therapy reduces glomerular hyperfiltration with a consequent increase of renal plasma flow in obese OSAS patients (33). With this regard, it is important to remark that also cigarette smoking negatively impacts on renal function (34, 35); in our study population, in the OSAS+ group we observed a greater prevalence of current smokers than in the OSAS– subjects, contributing to the impairment of e-GFR in these subjects.
The novelty of our study is that, differently from other Authors, we considered a group of obese subjects with preserved renal function. Many studies describe a major prevalence of breathing sleep disorders in subjects affected by ESRD in comparison with those with normal renal function (36, 37). On the other hand, in the last few years, some Authors focused their attention on the prevalence of OSAS in the first stages of renal dysfunction, even if the majority of the studies is based on patients' history collection and not on sleep instrumental study (36). It is well-known that obesity, a risk factor of OSAS, is associated with glomerular hyperfiltration but, on the contrary, very little is known about the impact of breathing sleep disorders on renal hemodynamic in these subjects (20, 21, 37–39).
In conclusion, even if this study has several limitations such as the single-center non-blinded design and the small sample size, our data confirm previously published literature about the detrimental effect of OSAS on renal function also in subjects free from kidney disease; this detrimental effect may be reverted, at least in part, by CPAP therapy.
Data Availability Statement
The raw data supporting the conclusions of this article will be made available by the authors, without undue reservation.
Ethics Statement
The studies involving human participants were reviewed and approved by Comitato Etico Calabria Centro. The patients/participants provided their written informed consent to participate in this study.
Author Contributions
MP, FP, and RM: Conceptualization; PS and AS: methodology; RM and PS: software; MP, GS, and FP: validation; RM: formal analysis; PS, BC, LM, MV, and ES: investigation; MP, RM, and PS: writing—original draft preparation; MP and FP: writing—review and editing; MP, AS, and FP: supervision. All authors have read and agreed to the published version of the manuscript.
Conflict of Interest
The authors declare that the research was conducted in the absence of any commercial or financial relationships that could be construed as a potential conflict of interest.
Supplementary Material
The Supplementary Material for this article can be found online at: https://www.frontiersin.org/articles/10.3389/fmed.2021.642086/full#supplementary-material
References
1. Gilat H, Vinker S, Buda I, Soudry E, Shani M, Bachar G. Obstructive sleep apnea and cardiovascular comorbidities: a large epidemiologic study. Medicine (Baltimore). (2014) 93:9. doi: 10.1097/MD.0000000000000045
2. Foster GE, Poulin MJ, Hanly PJ. Intermittent hypoxia and vascular function: implications for obstructive sleep apnoea. Exp Physiol. (2007) 92:51–65. doi: 10.1113/expphysiol.2006.035204
3. Suzuki YJ, Jain V, Park AM. Oxidative stress and oxidant signaling in obstructive sleep apnea and associated cardiovascular diseases. Free Radic Biol Med. (2006) 40:1683–92. doi: 10.1016/j.freeradbiomed.2006.01.008
4. Javaheri S, Barbe F, Campos-Rodriguez F, Dempsey JA, Khayat R, Javaheri S, et al. Sleep apnea: types, mechanisms, and clinical cardiovascular consequences. J Am Coll Cardiol. (2017) 69:841–58. doi: 10.1016/j.jacc.2016.11.069
5. Narkiewicz K, Somers VK. The sympathetic nervous system and obstructive sleep apnea: implications for hypertension. J Hypertens. (1997) 15(12 Pt 2):1613–19. doi: 10.1097/00004872-199715120-00062
6. Bisogni V, Pengo MF, Maiolino G. The sympathetic nervous system and catecholamines metabolism in obstructive sleep apnoea. J Thorac Dis. (2016) 8:243–54. doi: 10.3978/j.issn.2072-1439.2015.11.14
7. Manjunath G, Tighiouart H, Ibrahim H, MacLeod B, Salem DN, Griffith JL, et al. Level of kidney function as a risk factor for atherosclerotic cardiovascular outcomes in the community. J Am Coll Cardiol. (2003) 41:47–55. doi: 10.1016/S0735-1097(02)02663-3
8. Parikh NI, Hwang SJ, Larson MG, Meigs JB, Levy D, Fox CS. Cardiovascular disease risk factors in chronic kidney disease: overall burden and rates of treatment and control. Arch Intern Med. (2006) 166:1884−91. doi: 10.1001/archinte.166.17.1884
9. Go AS, Chertow GM, Fan D. Chronic kidney disease and the risks of death, cardiovascular events, and hospitalization. N Engl J Med. (2004) 351:1296 −305. doi: 10.1056/NEJMoa041031
10. Kanbay A, Buyukoglan H, Ozdogan N, Kaya E, Oymak FS, Gulmez I, et al. Obstructive sleep apnea syndrome is related to the progression of chronic kidney disease. Int Urol Nephrol. (2012) 44:535–9. doi: 10.1007/s11255-011-9927-8
11. Ruilope LM, Salvetti A, Jamerson K, Hansson L, Warnold I, Wedel H, et al. Renal function and intensive lowering of blood pressure in hypertensive participants of the hypertension optimal treatment (HOT) study. J Am Soc Nephrol. (2001) 12:218–25.
12. Anavekar NS, McMurray JJV, Velazquez EJ, Solomon SD, Kober L, Rouleau JL, et al. Relation between renal dysfunction and cardiovascular outcomes after myocardial infarction. N Engl J Med. (2004) 351:1285–95. doi: 10.1056/NEJMoa041365
13. Unruh ML. Sleep apnea and dialysis therapies: things that go bump in the night? Hemodial Int. (2007) 11:369–78. doi: 10.1111/j.1542-4758.2007.00203.x
14. Sim JJ, Rasgon SA, Kujubu DA, Kumar VA, Liu ILA, Shi JM, et al. Sleep apnea in early and advanced chronic kidney disease: Kaiser Permanente Southern California cohort. Chest. (2009) 135:710–6 doi: 10.1378/chest.08-2248
15. Lin CH, Lurie RC, Lyons OD. Sleep apnea and chronic kidney disease: a state-of-the-art review. Chest. (2020) 157:673–85. doi: 10.1016/j.chest.2019.09.004
16. Faulx MD, Storfer-Isser A, Kirchner HL. Obstructive sleep apnea is associated with increased urinary albumin excretion. Sleep. (2007) 30:923–29. doi: 10.1093/sleep/30.7.923
17. Kramer H, Luke A, Bidani A. Obesity and prevalent and incident CKD: the hypertension detection and follow-up program. Am J Kidney Dis. (2005) 46:587–94. doi: 10.1053/j.ajkd.2005.06.007
18. Hsu CY, McCulloch CE, Iribarren C. Body mass index and risk for end-stage renal disease. Ann Intern Med. (2006) 144:21–8. doi: 10.7326/0003-4819-144-1-200601030-00006
19. Buchner NJ, Sanner BM, Borgel J. Continuous positive airway pressure treatment of mild to moderate obstructive sleep apnea reduces cardiovascular risk. Am J Respir Crit Care Med. (2007) 176:1274–80. doi: 10.1164/rccm.200611-1588OC
20. Rimke AN, Ahmed SB, Turin TC, Pendharkar SR, Raneri JK, Lynch EJ, et al. Effect of CPAP therapy on kidney function in patients with chronic kidney disease: a pilot randomized controlled trial. Chest. (2020) S0012–3692(20)35354–X. doi: 10.1016/j.chest.2020.11.052
21. Koga S, Ikeda S, Yasunaga T, Nakata T, Maemura K. Effects of nasal continuous positive airway pressure on the glomerular filtration rate in patients with obstructive sleep apnea syndrome. Intern Med. (2013) 52:345–9. doi: 10.2169/internalmedicine.52.8468
22. Epstein LJ, Kristo D, Strollo PJ. Adult obstructive sleep apnea task force of the American academy of sleep medicine. Clinical guideline for the evaluation, management and long-term care of obstructive sleep apnea in adults. J Clin Sleep Med. (2009) 5:263–76. doi: 10.5664/jcsm.27497
23. Matthews DR, Hosker JP, Rudenski AS. Homeostasis model assessment: insulin resistance and beta-cell function from fasting plasma glucose and insulin concentrations in man. Diabetologia. (1985) 28:412–19. doi: 10.1007/BF00280883
24. Levey AS, Stevens LA, Schmid CH, Zhang YL, Castro AF III, Feldman HI, et al. A new equation to estimate glomerular filtration rate. Ann Intern Med. (2009) 150:604–12. doi: 10.7326/0003-4819-150-9-200905050-00006
25. Kapur VK, Auckley DH, Chowdhuri S, Kuhlmann DC, Mehra R, Ramar K, et al. Clinical practice guideline for diagnostic testing for adult obstructive sleep apnea: an American Academy of sleep medicine clinical practice guideline. J Clin Sleep Med. (2017) 13:479–504. doi: 10.5664/jcsm.6506
26. Mancia G, Fagard R, Narkiewicz K, Redón J, Zanchetti A, Böhm M, et al. 2013 ESH/ESC Guidelines for the management of arterial hypertension: the task force for the management of arterial hypertension of the European Society of Hypertension (ESH) and of the European Society of Cardiology (ESC). J Hypertens. (2013) 31:1281–357. doi: 10.1097/01.hjh.0000431740.32696.cc
27. Lavie L. Oxidative stress-a unifying paradigm in obstructive sleep apnea and comorbidities. Prog Cardiovasc Dis. (2009) 51:303–12. doi: 10.1016/j.pcad.2008.08.003
28. Yamauchi M, Kimura H. Oxidative stress in obstructive sleep apnea: putative pathways to the cardiovascular complications. Antioxid Redox Signal. (2008) 10:755–68. doi: 10.1089/ars.2007.1946
29. Sciacqua A, Maio R, Miceli S, Pascale A, Carullo G, Grillo N, et al. Association between one-hour post-load plasma glucose levels and vascular stiffness in essential hypertension. PLoS One. (2012) 7:e44470. doi: 10.1371/journal.pone.0044470
30. Georgianos PI, Pikilidou MI, Liakopoulos V, Balaskas EV, Zebekakis PE. Arterial stiffness in end-stage renal disease- pathogenesis, clinical epidemiology, and therapeutic potentials. Hypertens Res. (2018) 41:309–19. doi: 10.1038/s41440-018-0025-5
31. Marin JM, Carrizo SJ, Vicente E. Long-term cardiovascular outcomes in men with obstructive sleep apnoea-hypopnoea with or without treatment with continuous positive airway pressure: an observational study. Lancet. (2005) 365:1046–53. doi: 10.1016/S0140-6736(05)71141-7
32. Puckrin R, Iqbal S, Zidulka A. Renoprotective effects of continuous positive airway pressure in chronic kidney disease patients with sleep apnea. Int Urol Nephrol. (2015) 47:1839–45. doi: 10.1007/s11255-015-1113-y
33. Hammer Y, Cohen E, Levi A, Krause I. The relationship between cigarette smoking and renal function: a large cohort study. Isr Med Assoc J. (2016) 18:553–6.
34. Huang F, Chen J, Liu X, Cai Q, Peng G, Zhang K, et al. Cigarette smoking reduced renal function deterioration in hypertensive patients may be mediated by elevated homocysteine. Oncotarget. (2016) 7:86000–10. doi: 10.18632/oncotarget.13308
35. Kinebuchi S, Kazama JJ, Satoh M, Sakai K, Nakayama H, Yoshizawa H, et al. Short-term use of continuous positive airway pressure ameliorates glomerular hyperfiltration in patients with obstructive sleep apnoea syndrome. Clin Sci (Lond). (2004) 107:317–22. doi: 10.1042/CS20040074
36. Benz RL, Pressman MR, Hovick ET. Potential novel predictors of mortality in end-stage renal disease patients with sleep disorders. Am J Kidney Dis. (2000) 35:1052–60. doi: 10.1016/S0272-6386(00)70039-4
37. Hanly P. Sleep apnea and daytime sleepiness in end-stage renal disease. Semin Dial. (2004) 17:109–14. doi: 10.1111/j.0894-0959.2004.17206.x
38. Turek NF, Ricardo AC, Lash JP. Sleep Disturbances as nontraditional risk factors for development and progression of CKD: review of the evidence. Am J Kidney Dis. (2012) 60:823–33. doi: 10.1053/j.ajkd.2012.04.027
Keywords: chronic kidney disease, renal function, OSAS, CPAP, cardiovascular risk factors
Citation: Perticone M, Maio R, Scarpino PE, Mancuso L, Volpentesta M, Caroleo B, Suraci E, Sciacqua A, Sesti G and Perticone F (2021) Continuous Positive Airway Pressure Improves Renal Function in Obese Patients With Obstructive Sleep Apnea Syndrome. Front. Med. 8:642086. doi: 10.3389/fmed.2021.642086
Received: 15 December 2020; Accepted: 10 February 2021;
Published: 03 March 2021.
Edited by:
Michele Provenzano, University of Catanzaro, ItalyReviewed by:
Corrado Pelaia, University of Catanzaro, ItalyLajos Markó, Charité – Universitätsmedizin Berlin, Germany
Copyright © 2021 Perticone, Maio, Scarpino, Mancuso, Volpentesta, Caroleo, Suraci, Sciacqua, Sesti and Perticone. This is an open-access article distributed under the terms of the Creative Commons Attribution License (CC BY). The use, distribution or reproduction in other forums is permitted, provided the original author(s) and the copyright owner(s) are credited and that the original publication in this journal is cited, in accordance with accepted academic practice. No use, distribution or reproduction is permitted which does not comply with these terms.
*Correspondence: Maria Perticone, bWFyaWFwZXJ0aWNvbmUmI3gwMDA0MDt1bmljei5pdA==
†These authors have contributed equally to this work