- 1Jiangsu Provincial Key Laboratory of Critical Care Medicine, Department of Critical Care Medicine, School of Medicine, Zhongda Hospital, Southeast University, Nanjing, China
- 2Department of Critical Care, Keenan Research Centre and Li Ka Shing Knowledge Institute, St Michael's Hospital, Toronto, ON, Canada
- 3Interdepartmental Division of Critical Care Medicine, University of Toronto, Toronto, ON, Canada
- 4Department of Critical Care Medicine, Qinghai University Affiliated Hospital, Xining, China
- 5Department of Critical Care Medicine, Wuhan Asia General Hospital, Wuhan, China
- 6Department of Critical Care Medicine, Jinggangshan University Affiliated Hospital, Jinggangshan, China
- 7Department of Critical Care Medicine, Wuhan Wuchang Hospital, Wuhan, China
- 8Department of Critical Care Medicine, Wuhan Jinyintan Hospital, Wuhan, China
- 9Department of Critical Care Medicine, School of Medicine, Ren Ji Hospital, Shanghai Jiao Tong University, Shanghai, China
- 10Medical Intensive Care Medicine, Peking Union Medical College Hospital, Peking Union Medical College, Chinese Academy of Medical Sciences, Beijing, China
Background: Different positive end-expiratory pressure (PEEP) strategies are available for subjects with coronavirus disease 2019 (COVID-19)–induced acute respiratory distress syndrome (ARDS) requiring invasive mechanical ventilation. We aimed to evaluate three conventional PEEP strategies on their effects on respiratory mechanics, gas exchanges, and hemodynamics.
Methods: This is a prospective, physiologic, multicenter study conducted in China. We recruited 20 intubated subjects with ARDS and confirmed COVID-19. We first set PEEP by the ARDSnet low PEEP–fraction of inspired oxygen (FIO2) table. After a recruitment maneuver, PEEP was set at 15, 10, and 5 cm H2O for 10 min, respectively. Among these three PEEP levels, best-compliance PEEP was the one providing the highest respiratory system compliance; best-oxygenation PEEP was the one providing the highest PaO2 (partial pressure of arterial oxygen)/FIO2.
Results: At each PEEP level, we assessed respiratory mechanics, arterial blood gas, and hemodynamics. Among three PEEP levels, plateau pressure, driving pressure, mechanical power, and blood pressure improved with lower PEEP. The ARDSnet low PEEP–FIO2 table and the best-oxygenation strategies provided higher PEEP than the best-compliance strategy (11 ± 6 cm H2O vs. 11 ± 3 cm H2O vs. 6 ± 2 cm H2O, p = 0.001), leading to higher plateau pressure, driving pressure, and mechanical power. The three PEEP strategies were not significantly different in gas exchange. The subgroup analysis showed that three PEEP strategies generated different effects in subjects with moderate or severe ARDS (n = 12) but not in subjects with mild ARDS (n = 8).
Conclusions: In our cohort with COVID-19–induced ARDS, the ARDSnet low PEEP/FIO2 table and the best-oxygenation strategies led to higher PEEP and potentially higher risk of ventilator-induced lung injury than the best-compliance strategy.
Clinical Trial Registration: www.ClinicalTrials.gov, identifier: NCT04359251.
Introduction
The World Health Organization announced the coronavirus disease 2019 (COVID-19) outbreak a pandemic on March 11, 2020. It has been reported that 67% of critically ill subjects with COVID-19 developed acute respiratory distress syndrome (ARDS) requiring invasive mechanical ventilation (1). Setting a sufficient positive end-expiratory pressure (PEEP) level is crucial for improving oxygenation, keeping alveoli open, and reducing cyclic reopening–closing (“atelectrauma”) (2, 3). However, an unnecessarily high PEEP can increase the risk of overdistension, especially in subjects with low recruitability and severe lung inhomogeneity (4–8).
Determining the appropriate or “best” PEEP is, however, challenging (9). Several methods have been used in clinical trials and routine practice. ARDSnet low and high PEEP–fraction of inspired oxygen (FIO2) tables are probably the most widely used methods in randomized controlled trials (10, 11). These tables, aiming at maintaining oxygenation while minimizing the use of high FIO2, provided great feasibility for clinical practice. Alternatively, clinicians can perform a decremental PEEP trial (12), to find the PEEP providing the highest compliance (“best-compliance” strategy) or providing the highest ratio of partial pressure of arterial oxygen (PaO2) to FIO2 (“best-oxygenation” strategy) (13–15). The so-called best-oxygenation strategy has not been used in large clinical trials but might be widely embedded in clinical practice where the oxygen saturation by pulse oximetry is often used as a surrogate.
While the current guidelines suggest using higher PEEP (>10 cm H2O) in subjects with COVID-19–induced ARDS (16), varied lung recruitability and responses to PEEP were reported from monocenter studies (17–19). Meanwhile, Marini and Gattinoni proposed that COVID-19–induced ARDS is probably different from conventional ARDS (20–22). It is unclear how those conventional PEEP strategies perform in this particular population. We thus want to evaluate three conventional PEEP strategies (ARDS low PEEP–FIO2 table, best-compliance, and best-oxygenation strategies) in subjects with COVID-19–induced ARDS, to see whether they result in different PEEP settings, respiratory mechanics, gas exchange, hemodynamics, and potential risk of ventilator-induced lung injury (VILI).
Materials and Methods
Study Population
This is a prospective physiologic study conducted in seven intensive care units (ICUs) of seven hospitals (Wuhan Jinyintan Hospital, The People's Hospital of Wuhan Xinzhou, Huangshi Hospital of Traditional Chinese Medicine, Wuhan Asia General Hospital, Wuhan Fifth Hospital, Wuhan Wuchang Hospital, and Wuhan Pulmonary Hospital, Wuhan, China) from March 5 to 16, 2020. Inclusion criteria were between 18 and 80 years old, laboratory-confirmed COVID-19, receiving invasive mechanical ventilation, and meeting the Berlin Definition of ARDS at clinical PEEP level. Exclusion criteria were pregnancy, hemodynamic instability (i.e., norepinephrine >0.05 μg/kg per minute or dopamine >5 μg/kg per minute), acute brain injury, pneumothorax, or pneumomediastinum.
The study was approved by the local Research Ethics Board. Written informed consent was obtained from substitute decision makers. The study was registered at ClinicalTrials.gov (NCT04359251).
Study Protocol
During the study, subjects were measured at a semirecumbent position under volume control ventilation with a square flow waveform. Tidal volume (VT) was kept at 6 ml/kg per predicted body weight, and respiratory rate was kept the same at different PEEP levels. Subjects were administered a continuous infusion of analgesia and sedation. If spontaneous breathing effort was strong during sedation, neuromuscular-blocking agents were administered to suppress spontaneous breathing.
PEEP trial: (1) We first set PEEP by the ARDSnet low PEEP/FIO2 table; goals of PEEP and FIO2 settings were PaO2 55-80 mm Hg, or SpO2 88-95%. An arterial blood gas (ABG) was obtained at this PEEP level. (2) We then performed a recruitment maneuver by using continuous positive airway pressure at 30 cm H2O for 30 s, to standardize lung volume history. (3) Thereafter, PEEP was set at 15, 10, and 5 cm H2O for 10 min, respectively. At each PEEP level, an ABG was obtained. In addition, if a subject had extremely high plateau pressure (Pplat, e.g., >35 cm H2O) or hemodynamic instable or refractory desaturation, the duration of that PEEP level was reduced for safety consideration (Figure 1).
“Best-compliance PEEP” was defined as the PEEP (among 15, 10, and 5 cm H2O) providing the highest respiratory system compliance (Crs).
“Best-oxygenation PEEP” was defined as the PEEP (among 15, 10, and 5 cm H2O) providing the highest PaO2/FIO2 ratio.
Measurements
Airway pressure was measured using a ventilator (SV300, Mindray, China). Pplat was measured by performing end-inspiratory occlusion. Total PEEP (PEEPtot) was measured by performing end-expiratory occlusion. Static Crs was calculated with the following formula: VT/(Pplat – PEEPtot) (23). Driving pressure was calculated with the following formula: Pplat – PEEPtot. Mechanical power was calculated with the following formula: 0.098 × RR × VT × (Ppeak – × driving pressure) (24).
Criteria of “High Risk of VILI”
To determine how many subjects were potentially exposed to high risk of VILI by three PEEP strategies, we defined the high risk of VILI by meeting one of the following criteria: (1) Pplat > 30 cm H2O, (2) driving pressure > 15 cm H2O, and (3) mechanical power > 25 J/min (11, 25, 26).
Data Collection
The general characteristics of subjects such as gender, age, height, Sequential Organ Failure Assessment (SOFA) score, and days of invasive mechanical ventilation were collected. Ventilator settings, mechanics parameters, ABG, blood pressure, and heart rate were also documented at study enrollment and during the PEEP trial.
Statistical Analysis
Data are presented as the mean ± standard deviation, unless specified otherwise. Comparisons between three PEEP levels were conducted by using analysis of variance with repeated measures or paired t-test, when appropriate. Comparisons between two groups (separated by PaO2/FIO2) were conducted by using independent t-test. p < 0.05 was considered statistically significant. SPSS 20.0 (Statistical Product and Service Solutions, Chicago, IL, USA) was used for statistical analysis.
Results
Twenty subjects (12 men and eight women, aged 64 ± 7 years, SOFA score 11 ± 2) were enrolled. The subjects received various durations of invasive mechanical ventilation before measurement (11 ± 6 days). ICU mortality was 60% (12/20). The detailed characteristics including the baseline respiratory mechanics at clinical PEEP level are reported in Table 1.
Responses to 15, 10, and 5 cm H2O of PEEP
As shown in Figure 2, Pplat, driving pressure, and mechanical power were significantly decreased when PEEP was reduced from 15 to 10 cm H2O and from 10 to 5 cm H2O. We failed to obtain ABG at PEEP 15 cm H2O in three subjects because of extremely high Pplat (>50 cm H2O) at high PEEP. After the recruitment maneuver, PaO2/FIO2 was similar at 15 and 10 cm H2O of PEEP but dropped at 5 cm H2O of PEEP (p = 0.005). PaCO2 was significantly higher at 15 cm H2O of PEEP than at 10 and 5 cm H2O of PEEP (p = 0.018). Heart rate was similar at three PEEP levels, but mean arterial pressure (MAP) was lower at 15 cm H2O of PEEP (p = 0.012).
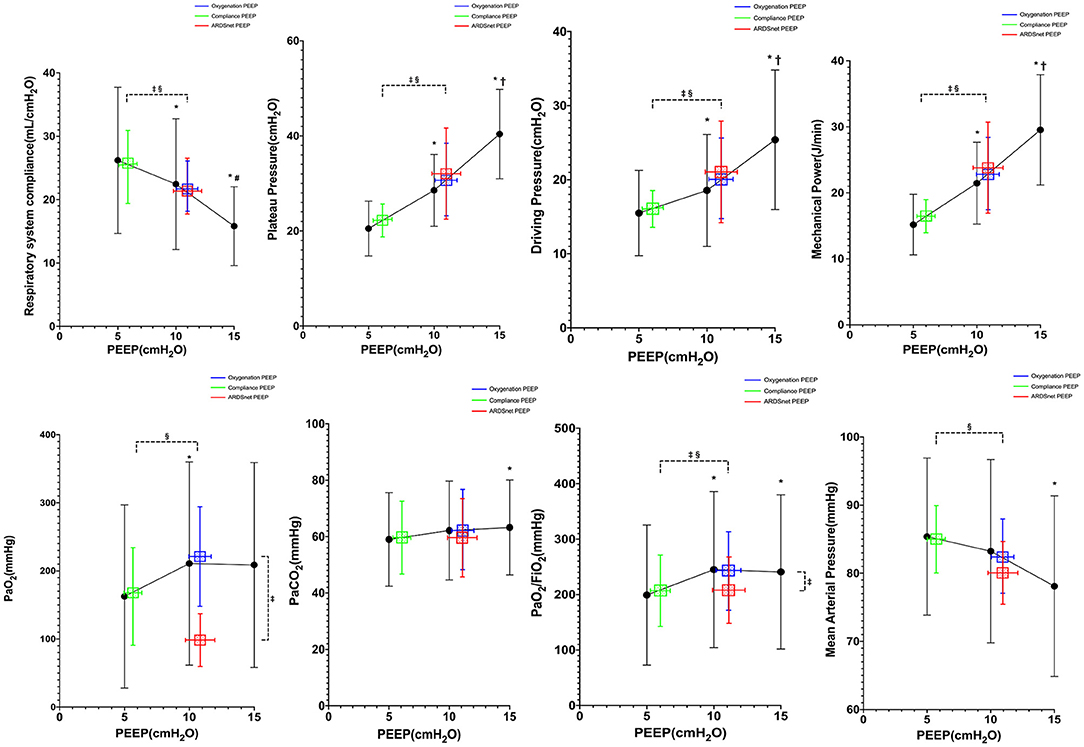
Figure 2. Reponses to PEEP in all subjects (n = 20). *vs. PEEP 5 cm H2O, p < 0.05; †vs. PEEP 10 cm H2O, p < 0.05; ‡vs. oxygenation PEEP, p < 0.05; §vs. ARDSnet PEEP, p < 0.05.
Comparisons of Three PEEP Strategies
As shown in Figure 2, the PEEP guided by best compliance was lower than the PEEP guided by best oxygenation and the PEEP guided by ARDSnet low PEEP/FIO2 table (6 ± 2 vs. 11 ± 4 and 11 ± 6 cm H2O, p = 0.001). Pplat, driving pressure, and mechanical power by best-compliance strategy were lower than those by best-oxygenation strategy and ARDSnet low PEEP–FIO2 table (Pplat: 21 ± 6 vs. 31 ± 11 and 32 ± 15 cm H2O, p <0.001; driving pressure: 15 ± 6 vs. 20 ± 9 and 21 ± 10 cm H2O, p < 0.001; mechanical power: 15.9 ± 4.5 vs. 23.5 ± 9.6 and 22.9 ± 10.9 J/min, p = 0.001). Crs by best-compliance strategy were higher than those by best-oxygenation strategy and ARDSnet low PEEP–FIO2 table (26.8 ± 11.8 vs. 20.6 ± 8.0 and 20.3 ± 9.3 mL/cm H2O, p <0.01). PaO2 by ARDSnet low PEEP–FIO2 table was lower than those by best-compliance strategy and best-oxygenation strategy (93.3 ± 40.0 vs. 229.7 ± 159.8 and 187.4 ± 146.2 mm Hg, p < 0.01). Best-oxygenation PEEP provided highest PaO2/FIO2 (293 ± 137.8 mm Hg), whereas best-compliance PEEP provided higher PaO2/FIO2 than the ARDSnet low PEEP–FIO2 table (252 ± 130 vs. 204 ± 103 mm Hg). PaCO2 was similar among the three strategies (p = 0.58). MAP was significantly higher with the PEEP guided by best compliance than MAP with the other two strategies (p < 0.001), whereas heart rate was similar (p = 0.96).
Subgroup Analysis
We divided subjects into two subgroups (mild ARDS and moderate/severe ARDS) according to PaO2/FIO2 at study enrollment based on Berlin Definition (27). Characteristics of the subjects, including gender, age, SOFA score, and duration of mechanical ventilation were similar between two subgroups. PaO2 and PaO2/FIO2 were higher in the mild ARDS group than those in the moderate/severe ARDS group. VT, FIO2, PaCO2, Pplat, PEEP, driving pressure, Crs, MAP, and heart rate at baseline (before initiation of the protocol) were not different between the two groups (Table 1).
There were eight subjects in the study with mild ARDS at study enrollment. Pplat, driving pressure, and mechanical power decreased significantly from PEEP 15 to 5 cm H2O (Figure 3). PEEP levels were not significantly different among the three PEEP strategies. PaO2, Pplat, Crs, and mechanical power were not different among the three PEEP strategies (Figure 3). However, driving pressure at best-oxygenation PEEP and that at ARDSnet PEEP were higher than driving pressure at best-compliance PEEP (16 ± 8 and 18 ± 11 vs. 14 ± 7 cm H2O, p = 0.03). There was one subject with missing ABG results. PaCO2, MAP, and HR at PEEP guided by the three strategies were similar (Figure 3).
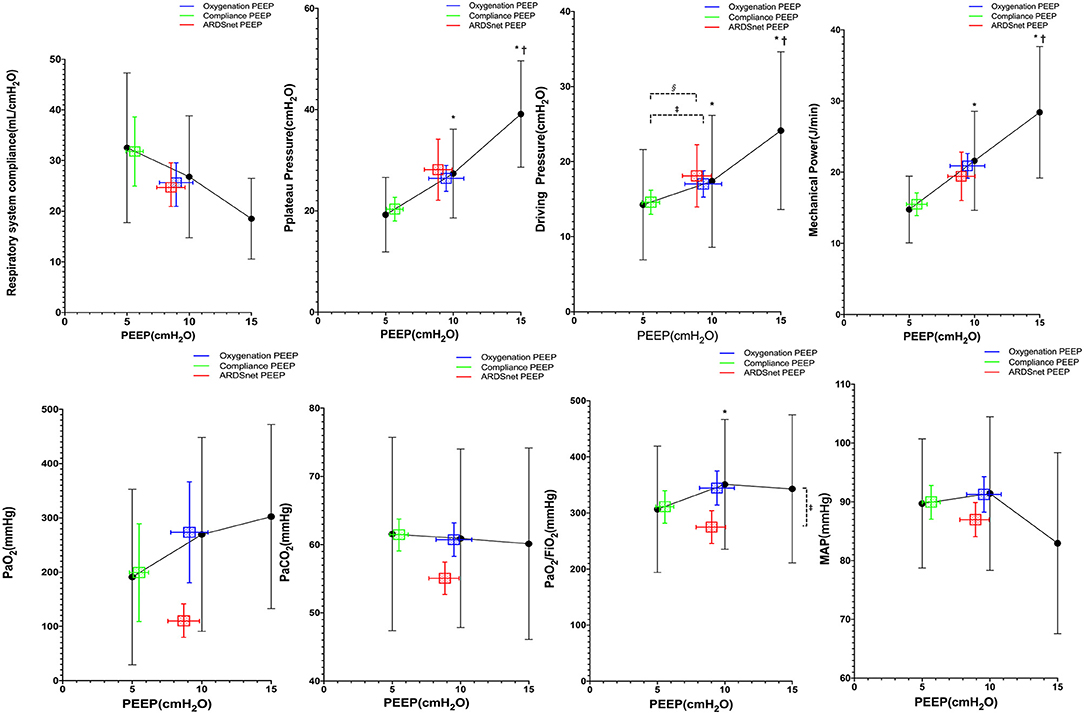
Figure 3. Reponses to PEEP in subjects with mild ARDS (n = 8). *vs. PEEP 5 cm H2O, p <0.05; †vs. PEEP 10 cm H2O, p < 0.05; ‡vs. oxygenation PEEP, p < 0.05; §vs. ARDSnet PEEP, p < 0.05.
There were 12 subjects with moderate/severe ARDS at study enrollment. Pplat, driving pressure, and mechanical power decreased significantly from PEEP 15 to 5 cm H2O (Figure 4), whereas MAP increased significantly at lower PEEP (Figure 4). ARDSnet PEEP and best-oxygenation PEEP were higher than the best-compliance PEEP (12 ± 7 and 12 ± 4 vs. 6 ± 2 cm H2O, p = 0.007). Pplat, driving pressure, and mechanical power at best-oxygenation PEEP and ARDSnet PEEP were higher than at best-compliance PEEP (Pplat: 35 ± 12 and 36 ± 15 vs. 22 ± 14 cm H2O, p = 0.002; driving pressure: 23 ± 10 and 24 ± 10 vs. 16 ± 5 cm H2O, p = 0.002; mechanical power: 26 ± 11 and 25 ± 11 vs. 16 ± 5 J/min, p = 0.004) (Figure 4). Crs at best-compliance PEEP was higher than those at best-oxygenation PEEP and ARDSnet PEEP (23.0 ± 7.8 vs. 16.6 ± 4.8 and 16.7 ± 7.5 ml/cm H2O, p < 0.01). There were three subjects without ABG measurements at PEEP 15 cm H2O as previously mentioned. PaO2 and PaO2/FIO2 were lower with ARDSnet PEEP. pH, PaCO2, MAP, and HR between the three strategies were similar.
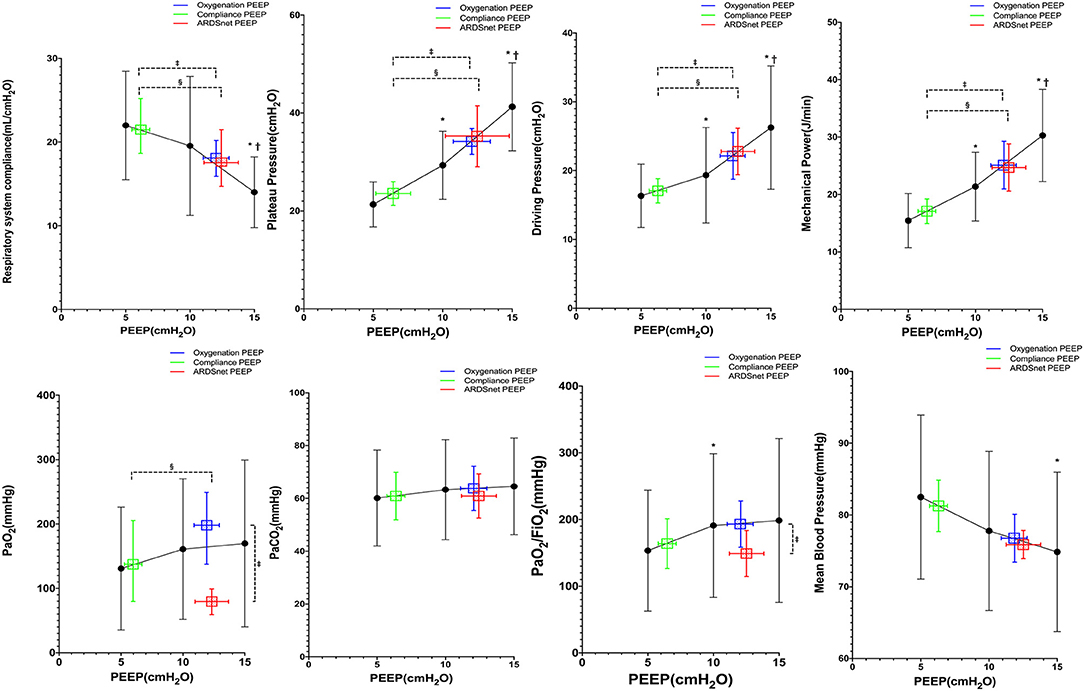
Figure 4. Reponses to PEEP in subjects with moderate or severe ARDS (n = 12). *vs. PEEP 5 cm H2O, p < 0.05; †vs. PEEP 10 cm H2O, p < 0.05; ‡vs. oxygenation PEEP, p < 0.05; §vs. ARDSnet PEEP, p < 0.05.
Potential Risk of VILI
In mild ARDS subjects, the potential risks of lung injury induced by three PEEP strategies were similar and relatively low (Table 2). In moderate/severe ARDS subjects, however, both best-oxygenation PEEP and ARDSnet PEEP generated “injurious” Pplat (>30 cm H2O) and mechanical power (>25 J/min) more frequently than best-compliance PEEP (Table 2).
Discussion
The main findings of the present study are that in COVID-19–induced ARDS subjects mechanically ventilated in Wuhan, China, most of the subjects had a poor response to high PEEP. The PEEP/FIO2 table and best-oxygenation PEEP had similar effects to best-compliance PEEP on gas exchanges and hemodynamics, but there was an increased risk of lung injury due to high PEEP levels particularly for the subjects with moderate/severe ARDS.
Lung recruitability of COVID-19–induced ARDS is highly variable. Our previous study assessed the potential for lung recruitment through the recruitment-to-inflation ratio (28), showing that the majority of the cohort had poor recruitability to high PEEP in subjects with COVID-19–induced ARDS (19). In two recent studies by Beloncle et al. and Mauri et al., however, the potential for lung recruitment (assessed by the recruitment-to-inflation ratio) of subjects with COVID-19–induced ARDS in Italy and France was much higher than what we found in Wuhan, with larger intersubject variability (17, 18). Gattinoni and Marini proposed a hypothesis that COVID-19 pneumonia subjects should be divided into different phenotypes to offer different respiratory support (20–22, 29, 30). Subjects in the present study had poor response to high PEEP (dramatic increase in Pplat, driving pressure, mechanical power with reduction in MAP). These poor responses to high PEEP suggest poor lung recruitability in these subjects, which is consistent with our previous study in which lung recruitability was directly measured (19) and other studies (31–35). However, subjects in our study also presented with low baseline compliance, which were not the proposed “type H phenotype” patients and differ from other studies.
We suspect that the differences in lung recruitability and compliance among studies might be caused by different durations of invasive ventilation prior to study enrollment. The subjects in our study received relatively long durations of invasive mechanical ventilation before measurement (11 ± 6 days), which can generate progressive lung fibrosis and thus worsen the compliance and lower the lung recruitability. The time course of changes in mechanics has been well-illustrated by (29). Alternatively, these differences might be related to the different lineages of coronavirus (36–39).
Limiting the risk of hyperinflation of the “baby lung” when applying high PEEP to promote the recruitment of the collapsed lung is essential. The present study showed that the PEEP selected by ARDSnet low PEEP–FIO2 table or the best-oxygenation methods was significantly higher than the PEEP selected by the best-compliance method. As a consequence, both ARDSnet low PEEP–FIO2 table and the best-oxygenation strategies led to higher Pplat, driving pressure, and mechanical power. Although the thresholds of the limits of these parameters can be debatable, the present study showed that the incidences of “injurious” Pplat (>30 cm H2O) and mechanical power (>25 J/min) were higher in ARDSnet low PEEP–FIO2 table and the best-oxygenation strategy in subjects with moderate or severe ARDS. Although we did not test the ARDSnet high PEEP–FIO2 table in our study, it obviously would have increased the risk of overdistension in our subjects. By contrast, the best-compliance strategy did not bring notable risk of overdistension in mild or moderate/severe ARDS subjects.
There are some limitations in our study. (1) Although it is a multicenter study, the sample size is less than expected because of a dramatic reduction in the number of newly admitted ICU subjects during the study. (2) Duration of mechanical ventilation of subjects involved after intubation was 11 ± 6 days in this study. Caution is required for comparing our results with other studies, which enrolled subjects in an earlier phase of mechanical ventilation. (3) We did not assess lung recruitability directly. Instead, we assessed the change in respiratory mechanics, gas exchange, and hemodynamic effects during the PEEP trial, which reflect the subjects' response to PEEP. (4) We simplified the PEEP trial compared to other studies, which used a decremental PEEP trial by 2 cm H2O after a recruitment maneuver. (5) We did not measure biomarkers at different PEEP settings, which can help us better assess the risk of VILI.
In our cohort with COVID-19–induced ARDS from Wuhan, the ARDSnet low PEEP/FIO2 table and the best-oxygenation strategies provide higher PEEP than the best-compliance strategy. Our subjects had poor responses to high PEEP as high PEEP often led to excessive Pplat, driving pressure mechanical power, and worse MAP. Further studies on the effects of PEEP on COVID-19–induced ARDS are needed to confirm our findings.
Data Availability Statement
The original contributions generated for the study are included in the article/supplementary material, further inquiries can be directed to the corresponding author/s.
Ethics Statement
The studies involving human participants were reviewed and approved by Wuhan Jinyintan Research Ethics Board. Written informed consent for participation was not required for this study in accordance with the national legislation and the institutional requirements.
Author Contributions
BD and HQ: conceptualization, methodology, project administration, visualization, funding acquisition, and supervision. CP and CL: formal analysis. CP, XS, HR, HW, LX, YH, and JX: investigation. CP, CL, and LC: writing—original draft. CP, CL, YY, and LC: writing—review and editing. All authors have read and agreed to the published version of the manuscript.
Funding
This work was funded by Jiangsu Provincial Special Program of Medical Science (BE2018743 and BE2019749), National Science and Technology Major Project for Control and Prevention of Major Infectious Diseases of China (2017ZX10103004), National Natural Science Foundation of China (81571847 and 81930058), Jiangsu Provincial Key Laboratory of Critical Care Medicine, Department of Critical Care Medicine, Zhongda Hospital, School of Medicine, Southeast University, Grants from the Ministry of Science and Technology of the People's Republic of China (2020YFC0843700), and Open Project Fund of Key Laboratory of Environmental Medical Engineering from Ministry of Education (2020EME001).
Conflict of Interest
The authors declare that the research was conducted in the absence of any commercial or financial relationships that could be construed as a potential conflict of interest.
References
1. Yang X, Yu Y, Xu J, Shu H, Xia J, Liu H, et al. Clinical course and outcomes of critically ill patients with SARS-CoV-2 pneumonia in Wuhan, China: a single-centered, retrospective, observational study. Lancet Respir Med. (2020) 8:475–81. doi: 10.1016/S2213-2600(20)30079-5
2. Muscedere JG, Mullen JB, Gan K, Slutsky AS. Tidal ventilation at low airway pressures can augment lung injury. Am J Respir Crit Care Med. (1994) 149:1327–34. doi: 10.1164/ajrccm.149.5.8173774
3. Briel M, Meade M, Mercat A, Brower RG, Talmor D, Walter SD, et al. Higher vs lower positive end-expiratory pressure in patients with acute lung injury and acute respiratory distress syndrome: systematic review and meta-analysis. JAMA. (2010) 303:865–73. doi: 10.1001/jama.2010.218
4. Rouby JJ. Lung overinflation. The hidden face of alveolar recruitment. Anesthesiology. (2003) 99:2–4. doi: 10.1097/00000542-200307000-00003
5. Gattinoni L, Caironi P, Cressoni M, Chiumello D, Ranieri VM, Quintel M, et al. Lung recruitment in patients with the acute respiratory distress syndrome. N Engl J Med. (2006) 354:1775–86. doi: 10.1056/NEJMoa052052
6. Grasso S, Stripoli T, Sacchi M, Trerotoli P, Staffieri F, Franchini D, et al. Inhomogeneity of lung parenchyma during the open lung strategy: a computed tomography scan study. Am J Respir Crit Care Med. (2009) 180:415–23. doi: 10.1164/rccm.200901-0156OC
7. Chiumello D, Cressoni M, Carlesso E, Caspani ML, Marino A, Gallazzi E, et al. Bedside selection of positive end-expiratory pressure in mild, moderate, and severe acute respiratory distress syndrome. Crit Care Med. (2014) 42:252–64. doi: 10.1097/CCM.0b013e3182a6384f
8. Camporota L, Caricola EV, Bartolomeo N, Di Mussi R, Wyncoll DLA, Meadows CIS, et al. Lung recruitability in severe acute respiratory distress syndrome requiring extracorporeal membrane oxygenation. Crit Care Med. (2019) 47:1177–83. doi: 10.1097/CCM.0000000000003837
9. Sahetya SK, Goligher EC, Brower RG. Fifty years of research in ards. Setting positive end-expiratory pressure in acute respiratory distress syndrome. Am J Respir Crit Care Med. (2017) 195:1429–38. doi: 10.1164/rccm.201610-2035CI
10. Brower RG, Lanken PN, Macintyre N, Matthay MA, Morris A, Ancukiewicz M, et al. Higher versus lower positive end-expiratory pressures in patients with the acute respiratory distress syndrome. N Engl J Med. (2004) 351:327–36. doi: 10.1056/NEJMoa032193
11. Mercat A, Richard JC, Vielle B, Jaber S, Osman D, Diehl JL, et al. Positive end-expiratory pressure setting in adults with acute lung injury and acute respiratory distress syndrome: a randomized controlled trial. JAMA. (2008) 299:646–55. doi: 10.1001/jama.299.6.646
12. Hickling KG. Best compliance during a decremental, but not incremental, positive end-expiratory pressure trial is related to open-lung positive end-expiratory pressure: a mathematical model of acute respiratory distress syndrome lungs. Am J Respir Crit Care Med. (2001) 163:69–78. doi: 10.1164/ajrccm.163.1.9905084
13. Suter PM, Fairley B, Isenberg MD. Optimum end-expiratory airway pressure in patients with acute pulmonary failure. N Engl J Med. (1975) 292:284–9. doi: 10.1056/NEJM197502062920604
14. Caramez MP, Kacmarek RM, Helmy M, Miyoshi E, Malhotra A, Amato MB, et al. A comparison of methods to identify open-lung PEEP. Intensive Care Med. (2009) 35:740–7. doi: 10.1007/s00134-009-1412-9
15. Writing Group for the Alveolar Recruitment for Acute Respiratory Distress Syndrome Trial I Cavalcanti AB Suzumura EA Laranjeira LN Paisani DM Damiani LP . Effect of lung recruitment and titrated positive end-expiratory pressure (PEEP) vs low peep on mortality in patients with acute respiratory distress syndrome: a randomized clinical trial. JAMA. (2017) 318:1335–45. doi: 10.1001/jama.2017.14171
16. Alhazzani W, Moller MH, Arabi YM, Loeb M, Gong MN, Fan E, et al. Surviving sepsis campaign: guidelines on the management of critically ill adults with coronavirus disease 2019 (COVID-19). Crit Care Med. (2020) 48:e440–69. doi: 10.1097/CCM.0000000000004363
17. Beloncle FM, Pavlovsky B, Desprez C, Fage N, Olivier PY, Asfar P, et al. Recruitability and effect of PEEP in SARS-Cov-2-associated acute respiratory distress syndrome. Ann Intensive Care. (2020) 10:55. doi: 10.1186/s13613-020-00675-7
18. Mauri T, Spinelli E, Scotti E, Colussi G, Basile MC, Crotti S, et al. Potential for lung recruitment and ventilation-perfusion mismatch in patients with the acute respiratory distress syndrome from coronavirus disease 2019. Crit Care Med. (2020) 48:1129–34. doi: 10.1097/CCM.0000000000004386
19. Pan C, Chen L, Lu C, Zhang W, Xia JA, Sklar MC, et al. Lung recruitability in COVID-19-associated acute respiratory distress syndrome: a single-center observational study. Am J Respir Crit Care Med. (2020) 201:1294–7. doi: 10.1164/rccm.202003-0527LE
20. Gattinoni L, Coppola S, Cressoni M, Busana M, Rossi S, Chiumello D. COVID-19 does not lead to a “typical” acute respiratory distress syndrome. Am J Respir Crit Care Med. (2020) 201:1299–300. doi: 10.1164/rccm.202003-0817LE
21. Marini JJ. Dealing with the CARDS of COVID-19. Crit Care Med. (2020) 48:1239–41. doi: 10.1097/CCM.0000000000004427
22. Marini JJ, Gattinoni L. Management of COVID-19 respiratory distress. JAMA. (2020) 323:2329–30. doi: 10.1001/jama.2020.6825
23. Protti A, Andreis DT, Monti M, Santini A, Sparacino CC, Langer T, et al. Lung stress and strain during mechanical ventilation: any difference between statics and dynamics? Crit Care Med. (2013) 41:1046–55. doi: 10.1097/CCM.0b013e31827417a6
24. Gattinoni L, Tonetti T, Cressoni M, Cadringher P, Herrmann P, Moerer O, et al. Ventilator-related causes of lung injury: the mechanical power. Intensive Care Med. (2016) 42:1567–75. doi: 10.1007/s00134-016-4505-2
25. Amato MB, Meade MO, Slutsky AS, Brochard L, Costa EL, Schoenfeld DA, et al. Driving pressure and survival in the acute respiratory distress syndrome. N Engl J Med. (2015) 372:747–55. doi: 10.1056/NEJMsa1410639
26. Collino F, Rapetti F, Vasques F, Maiolo G, Tonetti T, Romitti F, et al. Positive end-expiratory pressure and mechanical power. Anesthesiology. (2019) 130:119–30. doi: 10.1097/ALN.0000000000002458
27. Force ADT, Ranieri VM, Rubenfeld GD, Thompson BT, Ferguson ND, Caldwell E, et al. Acute respiratory distress syndrome: the Berlin Definition. JAMA. (2012) 307:2526–33. doi: 10.1001/jama.2012.5669
28. Chen L, Del Sorbo L, Grieco DL, Junhasavasdikul D, Rittayamai N, Soliman I, et al. Potential for lung recruitment estimated by the recruitment-to-inflation ratio in acute respiratory distress syndrome. A clinical trial. Am J Respir Crit Care Med. (2020) 201:178–87. doi: 10.1164/rccm.201902-0334OC
29. Camporota L, Vasques F, Sanderson B, Barrett NA, Gattinoni L. Identification of pathophysiological patterns for triage and respiratory support in COVID-19. Lancet Respir Med. (2020) 8:752–4. doi: 10.1016/S2213-2600(20)30279-4
30. Grasso S, Mirabella L, Murgolo F, Di Mussi R, Pisani L, Dalfino L, et al. Effects of positive end-expiratory pressure in “high compliance” severe acute respiratory syndrome Coronavirus 2 acute respiratory distress syndrome. Crit Care Med. (2020) 48:E1332–6. doi: 10.1097/CCM.0000000000004640
31. Bonny V, Janiak V, Spadaro S, Pinna A, Demoule A, Dres M. Effect of PEEP decremental on respiratory mechanics, gasses exchanges, pulmonary regional ventilation, and hemodynamics in patients with SARS-Cov-2-associated acute respiratory distress syndrome. Crit Care. (2020) 24:596. doi: 10.1186/s13054-020-03392-6
32. Chiumello D, Busana M, Coppola S, Romitti F, Formenti P, Bonifazi M, et al. Physiological and quantitative CT-scan characterization of COVID-19 and typical ARDS: a matched cohort study. Intensive Care Med. (2020) 46:2187–96. doi: 10.1007/s00134-020-06281-2
33. Das A, Saffaran S, Chikhani M, Scott TE, Laviola M, Yehya N, et al. In silico modeling of Coronavirus Disease 2019 acute respiratory distress syndrome: pathophysiologic insights and potential management implications. Crit Care Explor. (2020) 2:e0202. doi: 10.1097/CCE.0000000000000202
34. Roesthuis L, Van Den Berg M, Van Der Hoeven H. Advanced respiratory monitoring in COVID-19 patients: use less PEEP! Crit Care. (2020) 24:230. doi: 10.1186/s13054-020-02953-z
35. Tsolaki V, Siempos I, Magira E, Kokkoris S, Zakynthinos GE, Zakynthinos S. PEEP levels in COVID-19 pneumonia. Crit Care. (2020) 24:303. doi: 10.1186/s13054-020-03049-4
36. Eskier D, Suner A, Karakulah G, Oktay Y. Mutation density changes in SARS-CoV-2 are related to the pandemic stage but to a lesser extent in the dominant strain with mutations in spike and RdRp. PeerJ. (2020) 8:e9703. doi: 10.7717/peerj.9703
37. Tang X, Wu C, Li X, Song Y, Yao X, Wu X, et al. On the origin and continuing evolution of SARS-CoV-2. Natl Sci Rev. (2020) 7:1012–23. doi: 10.1093/nsr/nwaa036
38. Lythgoe KA, Hall M, Ferretti L, De Cesare M, Macintyre-Cockett G, Trebes A, et al. SARS-CoV-2 within-host diversity and transmission. Science. (2021) 372:eabg0821. doi: 10.1126/science.abg0821
Keywords: acute respiratory distress syndrome, coronavirus disease 2019, positive end-expiratory pressure, mechanical ventilation, lung injury
Citation: Pan C, Lu C, She X, Ren H, Wei H, Xu L, Huang Y, Xia J, Yu Y, Chen L, Du B and Qiu H (2021) Evaluation of Positive End-Expiratory Pressure Strategies in Patients With Coronavirus Disease 2019–Induced Acute Respiratory Distress Syndrome. Front. Med. 8:637747. doi: 10.3389/fmed.2021.637747
Received: 04 December 2020; Accepted: 04 June 2021;
Published: 20 July 2021.
Edited by:
Jiapeng Huang, University of Louisville, United StatesReviewed by:
Declan Bates, University of Warwick, United KingdomE. Wang, Central South University, China
Copyright © 2021 Pan, Lu, She, Ren, Wei, Xu, Huang, Xia, Yu, Chen, Du and Qiu. This is an open-access article distributed under the terms of the Creative Commons Attribution License (CC BY). The use, distribution or reproduction in other forums is permitted, provided the original author(s) and the copyright owner(s) are credited and that the original publication in this journal is cited, in accordance with accepted academic practice. No use, distribution or reproduction is permitted which does not comply with these terms.
*Correspondence: Haibo Qiu, haiboq2000@163.com
†These authors have contributed equally to this work