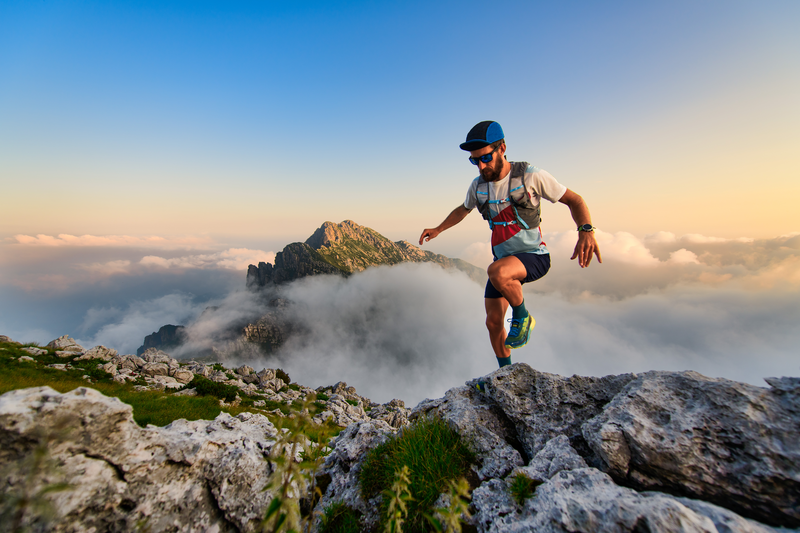
94% of researchers rate our articles as excellent or good
Learn more about the work of our research integrity team to safeguard the quality of each article we publish.
Find out more
ORIGINAL RESEARCH article
Front. Med. , 30 March 2021
Sec. Nephrology
Volume 8 - 2021 | https://doi.org/10.3389/fmed.2021.626909
Zinc (Zn) and magnesium (Mg) are essential trace elements in humans. Their deficiency may be associated with inflammation and oxidative stress (OS) in patients with diabetic nephropathy (DN), but the mechanisms involved have not been fully characterized. We aimed to investigate the relationships between circulating concentrations of Zn and Mg and pro-inflammatory factors with DN-associated renal functional damage in patients with type 2 diabetes mellitus (T2DM). To this end, we studied 20 healthy people, 24 patients with T2DM, and 59 patients with T2DM and T2DN. Serum and urine Zn and Mg concentrations were measured using the 2-(5-nitro-2-pyridylazo)-5-(N-propyl-N-sulfopropylamine) phenol (nitro-PAPS) chromogenic method and the xylidyl blue method, respectively, and the circulating concentrations of pro-inflammatory cytokines [interleukin (IL)-1β, IL-6, IL-8, IL-10, IL-12, and tumor necrosis factor-α (TNF-α)] were measured using flow cytometry. The serum concentrations of Zn and Mg were significantly lower in patients with T2DM and DN than in healthy controls. Serum Zn, urine Zn, and urine Mg concentrations decreased, while those of IL-6 and IL-8 increased with the progression of DN-associated renal functional damage. Furthermore, the serum and urine Zn concentrations negatively correlated with the serum IL-6 and IL-8 concentrations. Notably, the serum Zn concentration was found to independently protect against DN in patients with T2DM. Hypozincemia may be associated with the T2DN-associated renal functional damage because it exacerbates inflammation.
Diabetes mellitus (DM) is a well-established public health problem because of its high prevalence and morbidity (1). Diabetic nephropathy (DN) is one of the most important microvascular complications of DM. The principal pathological features of DN are glomerular sclerosis and interstitial fibrosis (2), and the mechanism is thought to involve the induction of oxidative stress (OS) (3), the activation of inflammatory and fibrosis signaling pathways (4), the accumulation of advanced glycation end-products (5), abnormal renal hemodynamics, and abnormal activation of the renin-angiotensin-aldosterone system, secondary to chronic hyperglycemia (6). In particular, OS and inflammation are thought to play important roles in the pathogenesis of DN (7, 8).
The trace elements zinc (Zn) and magnesium (Mg) play important roles in the function of various enzymes and transcription factors (9, 10). Zn has anti-inflammatory effects and is involved in the induction of antioxidant proteins (11). Many previous studies have shown Zn deficiency in patients with type 2 diabetes mellitus (T2DM) and DN, and in animal models of nephropathy (12, 13). Specifically, Li et al. (14) have shown that in diabetes, Zn promotes the dissociation of nuclear factor-erythroid 2-related factor 2 from Kelch-like ECH-associated protein-1 and its translocation to the nucleus, where it promotes the expression of downstream antioxidant genes. In addition, Barman et al. (13) showed that Zn administration inhibits activation of the nuclear factor kappa-B (NF-κB) signaling pathway, thereby reducing oxidative damage and the inflammatory response in the kidneys of rats with streptozotocin-induced diabetes.
Mg is not only involved in many important metabolic processes, but also has antioxidant, anti-inflammatory, and anti-apoptotic effects (15). Mg deficiency leads to impaired glucose tolerance, insulin resistance, abnormal lipid metabolism, and OS (10). Previous studies have shown that the prevalence of hypomagnesemia in patients with DN is significantly higher than that in those without (16). In addition, Mg deficiency leads to the opening of L-type calcium (Ca2+) channels in macrophage, which increases the intracellular Ca2+ concentration and promotes the release of pro-inflammatory cytokines. Conversely, supplementation of Mg reduces the production of pro-inflammatory cytokines (17).
In the present study, we aimed to determine (1) the predictors of DN in patients with T2DM; (2) the relationships between Zn and Mg concentrations and DN-associated renal functional damage in patients with T2DM; and (3) the relationships between Zn and Mg concentrations and pro-inflammatory cytokines in patients with T2DM.
Clinical data was collected for patients with T2DM and DN who were hospitalized in the nephrology and endocrinology department of the First Hospital of Jilin University between September 2017 and January 2019. At the same time, we recruited a group of healthy individuals who underwent check-ups at a physical examination center.
The inclusion criteria for the study were (1) conformation with the 1999 World Health Organization diagnostic criteria for T2DM and/or the 2007 National Kidney Foundation's Kidney Disease Outcomes Quality Initiative guidelines for the diagnosis of DN; (2) patients who were not undergoing dialysis; and (3) a complete set of clinical data. The exclusion criteria were (1) presence of a malignant tumor; (2) presence of severe cardiac insufficiency; (3) presence of non-diabetic nephropathy; (4) recent infection, fever, or immune-mediated disease; and (5) lack of compliance with the protocol; (6) use of dietary Zn and/or Mg supplements in the past 3 months.
T2DM was defined using the World Health Organization 1999 criteria as follows: (1) presence of typical symptoms, with a random plasma glucose of ≥11.1 mmol/L, (2) fasting plasma glucose (FPG) ≥ 7.0 mmol/L, or (3) 2-h glucose concentration during an oral glucose tolerance test of ≥11.1 mmol/L. Individuals without typical symptoms had their plasma glucose measured on a second day. Individuals fulfilling one of the above criteria were diagnosed with diabetes. DN was diagnosed using the 2007 KDOQI guidelines as follows: (1) macroalbuminuria (albumin/creatinine ratio (ACR) >300 mg/g), (2) presence of diabetic retinopathy and microalbuminuria, or (3) duration of T2DM > 10 years and microalbuminuria.
The participants were allocated to the following groups according to the presence of T2DM and/or DN: (1) T2DM + DN group (n = 59), with ACR ≥30 mg/g; (2) T2DM group, with ACR <30 mg/g (n = 24); and (3) healthy control group (n = 20). Participants in the control group were normal on physical examination and did not have a family history of diabetes, cardiovascular disease, or serious liver or kidney disease.
According to the ACR and estimated glomerular filtration rate (eGFR), the participants with DN were further allocated to three subgroups as follows: (1) microalbuminuria group, with 30 ≤ ACR <300 mg/g (n = 18); (2) macroalbuminuria group, with ACR ≥ 300 mg/g (n = 24); and (3) renal failure group, in which the participants had an eGFR <15 ml min−1.1.73 m−2 but were not undergoing dialysis (n = 18).
Clinical data were collected from medical records and comprised sex, age, height, body mass, history of smoking, and history of hypertension.
Glycated hemoglobin (HbA1c) was measured using high-performance liquid chromatography (Variant II; Bio-Rad, CA, USA). FPG, blood urea nitrogen (BUN), serum creatinine (SCr), serum uric acid (UA), total cholesterol (TC), and triglyceride (TG) concentrations were measured using enzymic methods (7600-210 automatic biochemical analyzer; Hitachi, Tokyo, Japan). Twenty-four-hour urine albumin loss was measured using a turbidimetric immunoassay (BN II; Siemens, Marburg Germany). The pyrogallol red and amino acid oxidase methods were used to determine urinary albumin and creatinine concentrations, respectively, which were used to calculate the ACR.
eGFR was calculated using the Modification of Diet in Renal Disease formula as follows: eGFR (ml min−1 1.73 m−2) = 186 × SCr (mg/dl)−1.154 × age (years)−0.203 ( ×0.742 for women).
The concentrations of Zn and Mg were measured on a Hitachi 7600-210 automatic biochemical analyzer (Hitachi, Tokyo, Japan) using the PAPS chromogenic method (BEBS, Beijing, China) and the Xylidyl blue method (Leadman, Beijing, China), respectively.
Plasma levels of IL-1β, IL-6, IL-8, IL-10, IL-12, and TNF-α were measured using the Cytometric Bead Array Human Inflammation kit (CBA; BD Biosciences, NJ, USA). Plasma was stored at −80°C until use. Six human inflammatory cytokine standard curves with concentrations standard ranging from 20 to 5,000 pg/ml were obtained using a set of standard solutions. The capture beads were added to the assay tubes and vortexed. Next, 50 μL of each plasma sample was added to the sample tubes and 50 μL of each human standard dilution was added to the control tubes. Then, 50 μL of PE detection reagent was added to all assay tubes and the tubes were incubated for 3 h. The results were acquired and the data were analyzed using Cell Quest software and FCAP Array software. C-reactive protein (CRP) concentration was measured using a turbidimetric immunoassay method on an AU5800 (Beckman Coulter).
SPSS 22.0 (IBM, Inc., Armonk, NY, USA) was used to analyze the data. Normally distributed data are expressed as mean ± standard deviation (SD). One-way analysis of variance (ANOVA) was used to compare normally distributed data between multiple groups. Non-normally distributed data are expressed as median (range). The Mann–Whitney U-test was used to compare non-normally distributed data between two groups, and the Kruskal–Wallis test was used to compare such data between multiple groups. The chi-square test was used to compare ratios among groups. A bivariate regression model was used to identify risk factors for DN. The relationships of Zn, Mg, and cytokine concentrations with renal function were analyzed using Pearson's correlation for normally distributed data and Spearman's correlation for non-normally distributed data. P < 0.05 was regarded as statistically significant.
Of the 103 participants, 59 had T2DN, 24 had T2DM alone, and there were 20 controls. The T2DN group contained 39 men (66.1%) and had a mean age of 56.4 ± 7.3 years; the T2DM group contained 17 men (70.8%) and had a mean age of 52.7 ± 10.1 years; and the healthy control group contained 10 men (50%) and had a mean age of 53.0 ± 5.7 years. Table 1 shows that there were no significant differences between the T2DM, T2DM + T2DN, and control groups with respect to sex ratio, age, smoking history, serum TC, 24 h urine Mg loss, serum IL-12 concentration, or serum TNF-α concentration. The T2DM + T2DN and T2DM groups had a higher body mass index (BMI) and higher FPG, HbA1c, 24 h urine Zn, and serum CRP, IL-6, and IL-10 concentrations, as well as lower serum Mg and Zn concentrations than the control group (P < 0.05). The T2DM + T2DN group contained more participants with hypertension; had higher BUN, SCr, UA, TC, IL-6, and IL-8 concentrations; and had lower serum Zn and eGFR than the T2DM and healthy control groups (P < 0.05). Finally, the T2DM + T2DN group had lower 24 h urine Zn loss, and serum IL-1β and IL-10 concentrations, than the T2DM group (P < 0.05).
Table 1. Demographic and biochemical parameters of the studied population with and without T2DM and T2DN.
Table 2 shows the results of logistic regression analysis to identify predictors for T2DN. Single-factor logistic regression analysis showed that BMI, a history of hypertension, FPG, HbA1c, and the serum TC, IL-6, and IL-10 concentrations were risk factors; whereas, the serum Zn and Mg concentrations and eGFR were protective. Using these findings, we conducted multiple factor analysis, which showed that FPG and IL-10 are risk factors for T2DN, and that Zn is protective. These three factors were considered independent predictors of T2DN using a model adjusted for a history of hypertension.
Table 2. Predictors of DN identified by logistic regression analysis, adjusted for a history of hypertension.
Table 3 shows the results of an evaluation of possible predictors of T2DN. The sensitivity and specificity for the prediction of T2DN using serum Zn concentration were 84.3 and 95%, respectively; those for the prediction of T2DN using FPG concentration were 82.9 and 78.9%; and those for the prediction of T2DN using IL-10 were 70.7 and 78.9%. Figures 1–3 show the receiver operating characteristic (ROC) curves for the prediction of T2DN using serum Zn, FPG, and IL-10, respectively.
Table 4 shows that there were no significant differences in sex ratio; history of smoking; BMI; or the serum Mg, IL-1β, IL-12, TNF-α, or CRP concentrations between the T2DM and T2DN subgroups. The macroalbuminuria and renal failure groups had higher prevalences of a history of hypertension, and higher IL-6 and IL-8 concentrations, but lower serum Zn, 24 h urine Zn loss, 24 h urine Mg loss, and eGFR than the normal and microalbuminuria groups (P < 0.05). In addition, the microalbuminuria and renal failure groups had lower IL-10 concentrations than the normal group (P < 0.05), and the microalbuminuria group also had a lower IL-10 concentration than the macroalbuminuria group (P < 0.05).
Table 5 lists the correlations between Zn concentration and indices of clinical parameters and renal function in patients with T2DN. There were positive correlations between serum Zn and 24 h urine Zn, history of hypertension, and eGFR (P < 0.05), and negative correlations between a history of smoking, SCr, and ACR and 24 h urine albumin loss (P < 0.05).
Table 5. Correlation coefficients between Zn and clinical parameters and indices of renal function in DN patients.
Table 6 shows the correlations between serum Mg concentration and indices of clinical parameters and renal function in patients with T2DN. There were positive correlations between serum Mg and a history of hypertension and smoking. Serum Mg concentration was not related to renal function in patients with T2DN. However, there were positive correlations between 24 h urine Mg concentration and eGFR and a history of hypertension (P < 0.05) and a negative correlation between 24 h urine Mg concentration and ACR and a history of smoking (P < 0.05).
Table 6. Correlation coefficients between Zn and clinical characteristics and indices of renal function in DN patients.
Table 7 shows that there were negative correlations of serum Zn concentration with IL-6 and IL-8 (P < 0.05), and of 24 h urine Zn loss with IL-6 and IL-8 (P < 0.05). Table 8 shows that there were no correlations between Mg concentration and serum pro-inflammatory cytokine concentrations in patients with T2DN.
Although trace elements are found only in small quantities in the human body, they are extremely important for human health. A previous study of the relationship between DM and trace elements showed that appropriate homeostasis of trace elements assists with the control of blood glucose and slows tissue damage (18). The trace elements Zn and Mg are known to influence the development of diabetes and its complications. Zn is involved in the production, storage, and secretion of insulin by islet β-cells, and protects vascular endothelial integrity via its anti-oxidative, anti-apoptotic, and membrane stabilizing effects (19). In addition, Mg plays an important role in glucose homeostasis and the maintenance of insulin bioactivity (20).
Zn homeostasis is associated with DN, and in the present study we found that the serum Zn concentration of patients with DN is significantly lower than that of healthy individuals. Logistic regression and ROC analysis were used to identify predictors of DN, and both methods showed that serum Zn concentration is an independent and specific predictor of DN, which is a similar finding to that of Luo et al. (12).
We also found that in patients with macroalbuminuria or renal failure, the serum Zn concentration was significantly lower than in patients with T2DM but not DN, and in those with microalbuminuria. Furthermore, the serum Zn concentration in patients with DN negatively correlated with 24 h urinary albumin loss (r = −0.573); and the serum concentrations of IL-6 (r = −0.446) and IL-8 (r = −0.514). This suggests that Zn homeostasis is associated not only with DN-associated renal functional damage, but also with inflammatory responses in patients with DN. Similarly, in previous studies, Al-timimi et al. (21) found that serum Zn concentration decreases in patients with DN and that this is accompanied by a gradual decrease in eGFR and an increase in albuminuria. Furthermore, Zhang et al. (22) found that dietary Zn restriction significantly reduced the Zn concentrations in the plasma and kidneys of mice, and exacerbated tubulointerstitial fibrosis in diabetic mice. Khan et al. (23) demonstrated that dietary Zn supplementation reduces urinary albumin loss in patients with T2DM and DN, and Tang et al. (24) showed that dietary Zn supplementation prevents diabetes-related renal fibrosis and reduces 24 h urinary protein loss.
Previous studies have also shown that Zn administration reduces NF-κB activation and ameliorates vascular endothelial cell dysfunction (25). NF-κB is an important pro-inflammatory mediator in patients with DN, with its activation resulting in the production of a variety of pro-inflammatory cytokines, the proliferation, and hypertrophy of mesangial cells, and tubulointerstitial fibrosis (26). Specifically, NF-κB activation results in greater release of TNF-α, IL-6, and IL-8, and in turn, TNF-α increases the production of IL-1, IL-6, IL-8, and IL-18 (27). Three mechanisms have been suggested for the downregulation of NF-κB by Zn. Firstly, Zn may act as an immunomodulator to inhibit nucleotide phosphodiesterase, leading to an increase in cyclic-guanosine monophosphate concentration and the activation of protein kinase A, which prevents NF-κB entry into the nucleus and inhibits the phosphorylation of IκB kinase (IKK), and thereby NF-κB target gene transcription (28, 29). Secondly, zinc-regulated transporters, iron-regulated transporter-like protein-8 (ZIP8) may be involved. These are NF-κB targets that are significantly upregulated/ in inflammation. ZIP8 increases the intracellular concentration of Zn by importing it from the extracellular fluid and promoting its release from intracellular depots. Zn directly inhibits IKK via the thiol reaction. Thus, Zn can negatively regulate NF-κB pathways (30–32). Thirdly, serum Zn affects the expression of the Zn-finger protein A20. In the TNF receptor and toll-like receptor signaling pathways, A20 prevents IKK phosphorylation, inhibits NF-κB activation, and also acts on key upstream molecules that inhibit inflammatory signaling (11, 33). The regulation of ubiquitin-dependent signaling by A20 is dependent on serum Zn concentration. Prasad et al. (34) has shown that in monocytes, endothelial cells, and cancer cells, A20 expression is affected by serum Zn concentration, and Li et al. (35) found that Zn stimulates A20 transcription by causing epigenetic modification of the A20 promoter. Finally, Zn acts as a free radical scavenger, thereby contributing to the stability of A20 (11, 36). Thus, in summary, Zn homeostasis is closely related to the inflammatory response and the progression of DN, but the specific mechanisms involved require further study.
In the present study, we found that the urine Zn concentration of patients with DN is higher than that of healthy individuals, which may at least in part explain the low serum Zn concentration in patients with DN. This high urine zinc concentration may be related to polyuria, the blood glucose concentration, glycosuria, and/or proteinuria. With the progression of DN, the urine Zn concentrations gradually decreased, and when patients with DN had an eGFR <15 ml min−1 1.73 m−2, this decrease became significant. This may be explained by a decrease in urine volume or even anuria in patients with renal failure, and abnormal Zn metabolism is more common in patients at this stage of progression of DN.
DN is often associated with Mg deficiency. In the present study, we also found that the serum Mg concentration of patients with T2DM and DN was significantly lower than that in healthy individuals. A recent study found that hypomagnesemia is associated with a high incidence of DN and is an independent predictor of DN (16), and others have shown that hypomagnesemia is associated with both the development and progression of DN (37, 38).
Mg is an important cofactor and activator of many enzymes that are involved in glucose metabolism. In the absence of Mg, these enzymes cannot utilize high-energy phosphate bonds, which prevents phosphorylation, thereby directly affecting carbohydrate metabolism (39). Mg deficiency adversely affects cell homeostasis and may cause endothelial dysfunction and thrombosis by increasing platelet aggregation and vascular calcification (40). It can also lead to the excessive production of free radicals, which can damage mitochondria and change their permeability, which accelerates cellular necrosis and apoptosis (41), and can reduce the activity of antioxidant enzymes (42, 43), all of which are factors that facilitate the development of DN.
In the present study, there was no difference in the urine Mg concentration between patients with DN and healthy controls, which is not consistent with the results of previous studies (44). When healthy people eat a normal diet, about 30–50% of Mg can be absorbed, and the higher their energy intake, the larger the amount of Mg that is absorbed. Furthermore, dietary protein and amino acids also increase Mg absorption (45). Thus, long-term dietary therapy in DN may reduce Mg intake. Furthermore, gastroparesis and diarrhea caused by diabetic autonomic neuropathy can also reduce Mg absorption in the gastrointestinal tract (46). Normally, the kidneys are important regulators of Mg homeostasis, and the renal excretion of Mg largely depends on the serum Mg concentration (47). We found that serum Mg concentration was lower in patients with DN. To maintain serum Mg homeostasis, the kidney reduces urinary Mg excretion. However, in the present study, we found no difference in urine Mg concentration between healthy controls and patients with DN. Therefore, urinary Mg excretion in patients with DN would appear to be affected by many factors and may not be an accurate guide to overall Mg status. Thus, patients with DN should be considered Mg deficient, even if their urine Mg concentrations are normal. With the progression of DN, urinary Mg excretion decreased gradually in the present study. This may be because in the early stages of DN, the eGFR is normal; there may even be hyperfiltration, but with the progression of DN the compensation for renal dysfunction becomes insufficient, and the urine Mg concentration decreases with the volume of urine excreted, such that ultimately urine Mg is significantly reduced. Future studies will be directed toward collecting more information on the daily dietary habits of patients with DN and exploring the metabolism and distribution of trace elements Mg and Zn in DN patients, such as their cellular concentrations, as well as the concentrations of Mg and Zn in their diets. This will contribute to the development of appropriate evaluation indicators of the precise levels of trace elements in DN patients.
In summary, in the present study, we have demonstrated that the serum concentrations of the trace elements Zn and Mg in patients with T2DM and DN are significantly lower than those of healthy individuals. Serum Zn was found to be an independent predictor of DN and to have a protective role. Furthermore, the serum Zn, urine Zn, and urine Mg concentrations were found to decrease with the progression of DN-associated renal functional damage. Hypozincemia may be associated with DN-associated renal functional damage because it exacerbates renal inflammation. This study has several limitations. First, because it was a cross-sectional study, it was not possible to determine whether hypozincemia was a pathogenic factor or solely a risk biomarker. Second, due to time constraints, the sample size in our study was too small. In future studies, we will increase the sample size to improve the accuracy of the results.
The original contributions presented in the study are included in the article/supplementary material, further inquiries can be directed to the corresponding author/s.
The studies involving human participants were reviewed and approved by Ethics committee of the First Hospital of Jilin University. The patients/participants provided their written informed consent to participate in this study.
WS, JF, and YJ designed the experiments. WS and JF wrote the paper. JF, HW, ZJ, YW, WW, and WS performed the experiments and collected and analyzed the data. All authors read and approved the final manuscript.
The study was supported by the 13th Five-Year Plan for Scientific Research of the Jilin Provincial Education Department (Grant no. JJKH20180210KJ to WS) and the Natural Science Foundation of Jilin Province (Grant no. 20200201428JC to WS). Both funding bodies funded the study design, and the collection, analysis, and interpretation of the data, as well as the writing of the manuscript.
The authors declare that the research was conducted in the absence of any commercial or financial relationships that could be construed as a potential conflict of interest.
Zn, Zinc; Mg, magnesium; OS, oxidative stress; DN, diabetic nephropathy; T2DM, type 2 diabetes mellitus; IL, interleukin; TNF-α, tumor necrosis factor-α; NF-κB, nuclear factor kappa-B; ACR, albumin/creatinine ratio; FPG, fasting plasma glucose; eGFR, estimated glomerular filtration rate; BUN, blood urea nitrogen; Scr, serum creatinine; TC, total cholesterol; TG, triglyceride; IKK, IκB kinase; ZIP8, zinc-regulated transporters, iron-regulated transporter-like protein-8.
1. Migdalis I, Leslie D, Mavrogiannaki A, Papanas N, Valensi P, Vlassara H. Diabetes mellitus 2014. Int J Endocrinol. (2015) 2015:845759. doi: 10.1155/2015/845759
2. Meza Letelier CE, San Martín Ojeda CA, Ruiz Provoste JJ, Frugone Zaror CJ. Pathophysiology of diabetic nephropathy: a literature review. Medwave. (2017) 17:e6839. doi: 10.5867/medwave.2017.01.6839
3. Jha JC, Banal C, Chow BS, Cooper ME, Jandeleit-Dahm K. Diabetes and kidney disease: role of oxidative stress. Antioxid Redox Signal. (2016) 25:657–684. doi: 10.1089/ars.2016.6664
4. Zha F, Qu X, Tang B, Li J, Wang Y, Zheng P, et al. Long non-coding RNA MEG3 promotes fibrosis and inflammatory response in diabetic nephropathy via miR-181a/Egr-1/TLR4 axis. Aging (Albany NY). (2019) 11:3716–30. doi: 10.18632/aging.102011
5. Rabbani N, Thornalley PJ. Advanced glycation end products in the pathogenesis of chronic kidney disease. Kidney Int. (2018) 93:803–13. doi: 10.1016/j.kint.2017.11.034
6. Bermejo S, García CO, Rodríguez E, Barrios C, Otero S, Mojal S, et al. The renin-angiotensin-aldosterone system blockade in patients with advanced diabetic kidney disease. Nefrologia. (2018) 38:197–206. doi: 10.1016/j.nefroe.2017.12.002
7. Bao L, Li J, Zha D, Zhang L, Gao P, Yao T, et al. Chlorogenic acid prevents diabetic nephropathy by inhibiting oxidative stress and inflammation through modulation of the Nrf2/HO-1 and NF-κB pathways. Int Immunopharmacol. (2018) 54:245–53. doi: 10.1016/j.intimp.2017.11.021
8. Tong Y, Liu S, Gong R, Zhong L, Duan X, Zhu Y. Ethyl vanillin protects against kidney injury in diabetic nephropathy by inhibiting oxidative stress and apoptosis. Oxid Med Cell Longev. (2019) 2019:2129350. doi: 10.1155/2019/2129350
9. Gammoh NZ, Rink L. Zinc in infection and inflammation. Nutrients. (2017) 9:624. doi: 10.20944/preprints201705.0176.v1
10. Feng J, Wang H, Jing Z, Wang Y, Cheng Y, Wang W, et al. Role of magnesium in Type 2 diabetes mellitus. Biol Trace Elem Res. (2020) 196:74–85. doi: 10.1007/s12011-019-01922-0
11. Jarosz M, Olbert M, Wyszogrodzka G, Młyniec K, Librowski T. Antioxidant and anti-inflammatory effects of zinc. Zinc-dependent NF-κB signaling. Inflammopharmacology. (2017) 25:11–24. doi: 10.1007/s10787-017-0309-4
12. Luo YY, Zhao J, Han XY, Zhou XH, Wu J, Ji LN. Relationship between serum zinc level and microvascular complications in patients with type 2 diabetes. Chin Med J (Engl). (2015) 128:3276–82. doi: 10.4103/0366-6999.171357
13. Barman S, Pradeep SR, Srinivasan K. Zinc supplementation alleviates the progression of diabetic nephropathy by inhibiting the overexpression of oxidative-stress-mediated molecular markers in streptozotocin-induced experimental rats. J Nutr Biochem. (2018) 54:113–29. doi: 10.1016/j.jnutbio.2017.11.008
14. Li B, Cui W, Tan Y, Luo P, Chen Q, Zhang C, et al. Zinc is essential for the transcription function of Nrf2 in human renal tubule cells in vitro and mouse kidney in vivo under the diabetic condition. J Cell Mol Med. (2014) 18:895–906. doi: 10.1111/jcmm.12239
15. Golshani-Hebroni S. Mg(++) requirement for MtHK binding, and Mg(++) stabilization of mitochondrial membranes via activation of MtHK & MtCK and promotion of mitochondrial permeability transition pore closure: a hypothesis on mechanisms underlying Mg(++)'s antioxidant and cytoprotective effects. Gene. (2016) 581:1–13. doi: 10.1016/j.gene.2015.12.046
16. Bherwani S, Jibhkate SB, Saumya AS, Patel SK, Singh R, Ghotekar LH. Hypomagnesaemia: a modifiable risk factor of diabetic nephropathy. Horm Mol Biol Clin Investig. (2017) 29:79–84. doi: 10.1515/hmbci-2016-0024
17. Lin CY, Tsai PS, Hung YC, Huang CJ. L-type calcium channels are involved in mediating the anti-inflammatory effects of magnesium sulphate. Br J Anaesth. (2010) 104:44–51. doi: 10.1093/bja/aep336
18. Siddiqui K, Bawazeer N, Joy SS. Variation in macro and trace elements in progression of type 2 diabetes. ScientificWorldJournal. (2014) 2014:461591. doi: 10.1155/2014/461591
19. Nazarizadeh A, Asri-Rezaie S. Comparative study of antidiabetic activity and oxidative stress induced by zinc oxide nanoparticles and zinc sulfate in diabetic rats. AAPS PharmSciTech. (2016) 17:834–3. doi: 10.1208/s12249-015-0405-y
20. Gröber U, Schmidt J, Kisters K. Magnesium in prevention and therapy. Nutrients. (2015) 7:8199–226. doi: 10.3390/nu7095388
21. Al-Timimi DJ, Sulieman DM, Hussen KR. Zinc status in type 2 diabetic patients: relation to the progression of diabetic nephropathy. J Clin Diagn Res. (2014) 8:CC04–8. doi: 10.7860/JCDR/2014/10090.5082
22. Zhang X, Liang D, Lian X, Chi ZH, Wang X, Zhao Y, et al. Effect of zinc deficiency on mouse renal interstitial fibrosis in diabetic nephropathy. Mol Med Rep. (2016) 14:5245–52. doi: 10.3892/mmr.2016.5870
23. Khan MI, Siddique KU, Ashfaq F, Ali W, Reddy HD, Mishra A. Effect of high-dose zinc supplementation with oral hypoglycemic agents on glycemic control and inflammation in type-2 diabetic nephropathy patients. J Nat Sci Biol Med. (2013) 4:336–40. doi: 10.4103/0976-9668.117002
24. Tang Y, Yang Q, Lu J, Zhang X, Suen D, Tan Y, et al. Zinc supplementation partially prevents renal pathological changes in diabetic rats. J Nutr Biochem. (2010) 21:237–46. doi: 10.1016/j.jnutbio.2008.12.010
25. Voelkl J, Tuffaha R, Luong TTD, Zickler D, Masyout J, Feger M, et al. Zinc inhibits phosphate-induced vascular calcification through TNFAIP3-mediated suppression of NF-κB. J Am Soc Nephrol. (2018) 29:1636–48. doi: 10.1681/ASN.2017050492
26. Opazo-Ríos L, Plaza A, Sánchez Matus Y, Bernal S, Lopez-Sanz L, Jimenez-Castilla L, et al. Targeting NF-κB by the cell-permeable NEMO-binding domain peptide improves albuminuria and renal lesions in an experimental model of type 2 diabetic nephropathy. Int J Mol Sci. (2020) 21:4225. doi: 10.3390/ijms21124225
27. Rehman K, Akash MS. Mechanisms of inflammatory responses and development of insulin resistance: how are they interlinked? J Biomed Sci. (2016) 23:87. doi: 10.1186/s12929-016-0303-y
28. von Bülow V, Dubben S, Engelhardt G, Hebel S, Plümäkers B, Heine H, et al. Zinc-dependent suppression of TNF-alpha production is mediated by protein kinase A-induced inhibition of Raf-1, I kappa B kinase beta, NF-kappa B. J Immunol. (2007) 179:4180–6. doi: 10.4049/jimmunol.179.6.4180
29. Mulero MC, Huxford T, Ghosh G. NF-κB, IκB, and IKK: integral components of immune system signaling. Adv Exp Med Biol. (2019) 1172:207–26. doi: 10.1007/978-981-13-9367-9_10
30. Lehvy AI, Horev G, Golan Y, Glaser F, Shammai Y, Assaraf YG. Alterations in ZnT1 expression and function lead to impaired intracellular zinc homeostasis in cancer. Cell Death Discov. (2019) 5:144. doi: 10.1038/s41420-019-0224-0
31. Geng X, Liu L, Banes-Berceli A, Yang Z, Kang P, Shen J, et al. Role of ZIP8 in regulating cell morphology and NF-κB/Snail2 signaling. Metallomics. (2018) 10:953–64. doi: 10.1039/C8MT00079D
32. Liu MJ, Bao S, Gálvez-Peralta M, Pyle CJ, Rudawsky AC, Pavlovicz RE, et al. ZIP8 regulates host defense through zinc-mediated inhibition of NF-κB. Cell Rep. (2013) 3:386–400. doi: 10.1016/j.celrep.2013.01.009
33. Lork M, Verhelst K, Beyaert R. CYLD A20 and OTULIN deubiquitinases in NF-κB signaling and cell death: so similar, yet so different. Cell Death Differ. (2017) 24:1172–83. doi: 10.1038/cdd.2017.46
34. Prasad AS, Bao B, Beck FW, Sarkar FH. Zinc-suppressed inflammatory cytokines by induction of A20-mediated inhibition of nuclear factor-κB. Nutrition. (2011) 27:816–23. doi: 10.1016/j.nut.2010.08.010
35. Li C, Guo S, Gao J, Guo Y, Du E, Lv Z, et al. Maternal high-zinc diet attenuates intestinal inflammation by reducing DNA methylation and elevating H3K9 acetylation in the A20 promoter of offspring chicks. J Nutr Biochem. (2015) 26:173–83. doi: 10.1016/j.jnutbio.2014.10.005
36. Catrysse L, Vereecke L, Beyaert R, van Loo G. A20 in inflammation and autoimmunity. Trends Immunol. (2014) 35:22–31. doi: 10.1016/j.it.2013.10.005
37. Zhang Y, Li Q, Xin Y, Lv W, Ge C. Association between serum magnesium and common complications of diabetes mellitus. Technol Health Care. (2018) 26:379–87. doi: 10.3233/THC-174702
38. Prabodh S, Prakash DS, Sudhakar G, Chowdary NV, Desai V, Shekhar R. Status of copper and magnesium levels in diabetic nephropathy cases: a case-control study from South India. Biol Trace Elem Res. (2011) 142:29–35. doi: 10.1007/s12011-010-8750-x
39. Mooren FC. Magnesium and disturbances in carbohydrate metabolism. Diabetes Obes Metab. (2015) 17:813–23. doi: 10.1111/dom.12492
40. Sakaguchi Y, Hamano T, Isaka Y. Magnesium and progression of chronic kidney disease: benefits beyond cardiovascular protection? Adv Chronic Kidney Dis. (2018) 25:274–80. doi: 10.1053/j.ackd.2017.11.001
41. Liu M, Jeong EM, Liu H, Xie A, So EY, Shi G, et al. Magnesium supplementation improves diabetic mitochondrial and cardiac diastolic function. JCI Insight. (2019) 4:e123182. doi: 10.1172/jci.insight.123182
42. Zhang Q, Zhou PH, Zhou XL, Zhang DL, Gu Q, Zhang SJ, et al. Effect of magnesium gluconate administration on lipid metabolism, antioxidative status, and related gene expression in rats fed a high-fat diet. Magnes Res. (2018) 31:117–30. doi: 10.1186/s12944-018-0815-4
43. Kachhawa K, Kachhawa P, Agrawal D, Kumar S, Sarkar PD. Effects and association of pro-oxidants with magnesium in patients with diabetic nephropathy. Saudi J Kidney Dis Transpl. (2019) 30:1032–1037. doi: 10.4103/1319-2442.270257
44. Felsenfeld AJ, Levine BS, Rodriguez M. Pathophysiology of calcium, phosphorus, and magnesium dysregulation in chronic kidney disease. Semin Dial. (2015) 28:564–77. doi: 10.1111/sdi.12411
45. Saris NE, Mervaala E, Karppanen H, Khawaja JA, Lewenstam A. Magnesium. An update on physiological, clinical and analytical aspects. Clin Chim Acta. (2000) 294:1–26. doi: 10.1016/S0009-8981(99)00258-2
46. Boulton AJ, Vinik AI, Arezzo JC, Bril V, Feldman EL, Freeman R, et al. Diabetic neuropathies: a statement by the American Diabetes Association. Diabetes Care. (2005) 28:956–62. doi: 10.2337/diacare.28.4.956
Keywords: type 2 diabetes mellitus, diabetic nephropathy, trace element, zinc, magnesium, pro-inflammatory cytokine
Citation: Feng J, Wang H, Jing Z, Wang Y, Wang W, Jiang Y and Sun W (2021) Relationships of the Trace Elements Zinc and Magnesium With Diabetic Nephropathy-Associated Renal Functional Damage in Patients With Type 2 Diabetes Mellitus. Front. Med. 8:626909. doi: 10.3389/fmed.2021.626909
Received: 07 November 2020; Accepted: 09 March 2021;
Published: 30 March 2021.
Edited by:
Nehal Mohsen Elsherbiny, Mansoura University, EgyptCopyright © 2021 Feng, Wang, Jing, Wang, Wang, Jiang and Sun. This is an open-access article distributed under the terms of the Creative Commons Attribution License (CC BY). The use, distribution or reproduction in other forums is permitted, provided the original author(s) and the copyright owner(s) are credited and that the original publication in this journal is cited, in accordance with accepted academic practice. No use, distribution or reproduction is permitted which does not comply with these terms.
*Correspondence: Yanfang Jiang, eWFuZmFuZ2ppYW5nQGhvdG1haWwuY29t; Weixia Sun, c3Vud2VpeGlhXzc4QDE2My5jb20=
Disclaimer: All claims expressed in this article are solely those of the authors and do not necessarily represent those of their affiliated organizations, or those of the publisher, the editors and the reviewers. Any product that may be evaluated in this article or claim that may be made by its manufacturer is not guaranteed or endorsed by the publisher.
Research integrity at Frontiers
Learn more about the work of our research integrity team to safeguard the quality of each article we publish.