- 1CEiiA-Centre of Engineering and Product Development, Matosinhos, Portugal
- 2Serviço de Anestesiologia, Centro Hospitalar Universitário Do Porto, Porto, Portugal
- 3Departamento de Cirurgia, Centro Hospitalar Universitário Do Porto, Porto, Portugal
- 4Center for Innovation, Technology and Policy Research (IN+), Instituto Superior Técnico, University of Lisbon, Lisbon, Portugal
- 5Life and Health Sciences Research Institute, School of Medicine, University of Minho, Braga, Portugal
- 6Instituto Ciências da Vida e da Saúde in Portuguese (ICVS)/3B's-PT Government Associate Laboratory, Braga, Portugal
- 7Faculty of Health Sciences, University Fernando Pessoa, Porto, Portugal
- 8Institute for Research and Innovation in Health, University of Porto, Porto, Portugal
- 9Centro Hospitalar de Trás-os-Montes e Alto Douro, Vila Real, Portugal
- 10Clinical Academic Center, Hospital of Braga, Braga, Portugal
Background: The urgent need for mechanical ventilators to support respiratory insufficiency due to SARS-CoV-2 led to a worldwide effort to develop low-cost, easily assembled, and locally manufactured ventilators. The ATENA ventilator project was developed in a community-based approach targeting the development, prototyping, testing, and decentralized manufacturing of a new mechanical ventilator.
Objective: This article aims to demonstrate ATENA's adequate performance and safety for clinical use.
Material: ATENA is a low-cost ventilator that can be rapidly manufactured, easily assembled, and locally produced anywhere in the world. It was developed following the guidelines and requirements provided by European and International Regulatory Authorities (MHRA, ISO 86201) and National Authorities (INFARMED). The device was thoroughly tested using laboratory lung simulators and animal models.
Results: The device meets all the regulatory requirements for pandemic ventilators. Additionally, the pre-clinical experiences demonstrated security and adequate ventilation and oxygenation, in vivo.
Conclusion: The ATENA ventilator had a good performance in required tests in laboratory scenarios and pre-clinical studies. In a pandemic context, ATENA is perfectly suited for safely treating patients in need of mechanical ventilation.
Introduction
The global pandemic Coronavirus Disease 2019 (COVID-19) caused by SARS-CoV-2 created an urgent need for mechanical ventilators around the world (1). Even though the majority of patients develop mild (40%) or moderate (40%) symptoms, ~15% develop a severe condition that requires oxygen support and 5% end up with respiratory failure and need intensive care admission (2–4).
The exponential pattern of viral transmission led to a rapid and overwhelming increase in hospitalizations and overflow to intensive care units for invasive ventilation. At the beginning of 2020, the number of available ventilators was scarce and contributed to a significant increase in morbimortality worldwide (5–7). The fight against the global COVID-19 pandemic required innovative actions. Globally, thousands of experts, companies, and volunteers worked to fill the global shortage of commercial ventilators, by developing open-source ventilators or finding strategies (8–12) for shared ventilation (13, 14). Likewise, in Portugal, CEiiA—an Engineering and Product Development Centre—led (15) the development of the ATENA ventilator in a community-based approach.
ATENA is a rapidly manufactured, low-cost, easily assembled, and locally produced mechanical invasive ventilator. It was developed in a short time, from design to prototype. The requirements for the ATENA ventilator were common to other proposals (11, 16): (i) easily sourced components available to the general public; (ii) “open-source” compatibility, namely, availability of design and easiness to replicate; and (iii) high accuracy in a range of ventilation strategies that allow high airway pressures for ARDS patients.
This paper aims to demonstrate the performance and safety of ATENA as a pandemic ventilator adequate for COVID-19 patients, following the requirements of different regulatory agencies.
Materials and Methods
ATENA Design and Systems Breakdown
Overview
ATENA is a pneumatic ventilator, requiring high-pressure air and oxygen supplies to drive the respiratory cycles. Its control system hardware was locally designed, based on commercially available and inexpensive components. The software was developed in-house.
The main body is built using a stainless-steel wheeled structure and four sealed metal industrial boxes. These boxes are attached on each side of the structure (see Figure 1). They contain the pneumatic, electrical, and control modules. ATENA's overall specifications are described in Table 1 (see also Supplementary Table 1).
The two upper boxes contain the respiratory circuit, with the required valves and sensors, and the control system. Gases in the respiratory circuit are at low pressure (<60 cmH2O). One of the lower housing boxes handles the input of air and oxygen at high pressure (>3 bar). The other contains the power module, composed of a battery, transformers, and safety fuses and breakers.
A touch screen displays pressure, flow, and volume curves in addition to other important ventilation variables (see Figures 3A,C). Ventilation modes and parameters are configurable via the touch screen (Figure 3B). Operation is started/stopped by pressing a physical button on the front of the ventilator.
Alarms are signaled by sound, light, and a specific alarm text on the display (see the top bar in Figure 3A). Alarms need to be acknowledged and muted by pressing another physical button.
Operation
Ventilation mode and parameters are configured via the touch display. Figure 3B shows the VCV (volume-controlled ventilation) mode configuration screen. Other modes are visible as extra tabs. Once the operation mode is selected and configured, ventilation can be started by pressing the START/STOP button next to the display.
Air and oxygen enter the system at a pressure of about 4.5 bar (air and O2 inlets are indicated in Figure 2). To ensure adequate availability of oxygen for each inhalation, we installed a 0.75-L buffer tank after the oxygen inlet. Pressure is monitored via pressure sensors in each of the high-pressure circuits to detect any fault in the gas supply. Such faults trigger an alarm. These pressures can be checked on the ventilator display (Figure 3C).
Piezo-electric valves on the air and oxygen lines control the flow and oxygen content of the mixture delivered to the patient. The control system uses two flow sensors, one for each gas, for feedback control loops. An oxygen cell measuring the fraction of inspired oxygen (FiO2) allows for mixture correction. Data from pressure sensors on both inspiratory and expiratory circuits are used to estimate the airway opening pressure. Depending on the mode of ventilation, the control system will deliver a constant flow, or variable flow, to reach a set pressure. All the aforementioned sensed variables are shown on the ventilator display (Figures 3A,C).
The gas mixture leaves the ventilator through a 22-mm tube outlet, compatible with standard medical tracheas, check valves, and filters. Additionally, two safety mechanisms prevent exposure to overpressure (manually adjustable pressure relief valve, maximum 60 cmH2O) and suffocation (one-way under-pressure valve). The connection ports for the overpressure and anti-suffocation valves are also standard 22-mm tubes. Under normal operating conditions, the control system will prevent those conditions; the safety mechanisms are redundant systems.
To allow for both an unobstructed exhalation and fine control of flow during the PEEP phase, we selected a pneumatically controlled pinch valve. This valve has a large internal diameter, capable of large flows even in low-pressure conditions. During inhalation, the exhalation valve is closed. At the start of the exhalation, the valve fully opens until the pressure reaches the configured PEEP value. While keeping the PEEP, the exhalation valve is slightly open so that a small bias flow is present. This bias flow is useful for detecting an attempt by the patient to initiate a breath. A flow sensor in the exhalation circuit, in combination with both flow sensors in the inhalation circuit, lets us estimate the actual flow from/to the patient.
Spontaneous breaths are detected by both a variation in pressure and a flow toward the patient during the exhalation period. We found this combined approach to be more robust than a single breath variable measurement.
Requirements and Specifications
ATENA was developed following the clinical requirements for the “minimally acceptable” performance of a mechanical ventilator aimed at the COVID-19 pandemic crisis. Those requirements and specifications were described in the Rapid Manufactured Ventilator System (RMVS) document by the UK Medicines & Healthcare products Regulatory Agency (MHRA) (17) and in the exceptional authorization by the National Authority for Drugs and Healthcare Products (deliberation of 29 June 2020, INFARMED) (18).
The ATENA ventilator can operate in continuous mandatory ventilation (pressure-regulated volume control and pressure- and volume-controlled modes) and in pressure support mode. ATENA's overall specifications and control ranges are presented in Table 1.
Testing Protocol
The main objective of the testing protocol was to demonstrate adequate safety and performance by the ATENA ventilator. We evaluated ATENA's accuracy and performance in five steps: (1) MHRA protocol, including endurance testing (17); (2) International Organization for Standardization (ISO 80601-2-12:2020) (19); (3) additional tests for extreme values of tidal volume, PEEP, and FiO2; (4) pressure support ventilation; and (5) pre-clinical studies.
ATENA was attached via the breathing circuit to a calibrated electronic gas flow analyzer ventilator tester (VT900A, Fluke Biomedical) and then to an adult test lung (ACCU LUNG™ Precision test, Fluke Biomedical) that simulates different respiratory systems with variable compliance (C) and resistance (R). The ventilator was also linked to an external computer that allowed the recording of pressure, flow, FiO2, and volume waveforms.
MHRA Protocol
The assessment of the performance of ATENA was done under controlled conditions following MHRA's RMVS protocol (17). We performed three sets of 36 trials for VC: (i) compliance, (ii) resistance, and (iii) tidal volume, and two sets of 36 trials for PC: (i) plateau pressure at 15 cmH2O and (ii) 30 cmH2O.
Concerning endurance testing, ATENA was connected to a passive lung simulator for 24 h. Ventilator settings at the beginning and the end of the test were reported, and the variation of the settings was analyzed.
Electromagnetic compatibility, electrical interference, emission, and immunity tests were made following European and international standards (EN 60601-1-2, EN 61000-6-1, EN 61000-4-4, EN 61000-4-6, EN 55011, and ISO 80601-2-12).
International Organization for Standardization
Simultaneously, the ATENA ventilator was submitted to ISO requirements testing for basic safety and essential performance of critical care ventilators (ISO 80601-2-12:2020) (19). For volume-controlled ventilation, seven tests were done with variable compliance and resistance lung models, using different tidal volume, PEEP, and FiO2. To evaluate the performance of pressure-controlled ventilation, seven tests were done also with different compliance and resistance levels, using different airway pressure, PEEP, and FiO2. Additionally, one set of 14 tests was done to assess ATENA's accuracy in the measurement of tidal volume.
Additional Tests
Following recommendations from INFARMED (19), ATENA performed an additional set of tests with a simulated ARDS lung model (C = 20 ml/cmH2O and R = 5 cmH2O/L/s). This allowed us to evaluate the accuracy of the ventilator with an extreme variation of tidal volume (250–800 ml) in VCV and of PEEP (0–40 cmH2O) in PCV modes. We ran an extra test with a healthy lung model, to analyze ATENA's accuracy on FiO2 control (21–100%). Those tests were done without pausing the ventilator, to evaluate ATENA's response to a change in configuration parameters. The same breathing simulator and gas flow analyzer were used.
Pressure Support Ventilation
To evaluate the performance of the pressure support ventilation mode, we used an ASL5000 breathing simulator (version 3.6, Active Servo Lung, IngMar Medical, Pittsburgh, PA, USA) with an active lung model in both healthy and ARDS conditions. The settings used for each healthy (C = 50 ml/cmH2O and R = 10 cmH2O/L/s) and ARDS patient (C = 30 ml/cmH2O and R = 20 cmH2O/L/s) were available from the simulator's patient library and were validated in previous studies (11, 20–22).
ASL5000 tested ATENA's (i) capacity to detect apnea and switch to backup controlled mandatory ventilation (pressure-controlled ventilation) and (ii) performance on the pressure support mode with increasing inspiratory muscle strength. Given that the ATENA ventilator has an inspiratory trigger flow with a sensitivity that varies between 0.2 SLPM (high sensitivity) and 3 SLPM (low sensitivity), we decided to test three trigger points (0.2, 1, and 2 SLPM). For both healthy and ARDS lung models, the same spontaneous breathing parameters were used (respiratory rate: 3/15 cpm; uncompensated residual capacity: 0.5 L; pause: 0%; inspiratory muscle pressure: 0/10/15 cmH2O; expiratory muscle pressure: 0 cmH2O; inspiratory rise time: 10%; inspiratory hold: 5%; inspiratory release time: 10%; expiratory rise time: 0%; expiratory hold: 0%; expiratory release time: 0%; effort: sinusoidal). A total of 21 tests were performed on pressure support of 10 cmH2O with a PEEP of 5 cmH2O for testing ATENA's capacity to support the weaning process from mechanical ventilation (23, 24).
Pre-Clinical Test
Pre-clinical tests were performed following the EU Directive 2010/63/EU, approved by the Animal Welfare Body (ORBEA EM/ICVS-I3Bs_005/2020) of the institution where the study was conducted (University of Minho) and by the national authority for animal protection—Direção Geral de Alimentação e Veterinária (DGAV 008337). Four porcine animals (Sus scrofa domesticus) (14 weeks; average weight 32 ± 3 kg) were used to evaluate ATENA's performance in vivo, with a special focus on blood gas exchange during the MRHA test protocol. Two animals were allocated to the volume-controlled (group 1), and two animals were allocated to the pressure-controlled (group 2) ventilation. Animals were anesthetized with an intramuscular administration of ketamine (20 mg/kg) and xylazine (2 mg/kg), followed by intravenous propofol administration (4 mg/kg). Total Intravenous Anesthesia (TIVA) was maintained with continuous propofol infusion (4.4 mg/kg) in combination with fentanyl (0.005 mg/kg/h) and midazolam (0.7 mg/kg/h) administered through a central venous catheter, together with the parenteral isotonic fluids administered for maintenance of water and electrolyte balance (6–10 ml/kg/h). Rocuronium (2.5 mg/kg/h) was administered to provide muscle relaxation and improve ventilator adaptation. After intubation, animals were adapted to the ATENA ventilator with a FiO2 of 60%. An invasive arterial line was achieved for a continuous hemodynamic (Combitrans Monitoring-Set arterial, BBraun, Germany) and blood gas (CG4+ cartridges, i-Stat analyzer, Abbott, Chicago, IL) monitoring.
In both groups, after 5 min of ventilation, arterial blood gas was collected to measure and record pH, PO2 and PCO2, and HCO3, and a new ventilatory setting was selected. At the end of the experiment, with the animals still under deep anesthesia, euthanasia was performed by an intravenous administration of pentobarbital (200 mg/kg), with veterinary support.
Results
MHRA Protocol
In volume-controlled ventilation, a triplet of 36 trials for compliance, resistance, and tidal volume were done. In pressure-controlled ventilation, the same number of tests were completed for PEEP, plateau pressure, and FiO2 for an inspiratory pressure of 15 cmH2O or 30 cmH2O.
The histograms in Figures 4, 5 represent the relative frequency of the error (%) in volume-controlled ventilation for different PEEP values (5, 10, and 15 cmH2O); tidal volume (300–500 ml) and FiO2 (55–95%) and pressure-controlled ventilation for different PEEP values (5, 10, and 15 cmH2O); and plateau pressure (15–30 cmH2O) and FiO2 (55–95%).
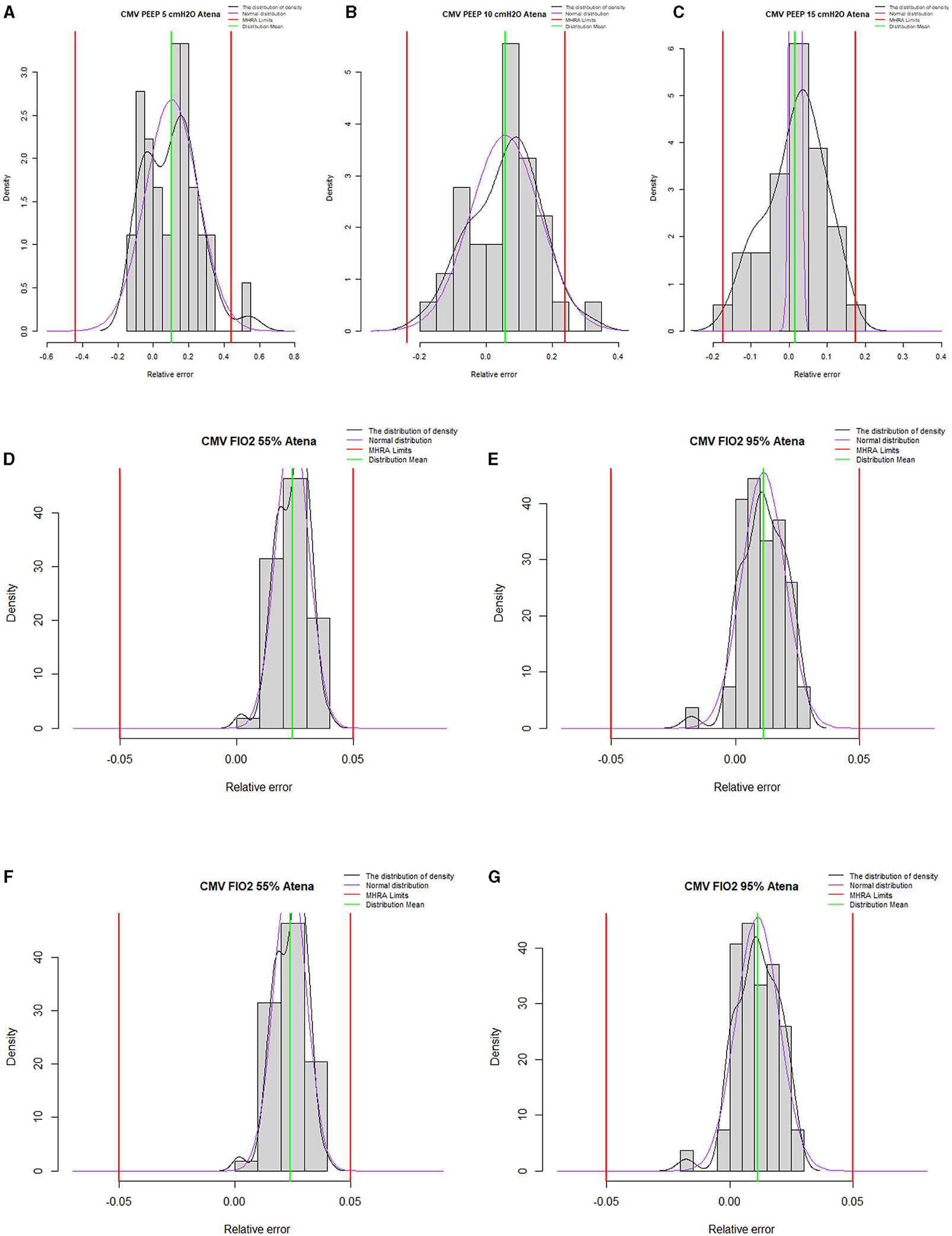
Figure 4. Summary histograms for all volume-controlled ventilation test conditions. (A–C) represent histograms for PEEP 5, 10, and 15, respectively. (D,E) represent histograms for tidal volume 500 and 300 ml, respectively. (F,G) represent histograms for 55 and 95% of FiO2. Outside the red limits data points out-of-performance.
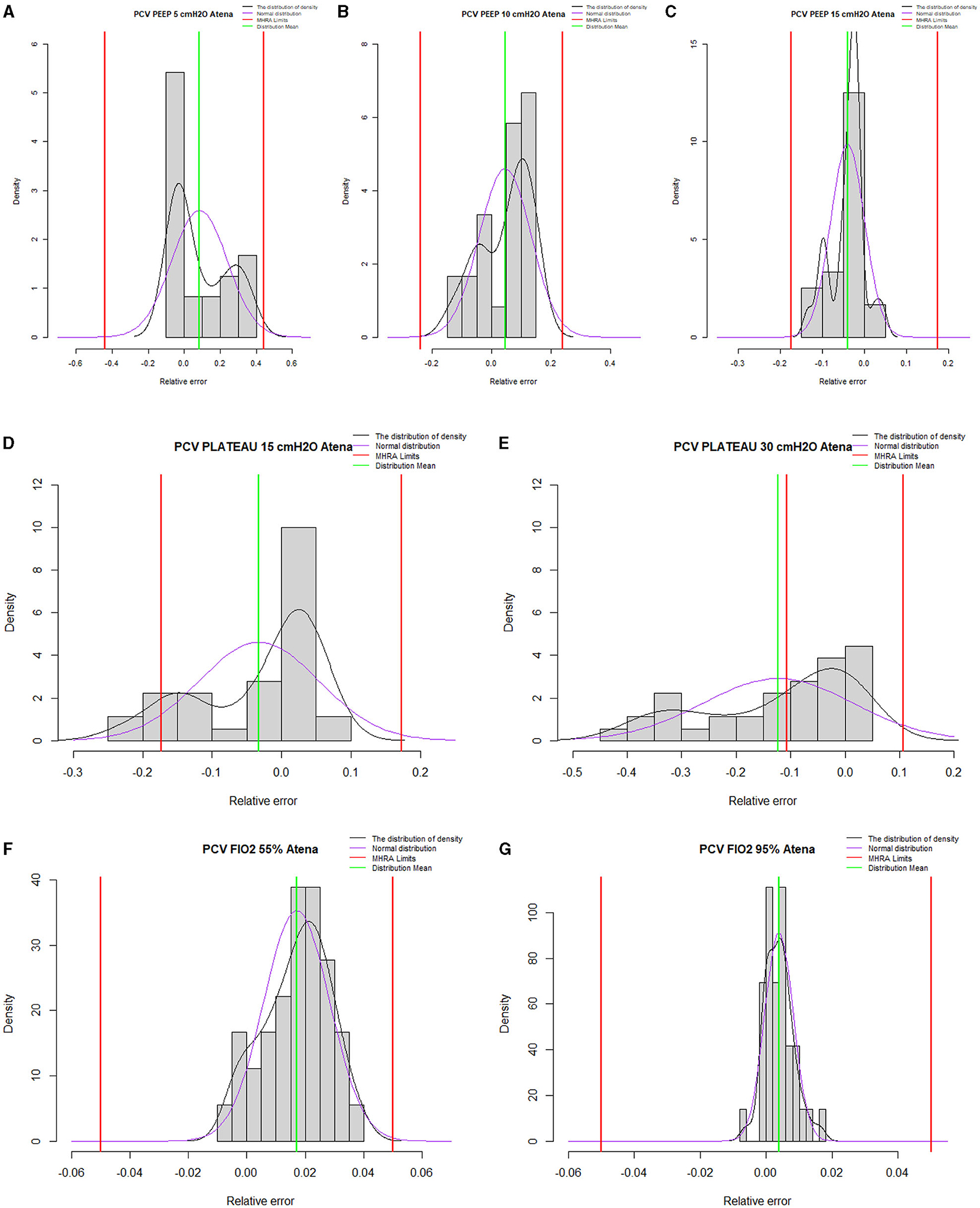
Figure 5. Summary histograms for all pressure-controlled ventilation test conditions. (A–C) represent histograms for PEEP 5, 10, and 15 cmH2O, respectively. (D,E) represent histograms for plateau pressure of 15 and 30 cmH2O, respectively. (F,G) represent histograms for 55 and 95% of FiO2. Outside the red limits data points out-of-performance.
Concerning endurance testing, ATENA ran uninterrupted for 24 h, which represents 28,800 cycles. In volume-controlled ventilation, the set at the beginning of the test was as follows: tidal volume of 500 ml, respiratory rate 20 breaths/min, PEEP 5 cmH2O, and FiO2 40%. The average of measured parameters in the first 13 min was a tidal volume of 512.73 ml, FiO2 = 39.81%, and PEEP = 4.66 cmH2O. In the last 13 min of the 24 h, the average of the values measured were as follows: tidal volume = 519.57 ml, O2 = 39.72%, and PEEP = 5.47 cmH2O at the final (time = 24 h).
For the pressure-controlled ventilation mode, a goal was set for the plateau pressure of 20 cmH2O, respiratory rate of 20 breaths/min, PEEP 5 cmH2O, and FiO2 40%. The average values measured after 13 min of ventilation were as follows: plateau pressure = 22.61 cmH2O, O2 = 39.81, and PEEP = 4.47 cmH2O. In the last 13 min of 24 h of ventilation, the average values were as follows: plateau pressure = 22.61 cmH2O, O2 = 40.3%, and PEEP = 4.81 cmH2O.
The relative error values measured between the initial and the last 13 min are tidal volume = 1.3%, FiO2 = 0.2%, and PEEP = 7.4% for controlled ventilation and plateau pressure = 0%, O2 = −1.2%, and PEEP = −7.6%.
In terms of electronic safety, the ATENA ventilator complies with the relevant requirements studied (EN 60601-1-2, EN 61000-6-1, EN 61000-4-4, EN 61000-4-6, EN 55011, and ISO 80601-2-12).
International Organization of Standards
ATENA fulfilled the following criteria for basic safety and essential performance of critical care ventilators (ISO 80601-2-12:2020):
• Maximum error of the delivered and monitored tidal volume compared to the set value: ± (4 ml + 15% of the set value);
• Maximum error of the PEEP compared to the set value: ± (2 cmH2O + 4% of the set value);
• Maximum error of FiO2 compared to the set value: ± (5% of the set value); and
• Maximum error of PPlateau compared to the set value: ± (2 cmH2O + 4% of the set value).
Relevant data from volume- and pressure-controlled ventilation, as well as expired volume, measured criteria are presented in Supplementary Tables 2, 3.
Additional Tests
The results of additional tests are presented in Figure 6. The data are presented and analyzed in terms of relative error between the values defined by the test conditions, configured in ATENA, and the values measured by the gas flow analyzer. The relationship is linear for all evaluated parameters (tidal volume, PEEP, FiO2, and PPlateau).
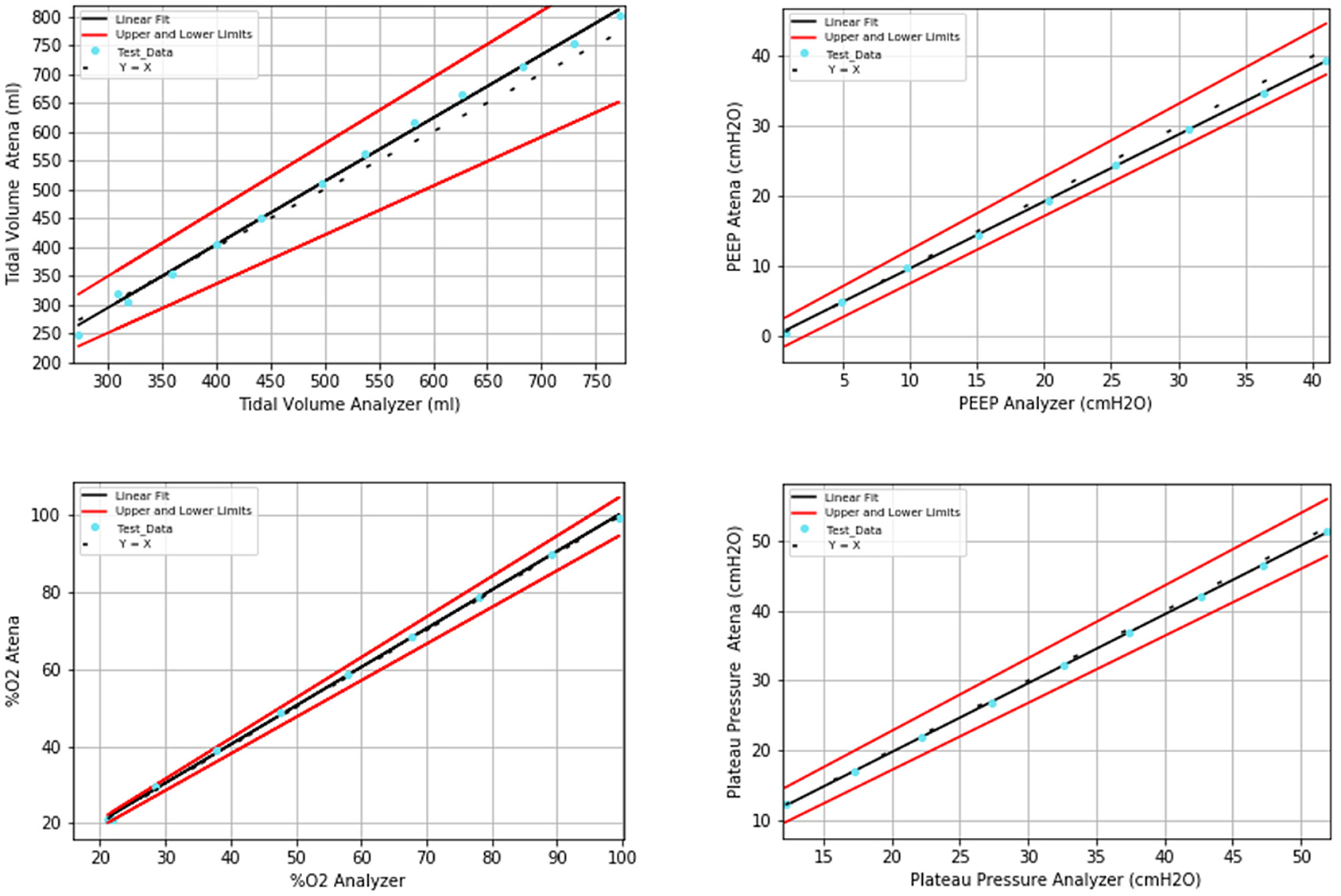
Figure 6. Linear-fit results from additional tests for a wide range of settings (tidal volume, PEEP, FiO2, and plateau pressure, respectively).
Pressure Support Ventilation
The results obtained in tests 1 to 21 show that ATENA had an adequate performance for all trigger levels, for all inspiratory muscle pressures used in both healthy and ARDS simulated lungs (see Supplementary Table 4).
Pre-Clinical Test
During the study period, all animals remained hemodynamically stable with no need for vasopressor support. Supplementary Tables 5, 6 display the relevant ventilatory settings and measures of ventilation variables in pre-clinical testing for volume- and pressure-controlled modes, respectively.
Discussion
Analysis of Results
Test results demonstrated that the ATENA ventilator met the specifications in the MHRA RMVS guidelines. Its accuracy in terms of maximum bias and linearity errors is inside the performance limits for ISO 80601-2-12-2020 requirements.
ATENA can achieve satisfactory performances for all volume-controlled ventilation and pressure-controlled ventilation test conditions with a plateau pressure of 15 cmH2O. Limited performance was observed in only three tests for plateau pressure of 30 cmH2O, which explains the outlier values presented in Figure 5:
#1 and 2–In the test with a plateau pressure of 30 cmH2O, compliance of 50 ml/cmH2O, resistance of 5 cmH2O/L/s, and respiratory rate of 20 cpm, both plateau pressure and PEEP were almost achieved. A high instantaneous flow (SLPM) would be required for the PEEP and plateau pressure setpoints to be reached. In fact, during these tests, tidal volumes as high as 1,000 ml were recorded. For a desired FiO2 of 95%, the required instantaneous flow would exceed the maximum capabilities of ATENA's hardware. Due to the high FiO2 setpoint, a single piezo-electric valve, the oxygen one, would limit the maximum flow to its capacity, ~60 SLPM. It is important to notice that ATENA contains two piezo-electric valves, one for air and one for oxygen, each rated to a maximum of 60 SLPM. Again, the tidal volume observed in these tests is not adequate for the protective ventilation that is needed for COVID-19 patients.
#3–For a plateau pressure of 30 cmH2O, compliance of 50 ml/cmH2O, and FiO2 set to 95%, this last parameter could not be reached. This limitation is once again explained by ATENA's hardware capabilities that, when mostly using a single piezo-electric valve, are capable of reaching instantaneous flows of 60 SLPM.
The majority of ISO requirements were met by ATENA. However, for the accuracy tests of volume-controlled ventilation (Supplementary Table 2, tests 1 and 2), in a simulated healthy patient (C = 50 ml/cmH2O and linear R of up to 20 cmH2O/L/s, tidal volume 500 ml), the PEEP parameter was underperforming. These results were considered to be sufficient for the adequate validation of ATENA since it is not expected that patients who developed bilateral pneumonia due to COVID-19 with the need for invasive ventilation (the scope of use for the ATENA ventilator) present a lung compliance of 50 ml/cmH2O (25). Furthermore, ATENA displays the accurately measured PEEP, so even if underperforming, it will provide the clinician the required data to make an informed decision.
Simultaneously, the analysis of the small PEEP coefficients of variation in tests performed in volume- and pressure-controlled ventilation allows us to affirm the stability and safety of this parameter in situations that mimic clinical practice.
Taking into account all of the above, it is possible to conclude that the ATENA adequately performed for all test conditions set by the MHRA's RMVS and ISO guidelines, except for a single set of conditions not commonly found in day-to-day clinical practice and may therefore be ruled as less relevant for a pandemic COVID-19 ventilator.
Results from the additional tests that extended the setting parameters of tidal volume, PEEP, FiO2, and plateau pressure to their extreme limits prove that ATENA can perform adequately in an enlarged range of values.
We studied ATENA's performance on pressure support (PS) ventilation mode with the settings commonly used for weaning a patient from the ventilator (pressure support of 10 cmH2O and PEEP of 5 cmH2O). The analysis of the results in these settings revealed that ATENA was able to provide PS ventilation in the range of flow trigger values under study and its performance was not impaired when we assessed different levels of inspiratory muscle strength, in both healthy and ARDS lung models.
Noteworthy, even though two HME/HEPA filters were included on the 1.5-m breathing tubes, during the pressure support tests, the muscle pressure required to successfully trigger the ventilator was adequate. This indicates that the trigger sensitivity is robust and is adapted to real working conditions.
During in vivo animal model studies, ATENA behaved within expectations for the required standards across the full range of configurations, in both volume- and pressure-controlled modes, maintaining excellent oxygenation and ventilation performance. In both groups, changes in partial gas pressure and arterial pH secondary to changes in ventilatory parameters were biologically expected. In no trial was oxygenation compromised, and an improvement in the partial pressure of oxygen in arterial blood in response to the increase in ventilation/min was always observed. At the same time, the hyperventilation that conditioned the presence of respiratory alkalosis in numerous blood gases assertively demonstrates the effectiveness of ATENA in ventilation.
Conclusions
The novel ATENA Medical Ventilator, here described, was extensively tested, fulfilling MHRA and ISO requirements and specifications. Additional tests for extended parameter limits, pressure support, and pre-clinical studies demonstrated that it can perform as required. The ventilator is capable of controlled modes VCV, PCV, and PRVC, and mode PSV, and is therefore entirely appropriate for clinical use in adult COVID-19 patients. Further clinical trials must be performed to ultimately and unequivocally validate ATENA in clinical practice.
Data Availability Statement
The raw data supporting the conclusions of this article will be made available by the authors, without undue reservation.
Ethics Statement
The animal study was reviewed and approved by EU Directive 2010/63/EU, under project authorization attributed by local ethics committee (Subcomissão de Ética para as Ciências da Vida e da Saúde - SECVS 004/2016, Chairperson Prof. Cecília Leão) and by the National Authority for animal protection (Direção Geral de Alimentação e Veterinária - et al. DGAV 015296, Chairperson Dr. Fernando Bernardo).
Author Contributions
TR, FB, HO, JM, JA, JL, JS, MO, PF, RaM, and RuM: CEiiA's engineering team and were responsible for conceiving, developing, prototyping, and testing the ventilator throughout its development and validation. EN, EA, HM, JP, NS, PB, and SG: medical core team and Defined the specifications, tested, and validated the ATENA ventilator throughout its development and validation phases. TR, JA, SG, and JP: study conception and design of the paper. TR, JA, FB, PF, JP, RaM, and SG: acquisition of data, analysis and interpretation of data, and drafting the article. TR, JA, SG, RaM, and JP: in-depth revising the manuscript critically for important intellectual content. All authors contributed to the study and final approval of the version to be published and all agree to be accountable for all aspects of the work, thereby ensuring that questions related to the accuracy or integrity of any part of the work are appropriately investigated and resolved.
Funding
Financial support and sponsorship by CEiiA, INOV4COVID program, donations from scientific patronage, and commercial sales.
Conflict of Interest
The authors declare that the research was conducted in the absence of any commercial or financial relationships that could be construed as a potential conflict of interest.
Publisher's Note
All claims expressed in this article are solely those of the authors and do not necessarily represent those of their affiliated organizations, or those of the publisher, the editors and the reviewers. Any product that may be evaluated in this article, or claim that may be made by its manufacturer, is not guaranteed or endorsed by the publisher.
Acknowledgments
The authors of this paper would like to acknowledge the support of all patrons, public and private institutions, medical and healthcare staff, universities, research institutions, enterprises, and ultimately all individuals who kindly gave their time, effort, and resources to the development of the ATENA Rapidly Manufactured Medical Ventilator. The development of this life-saving equipment would not have been possible without their support. To all the members of the CEiiA team that supported the development of the device: Abílio Chaves Teles, Alberto Couto, Alexander Costa, Álvaro Santos, Ana Azevedo, André Gonçalves, André Guerra, André João, André Moura, André Oliveira, António Cunha, António Marques, António Romão, Armindo Torres, Artur Costa, Beatriz Cruz, Bruno Oliveira, Bruno Rodrigues, Caio Lomba, Carlos Cruz, Carlos Fernandes, Carlos Marques, Carlos Moura, Carlos Rijo, Carlos Sousa, Cristiana Pereira, Dania Ferreira, Daniel Martins, Daniel Pereira, Daniel Vaz, Diana Ferraz, Duarte Brandão, Dulce Fernandes, Fábio Cunha, Fernando Santos, Filipe Bastos, Francisco Carrola, Francisco Sousa, Francisco Vasconcelos, Gustavo Burmester, Hélder Cunha, Hélder Pereira, Helena Silva, Henrique Pimentel, Hugo Lamarão, Ilídio Magalhães, Inês Furtado, Joana Canossa, João Aguiar, João Ferreira, João Figueiredo, João Pereira, João Teixeira, Joaquim Rocha, Joaquim Teles, Jorge Carneiro, José Espogeira, José Ginjo, José Rui Felizardo, Luís Carneiro, Luís Colaço, Luís Leitão, Luís Pinheiro, Luís Reis, Lurdes Ferreira, Marco Carmo, Marcos Moreira, Miguel Braga, Miguel Fernandes, Miguel Ferreira, Miguel Machado, Miguel Ribeiro, Mónica Moitas, Nélson Maravalhas, Nuno Carneiro, Nuno Lourenço, Oleh Tkachuk, Paulo Encarnação, Paulo Ferreira, Paulo Maia, Pedro Fontinha, Pedro Lopes, Pedro Marques, Pedro Pinheiro, Pedro Queiroz, Pedro Reguenga, Pedro Silva, Renato Machado, Ricardo Campos, Ricardo Guimarães, Ricardo Meireles, Roque Ferreira, Rúben Marques, Rui Machado, Sandra Fortuna, Sara Sá, Teresa Nascimento, Tiago Bartolomeu, Tiago Macedo, Tiago Marcelino, Tiago Pires, and Vladyslav Reznikov. To all the members of the medical community that helped understand, design, and test the device: Ana Cristina Pereira, Ângela Cunha, Carolina Santos, Filipa Cunha, Gonçalo Fernandes, Irene Aragão, Joana C. Azevedo, João Colaço, João Gonçalves, João Gouveia, Jorge Marvão, Luis Lencastre, Milagros Garcia, Patrícia Santos, Paula Simão, Paulo Gomes, Rosário Fortuna, Rita M. Oliveira, and Sérgio Vide. Special thanks to Alice Miranda VM, Ph.D., for all the support during the pre-clinical tests.
Supplementary Material
The Supplementary Material for this article can be found online at: https://www.frontiersin.org/articles/10.3389/fmed.2021.614580/full#supplementary-material
Supplementary Table 1. Description of each ATENA's component and the corresponding manufacturer.
Supplementary Table 2. ATENA accuracy of volume and pressure-controlled ventilation (ISO 80601-2-12, clause 201.12.1.102).
Supplementary Table 3. ATENA accuracy of expired volume indication (ISO 80601-2-12, clause 201.12.4.103.1).
Supplementary Table 4. Results from ASL5000, on pressure support ventilation in healthy and ARDS lung model.
Supplementary Table 5. Relevant ventilatory settings and measures of ventilation (average ± standard deviation) in pre-clinical testing for volume-controlled ventilation. TV—tidal volume; RR—respiratory rate; PEEP—positive end-expiratory pressure; PO2–oxygen arterial partial pressure; PCO2–CO2 arterial partial pressure; –bicarbonate arterial concentration.
Supplementary Table 6. Relevant ventilatory settings and measures of ventilation (average ± standard deviation) in pre-clinical testing for pressure-controlled ventilation. TV—tidal volume; RR—respiratory rate; PEEP—positive end-expiratory pressure; PO2–oxygen arterial partial pressure; PCO2–CO2 arterial partial pressure; –bicarbonate arterial concentration.
References
1. Zhu N, Zhang D, Wang W, Li X, Yang B, Song J. A novel coronavirus from patients with pneumonia in China. N Engl J Med. (2019) 382:727–33. doi: 10.1056/NEJMoa2001017
2. Gavriatopoulou M, Ntanasis-Stathopoulos I, Korompoki E, Fotiou D, Migkou M, Tzanninis IG, et al. Emerging treatment strategies for COVID-19 infection. Clin Exp Med. (2021) 21:167–79. doi: 10.1007/s10238-020-00671-y
3. Ferguson NM, Laydon D, Nedjati-Gilani G, Imai N, Ainslie K, Baguelin M, et al. Impact of Non-Pharmaceutical Interventions (NPIs) to Reduce COVID-19 Mortality and Healthcare Demand. Imperial College London (2020).
4. Yang X, Yu Y, Xu J, Shu H, Xia J, Liu H. Clinical course and outcomes of critically ill patients with sARS-CoV-2 pneumonia in Wuhan, China: a single-centered, retrospective, observational study. Lancet Respir Med. (2020) 8:475–81. doi: 10.1016/S2213-2600(20)30079-5
5. Guérin C, Lévy P. Easier access to mechanical ventilation worldwide: an urgent need for low income countries, especially in face of the growing COVID-19 crisis. Eur Respir J. (2020) 55:2001271. doi: 10.1183/13993003.01271-2020
6. Ranney ML, Griffeth V, Jha AK. Critical supply shortages—the need for ventilators and personal protective equipment during the covid-19 pandemic. N Engl J Med. (2020) 382:e41. doi: 10.1056/NEJMp2006141
7. Truog RD, Mitchell C, Daley GQ. The toughest triage—allocating ventilators in a pandemic. N Engl J Med. (2020) 382:1973–5. doi: 10.1056/NEJMp2005689
8. Fang Z, Li AI, Wang H, Zhang R, Mai X, Pan T. AmbuBox: a fast-deployable low-cost ventilator for COVID-19 emergent care. SLAS Technol. (2020) 25:573–84. doi: 10.1177/2472630320953801
9. Dhanani J, Pang G, Pincus J, Ahern B, Goodwin W, Cowling N, et al. Increasing ventilator surge capacity in cOVID 19 pandemic: design, manufacture and in vitro-in vivo testing in anaesthetized healthy pigs of a rapid prototyped mechanical ventilator. BMC Res Notes. (2020) 13:421. doi: 10.1186/s13104-020-05259-z
10. Kwon AH, Slocum AH Jr, Varelmann D, Nabzdyk CGS, MIT E-Vent Team. Rapidly scalable mechanical ventilator for the COVID-19 pandemic. Intens Care Med. (2020) 46:1642–4. doi: 10.1007/s00134-020-06113-3
11. Cole JH, Hughey SB, Rector CH, Booth GJ. A novel low-cost ventilator for use in a worldwide pandemic: the portsmouth ventilator. Crit Care Explor. (2020) 2:e0292. doi: 10.1097/CCE.0000000000000292
12. Knorr JM, Sheehan MM, Santana DC, Samorezov S, Sammour I, Deblock M, et al. Design and performance testing of a novel emergency ventilator for in-hospital use. Can J Respir Ther. (2020) 56:42–51. doi: 10.29390/cjrt-2020-023
13. Herrmann J, Fonseca da Cruz A, Hawley ML, Branson RD, Kaczka DW. Shared ventilation in the era of COVID-19: a theoretical consideration of the dangers and potential solutions. Respir Care. (2020) 65:932–45. doi: 10.4187/respcare.07919
14. Branson RD, Rodriquez D Jr. 2020 year in review: shared ventilation for COVID-19. Respir Care. (2021) 66:1173–83. doi: 10.4187/respcare.09198
15. Garmendia O, Rodríguez-Lazaro MA, Otero J, Phan P, Stoyanova A, Dinh-Xuan AT, et al. Low-cost, easy-to-build noninvasive pressure support ventilator for under-resourced regions: open source hardware description, performance and feasibility testing. Eur Respir J. (2020) 55:2000846. doi: 10.1183/13993003.00846-2020
16. Medicines & Healthcare products Regulatory Agency. Rapidly Manufactured Ventilator System (RMVS). (2020). Available online at: https://assets.publishing.service.gov.uk/government (accessed January 7, 2021).
17. Infarmed. Procedimento Especial de Avaliação de Dispositivos Médicos no Âmbito COVID-19: Ventiladores. Available online at: https://www.infarmed.pt/ (accessed January 7, 2021).
18. International Organization for Standardization. Medical Electrical Equipment- Part 2-12: Particular Requirements for Basic Safety and Essential Performance of Critical Care Ventilators. Available online at: https://www.iso.org/standard/72069.html (accessed October 10, 2020).
19. Chen Y, Yuan Y, Cai C, Li F, Zhou X. Effects of assist parameter on the performance of proportional assist ventilation in a lung model of chronic obstructive pulmonary disease. Respir Med Res. (2020) 78:100766. doi: 10.1016/j.resmer.2020.100766
20. Terado M, Ichiba S, Nagano O, Ujike Y. Evaluation of pressure support ventilation with seven different ventilators using active servo lung 5000. Acta Med Okayama. (2008) 62:127–33. doi: 10.18926/AMO/30963
21. Daoud EG, Katigbak R, Ottochian M. Accuracy of the ventilator automated displayed respiratory mechanics in passive and active breathing conditions: a Bench study. Respir Care. (2019) 64:1555–60. doi: 10.4187/respcare.06422
22. Abramovitz A, Sung S. Pressure support ventilation. In: StatPearls. Treasure Island, FL: StatPearls Publishing (2021).
23. Ou-Yang LJ, Chen PH, Jhou HJ, Su VY, Lee CH. Proportional assist ventilation versus pressure support ventilation for weaning from mechanical ventilation in adults: a meta-analysis and trial sequential analysis. Crit Care. (2020) 24:556. doi: 10.1186/s13054-020-03251-4
24. Gattinoni L, Chiumello D, Caironi P, Busana M, Romitti F, Brazzi L, et al. COVID-19 pneumonia: different respiratory treatments for different phenotypes? Intensive Care Med. (2020) 46:1099–102. doi: 10.1007/s00134-020-06033-2
25. Medicines & Healthcare products Regulatory Agency. Rapidly Manufactured Ventilator System (RMVS) (2020). Available online at: https://assets.publishing.service.gov.uk/government/uploads/system/uploads/attachment_data/file/879382/RMVS001_v4.pdf
Keywords: COVID-19, ATENA, pandemic, ventilator, safety, performance
Citation: Rebelo T, Neutel E, Alves EC, Barros F, Oliveira H, Machado H, Mendonça J, Araújo JF, Luís J, Pêgo JM, Silva J, Oliveira M, Sousa N, Figueiredo P, Barata P, Magalhães RS, Magalhães RM and Gomes SH (2021) ATENA–A Novel Rapidly Manufactured Medical Invasive Ventilator Designed as a Response to the COVID-19 Pandemic: Testing Protocol, Safety, and Performance Validation. Front. Med. 8:614580. doi: 10.3389/fmed.2021.614580
Received: 06 October 2020; Accepted: 19 July 2021;
Published: 19 August 2021.
Edited by:
Eric Azabou, Assistance Publique Hopitaux De Paris, FranceReviewed by:
Marina Soro, Hospital Clínico Universitario de Valencia, SpainGusztav Belteki, Cambridge University Hospitals NHS Foundation Trust, United Kingdom
Maolin Cai, Beihang University, China
Copyright © 2021 Rebelo, Neutel, Alves, Barros, Oliveira, Machado, Mendonça, Araújo, Luís, Pêgo, Silva, Oliveira, Sousa, Figueiredo, Barata, Magalhães, Magalhães and Gomes. This is an open-access article distributed under the terms of the Creative Commons Attribution License (CC BY). The use, distribution or reproduction in other forums is permitted, provided the original author(s) and the copyright owner(s) are credited and that the original publication in this journal is cited, in accordance with accepted academic practice. No use, distribution or reproduction is permitted which does not comply with these terms.
*Correspondence: Tiago Rebelo, tiago.rebelo@ceiia.com