- 1Elmezzi Graduate School of Molecular Medicine, Manhasset, NY, United States
- 2Feinstein Institutes for Medical Research, Manhasset, NY, United States
- 3Department of Surgery, North Shore University Hospital, Manhasset, NY, United States
- 4Department of Emergency Medicine, North Shore University Hospital, Manhasset, NY, United States
- 5Donald and Barbara Zucker School of Medicine at Hofstra/Northwell, Hempstead, NY, United States
- 6Department of Radiology, North Shore University Hospital, Manhasset, NY, United States
- 7Department of Medicine, North Shore University Hospital, Manhasset, NY, United States
- 8Department of Pharmacy, North Shore University Hospital, Manhasset, NY, United States
- 9Jacobs School of Medicine and Biomedical Sciences, University at Buffalo, Buffalo, NY, United States
- 10Department of Anesthesiology, North Shore University Hospital, Manhasset, NY, United States
This case series reviews four critically ill patients infected with severe acute respiratory syndrome coronavirus 2 (SARS-CoV-2) [coronavirus disease 2019 (COVID-19)] suffering from pneumatosis intestinalis (PI) during their hospital admission. All patients received the biological agent tocilizumab (TCZ), an interleukin (IL)-6 antagonist, as an experimental treatment for COVID-19 before developing PI. COVID-19 and TCZ have been independently linked to PI risk, yet the cause of this relationship is unknown and under speculation. PI is a rare condition, defined as the presence of gas in the intestinal wall, and although its pathogenesis is poorly understood, intestinal ischemia is one of its causative agents. Based on COVID-19's association with vasculopathic and ischemic insults, and IL-6's protective role in intestinal epithelial ischemia–reperfusion injury, an adverse synergistic association of COVID-19 and TCZ can be proposed in the setting of PI. To our knowledge, this is the first published, single center, case series of pneumatosis intestinalis in COVID-19 patients who received tocilizumab therapy.
Introduction
Pneumatosis intestinalis (PI) is a rare condition (prevalence ~0.03%) defined as the presence of gas in the wall of the small or large intestine (1–3). PI can represent an incidental, benign finding (primary or idiopathic PI, 15% of cases) or a potentially life-threatening gastrointestinal disease (secondary PI, 85% of cases) (4). Secondary PI is frequently associated with chronic obstructive pulmonary disease (COPD) as well as ischemic, necrotic, and obstructive gastrointestinal insults (5, 6). PI is a radiographic sign characterized by linear and/or curvilinear gas collections in the intestinal wall and is often indicative of systemic or local pathological processes affecting the bowel wall (7, 8). Secondary PI is frequently associated with different clinical scenarios, such as premature newborns with necrotizing enterocolitis, adults with obstructive pulmonary diseases as well as ischemic, necrotic, infectious, and obstructive gastrointestinal insults, celiac disease, amyloidosis, AIDS, rheumatic diseases, and certain drugs, particularly steroids, chemotherapeutics, glucosidase inhibitors, laxatives (lactulose), and molecular targeted agents such as tocilizumab (TCZ) (5, 7, 9–13). The clinical manifestations of PI depend on the bowel segments involved. When PI affects the small intestine, vomiting, abdominal distension, weight loss, and abdominal discomfort/pain are the most common manifestations. Less frequently, diarrhea, anorexia, and constipation can be present. When PI involves the large intestine, diarrhea, hematochezia, abdominal discomfort/pain, and distension are the most common signs and symptoms. Less frequently, constipation, weight loss, and tenesmus may occur (1, 14, 15). The pathogenesis of PI is poorly understood; however, clinical and preclinical studies suggest that PI results from a complex combination of abnormal biochemical, microbiological, and mechanical aspects of intestinal functioning (16).
In December 2019, a novel coronavirus [severe acute respiratory syndrome coronavirus 2 (SARS-CoV-2)] was identified in Wuhan, China as the primary cause of a potentially fatal and multisystemic disease [coronavirus disease 2019 (COVID-19)] (17–19). Since then, three meta-analyses reported that ~15% of COVID-19 patients had GI symptoms, most commonly diarrhea (20–22). GI manifestations in COVID-19 have been acknowledged as early signs of severe/critical disease, usually preceding respiratory symptoms (23, 24). How COVID-19 affects the GI tract is still an open question. Nonetheless, a growing body of evidence suggests that the interaction between SARS-CoV-2 and angiotensin-converting enzyme 2 (ACE2) receptors may result in impaired gut microbiome and immunity (24–26). Furthermore, hypoxia, which putatively has a physiological role in intestinal homeostasis, can be altered as a consequence of COVID-19-induced hypoxia (24, 27).
Systematic reviews and other large observational studies have discussed the association of GI complications in patients undergoing TCZ treatment; however, PI after TCZ use has been recognized by a small number of reports (9, 28, 29). Intestinal perforation is particularly an infrequent but feared complication during TCZ therapy. The exact mechanism of TCZ and GI insults is unknown, but interleukin (IL)-6 antagonism, the primary mechanism of action of TCZ, may impair intestinal homeostasis and recovery capacity after intestinal ischemia (9, 30–33). Remarkably, a history of diverticulitis, non-steroidal anti-inflammatory drugs (NSAIDs), and glucocorticoids use has also been recognized as a risk factor for GI perforation during TCZ treatment (30, 34).
Only four independent case reports have described PI in COVID-19 patients (35–38). Importantly, although TCZ is being utilized as an experimental treatment for COVID-19, the two together may have adverse synergistic effects resulting in increased risk for PI and other related complications. This is the first case series presenting the detailed clinical course of four COVID-19 patients who all received TCZ and developed PI.
Case Presentation 1
A 65-year-old man with a past medical history (PMH) of asthma, hypertension (HTN), hyperlipidemia (HLD), insulin resistance, and obstructive sleep apnea (OSA) was admitted to the hospital due to dyspnea, tachypnea [respiratory rate (RR), 22/min], cough, fever (103°F), and hypoxia [blood oxygen saturation levels (SpO2), 81%] after having a 2-week period of fever, chills, and body aches. Symptoms had worsened in the last 72 h before admission. On admission, the patient was placed on a non-rebreather mask (NRB) at 11 L/min (LPM) due to respiratory distress, which partially improved hypoxia from SpO2 81 to 91%. Three days after admission, the respiratory status worsened revealing diffuse bilateral ground-glass opacification on chest CT scan, along with increased counts of neutrophils in plasma, lymphopenia, and transaminitis. Labs showed increased ferritin and C-reactive protein (CRP) levels (Table 1), features compatible with the cytokine release-like syndrome associated with COVID-19 (39, 40). Based on his clinical condition with pending COVID-19 testing results, the patient was started on albuterol, meropenem, hydroxychloroquine, ascorbic acid, thiamine, TCZ, and enoxaparin prophylaxis. One day later, due to decreased oxygen saturation, the patient was placed in pronation. Upon worsening hypoxia, severe dyspnea, positive polymerase chain reaction (PCR) COVID-19 swab testing, and meeting the criteria for acute respiratory distress syndrome (ARDS), the patient was intubated. Furthermore, prophylactic enoxaparin was switched to intravenous (IV) heparin 1,500 U/h, and an IL-1 inhibitor (anakinra) was started. Shortly, a vasoplegic shock refractory to adjustments in sedative medications prompted onset of vasopressors (norepinephrine). Since admission, the patient had no bowel movements despite the use of laxatives (lactulose), prokinetics (metoclopramide), and enemas (saline laxative). Progressive abdominal distension warranted a CT scan demonstrating extensive colon and small bowel pneumatosis with mesenteric and portal venous gas, raising suspicions of bowel ischemia (Figures 1A–D). Based on the patient's clinical and pathological characteristics, lactate levels of 2.0 mmol/L, and no increased vasopressor requirement, surgical resection was not considered the best course of action at the time. Laxative regimen was enhanced with polyethylene glycol and senna. Monitoring of intra-abdominal pressure ranged from 13 to 19 mmHg, which suggested a likely abdominal compartment syndrome (41). Six days after initiation of mechanical ventilation, the patient developed non-oliguric acute kidney injury (AKI) likely secondary to COVID-19 sepsis and acute tubular necrosis (ATN) from hemodynamic instability. The patient was approached in a conservative, non-surgical fashion including switching propofol to ketamine, adjusting IV fluids, using piperacillin–tazobactam for enteric bacteria, and holding potential nephrotoxic agents. A repeated CT scan 12 days after the initial scan showed changes consistent with bowel ischemia, as well as signs suggestive of peritonitis, complicated by bowel perforation, pneumoperitoneum, small bowel obstruction (SBO), enterocutaneous fistulas, and abscess formation. Additionally, the patient developed hematochezia and melena, requiring aggressive resuscitation, including multiple blood and frozen plasma transfusions, as well as repeated drainage procedures to address the intra-abdominal collections. Noteworthy, a CT angiogram ruled out active bleeding at the time of melena and hematochezia. The patient slowly and progressively recovered and was finally discharged for rehabilitation, 90 days after admission. In a delayed fashion, he underwent a right colectomy. Currently, he is alive and well.
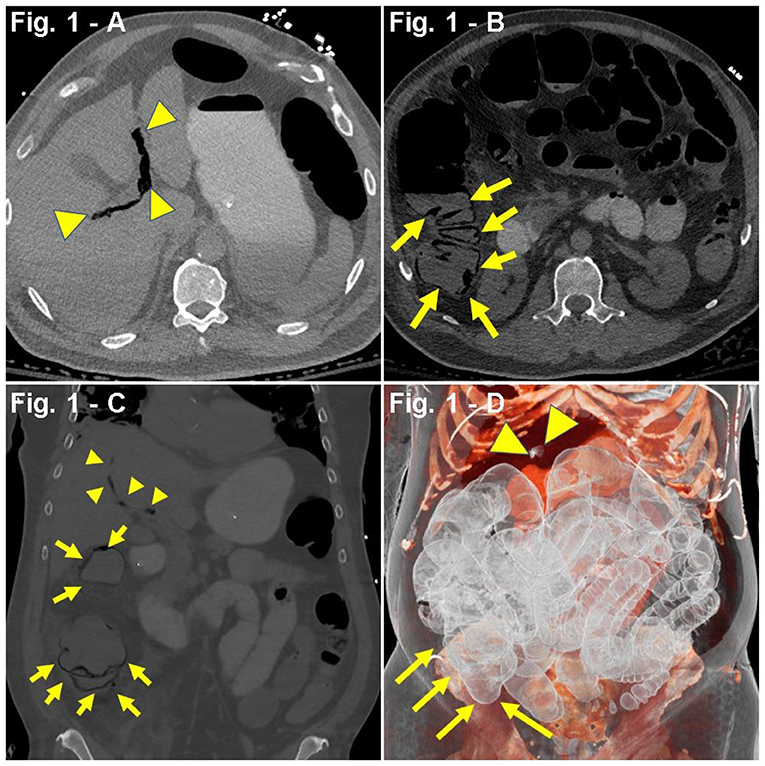
Figure 1. (A–D) Case presentation of 65-year-old male patient with COVID-19, 5 days after tocilizumab (TCZ), non-contrast abdominal CT. (A,B) Axial, (C) coronal, and (D) 3D reconstruction, pneumatosis intestinalis (PI) involving ascending colon (yellow arrows), with dilated multiple right lower quadrant small bowel loops with mesenteric and portal venous gas (yellow arrowheads).
Case Presentation 2
A 61-year-old man with a PMH of HTN, HLD, diabetes mellitus (DM), and OSA was admitted to the hospital after presenting with dyspnea, tachypnea (RR, 40/min), hypoxia (SpO2, 76%), worsening cough (productive, non-bloody), fever (103.9°F), chills, myalgias, hyporexia, and chest pain. Symptoms began 4 weeks prior, and 2 weeks prior to admission, he received a course of azithromycin and oseltamivir. One week prior to admission, the patient visited the ED for myalgias, chills, and cough but was discharged with a normal chest X-ray (CXR). The physical examination during the second ED visit was remarkable for severe respiratory distress and bilateral basilar crackles. His hypoxia initially improved with 6 LPM nasal cannula (NC) from SpO2 of 76–91%, albeit later requiring 6 L NRB (SpO2, 76–95%). CXR revealed bilateral infiltrates, and a CT angiogram with contrast confirmed lung parenchyma compromise with extensive bilateral ground-glass opacities in both lungs. Pulmonary embolism (PE) could not be excluded due to motion associated with the hyperdynamic state. Lab workup revealed positive COVID-19 PCR test through nasal swabbing, lymphopenia, increased D-dimer (453 ng/mL), brain-derived natriuretic peptide (BNP) (1,164 pg/mL), and procalcitonin (0.20 ng/mL) (Table 1). During the first night of admission, the patient became severely hypoxic (PaO2, 51%), with improvement in SpO2 from 80 to 88% after aggressive resuscitation with steroids (IV methylprednisolone, 100 mg), diuretics (IV furosemide, 40 mg), oxygen (15 LPM NRB), and pronation. On second day of admission, the patient was transferred to the ICU, received one dose of TCZ, and was started on IV methylprednisolone, 50 mg twice daily, IV furosemide, 40 mg daily, inhaled albuterol every 6 h, prophylactic enoxaparin, and non-invasive ventilation (BiLevel 18/14). On the second day of ICU admission, the patient received convalescent plasma (plasma from recovered COVID-19 patients). One week after admission, upon worsening hypoxia and hemodynamic instability, the patient underwent endotracheal intubation for mechanical ventilation and was started on a double regimen of vasopressors (vasopressin, 0.04 U/min and norepinephrine, 0.9 mcg/kg/min). The patient suffered two episodes of arterial thrombosis despite proper anticoagulation with argatroban. Five days after initiating mechanical ventilation, physical examination revealed a protuberant abdomen and dark output from the nasogastric tube (NGT). Further workup showed increased lactate and leukocytosis, hyperkalemia (5.8 mEq/L), increased creatinine (2.01 mg/dL), and oliguria. Abdominal CT scan reported gas in the portal vein and superior mesenteric artery, as well as cecal and small bowel pneumatosis (Figures 2A–D). The benefit of a surgical intervention was considered very low in the setting of an unstable patient with multiorgan failure; hence, it was approached conservatively, including antibiotics (metronidazole and vancomycin), proton-pump inhibitors (pantoprazole), renal replacement therapy [continuous veno-venous hemofiltration (CVVH)], fluid optimization, and metabolic support. On the 10th day of ICU admission, the patient developed refractory cardiopulmonary arrest associated with metabolic acidosis and lactate levels of 24 mmol/L.
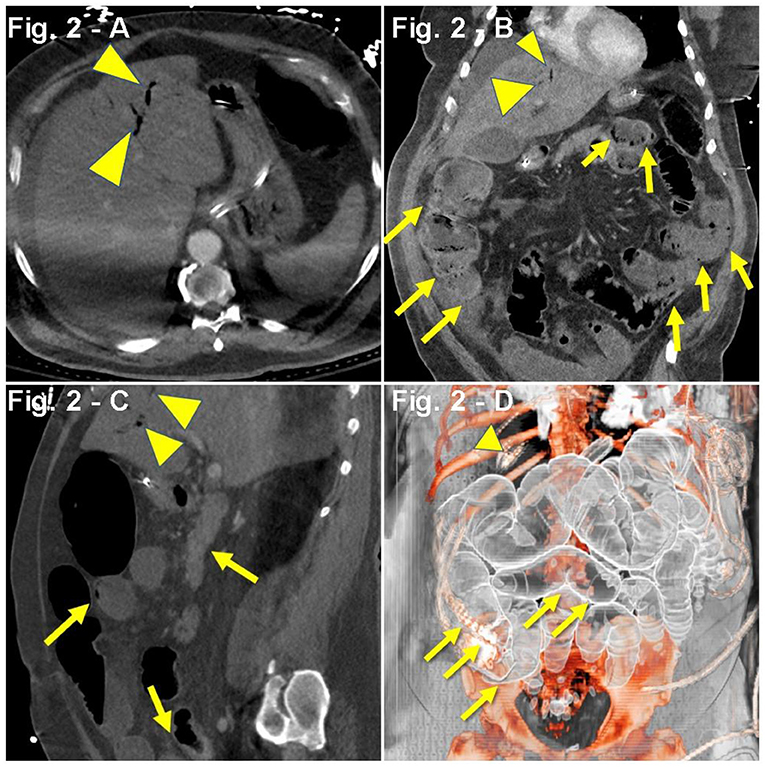
Figure 2. (A–D) Case presentation of a 61-year-old male COVID-19 patient with respiratory failure on TCZ with rising lactate, abdominal ileus, abdominal CT with intravenous and oral contrast, (A) axial, (B) coronal, (C) sagittal, and (D) 3D reconstruction, with ileus and small and large bowel dilatation, with small bowel and cecal pneumatosis (yellow arrows) with portal gas (yellow arrowheads), and splenic and mesenteric vein gas.
Case Presentation 3
A 64-year-old man with a PMH of HTN was admitted for 1 week history of dyspnea, cough, fever (100.9°F), and fatigue. Pulse oximetry revealed hypoxia (SpO2, 85%), which initially improved to SpO2 of 90% with NC at 6 LPM, and later on to SpO2 of 92–94% with 10 LPM NRB. A CXR showed bilateral ground-glass opacities. On the second day of admission, the patient developed non-bloody diarrhea. With pending results from PCR COVID-19 nasal swabbing, a presumptive diagnosis of COVID-19 pneumonia was established, and the patient was started on a regimen of PO hydroxychloroquine 200 mg twice daily, IV methylprednisolone 50 mg twice daily, SC prophylactic enoxaparin 40 mg daily, anakinra (IL-1 inhibitor, SC 100 mg every 6 h), and inhaled albuterol. Lab work revealed neutrophilia, lymphopenia, respiratory alkalosis, hypoalbuminemia, transaminitis, as well as increased d-dimer, CRP, lactate dehydrogenase (LDH), and ferritin (Table 1). Despite initial improvement, 5 days after admission, he presented with a nocturnal crisis of hypoxia (SpO2, ~60%), which improved after pronation. Four hours later, the patient (without any relevant psychiatric/neurological history) developed intermittent episodes of delirium, agitation, and altered mental status, which was treated with haloperidol. Subsequently, due to worsening hypoxia, the patient underwent sedation, endotracheal intubation, and mechanical ventilation. At this point, the patient was transferred to another ICU and, upon arrival, was found to have an unsecure airway, raising concern for potential aspiration. Based on this, the patient was started on a regimen of IV piperacillin–tazobactam and one dose of vancomycin. Nine days after initial hospital admission, the patient developed septic shock and prerenal acute kidney injury, which prompted hemodynamic support with a norepinephrine drip (0.02 mcg/kg/min). Enoxaparin was switched to IV sodium heparin due to a D-dimer of 987 ng/mL. Additionally, the patient received a single dose of TCZ. Three days after TCZ administration, routine physical examination showed abdominal distension and tympanism with digital percussion. An abdominal X-ray revealed features compatible with colonic ileus or pseudo-obstruction. Subsequent CT scan showed diffuse small and large bowel pneumatosis (Figures 3A–D). This was found in the setting of worsening kidney and liver function, increased ventilation requirements, acidosis (pH 7.17), and leukocytosis (35,000 WBC/mL). Due to broad multiorgan failure, the patient was not deemed a good surgical candidate for segmental resection. CXR showed additional bilateral consolidations in the lower lobes, along with worsening respiratory status, suggesting a superimposed pneumonia. At this point, with the diagnosis of septic shock and multiorgan failure, and considering the ominous prognosis, the family decided to prioritize comfort over other aggressive measures. The patient was withdrawn from mechanical ventilation and developed a cardiopulmonary arrest 4 min thereafter.
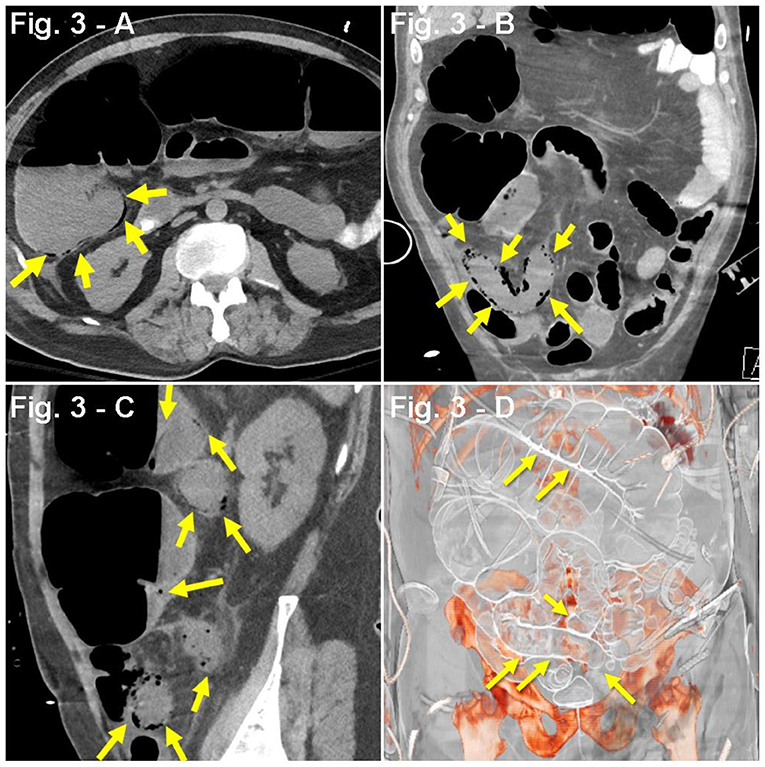
Figure 3. (A–D) Case presentation of a 63-year-old male patient, with dyspnea, cough, fever from COVID-19, with bloody diarrhea, and abdominal distention 3 days after receiving TCZ, abdominal CT with oral contrast only, (A) axial, (B) coronal, (C) sagittal, and (D) 3D reconstruction with consolidation seen along lung bases, and pneumatosis of small bowel loops (yellow arrows) with dilated small and large bowel loops consistent with ileus.
Case Presentation 4
A 64-year-old man with PMH of HTN, insulin-dependent type 2 DM, and stroke 3 years prior with no residual deficits was admitted to an out-of-network hospital due to altered mental status and acute kidney injury (serum creatinine, 2.2 mg/dL). The family reported upper respiratory symptoms 1 week before admission. No acute changes were observed in the patient's head CT scan. Due to concerns of a potential non-ST-segment elevation myocardial infarction (NSTEMI) in the setting of uncontrolled HTN, the patient was started on aspirin, clopidogrel, and a heparin drip. Based on a positive COVID-19 PCR nasal swabbing at admission and concerns for a potential bacterial pneumonia, hydroxychloroquine, remdesivir, ceftriaxone, and doxycycline were initiated. Worsening kidney function parameters (serum creatinine, 2.2–4.2 mg/dL) after antibiotic and antiviral treatment prompted suspension of these medications. One week after admission, the patient received a single dose of TCZ and was started on steroids (IV methylprednisolone, 40 mg every 8 h). By hospital day 10, despite non-invasive ventilation (BiPAP), the progressive worsening of the respiratory status required intubation and mechanical ventilation. One day later, the patient was transferred to our hospital upon family request. On initial assessment, the patient was found to be hypotensive, with oliguria (155 mL/24 h), mixed acidosis (pH 7.04, 17 mEq/L, pCO2 56 mmHg), hyperkalemia (5.6 mEq/L), hyperglycemia (478 mg/dL), hypertriglyceridemia (918 mg/dL), hypoalbuminemia (3.1 g/dL), and uremia [serum creatinine 3.85 mg/dL, blood urea nitrogen (BUN) 150 mg/dL], which prompted continuous renal replacement therapy (Table 1). Intensive resuscitation with albumin, bicarbonate, insulin, norepinephrine, and vancomycin was initiated. Propofol was discontinued and switched to dexmedetomidine. The patient also received IV pantoprazole 40 mg, polyethylene glycol, lactulose, senna, and methylnaltrexone. Subsequently, the patient developed fever (101.4°F), leukocytosis (33.16 × 109/L), and low platelets (85 × 109/L). Due to concerns of potential heparin-induced thrombocytopenia, heparin was suspended and replaced with argatroban. Despite intensive supportive care, vasopressor requirements increased prompting the addition of vasopressin at 0.04 U/min. Leukocytes count and lactate further increased (33.16 × 109/L to 40 × 109/L; 2.7–8.6 mmol/L, respectively). One week after the hospital transfer, abdominal distension on physical exam prompted a CT scan that revealed gas in the portal vein and mesentery as well as extensive intestinal pneumatosis (Figures 4A–D). Surgical assessment dismissed a potential bowel resection since the risks were considered greater than any potential benefit. The patient developed refractory septic shock and, 1 day later, a cardiopulmonary arrest.
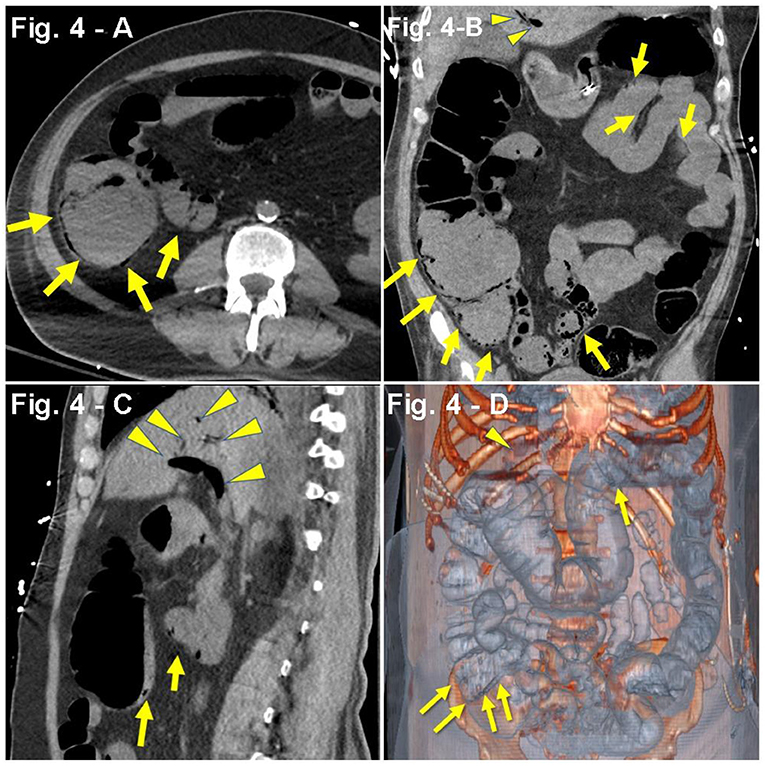
Figure 4. (A–D) Case presentation of a 64-year-old male patient, with altered mental status, acute kidney injury, DM2, stroke, bacterial pneumonia with COVID-19, single-dose TCZ, hypotensive, oliguric, non-contrast abdominal CT only, (A) axial, (B) coronal, (C) sagittal, and (D) 3D reconstruction with pneumatosis of distal transverse colon, cecum, terminal ileum, and mesenteric venous gas adjacent to the terminal ileum (yellow arrows) concerning for bowel ischemia. There are foci of air in mesenteric vessels in the right lower quadrant, with portal venous gas (yellow arrowheads).
Discussion
Pneumatosis intestinalis is thought to result from a complex combination of biochemical, microbiological, and mechanical insults to the intestine (16). The biochemical hypothesis argues that PI originates from excessive hydrogen production by enteric bacteria through chyme fermentation. Support of this hypothesis includes observational studies demonstrating that patients with PI have elevated levels of hydrogen in their breath compared with control patients (42, 43). The microbiological or bacterial hypothesis suggests that gas forming bacteria, such as Clostridium species, infringe upon the mucosa through breaches, reaching the submucosa and subsequently forming intramural gas collections (44). Experimental models supporting this hypothesis include the improvement and resolution of the aforementioned intramural gas collections by antibiotic treatment (45). An alternative mechanical hypothesis states that gas can reach the submucosal compartment of the intestinal wall either through breaks in the mucosa (intraluminal source) or the serosal surface (extraluminal source). Indeed, common conditions associated with mucosal disruption are consistently related with PI, such as necrotizing enterocolitis, inflammatory bowel disease, gastrointestinal (GI) tract infections, irritant ingestion, and intestinal ischemia (1). Gas originating extraluminally can be traced to conditions where air can diffuse through tissues, as occurs in COPD (46).
It should be acknowledged that PI is not a disease itself, but rather the imaging manifestation of an underlying pathology or combination of pathologies. As such, the underlying etiology (e.g., ischemia, drugs, infections) must always be addressed accordingly prior to specific treatments (47–49). When PI is accompanied with signs of peritonitis, pH <7.3, <20 mEq/L, lactate >2.0 mmol/L, and/or portal venous gas, emergent exploratory laparotomy should be considered (50, 51). Treatment is usually based on the severity of symptoms and can range from repeated imaging in asymptomatic patients to elemental diet for mild symptoms, to antibiotics, oxygen therapy, and hospitalization for severe cases. Notably, clinical judgement is an instrumental component of the decision-making process (7, 45, 52–54).
Since March 11th of 2020, when the global COVID-19 pandemic was formally declared, a growing body of evidence has shown the systemic and extrapulmonary compromise that can potentially occur in the setting of COVID-19 (55). The involvement of neurological, cardiovascular, gastrointestinal, hematopoietic, endocrine, and immune systems have been described in many studies (56–59). Additionally, the ischemic damage seen during COVID-19 infection has also been described in several systematic reviews (60–63). Indeed, observational studies have shown the association of COVID-19 with abdominal ischemia; nonetheless, its pathophysiology remains unknown (64). Research has shown a clear association between high levels of proinflammatory cytokines and inflammatory markers with severe, critical, and fatal forms of COVID-19 (40). Under the logic of specifically targeting the COVID-19-associated “cytokine storm”, trials with specific cytokine inhibitors, such as TCZ, sarilumab, siltuximab (all anti-IL-6), and anakinra (anti-IL-1), have been conducted worldwide1. TCZ is an evidence-based treatment for rheumatoid arthritis; however, there are no clear benefits in the setting of COVID-19. At the time of writing, double-blind, randomized, clinical trials have failed to prove any benefit from these drugs in COVID-19 (65, 66). An observational study that included a cohort of 1,351 COVID-19 patients showed that patients receiving TCZ had a decreased risk of invasive mechanical ventilation and death compared to matched controls (67). Another study suggested that TCZ was associated with decreased vasopressor requirements (68). Although PI and gastrointestinal perforation have been recognized as potential adverse effects of TCZ, the mechanisms are completely unknown (9, 30).
Several hypotheses argue in favor of a prothrombotic, microangiopathic, and therefore ischemic effect of COVID-19: (1) SARS-CoV-2 virus can directly invade endothelial cells, resulting in endothelialitis, endothelial dysfunction, and thrombosis (69–72); (2) capillary viscometry showed hyperviscosity in critically ill COVID-19 patients (73); (3) platelet activation and platelet–monocyte aggregation formation in severe COVID-19 patients was documented (74); and (4) thromboelastography (TEG) parameters (decreased R and K values, increased K angle, and MA) consistent with a hypercoagulability state have been found in COVID-19 patients (75).
In short, this case series portrays four critically ill patients who, in the setting of ARDS due to severe COVID-19, received TCZ as an experimental treatment, and all developed complex clinical courses of PI, which subsequently resulted in perforation, sepsis, hemodynamic instability, multiorgan failure, and death in three out of four patients. Herein, we highlight a potential correlation between an infectious disease (COVID-19), an experimental drug in this setting (TCZ), and a rare GI complication (PI). COVID-19 and TCZ have been independently associated with PI (9, 36–38). Intestinal ischemia is a well-established cause of PI; however, the causative mechanism of PI during TCZ treatment is unknown. Preclinical studies suggested that IL-6 plays a critical role in intestinal epithelial proliferation and repair after ischemia–reperfusion, traumatic, and microbiological insults (30, 76). Clinical studies have shown IL-6's pivotal role in vascular endothelial growth factor production, as well as angiogenesis and wound healing (31–33).
Taken together, we venture to think that a possible negative and devastating synergy occurs between the microvascular insults from COVID-19, along with the lack of epithelial protection and vascular support from IL-6 blocking, ultimately resulting in intestinal wall damage, epithelium dysfunction, and intraluminal gas diffusion (Pneumatosis Intestinalis). However, it must be acknowledged that COVID-19 may affect intestinal homeostasis by other complex mechanisms involving gut microbiome and barrier functioning (24). To the best of our knowledge, this is the first case series of PI in COVID-19 patients, as well as the first reported from the same institution, and the first report of COVID-19 patients treated with TCZ who subsequently developed PI.
At the time of writing, COVID-19 is a novel entity with poorly understood pathology. Experimental, emergency, and compassionate use of drugs in COVID-19 has been the object of recent discussions. It should always be cautioned that deleterious interactions between drug-related adverse effects and intrinsic features of an infectious disease, in this case COVID-19, can lead to further complications (77, 78) “Primum non nocere”.
Limitations
Discussions regarding the causal relationship between COVID-19 and TCZ with PI are beyond the scope of this publication. However, it is important to acknowledge that some medications that patients received (methylprednisolone and lactulose) may be linked to PI development (1, 13, 79–81). Furthermore, these four critically-ill patients received intensive care support, which inherently adds numerous variables, including known and unknown interactions between each other and the host, resulting in an uncertain number of potential confounders. Additionally, two patients (Cases 3 and 4) received initial care at an outside hospital, and although the hospital course summary was accessible, the variability of clinical setting may hide unknown confounders. Therefore, it is complex and not possible for the authors to establish any definite cause–effect connection between the described variables (COVID-19 and TCZ) with the highlighted clinical outcome (PI). Further preclinical and clinical research addressing interactions between COVID-19 and TCZ with PI is warranted.
Data Availability Statement
The original contributions presented in the study are included in the article/supplementary material, further inquiries can be directed to the corresponding author.
Ethics Statement
The studies involving human participants were reviewed and approved by Study approved by IRB #: 20-0884, Feinstein Institutes for Medical Research. Written informed consent for participation was not required for this study in accordance with the national legislation and the institutional requirements. Written informed consent was not obtained from the individual(s) for the publication of any potentially identifiable images or data included in this article.
Author Contributions
SM, LB, SG, CK, KH, and EM prepared the manuscript's first draft. SM, LB, and EM retrieved and corroborated the data. CK prepared the figures and edited the manuscript. LS-L, JA, TA, KS, EG, VN, NJ, CM, TY, RT, YC, MS, RC, SW, HH, MN, and SZaf collaborated in the discussion. AM-M, SZan, and CL collaborated in the review. All authors contributed to manuscript revision, read, and approved the submitted version.
Conflict of Interest
The authors declare that the research was conducted in the absence of any commercial or financial relationships that could be construed as a potential conflict of interest.
Footnotes
1. ^Available online at: https://clinicaltrials.gov/ct2/results?cond=&term=tocilizumab+covid-19&cntry=&state=&city=&dist=.
References
1. Pear BL. Pneumatosis intestinalis: a review. Radiology. (1998) 207:13–9. doi: 10.1148/radiology.207.1.9530294
2. Boerner RM, Fried DB, Warshauer DM, Isaacs K. Pneumatosis intestinalis. Digest Dis Sci. (1996) 41:2272–85. doi: 10.1007/BF02071412
3. Kang G. Benign pneumatosis intestinalis: dilemma for primary care clinicians. Can Fam Phys. (2017) 63:766–8.
4. Saul T, Palamidessi N. Pneumatosis intestinalis. J Emerg Med. (2011) 40:545–6. doi: 10.1016/j.jemermed.2008.10.019
5. Doumit M, Saloojee N, Seppala R. Pneumatosis intestinalis in a patient with chronic bronchiectasis. Can J Gastroenterol. (2008) 22:595297. doi: 10.1155/2008/595297
6. Iida A, Naito H, Tsukahara K, Yumoto T, Nosaka N, Kawana S, et al. Pneumatosis cystoides intestinalis presenting as pneumoperitoneum in a patient with chronic obstructive pulmonary disease: a case report. J Med Case Rep. (2017) 11:1–3. doi: 10.1186/s13256-017-1198-2
7. Heng Y, Schuffler MD, Haggitt RC, Rohrmann CA. Pneumatosis intestinalis: a review. Am J Gastroenterol. (1995) 90:1747–58.
8. Khalil PN, Huber-Wagner S, Ladurner R, Kleespies A, Siebeck M, Mutschler W, et al. Natural history, clinical pattern, and surgical considerations of pneumatosis intestinalis. Euro J Med Res. (2009) 14:231–9. doi: 10.1186/2047-783X-14-6-231
9. Jacobs B, Jawad A, Fattah Z. Pneumatosis intestinalis and intestinal perforation in a patient receiving tocilizumab. Arch Rheumatol. (2018) 33:372. doi: 10.5606/ArchRheumatol.2018.6668
10. Dawe N, Akhtar S. Pneumatosis intestinalis presenting with a pneumoperitoneum in a patient with chronic bronchiectasis: a delayed diagnosis of superior mesenteric artery ischaemia. BMJ Case Rep. (2010) 2010:bcr0120102622. doi: 10.1136/bcr.01.2010.2622
11. Dhadlie S, Mehanna D, McCourtney J. Pneumatosis intestinalis a trap for the unwary: case series and literature review. Int J Surg Case Rep. (2018) 53:214–7. doi: 10.1016/j.ijscr.2018.10.079
12. Ezuka A, Kawana K, Nagase H, Takahashi H, Nakajima A. Improvement of pneumatosis cystoides intestinalis after steroid tapering in a patient with bronchial asthma: a case report. J Med Case Rep. (2013) 7:163. doi: 10.1186/1752-1947-7-163
13. Goodman RA, Riley TR 3rd. Lactulose-induced pneumatosis intestinalis and pneumoperitoneum. Dig Dis Sci. (2001) 46:2549–53. doi: 10.1023/a:1012308911096
14. Wu LL, Yang YS, Dou Y, Liu QS. A systematic analysis of pneumatosis cystoids intestinalis. World J Gastroenterol. (2013) 19:4973. doi: 10.3748/wjg.v19.i30.4973
15. Jamart J. Pneumatosis cystoides intestinalis. A statistical study of 919 cases. Acta Hepato Gastroenterol. (1979) 26:419–22.
16. Blair HA, Baker R, Albazaz R. Pneumatosis intestinalis an increasingly common radiological finding, benign or life-threatening? A case series. Case Rep. (2015) 2015:bcr2014207234. doi: 10.1136/bcr-2014-207234
17. World Health Organization. WHO Director-General's Remarks at the Media Briefing on 2019-nCoV on 11 February 2020. Geneva: World Health Organization. Available online at: https://www.who.int/dg/speeches/detail/who-director-general-s-remarks-at-the-media-briefing-on-2019-ncov-on-11-february-2020 (accessed April 10, 2020).
18. Temgoua MN, Endomba FT, Nkeck JR, Kenfack GU, Tochie JN, Essouma M. Coronavirus disease 2019 (COVID-19) as a multi-systemic disease and its impact in low- and middle-income countries (LMICs). SN Compr Clin Med. (2020). doi: 10.1007/s42399-020-00417-7. [Epub ahead of print].
19. World Health Organization. Pneumonia of Unknown Cause—China. Available online at: https://www.who.int/csr/don/05-january-2020-17pneumonia-of-unknown-cause-china/en/ (accessed January 5, 2020).
20. Cheung KS, Hung IF, Chan PP, Lung KC, Tso E, Liu R, et al. Gastrointestinal manifestations of SARS-CoV-2 infection and virus load in fecal samples from the Hong Kong cohort and systematic review and meta-analysis. Gastroenterology. (2020) 159:81–95. doi: 10.1053/j.gastro.2020.03.065
21. Sultan S, Altayar O, Siddique SM, Davitkov P, Feuerstein JD, Lim JK, et al. AGA Institute rapid review of the gastrointestinal and liver manifestations of COVID-19, meta-analysis of international data, and recommendations for the consultative management of patients with COVID-19. Gastroenterology. (2020) 159:320–34. doi: 10.1053/j.gastro.2020.05.001
22. Mao R, Qiu Y, He JS, Tan JY, Li XH, Liang J, et al. Manifestations and prognosis of gastrointestinal and liver involvement in patients with COVID-19: a systematic review and meta-analysis. Lancet Gastroenterol Hepatol. (2020) 5:667–78. doi: 10.1016/S2468-1253(20)30126-6
23. Jin X, Lian JS, Hu JH, Gao J, Zheng L, Zhang YM, et al. Epidemiological, clinical and virological characteristics of 74 cases of coronavirus-infected disease 2019 (COVID-19) with gastrointestinal symptoms. Gut. (2020) 69:1002–9. doi: 10.1136/gutjnl-2020-320926
24. Trottein F, Sokol H. Potential causes and consequences of gastrointestinal disorders during a SARS-CoV-2 infection. Cell Rep. (2020) 32:107915. doi: 10.1016/j.celrep.2020.107915
25. Liang W, Feng Z, Rao S, Xiao C, Xue X, Lin Z, et al. Diarrhoea may be underestimated: a missing link in 2019 novel coronavirus. Gut. (2020) 69:1141–3. doi: 10.1136/gutjnl-2020-320832
26. Gu S, Chen Y, Wu Z, Chen Y, Gao H, Lv L, et al. Alterations of the gut microbiota in patients with COVID-19 or H1N1 influenza. Clin Infect Dis. (2020) 71:2669–78. doi: 10.1093/cid/ciaa709
27. Singhal R, Shah YM. Oxygen battle in the gut: hypoxia and hypoxia-inducible factors in metabolic and inflammatory responses in the intestine. J Biol Chem. (2020) 295:10493–505. doi: 10.1074/jbc.REV120.011188
28. Gout T, Östör AJ, Nisar MK. Lower gastrointestinal perforation in rheumatoid arthritis patients treated with conventional DMARDs or tocilizumab: a systematic literature review. Clin Rheumatol. (2011) 30:1471. doi: 10.1007/s10067-011-1827-x
29. Strangfeld A, Richter A, Siegmund B, Herzer P, Rockwitz K, Demary W, et al. Risk for lower intestinal perforations in patients with rheumatoid arthritis treated with tocilizumab in comparison to treatment with other biologic or conventional synthetic DMARDs. Ann Rheumatic Dis. (2017) 76:504–10. doi: 10.1136/annrheumdis-2016-209773
30. Kuhn KA, Manieri NA, Liu TC, Stappenbeck TS. IL-6 stimulates intestinal epithelial proliferation and repair after injury. PLoS ONE. (2014) 9:e114195. doi: 10.1371/journal.pone.0114195
31. Nakahara H, Song J, Sugimoto M, Hagihara K, Kishimoto T, Yoshizaki K, et al. Anti–interleukin-6 receptor antibody therapy reduces vascular endothelial growth factor production in rheumatoid arthritis. Arthritis Rheumatism. (2003) 48:1521–9. doi: 10.1002/art.11143
32. Verheul HM, Pinedo HM. Possible molecular mechanisms involved in the toxicity of angiogenesis inhibition. Nat Rev Cancer. (2007) 7:475–85. doi: 10.1038/nrc2152
33. Pawar A, Desai RJ, Solomon DH, Ortiz AJ, Gale S, Bao M, et al. Risk of serious infections in tocilizumab versus other biologic drugs in patients with rheumatoid arthritis: a multidatabase cohort study. Ann Rheumatic Dis. (2019) 78:456–64. doi: 10.1136/annrheumdis-2018-214367
34. Malaviya AP, Ledingham J, Bloxham J, Bosworth A, Buch M, Choy E, et al. The 2013 BSR and BHPR guideline for the use of intravenous tocilizumab in the treatment of adult patients with rheumatoid arthritis. Rheumatology. (2014) 53:1344–6. doi: 10.1093/rheumatology/keu168
35. Aiello P, Johnson S, Mercado AR, Hussein S. Pneumatosis intestinalis in a patient with COVID-19. BMJ Case Reports CP. (2020) 13:e237564. doi: 10.1136/bcr-2020-237564
36. Meini S, Zini C, Passaleva MT, Frullini A, Fusco F, Carpi R, et al. Pneumatosis intestinalis in COVID-19. BMJ Open Gastroenterol. (2020) 7:e000434. doi: 10.1136/bmjgast-2020-000434
37. Lakshmanan S, Toubia N. Pneumatosis intestinalis in COVID-19. Clin Gastroenterol Hepatol. (2020) 7:e000434. doi: 10.1016/j.cgh.2020.05.048
38. Kielty J, Duggan WP, O'Dwyer M. Extensive pneumatosis intestinalis and portal venous gas mimicking mesenteric ischaemia in a patient with SARS-CoV-2. Ann R Coll Surg Engl. (2020) 102:e145–7. doi: 10.1308/rcsann.2020.0145
39. Moore JB, June CH. Cytokine release syndrome in severe COVID-19. Science. (2020) 368:473–4. doi: 10.1126/science.abb8925
40. Mehta P, McAuley DF, Brown M, Sanchez E, Tattersall RS, Manson JJ, et al. COVID-19: consider cytokine storm syndromes and immunosuppression. Lancet. (2020) 395:1033. doi: 10.1016/S0140-6736(20)30628-0
41. De Laet IE, Malbrain ML, De Waele JJ. A clinician's guide to management of intra-abdominal hypertension and abdominal compartment syndrome in critically ill patients. Crit Care. (2020) 24:1–9. doi: 10.1186/s13054-020-2782-1
42. Read NW, Al-Janabi MN, Cann PA. Is raised breath hydrogen related to the pathogenesis of pneumatosis coli?. Gut. (1984) 25:839–45. doi: 10.1136/gut.25.8.839
43. Christl SU, Gibson GR, Murgatroyd PR, Scheppach W, Cummings JH. Impaired hydrogen metabolism in pneumatosis cystoides intestinalis. Gastroenterology. (1993) 104:392–7. doi: 10.1016/0016-5085(93)90406-3
44. Yale CE, Balish E, Wu JP. The bacterial etiology of pneumatosis cystoides intestinalis. Arch Surg. (1974) 109:89–94. doi: 10.1001/archsurg.1974.01360010067017
45. Ellis BW. Symptomatic treatment of primary pneumatosis coli with metronidazole. Br Med J. (1980) 280:763. doi: 10.1136/bmj.280.6216.763-a
46. Keyting WS, McCarver RR, Kovarik JL, Daywitt AL. Pneumatosis intestinalis: a new concept. Radiology. (1961) 76:733–41. doi: 10.1148/76.5.733
47. Shinagare AB, Howard SA, Krajewski KM, Zukotynski KA, Jagannathan JP, Ramaiya NH. Pneumatosis intestinalis and bowel perforation associated with molecular targeted therapy: an emerging problem and the role of radiologists in its management. Am J Roentgenol. (2012) 199:1259–65. doi: 10.2214/AJR.12.8782
48. Poor A, Braman SS. Pneumatosis intestinalis associated with the tyrosine kinase inhibitor nintedanib. Lung. (2018) 196:373–5. doi: 10.1007/s00408-018-0118-6
49. Yanaru R, Hizawa K, Nakamura S, Yoshimura R, Watanabe K, Nakamura U, et al. Regression of pneumatosis cystoides intestinalis after discontinuing of alpha-glucosidase inhibitor administration. J Clin Gastroenterol. (2002) 35:204–5. doi: 10.1097/00004836-200208000-00020
50. Knechtle SJ, Davidoff AM, Rice RP. Pneumatosis intestinalis. Surgical management and clinical outcome. Ann Surg. (1990) 212:160. doi: 10.1097/00000658-199008000-00008
51. Höer J, Truong S, Virnich N, Füzesi L, Schumpelick V. Pneumatosis cystoides intestinalis: confirmation of diagnosis by endoscopic puncture a review of pathogenesis, associated disease and therapy and a new theory of cyst formation. Endoscopy. (1998) 30:793–9. doi: 10.1055/s-2007-1001424
52. Holt S, Gilmour HM, Buist TA, Marwick K, Heading RC. High flow oxygen therapy for pneumatosis coli. Gut. (1979) 20:493–8. doi: 10.1136/gut.20.6.493
53. Grieve DA, Unsworth IP. Pneumatosis cystoides intestinalis: an experience with hyperbaric oxygen treatment. Aust N Zeal J Surg. (1991) 61:423–6. doi: 10.1111/j.1445-2197.1991.tb00255.x
54. Zhang H, Jun SL, Brennan TV. Pneumatosis intestinalis: not always a surgical indication. Case Rep Surg. (2012) 2012:719713. doi: 10.1155/2012/719713
55. Ng OT, Marimuthu K, Chia PY, Koh V, Chiew CJ, De Wang L, et al. SARS-CoV-2 infection among travelers returning from Wuhan, China. N Engl J Med. (2020) 382:1476–8. doi: 10.1056/NEJMc2003100
56. Duarte-Neto AN, Monteiro RAA, da Silva LFF, Malheiros DMAC, de Oliveira EP, Theodoro-Filho J, et al. Pulmonary and systemic involvement in COVID-19 patients assessed with ultrasound-guided minimally invasive autopsy. Histopathology. (2020) 77:186–97. doi: 10.1111/his.14160
57. Driggin E, Madhavan MV, Bikdeli B, Chuich T, Laracy J, Biondi-Zoccai G, et al. Cardiovascular considerations for patients, health care workers, and health systems during the COVID-19 pandemic. J Am Coll Cardiol. (2020) 75:2352–71. doi: 10.1016/j.jacc.2020.03.031
58. Muller I, Cannavaro D, Dazzi D, Covelli D, Mantovani G, Muscatello A, et al. SARS-CoV-2-related atypical thyroiditis. Lancet Diabetes Endocrinol. (2020) 8:739–41. doi: 10.1016/S2213-8587(20)30266-7
59. Bangash MN, Patel J, Parekh D. COVID-19 and the liver: little cause for concern. Lancet Gastroenterol Hepatol. (2020) 5:529. doi: 10.1016/S2468-1253(20)30084-4
60. Tan YK, Goh C, Leow AS, Tambyah PA, Ang A, Yap ES, et al. COVID-19 and ischemic stroke: a systematic review and meta-summary of the literature. J Thromb Thromb. (2020) 50:587–95. doi: 10.1007/s11239-020-02228-y
61. Merkler AE, Parikh NS, Mir S, Gupta A, Kamel H, Lin E, et al. Risk of ischemic stroke in patients with coronavirus disease 2019 (COVID-19) vs patients with influenza. JAMA Neurol. (2020) 77:1–7. doi: 10.1001/jamaneurol.2020.2730
62. Mao L, Jin H, Wang M, Hu Y, Chen S, He Q, et al. Neurologic manifestations of hospitalized patients with coronavirus disease 2019 in Wuhan, China. JAMA Neurol. (2020) 77:683–90. doi: 10.1001/jamaneurol.2020.1127
63. Helms J, Kremer S, Merdji H, Clere-Jehl R, Schenck M, Kummerlen C, et al. Neurologic features in severe SARS-CoV-2 infection. N Engl J Med. (2020) 382:2268–70. doi: 10.1056/NEJMc2008597
64. Bhayana R, Som A, Li MD, Carey DE, Anderson MA, Blake MA, et al. Abdominal imaging findings in COVID-19: preliminary observations. Radiology. (2020) 297:E207–15. doi: 10.1148/radiol.2020201908
65. Strand V, Burmester GR, Ogale S, Devenport J, John A, Emery P. Improvements in health-related quality of life after treatment with tocilizumab in patients with rheumatoid arthritis refractory to tumour necrosis factor inhibitors: results from the 24-week randomized controlled RADIATE study. Rheumatology. (2012) 51:1860–9. doi: 10.1093/rheumatology/kes131
66. Roche. Roche provides an update on the phase III COVACTA trial of Actemra/RoActemra in hospitalised patients with severe COVID-19 associated pneumonia. Available online at: https://www.roche.com/dam/jcr:6d8de90d-2e31-43c8-b4e1-0a24a2675015/en/29072020-mr-covacta.pdf
67. Guaraldi G, Meschiari M, Cozzi-Lepri A, Milic J, Tonelli R, Menozzi M, et al. Tocilizumab in patients with severe COVID-19: a retrospective cohort study. Lancet Rheumatol. (2020) 2:e474–84. doi: 10.1016/S2665-9913(20)30173-9
68. Kewan T, Covut F, Al–Jaghbeer MJ, Rose L, Gopalakrishna KV, Akbik B. Tocilizumab for treatment of patients with severe COVID−19: a retrospective cohort study. EClinicalMedicine. (2020) 24:100418. doi: 10.1016/j.eclinm.2020.100418
69. Ackermann M, Verleden SE, Kuehnel M, Haverich A, Welte T, Laenger F, et al. Pulmonary vascular endothelialitis, thrombosis, and angiogenesis in Covid-19. N Engl J Med. (2020) 382:120–8. doi: 10.1056/NEJMoa2015432
70. Teuwen LA, Geldhof V, Pasut A, Carmeliet P. COVID-19: the vasculature unleashed. Nat Rev Immunol. (2020) 20:1–3. doi: 10.1038/s41577-020-0356-8
71. Libby P, Lüscher T. COVID-19 is, in the end, an endothelial disease. Euro Heart J. (2020) 41:3038–44. doi: 10.1093/eurheartj/ehaa623
72. Varga Z, Flammer AJ, Steiger P, Haberecker M, Andermatt R, Zinkernagel AS, et al. Endothelial cell infection and endotheliitis in COVID-19. Lancet. (2020) 395:1417–8. doi: 10.1016/S0140-6736(20)30937-5
73. Maier CL, Truong AD, Auld SC, Polly DM, Tanksley CL, Duncan A. COVID-19-associated hyperviscosity: a link between inflammation and thrombophilia?. Lancet. (2020) 395:1758–9. doi: 10.2139/ssrn.3598209
74. Hottz ED, Azevedo-Quintanilha IG, Palhinha L, Teixeira L, Barreto EA, Pão CR, et al. Platelet activation and platelet-monocyte aggregate formation trigger tissue factor expression in patients with severe COVID-19. Blood J Am Soc Hematol. (2020) 136:1330–41. doi: 10.1182/blood.2020007252
75. Panigada M, Bottino N, Tagliabue P, Grasselli G, Novembrino C, Chantarangkul V, et al. Hypercoagulability of COVID-19 patients in intensive care unit. A report of thromboelastography findings and other parameters of hemostasis. J Thromb Haemost. (2020) 18:1738–42. doi: 10.1111/jth.14850
76. Jin X, Zimmers TA, Zhang Z, Pierce RH, Koniaris LG. Interleukin-6 is an important in vivo inhibitor of intestinal epithelial cell death in mice. Gut. (2010) 59:186–96. doi: 10.1136/gut.2008.151175
77. Jiang S. Don't rush to deploy COVID-19 vaccines and drugs without sufficient safety guarantees. Nature. (2020) 579:321. doi: 10.1038/d41586-020-00751-9
78. Grein J, Ohmagari N, Shin D, Diaz G, Asperges E, Castagna A, et al. Compassionate use of remdesivir for patients with severe Covid-19. N Engl J Med. (2020) 382:2327–36. doi: 10.1056/NEJMoa2007016
79. Ohara H, Kato Y, Nakano M, Ishii Y, Serizawa H, Watanabe N, et al. A case of pneumatosis cystoides intestinalis induced by steroid pulse therapy for severe acute hepatitis B. Nihon Shokakibyo Gakkai Zasshi. (2011) 108:1237. doi: 10.11405/nisshoshi.108.1237
80. Varelas LJ, Klinge MJ, Malik SM, Borhani AA, Neal M. Idiopathic Pneumatosis intestinalis secondary to lactulose use in patients with cirrhosis. J Gastroenterol Hepatol. (2020) 35:1065–8. doi: 10.1111/jgh.14920
Keywords: pneumatosis intestinalis, COVID-19, SARS-CoV-2, mesenteric ischemia, ischemia-reperfusion injury, molecular targeted therapy, tocilizumab, IL-6 inhibitor
Citation: Miyara SJ, Becker LB, Guevara S, Kirsch C, Metz CN, Shoaib M, Grodstein E, Nair VV, Jandovitz N, McCann-Molmenti A, Hayashida K, Takegawa R, Shinozaki K, Yagi T, Aoki T, Nishikimi M, Choudhary RC, Cho YM, Zanos S, Zafeiropoulos S, Hoffman HB, Watt S, Lumermann CM, Aronsohn J, Shore-Lesserson L and Molmenti EP (2021) Pneumatosis Intestinalis in the Setting of COVID-19: A Single Center Case Series From New York. Front. Med. 8:638075. doi: 10.3389/fmed.2021.638075
Received: 05 December 2020; Accepted: 08 March 2021;
Published: 04 June 2021.
Edited by:
Jiapeng Huang, University of Louisville, United StatesReviewed by:
Andre M. Japiassu, Oswaldo Cruz Foundation (Fiocruz), BrazilTadamitsu Kishimoto, Osaka University, Japan
Copyright © 2021 Miyara, Becker, Guevara, Kirsch, Metz, Shoaib, Grodstein, Nair, Jandovitz, McCann-Molmenti, Hayashida, Takegawa, Shinozaki, Yagi, Aoki, Nishikimi, Choudhary, Cho, Zanos, Zafeiropoulos, Hoffman, Watt, Lumermann, Aronsohn, Shore-Lesserson and Molmenti. This is an open-access article distributed under the terms of the Creative Commons Attribution License (CC BY). The use, distribution or reproduction in other forums is permitted, provided the original author(s) and the copyright owner(s) are credited and that the original publication in this journal is cited, in accordance with accepted academic practice. No use, distribution or reproduction is permitted which does not comply with these terms.
*Correspondence: Ernesto P. Molmenti, emolmenti@northwell.edu
†These authors have contributed equally to this work