- 1Team “Resist” UMR1184 “Immunology of Viral, Auto-Immune, Hematological and Bacterial diseases (IMVA-HB),” INSERM, Université Paris-Saclay, CEA, LabEx LERMIT, Faculty of Medicine, Le Kremlin-Bicêtre, France
- 2Associated French National Reference Center for Antibiotic Resistance: Carbapenemase-Producing Enterobacteriaceae, Le Kremlin-Bicêtre, France
- 3Evolution and Ecology of Resistance to Antibiotics Unit, Institut Pasteur-APHP-Université Paris-Sud, Paris, France
- 4Bacteriology-Hygiene Unit, Assistance Publique-Hôpitaux de Paris, AP-HP Paris Saclay, Bicêtre Hospital, Le Kremlin-Bicêtre, France
Gram-negative bacteria, especially Enterobacterales, have emerged as major players in antimicrobial resistance worldwide. Resistance may affect all major classes of anti-gram-negative agents, becoming multidrug resistant or even pan-drug resistant. Currently, β-lactamase-mediated resistance does not spare even the most powerful β-lactams (carbapenems), whose activity is challenged by carbapenemases. The dissemination of carbapenemases-encoding genes among Enterobacterales is a matter of concern, given the importance of carbapenems to treat nosocomial infections. Based on their amino acid sequences, carbapenemases are grouped into three major classes. Classes A and D use an active-site serine to catalyze hydrolysis, while class B (MBLs) require one or two zinc ions for their activity. The most important and clinically relevant carbapenemases are KPC, IMP/VIM/NDM, and OXA-48. However, several carbapenemases belonging to the different classes are less frequently detected. They correspond to class A (SME-, Nmc-A/IMI-, SFC-, GES-, BIC-like…), to class B (GIM, TMB, LMB…), class C (CMY-10 and ACT-28), and to class D (OXA-372). This review will address the genetic diversity, biochemical properties, and detection methods of minor acquired carbapenemases in Enterobacterales.
Nowadays antimicrobial resistance is of critical concern. Since the first identification of an enzyme able to destroy the penicillin by Abraham and Chain in the 1940s (1), more than 4,900 β-lactamases have been reported (http://www.bldb.eu/). Two main classifications are used: (i) the structural classification of Ambler and (ii) the functional classification of Bush and Jacoby (2, 3). Since the functional classification is more complicated, Ambler's classification will be used in this review. According to Ambler's structural classification, four classes (A to D) of β-lactamase are described (4). Briefly, the class A groups penicillinases and their extended-spectrum variants that are inhibited by clavulanate, sulbactam, and tazobactam. The class B corresponds to metallo-β-lactamases. All of the metallo-β-lactamases possess a carbapenemase activity. They are inhibited by ion chelator such as EDTA but not by class A inhibitor (clavulanate, tazobactam, sulbactam). Class C enzymes correspond to cephalosporinase and initially demonstrated better activity toward first generations cephalosporins compared to penicillins. They are inhibited by cloxacillin. Finally, class D enzymes, also named oxacillinases, group very diverse β-lactamase sub-families. They were initially reported to be inhibited in vitro by NaCl (5). Novel inhibitors such as avibactam, relebactam, or vaborbactam that possess inhibitory activity toward class A, C, and ± D will be described further in this review.
Thirty years of carbapenemase epidemiology demonstrated that these broad-spectrum enzymes might be split in two groups, the “Big Five” carbapenemases and the “rare” carbapenemases. The “Big Five” carbapenemases corresponds to the five main carbapenemases identified worldwide being class A KPC enzymes, metallo-β-lactamases of IMP, VIM & NDM groups, and class D OXA-48-like enzymes (4–7). The rare carbapenemases constitute a diverse group of enzymes belonging to the four classes of β-lactamases. The observed lower prevalence might be due to genetic features leading to a lower spread, or to the underdetection due to the lack of specific diagnostic tests targeting these enzymes.
Before starting this journey in carbapenemases-producing bacteria, it is important to overview the important changes in bacterial nomenclature. Indeed, with the massive use of whole genome sequencing, bacterial nomenclature has evolved rapidly during the last decade. Accordingly, this nomenclature evolution will lead to some changes in the old descriptions. Enterobacteria constitute a large and diverse group of facultative aerobic, gram negative rods. They are highly diverse regarding their biochemical properties, their pathogenicity, as well as for their ecological niches. The former order of Enterobacteriales has been reorganized recently based on the phylogenetic analysis of 1,500 protein sequences and overall genome similarity (8). The order of Enterobacterales is now composed of 7 families being Enterobacteriaceae, Erwiniaceae, Pectobacteriaceae, Yersiniaceae, Hafniaceae, Morganellaceae, and Budvicaceae. The most clinically relevant bacterial genera are part of the Enterobacteriaceae family, including Escherichia/Shigella, Salmonella, Klebsiella, Citrobacter, and Enterobacter. Yersiniaceae family contains Yersinia spp. and Serratia spp. and Morganellaceae contains Morganella spp., Proteus spp., and Providencia spp. To dive deeper in Enterobacterales classification and evolution, the case of Enterobacter genus is a key example. Described in the 1960s, this genus comprised more than 20 species (https://lpsn.dsmz.de/genus/enterobacter) but its classification is continuingly evolving. For instance, Enterobacter aerogenes (also renamed Klebsiella mobilis in the 1970s) has been officially reclassified as Klebsiella aerogenes (9). Another example of this constant evolution is the reclassification of Enterobacter sakazakii as Cronobacter sakazakii (10). Moreover, it is highly difficult, even almost impossible, to decipher the E. cloacae complex (ECC) using classical microbiological methods even using MALDI-TOF for that purpose (11). For all these reasons, ancient description of genus and species in this manuscript should be analyzed with the prism that they were not identified with phylogenetic analysis methods and thus can be misidentified.
Minor Class a Carbapenemases
The main class A carbapenemases in Enterobacterales correspond to KPC-type enzymes (4, 5). Beyond this major carbapenemase family, a wide diversity of unrelated minor class A carbapenemases have emerged including IMI-, FRI-, or GES-type enzymes. To complicate the situation, some of these carbapenemases possess a peculiar phenotype that can be missed on the antibiogram. This chapter will focus on the genetic diversity and phenotypes of these rarely described class A carbapenemases.
IMI / NMC-A
The IMI/NMC-A (Imipenemase/Non-metallo-carbapenemase A) carbapenemases form a group of carbapenemases identified in Enterobacter genus. They are among the oldest carbapenemases described (12, 13). IMI-1 confers resistance to penicillins alone and in combination with clavulanate, early generation cephalosporins, and carbapenems but spares broad-spectrum cephalosporins such as ceftazidime (Figure 1) (12). Despite rarely described, in comparison to “Big Five” carbapenemases, IMI-like carbapenemases have been described in different continents. IMI-1 was initially reported in the USA in an E. cloacae isolate from 1984, thus a year prior the US approval of imipenem. Since then, IMI-1 has been identified in Enterobacter genus in Singapore, China, French Polynesia, Vietnam, and Japan (14–17). IMI-2 was firstly identified in E. asburiae recovered from environmental samples in US rivers from 1999 to 2001 (18). It has been identified in Klebsiella variicola in UK, in E. asburiae in Czech Republic, in E. cloacae from Spanish rivers, France, and Canada, Enterobacter mori in Austria, and E. coli in Spain and China (19–24). IMI-3 was firstly detected in China and France (21, 25, 26). IMI-5, IMI-6 were identified in Canada (27). IMI-9 was identified in Enterobacter cloacae in Canada and Norway (27, 28). In addition, IMI-13 and IMI-17 were detected in France by the French National Center (unpublished data). To date, 19 variants of IMI plus NMC-A have been identified. Genetic analysis revealed interesting features related to the acquisition of those genes. Whereas, blaIMI−1 is often carried on the chromosome, blaIMI−2 is mainly carried on plasmids. The genetic element at the origin of the acquisition of blaIMI−1 is a genomic island involving XerC/XerD recombinases. These elements are named EcloIMEX-like elements (27). At least 8 EcloIMEX have been described in the literature. They differ by the diversity at the 3′ extremity of the structures. However, the 5′ extremity carrying the blaIMI-like gene is highly conserved and contained different hypothetical proteins, a putative protease, and ABC_ATPase and a glycosyltransferase (27). Some EcloIMEX elements (for XER-dependent integrative mobile elements) seem to have recombined and have lost part of their IMEX as observed for EcloIMEX-8 (17). All of these elements are inserted in a dif site between setB and yeiP genes. These elements are also responsible for the acquisition of blaIMI−9 carried by EcloIMEX-5 and EcloIMEX-6. The blaIMI−2 was carried on a self-transferable plasmid of ca. 66 kb in E. asburiae from US rivers (18). After initial identification, a blaIMI−2-carrying plasmid was sequenced from K. variicola (19). This plasmid belonged to IncFII-family plasmid and was of 77 kb in size. The blaIMI−2 gene has also been identified in IncFI-like plasmid in E. coli in Spain (20). The mechanism of acquisition of blaIMI−2 remains unclear. As for all blaIMI-like gene, a LysR family transcriptional regulator, blaIMI−R, is present upstream of blaIMI−2 (18). Different ISs or IS remnants have been identified bracketing blaIMI−2-blaIMI−R locus including ISEcl3, ISEcl1, ISEc36 (21). The presence of this gene on IncF-type plasmid families is very likely the reason of its occurrence out of Enterobacter genus. The blaIMI−3 gene was identified on an IncFIIY plasmid within a new composite transposon, Tn6306. This transposon is composed of 2 copies of ISEcl1-like bracketing the resistance gene (21). The blaIMI−5 and blaIMI−6 genes are also carried by different IncFII-type plasmids of c.a 90 and c.a 165 kb (27). Of note, the blaIMI−6 and blaIMI−3-carrying plasmids also carried a type VI secretion system that might give an advantage under certain ecological niches.
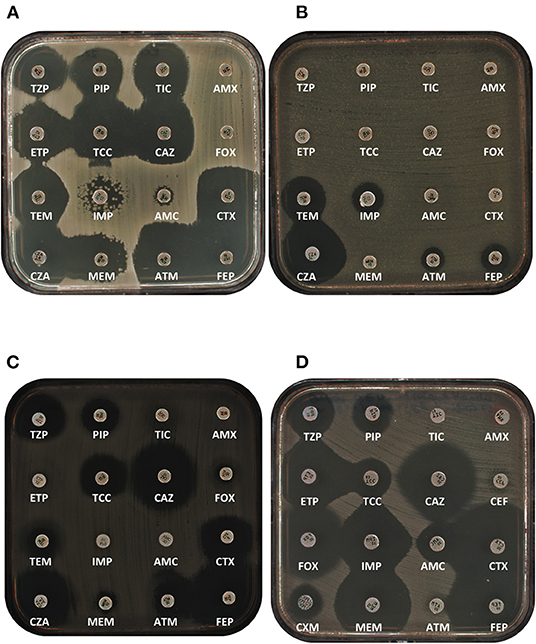
Figure 1. Antibiograms of representative class A carbapenemases. (A) IMI-1-producing E. cloacae complex clinical isolate. (B) GES-5 producing K. pneumoniae clinical isolate. (C) SME-3-producing S. marcescens. (D) FRI-1-producing E. coli transformant. AMX, Amoxicillin; AMC, Amoxicillin/clavulanate; ATM, aztreonam; CAZ, ceftazidime; CTX, Cefotaxime; CZA, Ceftazidime/avibactam; ETP, Ertapenem; FEP, Cefepime; FOX, Cefoxitine; IPM, Imipenem; MEM, Meropenem; PIP, Piperacillin; TCC, Ticarcillin/Clavulanate; TEM, Temocillin; TIC, Ticarcillin; TZP, Piperacillin/Tazobactam.
BKC-1
BKC-1, for Brazilian Klebsiella carbapenemase, is one of the latest carbapenemases described. The first occurrence of this carbapenemase was reported in three K. pneumoniae strains isolated in Sáo Paulo, Brazil (29). These three isolates were recovered from two different hospitals but showed the same pulse field gel electrophoresis (PFGE) pattern and belonged to same sequence type (ST), ST1781. Cloned in E. coli, the production of BKC-1 confers resistance to penicillins, broad-spectrum cephalosporins, aztreonam, and decreased susceptibility to carbapenems (Table 1). However, as observed for ESBLs, efficacy of cefoxitin is not altered by BKC-1. Purification of this enzyme confirmed the observed phenotype with hydrolysis of penicillins, cephalosporins, and carbapenems but not cefoxitin. Phylogenetic analysis revealed few similarities with other class A carbapenemases, e.g., 39% of amino-acid identity with KPC-2. The closest β-lactamase corresponds to an uncharacterized β-lactamase identified in Sinorhizobium meliloti with 63% amino acid identity (29). The blaBKC−1 is carried by a small non-conjugative IncQ-type plasmid of 9.7 kb in size. Upstream of blaBKC−1, a copy of ISKpn23 is inserted, likely leading to its expression and its probable mobilization (30). Indeed, ISKpn23 belongs to IS1380 family. The most famous member of this family is ISEcp1, known to mobilize adjacent genes by one-ended transposition (31). This gene has also been identified in C. freundii harboring the same plasmid (32). Until now, this enzyme has never been described out of Brazil and presented an overall low prevalence in this country with 0.3% (2/635) of Klebsiella spp. isolates randomly selected among strains collected from previous surveillance studies (33). In this study, the two isolates of K. pneumoniae were clonally related belonging to ST442 and possessed the same plasmid.
SHV-38
SHV is the natural class A β-lactamase of K. pneumoniae. Variants of SHV with changes in their hydrolytic properties were the main resistance mechanism to broad-spectrum cephalosporins in the 1980s (with TEM-like enzyme) before the emergence and the spread of CTX-M enzymes (34). These enzymes gave the name of extended-spectrum β-lactamase compared to narrow-spectrum SHV-1. Among more than 200 variants of SHV enzymes, SHV-38, possessing A146V substitution, has been described to be the only SHV variant with carbapenemase activity (35). Once cloned in E. coli, this enzyme conferred increased MICs to imipenem as compared with SHV-1 (Table 1). Enzymatic assays confirmed ability of SHV-38 to hydrolyze imipenem (Table 2). However, no characterization of the impact of the amino acid substitution was performed to date (e.g., replacement of the valine by another amino acid or the crystallization of this enzyme bound to imipenem). This enzyme has been detected in Brazil, in environmental samples in India and in Tunisia, but its presence is very likely underestimated (36–38).
CTX-M-33
CTX-M enzymes are the main ESBLs described worldwide and have replaced the “old” ESBLs, TEM-, and SHV-like enzymes (39). Despite the fact that the production of CTX-M-15 associated to porin deficiency can increase the MIC of ertapenem, this enzyme does not hydrolyze carbapenems at a significant level (40, 41). Recently, a variant of CTX-M-15, CTX-M-33, was reported to hydrolyze significantly carbapenems despite a very low kcat for imipenem and ertapenem (42). This enzyme has been firstly identified in Greece in 2007 and more recently in Portugal (43, 44). As mentioned above for SHV-38, the prevalence of this enzyme is likely underestimated since sequencing of the gene is mandatory to detect this peculiar variant.
GES
Among the wide diversity of ESBLs, some are major such as the pandemic CTX-M-family enzymes, and some are minor considering their rare identification or their restriction to certain areas. Among these ESBLs, a family is of particular interest: GES-type enzymes. Firstly identified in 1998 in France in a K. pneumoniae isolate (45), GES-1 production conferred resistance to penicillins, broad-spectrum cephalosporins, but not to cephamycins and carbapenems (45). After this first identification, a variant, GES-2, possessing a G170N substitution, was characterized. This variant was the first ESBL variant with a significant carbapenemase activity (46). Since then, more than 40 variants of GES-1 have been described. Among them, all variants with a substitution of the glycine 170 exhibited significant carbapenemase activity with the higher catalytic properties for G170S substitution. In addition to carbapenem hydrolysis, G170S substitution increased hydrolysis spectrum toward cephamycins (47). Noticeably, two other amino acid positions are involved in hydrolysis spectrum changes. Indeed, positions 104 and 243 are involved in increased hydrolysis toward oxyimino-cephalospoins and aztreonam, respectively (47, 48). Several GES-type carbapenemases have been identified in Enterobacterales being GES-3 in Greece, Japan, and Korea (49–51), GES-4 in Greece and Japan (49, 52), GES-5 in Korea, Brazil (including remote community in Amazonia), France (Figure 1), Czech Republic, South Africa, and Portugal (53–58), GES-6 in Belgium, Macedonia, and Israel (59, 60), and GES-16 in Brazil (61). The blaGES-type genes are usually carried by class 1 integron, but also more rarely by class 3 integron responsible for their expression. These genes have been described on a variety of plasmid families. Noticeably, this gene family is increasingly reported with, for instance, two recent reports of GES-5-producing K. pneumoniae in Poland and GES-5-producing K. oxytoca, E. coli, and E. cloacae in UK (62, 63).
SFC-1
Among rare class A carbapenemases, SFC-1 (Serratia fonticola resistant to carbapenems) and SME-like (Serratia marcescens enzyme) enzymes have been identified in Serratia fonticola and Serratia marcescens, respectively (64–66). Despite its identification on the chromosome of S. fonticola, blaSFC−1 is not shared by all S. fonticola but only in one isolate from Portugal. No information regarding its acquisition is available in the literature. SFC-1 hydrolyzes all β-lactams including broad-spectrum cephalosporins, cephamycins, and carbapenems.
SME
SME-1 was initially detected in two isolates recovered in England in early 1980s (67). Kinetics parameters of SME-1 and SME-2 demonstrated hydrolysis of penicillins, early-generation cephalosporins, and carbapenems but not cephamycins and broad-spectrum cephalosporins (68) (Figure 1). To date, five point-derivative variants of SME-1 were reported. SME-1 has been detected in UK and across the USA (68–71), SME-2 in Argentina, Switzerland, Canada, USA (68, 72, 73), SME-3 in USA (74), SME-4 in Brazil, Argentina, and USA (75–77), and SME-5 in Canada (Genbank accession number KJ188748). Analysis of the genetic context revealed that expression of this carbapenemase was under the control of a lysR-family transcriptional regulator, SmeR (78). SmeR acts as an inducer of carbapenemase expression in presence of cefoxitin or imipenem. Little information is available regarding the genetic environment of blaSME−like gene. The blaSME−1/−2 genes were embedded within a 28 kb genomic island named SmarGI1-1. This genomic island was inserted within the chromosome of S. marcescens at the locus ssrA coding for the tmRNA representing the att site (79). A similar structure is responsible for the acquisition of blaSME−4 in S. marcescens isolate in Argentina (76).
FRI
One of the last described class A carbapenemase family in Enterobacterales corresponds to FRI-1, for French resistance to imipenem (80). The blaFRI−1 gene was detected in a E. cloacae isolated in a patient hospitalized in Paris area, with a previous history of travel in Switzerland. Production of FRI-1 conferred resistance or reduced susceptibility to penicillins, cephalosporins, aztreonam, and carbapenems (Figure 1; Table 1). Purified FRI-1 enzyme exhibited hydrolysis of all tested β-lactams except ceftazidime. It can be noticed that Km were relatively high compared to other class A carbapenemases indicating a weak affinity (Table 2). Nine variants have been described to date. These variants did not correspond to point derivatives but exhibited 81–94% amino acid identities for FRI-8 and FRI-6, respectively. FRI-1 has been reported in France (80), FRI-2 in UK (81), FRI-3 in Germany (82), FRI-4 in Japan (83), FRI-5 in Japan (MH208723), FRI-6 in Canada (84), FRI-7/-8/-9 in Japan (AP019534, AP019635, AP019633). The blaFRI−1 gene was associated to a lysR-family transcriptional regulator as observed for blaSME−1 responsible for inducible expression of the gene (80). The blaFRI−1 gene has been identified on a 110 kb non conjugative and untypeable plasmid. The IncFII/IncR plasmid of 98 kb in size carrying the blaFRI−4 gene has been entirely sequenced. Interestingly, the blaFRI−4 gene and its surrounding region were duplicated on this plasmid (83). The last class A carbapenemase identified is FLC-1, for FRI-like carbapenemase, from Indian frozen seafood in Netherland in 2017 (24). This carbapenemase is 99.66 and 82.3% amino acids identical to FRI-8 and FRI-1, respectively. Therefore, this enzyme may be reclassified as FRI-like variant. The phenotype observed in FLC-1-producing is similar to FRI-1 (Table 1). This was confirmed in biochemical analysis being hydrolysis of penicillins and carbapenems but not ceftazidime or cefepime (Table 2). The blaFLC−1 was carried by a plasmid belonging to IncFII family. Surrounding the blaFLC−1 gene, remnants of IS belonging to IS3 family have been identified (24).
Minor Class b Carbapenemases
Metallo-β-lactamases belong to the molecular class B of Ambler's classification and group 3 of Bush & Jacoby's classification (2, 3). These enzymes are very diverse in term of structure and hydrolytic profile. They can be classified in different subgroups (B1, B2, & B3) based on their structures. The main metallo-β-lactamases that have been identified worldwide in Enterobacterales are NDM-, VIM-, and IMP-like enzymes (4). In addition, several rare class B carbapenemases have been described in Enterobacterales being class B1 GIM-1, TMB-1, and KHM-1, class B2 SFH-1, and class B3 LMB-1 and AIM-1.
GIM-1
GIM-1, for Germany Imipenemase, is a class B1 metallo-β-lactamase described in 2002 from five imipenem-resistant P. aeruginosa in Germany (85). This enzyme, as for most class B1 β-lactamases, confers resistance to penicillins, broad-spectrum cephalosporins, and carbapenems but spares aztreonam (85). It is not inhibited by clavulanate or avibactam but is inhibited in vitro by EDTA. Purified enzyme is able to hydrolyze all tested β-lactams except for aztreonam (Figure 2; Table 2). Crystal structures of GIM-1 revealed that the active site is narrower in comparison to VIM-1 but possessed flexibility in two loops likely explaining its wide variety of substrates (86). The blaGIM−1 gene was then identified in S. marcescens from a German patient over a 20-month period (87). After this first occurrence in S. marcescens, the blaGIM−1 gene was described in E. cloacae, K. oxytoca, E. coli, C. amalonaticus, and C. freundii (88–92). To date, this carbapenemase has spread only in Germany. Analysis of the genetic context revealed that blaGIM−1 is part of class 1 integron (85, 88, 89). This gene was carried by different conjugative plasmids but not typeable by PBRT (88, 90). Until now, only one variant, GIM-2, of this carbapenemase has been described in E. cloacae in Germany (93).
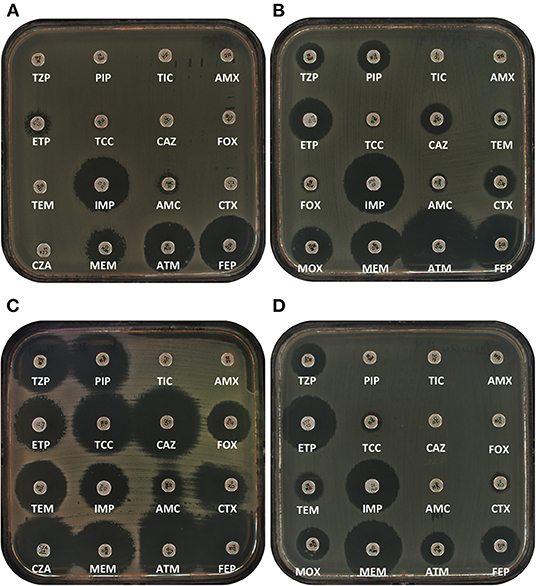
Figure 2. Antibiograms of representative class B carbapenemases. (A) GIM-1-producing E. cloacae complex clinical isolate. (B) TMB-1 producing E. coli transconjugant. (C) SFH-1-producing S. marcescens clinical isolate. (D) LMB-1-producing C. freundii clinical isolates. AMX, Amoxicillin; AMC, Amoxicillin/clavulanate; ATM, aztreonam; CAZ, ceftazidime; CTX, Cefotaxime; CZA, Ceftazidime/avibactam; ETP, Ertapenem; FEP, Cefepime; FOX, Cefoxitine; IPM, Imipenem; MEM, Meropenem; PIP, Piperacillin; TCC, Ticarcillin/Clavulanate; TEM, Temocillin; TIC, Ticarcillin; TZP, Piperacillin/Tazobactam.
KHM-1
KHM-1, for Kyorin Hospital Metallo-β-lactamase, is a class B1 metallo-β-lactamase identified in a clinical isolate of C. freundii in Japan (94). This carbapenemase confers resistance to penicillins, broad-spectrum cephalosporins, and carbapenems but spares aztreonam (94). This enzyme was purified and confirmed the hydrolytic properties observed for the recombinant strain (Table 2). The blaKHM−1 gene has never been described out of Japan. However, this gene was present not only in clinical settings but also in urban sewage that might indicate a spread in the community. This carbapenemase has been identified in C. freundii, K. quasipneumoniae, and E. hormaechei subsp. hoffmannii (94–96). Genetic analysis revealed that blaKHM−1 is not part of class 1 integron or “classical” transposon. In C. freundii, genes of unknown function bracketed the blaKHM−1 (94). In K. quasipneumoniae and E. homaechei, a copy of ISEc68 and IS5 have been identified, respectively (95, 96). The blaKHM−1 has been identified on different large IncA/C-type plasmids.
TMB-1
TMB-1, for Tripoli Metallo-β-lactamase, has been described from an environmental isolate of Achromobacter xylosoxidans in Libyan hospital in Tripoli, Libya (97). Since then, this carbapenemase have been identified in A. baumannii and Acinetobacter calcoaceticus in Japan (98). Of note, a point derivative variant of TMB-1, TMB-2, possessing the substitution S228P, has been described in Acinetobacter pittii and Acinetobacter genomospecies 14 BJ also in Japan (99). The production of TMB-1 confers resistance to penicillins/inhibitor combinations and broad-spectrum cephalosporins but spares aztreonam, as classically observed with class B1 enzymes. Of note, MIC for meropenem is higher than for imipenem with TMB-1 (MICs at 1 mg/L vs. 32 mg/L for imipenem and meropenem, respectively). This difference was not observed with TMB-2 for which MICs to imipenem and meropenem were similar (MICs at 2 mg/L) (99). To date, only one report of TMB-1 is available in Enterobacterales from France (100). Two TMB-1-producing clinical isolates of E. hormaechei and C. freundii were recovered from a patient previously hospitalized in Tunisia (Figure 2). The blaTMB−1 gene is embedded in class 1 integron as a gene cassette always in the first position. In A. xylosoxidans and Acinetobacter, it was likely carried on the chromosome whereas it was carried by an IncN-type plasmid in Enterobacterales (100).
SFH-1
SFH-1, for Serratia fonticola carbapenem hydrolase, is a particular case since it corresponds to the sole characterized metallo-β-lactamase belonging to B2 subclass in Enterobacterales. This gene has been only described in S. fonticola in Portugal (101, 102). However, no extensive genetic analysis was performed to characterize the genetic context of this gene. Interestingly, the phenotype conferred by the production of this enzyme is peculiar. Indeed, it confers resistance to carbapenems but neither to cephalosporins nor penicilins (Figure 2; Table 1).
AIM-1
AIM-1, for Adelaide imipenemase, was described in clinical isolates of P. aeruginosa in Adelaide, Australia, in 2002 (103). Very few reports of AIM-1 were available in the literature. The blaAIM−1 gene has been detected in urban wastewater in West Africa and in China (104, 105). The unique description in Enterobacterales corresponds to a AIM-1-producing K. pneumoniae in China (104). AIM-1 producing K. pneumoniae was resistant to penicillins, except piperacillin, to broad-spectrum cephalosporins and carbapenems, but remained susceptible to aztreonam. Crystal structure of AIM-1 revealed that the active site is narrower than other class B3 carbapenemases (106). However, this particular conformation might explain the higher efficiency compared to other B3. The genetic context of blaAIM−1 revealed its association with ISCR5, an insertion sequence moving by rolling circle transposition (103). However, the acquisition mechanism remains poorly understood. In K. pneumoniae, no information related to the genetic context is available in the manuscript.
LMB-1
LMB-1, for Linz Metallo-β-lactamase, is the last metallo-β-lactamase described in Enterobacterales (107). This carbapenemase has been firstly identified in E. cloacae in an Austrian patient hospitalized in Salzburg, Austria, in 2013. LMB-1 belongs to class B3 and the closest clinically relevant carbapenemase corresponds to AIM-1 (42% amino acid identity). However, analysis of available β-lactamase in genbank database indicated that LMB-1 presented 99% amino acid identity with a predicted β-lactamase from marine bacteria Rheinheimera pacifica (107). LMB-1-producing E. cloacae was resistant to penicillins, broad-spectrum cephalosporins, and carbapenems. Recently this carbapenemase has been identified in a clinical isolate of C. freundii in Buenos Aires, Argentina (Figure 2) (108). Until now, LMB-1 has not been purified due to some difficulty to express this carbapenemase (108). However, in both studies, specific activities indicated that LMB-1 hydrolyzed all β-lactams except aztreonam and cefepime. Analysis of the genetic context showed that blaLMB−1 is embedded in a complex genetic structure with different class 1 integrons. Immediately downstream of the blaLMB−1 gene, an ISCR1 is present, whereas a putative phosphodiesterase is found upstream. This phophodiesterase is likely part of the genome of the progenitor. In both isolates, the genetic context is similar but differs in the gene cassette arrays of class 1 integrons. However, in the Austrian isolate, two copies of IS6-family ISs bracket the whole structure forming a putative composite transposon. The blaLMB−1 is carried on conjugative plasmids. Nevertheless, these two plasmids do not belong to same incompatibility group being IncFIb-like in Austria and IncA/C in Argentina. This is an interesting phenomenon since its identification in distant geographic areas on different plasmids might indicate that this gene has spread more than expected.
Minor Class c Carbapenemases
Class C β-lactamases, also known as cephalosporinases, have been rarely reported as carbapenemases. To date, only two β-lactamases were described possessing a carbapenemase activity being CMY-10 and more recently ACT-28 (109, 110).
CMY-10
CMY-10 is a point variant of CMY, the natural cephalosporinase of Citrobacter spp. identified in Enterobacter aerogenes (111). CMY-10 conferred high levels of resistance to penicillins and penicillin/inhibitor combinations and cephalosporins such as cefoxitin, cephalothin, or ceftazidime. This variant was categorized as an extended-spectrum AmpC (ESAC) due to increased MICs toward carbapenems and aztreonam (Table 1). Carbapenem hydrolysis properties were explained by a widened active site as compared to P99 AmpC due to a three amino acids deleted in R2 loop (109). The blaCMY−10 gene was carried on a conjugative plasmid associated to a complex class 1 integron (112). It is usually located at the 3' end of integron and associated with ISCR1 leading to its expression. This β-lactamase have exclusively been reported in Korea (111–113). However, its prevalence may be underestimated. Indeed, this variant cannot be detected without sequencing and is not targeted by most detection tools available in the market. Regarding the treatment of this ESAC, scarce information is available. Avibactam is active against class C β-lactamases but it has been demonstrated that mutation in Ω-loop can increased MICs of ceftazidime-avibactam (114). Another study demonstrated that nucleotides guanosine monophosphate (GMP) and inosine monophosphate (IMP) are potential inhibitors for this enzyme (115).
ACT-28
The other class C β-lactamase with potential carbapenemase activity is ACT-28 (110). ACT-1 was firstly identified in a carbapenem resistant K. pneumoniae in US (116). Lately, it has been demonstrated that the progenitor of blaACT−like genes was the Enterobacter genus (117). ACT-28 was firstly identified in eight carbapenem non-susceptible Enterobacter kobei sent to the French National Reference Centre for antimicrobial resistance (110). All isolates presented a positive carbapenem hydrolysis using the Carba-NP test. ACT-28-producing E. kobei isolates were resistant to penicillins, to penicillin/inhibitor combination, to broad-spectrum cephalosporins except cefepime, to aztreonam, but remained susceptible to carbapenem according EUCAST guidelines (Figure 3) (110). Purified ACT-28 exhibits low catalytic activity for imipenem (kcat = 0.025 s−1) but presents a high affinity (Km = 1.9 μM) resulting in a catalytic efficiency (kcat/ Km) at 0.013 μM−1.s−1, which is twice the value of ACT-1 purified in parallel. This small difference of catalytic efficiency might explain the positivity of detection tests based on imipenem hydrolysis. Furthermore, despite the fact that this catalytic efficiency is low, it is in the same range as other carbapenemases such as OXA-23 (Table 2). Regarding the genetic context, the blaACT−28 was carried on the chromosome of a lineage of E. kobei ST-125. No mobile element was identified at the vicinity of this gene, indicating that this gene was not acquired but belongs to the core genome of this lineage. Search in the NCBI database identified this gene in different countries, including Brazil, USA, and UK, always in E. kobei of ST-125.
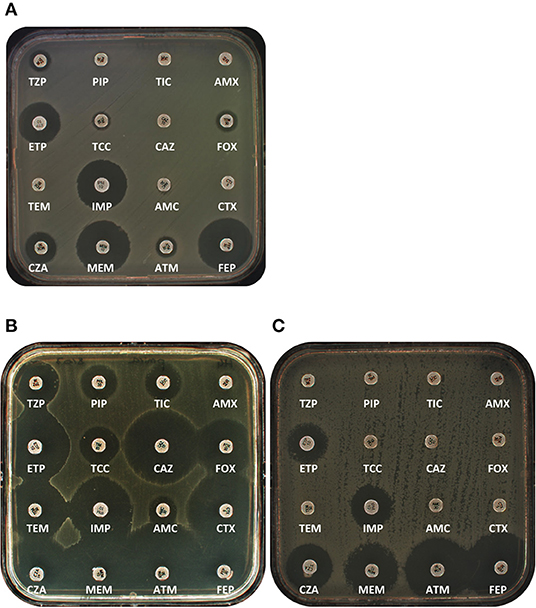
Figure 3. Antibiograms of representative class C & D carbapenemases. (A) ACT-28-producing E. kobei clinical isolate. (B) OXA-23 producing P. mirabilis clinical isolate. (C) OXA-372-producing C. freundii clinical isolates. AMX, Amoxicillin; AMC, Amoxicillin/clavulanate; ATM, aztreonam; CAZ, ceftazidime; CTX, Cefotaxime; CZA, Ceftazidime/avibactam; ETP, Ertapenem; FEP, Cefepime; FOX, Cefoxitine; IPM, Imipenem; MEM, Meropenem; PIP, Piperacillin; TCC, Ticarcillin/Clavulanate; TEM, Temocillin; TIC, Ticarcillin; TZP, Piperacillin/Tazobactam.
Minor Class d Carbapenemases
In Enterobacterales, the main class D carbapenemase is OXA-48 that has spread worldwide with endemic area in Mediterranean countries with the exception of Israel, Greece, and Italy for which KPC-like enzymes represent the main carbapenemase. In this part of the manuscript, we will focus on the class D carbapenemases other than OXA-48-like enzymes. We can divide these enzymes in two groups: (i) carbapenemases firstly identified in other bacterial families before secondary spreading in Enterobacterales (OXA-23, OXA-40, OXA-58, & OXA-198) and (ii) carbapenemases identified firstly in Enterobacterales (OXA-372 & OXA-427).
OXA-23
The blaOXA−23 gene codes for the main carbapenemase identified in Acinetobacter genus (118). In Acinetobacter spp. OXA-23 production confers high level resistance to all β-lactams. The blaOXA−23 gene was identified on many different conjugative plasmids, including GR6 incompatibility group, the most distributed plasmid family in Acinetobacter baumannii (119). The blaOXA−23 gene is most often found several transposons associated with ISAba1 (e.g., Tn2006 or Tn2008) or ISAba4 (Tn2007) (120, 121). This cabapenemase has been reported worldwide but until 2002, it has never been described out of the Acinetobacter genus. The first report of OXA-23-producing Enterobacterales was published in 2002 with the description of 10 isolates recovered from 1996 to 1999 in a French hospital. Clonal relationship identified that all these isolates were clonally related despite they were recovered over a 4-year period without any link between patients (122). Since then, several report of OXA-23-producing P. mirabilis were reported in France, Singapore, and Finland (123–125). Intriguingly, the genomes of 20 OXA-23-producing P. mirabilis isolates recovered from France and Belgium have been compared to all Proteus genomes available in the NCBI database. This study highlighted that all isolates belonged to a single lineage that has spread since 1996 (126). Moreover, veterinary samples were included in this study and revealed that this lineage has spread also in animals (126). Analysis of the genetic context revealed that the blaOXA−23 gene is mainly carried on the chromosome of P. mirabilis (122, 124, 127) except for one isolate from France and three isolates from Singapore. There, the blaOXA−23 gene has been identified carried on an AbaR4-like structure on an untypeable plasmid (125). The AbaR4 structure is composed of Tn2006, carrying the blaOXA−23 gene, embedded in a complex transposon usually inserted in comM gene on the chromosome of A. baumannii (128). Regarding the other isolates, the blaOXA−23 is carried by complex IS26-based transposon. However, it is always associated to ISAba1 in its 5' extremity leading to its expression (126). OXA-23-producing P. mirabilis can be hard to detect. Indeed, MICs to carbapenems are relatively low and may be hidden by the low affinity of PLP for imipenem in Proteus. A moderate increase of carbapenem MICs can be observed from 0.25 to 0.5 mg/L but the presence of this carbapenemase should be evocated mainly in case of an increase of MICs for amoxicillin-clavulanate combination (Figure 3) (129). In addition to the difficulty to detect a phenotype associated to the production of OXA-23, most of carbapenemase detection tests for Enterobaterales do not detect carbapenem hydolyzing class D β-lactamases (CHDLs) other than OXA-48 (126).
OXA-24/40
The blaOXA−24/−40 was firstly identified in Acinetobacter baumannii isolates from Spain and then described in several countries (130). Only one description of OXA-24-producing P. mirabilis is reported to date (131). No information is available regarding the genetic context of blaOXA−24/−40, except the impossibility to transfer the carbapenemase by conjugation.
OXA-58
The last major CHDL frequently identified in Acinetobacter is OXA-58. This carbapenemase was firstly identified in France in 2003 (132) but then has been reported worldwide in Acinetobacter genus (118). Since recently, this carbapenemase was limited to Acinetobacter spp. The first report of OXA-58 in Enterobacterales was published in 2013 from Sierra Leone (133). However, no genetic is available with these isolates. Intriguingly, in addition to the blaOXA−58 gene, some K. pneumoniae possessed the blaOXA−51-like gene also, which is the natural β-lactamase of A. baumannii, suggesting a misidentification of the bacterial isolates or a contamination with Acinetobacter DNA. Since then, three recent studies regarding OXA-58-producing P. mirabilis from Belgium, Poland, and Germany were published (134–136). Genetic analysis revealed that isolates from Poland and Germany shared the same genetic context. The blaOXA−58 was carried by a small untypeable plasmid of 6.2 kb also carrying aadA14 aminoglycoside resistance gene (135, 136). On the other hand, the Belgium isolate possessed a peculiar genetic context. The blaOXA−58 gene was localized on the chromosome associated to a class C β-lactamase ampC gene and was repeated in tandem (134). In all isolates, fragments of ISAba3-like were identified at the vicinity of blaOXA−58 as observed in Acinetobacter. ISAba3 was described as the probable mobile element at the origin of the expression of blaOXA−58 in Acinetobacter (137). It is likely that the same promoter was conserved in P. mirabilis explaining the conserved fragments of ISAba3.
OXA-198
OXA-198 is a CHDL initially described in P. aeruginosa (138). After this sole occurrence, it was recently described in a Citrobacter pasteurii clinical isolate from France (139). In P. aeruginosa, blaOXA−198 is carried on an IncP11 plasmid of 49 kb (140), but in C. pasteurii, it was carried on an IncHI-type of 183 Kb (139). In both cases, blaOXA−198 is embedded in a class 1 integron. In P. aeruginosa, blaOXA−198 is the second cassette whereas in C. freundii, it corresponds to the third cassette (139). To date, this carbapenamase has been identified only in France and Belgium. This carbapenemase confers only a slight decrease of susceptibility to carbapenems (MICs of 0.38 mg/L, 0.5 mg/L, and 1 mg/L for meropenem, ertapenem, and imipenem, respectively in the C. pasteurii clinical isolate) (Table 1). In addition, this carbapenemase confers resistance to penicillins/inhibitor combination but spares ceftazidime. Catalytic constants confirmed this resistance phenotype with hydrolysis of penicillins and carbapenems but no activity toward broad-spectrum cephalosporins (Table 2).
OXA-372
OXA-372 belongs to a new family of CHDLs described in Enterobacterales (141). This carbapenemase has been identified in C. freundii recovered from hospital wastewater in Italy (141). OXA-372 confers resistance to penicillins and diminished susceptibility to carbapenems but not to broad-spectrum cephalosporins, a common phenotype associated to the production of CHDLs (Figure 3; Table 1). Kinetic parameters confirmed that this enzyme is able to hydrolyze penicillins and carbapenems but not broad-spectrum cephalosprins and aztreonam (Table 2). Analysis of the genetic context revealed that the blaOXA−372 gene was carried by a multireplicon plasmid (IncA/C & IncN). This gene was embedded into a complex structure made of reminiscence of Tn6017 itself inserted in Tn6256, a Tn3-family transposon.
OXA-427
The last family of CHDL described in Enterobacterales corresponds to OXA-427 (142). This carbapenemase has been described in Belgium from various Enterobacterales being K. pneumoniae, E. coli, K. oxytoca, S. marcescens, and Providencia rettgeri. This carbapenemase confers resistance to penicillins including temocillin, ceftazidime, aztreonam, and ertapenem but spares cefotaxime (Table 1). Genetic analysis revealed that blaOXA−427 was localized on an IncA/C-type plasmid of 177kb (142). Immediate genetic context is made of a class 1 integron upstream of blaOXA−427 and a copy of IS1326 dowstream (142). A recent study identified the blaOXA−427 on a multi-replicon plasmid IncA/C-InFIb-like plasmid (143). This plasmid of 321 kb actually resulted of the co-integration of the IncA/C-type plasmid carrying blaOXA−427 and an IncFIb-like plasmid (143). Biochemical analysis confirmed that OXA-427 is able to hydrolyze ceftazidime and imipenem (142), and was inhibited by avibactam (144).
Detection Methods for Rare Carbapenemases
Since it is difficult (or impossible) to prevent the emergence of carbapenemases and more generally resistance genes, the most powerful method to bend their spread is to detect them. Indeed, earliest detection associated to hygiene measures and antimicrobial stewardship are our armamentarium against those bugs (145–147).
Mirroring the spread of carbapenemases numerous detection tools have emerged. These tests may be classified in different families based on their detection technology: (i) phenotypic tests, (ii) enzymatic tests based on hydrolysis, (iii) immuno-chromatographic assays, and (iv) molecular tests. This part of the manuscript will focus on the abilities of these tests to specifically detect rare carbapenemases and not on their global performance. Of note, taking in account the low number of isolates producing these rare cabapenemases, sensitivity and specificity might be false.
Electrochemical Assay
Among the biochemical tests, the BYG test, named after the name of developers, is an electrochemical assays able to detect carbapenemase activity via a variation of conductivity during the carbapenem hydrolysis (148). During its multicenter evaluation, the BYG test was able to detect all GIM-1 (n = 1), FRI-1 (n = 1), SME-like (n = 2) producers. However, only 5/7 IMI/NMC-A producing isolates and no GES-5 producers (0/4) could be detected (148).
Colorimetric Biochemical Assays
Different biochemical colorimetric tests based on the hydrolysis of carbapenem have been developed. The first test, the Carba-NP test, can detect imipenem hydrolysis via the production of acidic derivatives of imipenem (149). This test, along with the commercial test RAPIDEC® Carba NP (Biomérieux, France), has been extensively tested on major carbapenemases (149–151). Carba NP test was able to detect IMI/NMC-A-like, SME-like, FRI-1, and GIM-1 enzymes but failed to detect some GES-5 producing isolates (151). Another test based on colorimetric changes after hydrolysis of a chromogenic β-lactam is the β-CARBA™ test (Biorad, France) (152). This test showed similar sensitivity/specificity for the detection of the main carbapenemases compared to the Carba NP test and its commercial version, the RAPIDEC® Carba NP (152). It was also efficient in the detection of GIM-1. But it systematically failed to detect all minor class A carbapenemases including IMI-/NMC-A-like, SME-like, GES-5, and FRI-1 (151, 153). This result might be explained by the fact that the chromogenic β-lactam included in the β-CARBA™ test do not correspond to a true carbapenem, but a broad-spectrum cephalosporin that is likely not hydolzyzed by these minor class A carbapenemases.
MALDI-TOF Based Detection of Carbapenem Hydrolysis
The last group of β-lactam hydrolysis-based detection assay correspond to the use of MALDI-TOF for the detection of a carbapenemase activity (154). Several detection tests using MALDI-TOF were developed including only one commercial kit, the MBT STAR® Carba IVD Kit (Brucker). These tests are based on the detection of (i) the disappearance of the carbapenem peak and (ii) the concomitant appearance of the peak corresponding of the hydrolyzed carbapenem after incubation of the carbapenemase-producing bacteria in a carbapenem supplemented solution. Overall, these tests showed good sensitivity/specificity for the detection of the main carbapenemases (154, 155). A multicentric evaluation of two methods, an in-house MALDI-TOF based protocol and the MBT STAR® Carba IVD Kit, demonstrated that the two methods were able to efficiently detect IMI-/NMC-A-like, SME-like, GES-5, GIM-1, and FRI-1 producing isolates (155).
Hodge Test
One of the first phenotypic tests used for the detection of carbapenemase producers is the modified Hodge test, as known as cloverleaf test (156). Due to high number of false positive results as well as the weak sensitivity, this test is no longer considered as a good alternative for the detection of CPE including rare carbapenemase (156, 157). Of note, another modified Hodge test, Triton Hodge test, was developed by addition of Triton X-100 during the process (158). This test demonstrated good sensitivity to detect carbapenemases including NMC-A, SME-1, and GES-5 (produced by P. aeruginosa) (158).
CIM Test
Another phenotypic test based on indirect detection of carbapenemase production corresponds to CIM test, for Carbapenem Inactivation Method, and derivatives (159). The aim of this test is to detect the ability of a carbapenem susceptible bacteria to grow close to a carbapenem containing disc after incubation of this disc with the suspected carbapenemase-producing bacteria. In a retrospective and prospective evaluation, this test revealed a good specificity and sensitivity for the detection of IMI-1/-2/-3, NMC-A, SME-1/-2, FRI-1, GIM-1, GES-5, and OXA-372 producers (160). The mCIM, modified Carbapenem Inactivation Method, corresponds to a CIM test for which water was replaced with trypticase soy broth during the incubation phase between the carbapenem containing disc and the tested strain, and the time was extended (161). This test was able to detect the rare carbapenemases tested being NMC-A-like and SME-like enzymes (161). One of the most critical features with these tests is the 24 h delay to obtain the results. Another CIM derivative, rCIM for rapid Carbapenem Inactivation Method, was developed to target carbapenemase production in Enterobacterales (162). This test used a nephelometer to evaluate the growth of the susceptible bacteria instead of using a plate allowing a faster evaluation of the growth (few hours instead of 24 h). This tests accurately detected FRI-1, GES-5, IMI-1/-2, SME-1/-2, GIM-1, and OXA-372 (162).
Inhibition Phenotypic Tests
Among the phenotypic detection tests, a wide diversity of combined disk methodologies has been developed. The combined disk assays are based on the use of carbapenem impregnated disk associated to different inhibitors such as boronic acid, dipicolinic acid, and cloxacillin, inhibiting class A, B, and C β-lactamases, respectively. As observed with the CIM and the mCIM, a 24 h delay is required to obtain results. Few studies tested the accuracy of this test on rare carbapenemases. Recently, the Carbapenemase Detection Set® (MAST Diagnostic) was evaluated (163). This assay accurately detected IMI-/NMC-A-like, SME-1/-2, and FRI-1 as class A carbapenemase, GIM-1 as a class B, but complementary tests were needed to decipher the presence of GES-5 (due to the absence of any diameter differences with all tested inhibitors) (164). Other combined disc methods are commercially available but were not evaluated on rare carbapenemase-producing Enterobacterales.
Lateral Flow Immunoassays
Recently, lateral flow immunoassays have been developed for the detection of the main carbapenemases. The main tests are RESIST-4 O.K.V.M (Coris Bioconcept), which detect KPC, NDM, VIM, OXA-48-like enzymes, and NG-Test® CARBA 5 (NG Biotech), which detects KPC, NDM, VIM, OXA-48-like, and IMP enzymes. Both of these tests can deliver results in <15 min when performed on bacterial colonies (165–167) or directly from positive blood cultures (168, 169). Despite both of these tests possess excellent performance for the detection of the “Big five” carbapenemases encountered in Enterobacterales, none of the rare carbapenemase are included in the detection panel yet. More recently, the OXA-23 K-Set® (Coris Bioconcept) has been developed for the detection of OXA-23-producing Acinetobacter spp. However, OXA-23 is also rarely identified in P. mirabilis. Accordingly, a recent evaluation demonstrated that OXA-23 K-Set® accurately detects OXA-23-producing P. mirabilis (170).
Molecular Detection of Carbapenemase Encoding Genes
The last group of detection tests gathers the molecular test in which PCR is the warhorse for the detection of carbapenemase encoding genes. By contrast to biochemical tests that detect carbapenemase activity, molecular tests detect the presence of a specific gene. Thus, the main caution of these tests is “we are able to detect only what we target.” One of the most worldwide spread molecular assay for the detection of carbapenemase encoding genes corresponds to the GeneXpert® (Cepheid) (171). The current version Carba-R V2 is able to detect accurately and “Big Five” carbapenemase encoding genes but none of the rare carbapenemase encoding ones (172). Currently, this issue is common for most of PCR-based detection kit including Revogene (Meridian bioscience), Biofire filmArray Blood Culture identification panel (BioMérieux) Amplidiag CarbaR+MCR (Mobidiag), Luminex xTAG assay (Luminex corp), Check-MDR CT103 (Check-Points Health), or CRE ELITe MGB® (Elitech) kits (173–177). Interestingly, the blaOXA−23 and blaOXA−58 genes are detected by Amplidiag CarbaR+MCR kit.
Whole genome sequencing, despite not based directly on PCR, is a molecular method very useful for precise identification of resistance mechanism to carbapenems. The main weakness of this technology is that a genotype may not explain a phenotype and vice versa. Indeed, the presence of a gene does not necessarily prove its expression. That is the reason why in silico antibiogram is not widely used yet (178). However, this method is able to detect any gene related to β-lactamases whatever its homology or phenotype as well as undetected or totally novel β-lactamase family. Moreover, servers for analysis of WGS raw data are not appropriate for an easy interpretation of the data, e.g., Resfinder (179), CARD (180).
β-Lactamase Inhibitors and Rare Carbapenemases
The use of β-lactamase inhibitors is crucial to fight β-lactamase-producing isolates. Among the well-known inhibitors, the three inhibitors of class A β-lactamases, clavulanate, tazobactam, and sulbactam, are widely used in clinical practice (181). Unfortunately, carbapenem-resistant Enterobacterales (and particularly carbapenemase-producing Enterobacterales) are most often resistant to the classical β-lactam/β-lactamase inhibitors associations (e.g., amoxiciline-clavulanate, ticarcilline-clavulanate, piperacilline-tazobactam, ceftolozane-tazobactam) used to treat infected patients. Thus, an urgent need for new inhibitors was obvious. Recently, several inhibitors have been developed. Among them three inhibitors are now approved or in phase 3, avibactam (belonging to diazibyciclooctanone DBO), relebactam (DBO), and vaborbactam (boronic acid derivative) (182). Avibactam, formerly NXL-104, in combination with ceftazidime, proved its efficacy against class A, C, and some class D but not against class B β-lactamases (183). Among rare carbapenemases, avibactam demonstrated efficacy against CTX-M-33-, GES-5-, SME-2-producing isolates (42, 184). To this short list, zidebactam, a new DBO member, can be added (185), nacubactam, a bridged DBO, enmetazobactam, belonging to penicillanic acid sulfone class, taniborbactam a boronic acid derivative (186–188). Avibactam inhibits class A, C, and D whereas relebactam and vaborbactam mainly inhibit class A and C (189). Activity of vaborbactam in combination with meropenem has been described toward some rare class A carbapenemase such as SME-, NMC-A-, FRI-1-, and BKC-1-producing isolates (190). However, this combination has limited activity against class B (NDM-, VIM-, or IMP-producing isolates) and class D (OXA-48-like-producing isolates) (191). Relebactam demonstrated inhibition toward KPC-, SHV-, CTX-M-,TEM-, or class C-β-lactamases but exhibited moderate inhibition against OXA-48-like (183). However, it has been observed that, despite activity against class A carbapenemases such as KPC, relebactam does not inhibit SME-4 enzyme (192). A GES-20-producing K. pneumoniae resistant to imipenem-relebactam was also reported (193). Cefepime-enmetaozactam, formerly AAI101, demonstrated activity against ESBLs- or AMPc-producing isolates but limited activity toward KPC- and VIM-producing isolates (183). No data regarding its activity against minor carbapenemase is available. Zidebactam (formerly WCK 5107) exhibited activity against class A and B carbapenemases and moderate inhibition activity OXA-23/-40/-58-producing A. baumannii (194). Noticeably, zidebactam also inhibits PBP2 and thus possess intrinsic antibacterial activity (183). Among minor carbapenemases, cefepime/zidebactam demonstrated activity against a GES-18-producing isolate (194). Nacubactam, formerly FPI-1465, demonstrated in vitro inihibition against class A, C, and some class D β-lactamases (183). As observed for zidebactam, Nacubactam demonstrated affinity to PBP2 and thus also exhibited activity toward MBL-producing isolates (188). This molecule remains to be tested for minor carbapenemases. Taniborbactam, formerly VNRX-5133, is able to inhibit class A, C, and D β-lactamases and even class B carbapenemases (187, 195). Among MBLs inhibited by VIM-, NDM-, SPM-1-producing isolates but not IMP-like enzyme (187). Of note, GIM-1 and GES-5 are inhibited by taniborbactam (195).
Conclusions
Since the first description of “an enzyme able to destroy penicillin,” thousands of β-lactamases were identified from more than 50 families. The wide genetic diversity associated to very diverse phenotypes largely complicate the identification of the resistance mechanisms involved in carbapenem resistant Enterobacterales. Although several tools have been developed for the accurate detection of the 5 main carbapenemases, KPC, NDM, VIM, IMP, and OXA-48-like, it might be now interesting to developed multiplex tests (molecular tests or immunochromatographic assays) that will be able to fill the gap in the detection of rare carbapenemase-encoding Enterobacterales. It might be of particular interest for Ambler class A carbapenemases of GES-, IMI-, SME-, and FRI-type that have already been reported in different countries, and for which some of the widely used colorimetric biochemical tests (e.g., β-CARBA™ test) remain inefficient. Without efficient detection tools, these enzymes might be a concern in a near future in healthcare facilities.
Author Contributions
All authors listed have made a substantial, direct and intellectual contribution to the work, and approved it for publication.
Conflict of Interest
The authors declare that the research was conducted in the absence of any commercial or financial relationships that could be construed as a potential conflict of interest.
References
1. Abraham EP, Chain E. An enzyme from bacteria able to destroy penicillin. 1940. Rev Infect Dis. (1988) 10:677–8.
2. Ambler RP. The structure of β-lactamases. Philos Trans R Soc Lond, B, Biol Sci. (1980) 289:321–31. doi: 10.1098/rstb.1980.0049
3. Bush K, Jacoby GA. Updated functional classification of β-lactamases. Antimicrob Agents Chemother. (2010) 54:969–76. doi: 10.1128/AAC.01009-09
4. Nordmann P, Dortet L, Poirel L. Carbapenem resistance in Enterobacteriaceae: here is the storm! Trends Mol Med. (2012) 18:263–72. doi: 10.1016/j.molmed.2012.03.003
5. Queenan AM, Bush K. Carbapenemases: the versatile β-lactamases. Clin Microbiol Rev. (2007) 20:440–58. doi: 10.1128/CMR.00001-07
6. Nordmann P, Cuzon G, Naas T. The real threat of Klebsiella pneumoniae carbapenemase-producing bacteria. Lancet Infect Dis. (2009) 9:228–36. doi: 10.1016/S1473-3099(09)70054-4
7. David S, Reuter S, Harris SR, Glasner C, Feltwell T, Argimon S, et al. Epidemic of carbapenem-resistant Klebsiella pneumoniae in Europe is driven by nosocomial spread. Nat Microbiol. (2019) 4:1919–29. doi: 10.1038/s41564-019-0492-8
8. Adeolu M, Alnajar S, Naushad S, Gupta SR. Genome-based phylogeny and taxonomy of the “Enterobacteriales”: proposal for Enterobacterales ord. nov. divided into the families Enterobacteriaceae, Erwiniaceae fam. nov., Pectobacteriaceae fam. nov., Yersiniaceae fam. nov., Hafniaceae fam. nov., Morganellaceae fam. nov., and Budviciaceae fam. nov. Int J Syst Evol Microbiol. (2016) 66:5575–99. doi: 10.1099/ijsem.0.001485
9. Tindall BJ, Sutton G, Garrity GM. Enterobacter aerogenes Hormaeche and Edwards 1960 (Approved Lists 1980) and Klebsiella mobilis Bascomb et al. 1971 (Approved Lists 1980) share the same nomenclatural type (ATCC 13048) on the Approved Lists and are homotypic synonyms, with consequences for the name Klebsiella mobilis Bascomb et al. 1971 (Approved Lists 1980). Int J Syst Evol Microbiol. (2017) 67:502–4. doi: 10.1099/ijsem.0.001572
10. Iversen C, Mullane N, McCardell B, Tall BD, Lehner A, Fanning S, et al. Cronobacter gen. nov., a new genus to accommodate the biogroups of Enterobacter sakazakii, and proposal of Cronobacter sakazakii gen. nov., comb. nov., Cronobacter malonaticus sp. nov., Cronobacter turicensis sp. nov., Cronobacter muytjensii sp. nov., Cronobacter dublinensis sp. nov., Cronobacter genomospecies 1, and of three subspecies, Cronobacter dublinensis subsp. dublinensis subsp. nov., Cronobacter dublinensis subsp. lausannensis subsp. nov. and Cronobacter dublinensis subsp. lactaridi subsp. nov. Int J Syst Evol Microbiol. (2008) 58:1442–7. doi: 10.1099/ijs.0.65577-0
11. Davin-Regli A, Lavigne J-P, Pagès J-M. Enterobacter spp.: update on taxonomy, clinical aspects, and emerging antimicrobial resistance. Clin Microbiol Rev. (2019) 32. doi: 10.1128/CMR.00002-19
12. Rasmussen BA, Bush K, Keeney D, Yang Y, Hare R, O'Gara C, et al. Characterization of IMI-1 β-lactamase, a class A carbapenem-hydrolyzing enzyme from Enterobacter cloacae. Antimicrob Agents Chemother. (1996) 40:2080–6. doi: 10.1128/AAC.40.9.2080
13. Nordmann P, Mariotte S, Naas T, Labia R, Nicolas MH. Biochemical properties of a carbapenem-hydrolyzing β-lactamase from Enterobacter cloacae and cloning of the gene into Escherichia coli. Antimicrob Agents Chemother. (1993) 37:939–46. doi: 10.1128/AAC.37.5.939
14. Teo JWP, La M-V, Krishnan P, Ang B, Jureen R, Lin RTP. Enterobacter cloacae producing an uncommon class A carbapenemase, IMI-1, from Singapore. J Med Microbiol. (2013) 62:1086–8. doi: 10.1099/jmm.0.053363-0
15. Cuzon G, Levy M, Jacob E, Dortet L, Naas T. IMI-1-producing Enterobacter cloacae clinical isolate from Tahiti, French Polynesia. J Glob Antimicrob Resist. (2016) 5:1–2. doi: 10.1016/j.jgar.2016.01.005
16. Tarumoto N, Kodana M, Watanabe N, Sakai J, Imai K, Yamaguchi T, et al. First report of the isolation of blaIMI-1-producing colistin-heteroresistant Enterobacter cloacae in Japan, September 2016. J Infect Chemother. (2018) 24:941–3. doi: 10.1016/j.jiac.2018.04.004
17. Miltgen G, Bonnin RA, Avril C, Benoit-Cattin T, Martak D, Leclaire A, et al. Outbreak of IMI-1 carbapenemase-producing colistin-resistant Enterobacter cloacae on the French island of Mayotte (Indian Ocean). Int J Antimicrob Agents. (2018) 52:416–20. doi: 10.1016/j.ijantimicag.2018.05.015
18. Aubron C, Poirel L, Ash RJ, Nordmann P. Carbapenemase-producing Enterobacteriaceae, U.S. Rivers. Emerg Infect Dis. (2005) 11:260–4. doi: 10.3201/eid1102.030684
19. Hopkins KL, Findlay J, Doumith M, Mather B, Meunier D, D'Arcy S, et al. IMI-2 carbapenemase in a clinical Klebsiella variicola isolated in the UK. J Antimicrob Chemother. (2017) 72:2129–31. doi: 10.1093/jac/dkx103
20. Piedra-Carrasco N, Fàbrega A, Calero-Cáceres W, Cornejo-Sánchez T, Brown-Jaque M, Mir-Cros A, et al. Carbapenemase-producing enterobacteriaceae recovered from a Spanish river ecosystem. PLoS ONE. (2017) 12:e0175246. doi: 10.1371/journal.pone.0175246
21. Zhang F, Wang X, Xie L, Zheng Q, Guo X, Han L, et al. A novel transposon, Tn6306, mediates the spread of blaIMI in Enterobacteriaceae in hospitals. Int J Infect Dis. (2017) 65:22–6. doi: 10.1016/j.ijid.2017.09.014
22. Hartl R, Kerschner H, Gattringer R, Lepuschitz S, Allerberger F, Sorschag S, et al. Whole-genome analysis of a human Enterobacter mori isolate carrying a blaIMI-2 carbapenemase in Austria. Microb Drug Resist. (2019) 25:94–6. doi: 10.1089/mdr.2018.0098
23. Laurens C, Jean-Pierre H, Licznar-Fajardo P, Hantova S, Godreuil S, Martinez O, et al. Transmission of IMI-2 carbapenemase-producing Enterobacteriaceae from river water to human. J Glob Antimicrob Resist. (2018) 15:88–92. doi: 10.1016/j.jgar.2018.06.022
24. Brouwer MSM, Tehrani KHME, Rapallini M, Geurts Y, Kant A, Harders F, et al. Novel Carbapenemases FLC-1 and IMI-2 Encoded by an Enterobacter cloacae complex isolated from food products. Antimicrob Agents Chemother. (2019) 63:e02338–18. doi: 10.1128/AAC.02338-18
25. Dupont H, Gaillot O, Goetgheluck A-S, Plassart C, Emond J-P, Lecuru M, et al. Molecular characterization of carbapenem-nonsusceptible enterobacterial isolates collected during a prospective interregional survey in France and susceptibility to the novel ceftazidime-avibactam and aztreonam-avibactam combinations. Antimicrob Agents Chemother. (2016) 60:215–21. doi: 10.1128/AAC.01559-15
26. Chu Y-W, Tung VWN, Cheung TKM, Chu M-Y, Cheng N, Lai C, et al. Carbapenemases in Enterobacteria, Hong Kong, China, 2009. Emerg Infect Dis. (2011) 17:130–2. doi: 10.3201/eid1701.101443
27. Boyd DA, Mataseje LF, Davidson R, Delport JA, Fuller J, Hoang L, et al. Enterobacter cloacae complex isolates harboring blaNMC-A or blaIMI-type class A carbapenemase genes on novel chromosomal integrative elements and plasmids. Antimicrob Agents Chemother. (2017) 61:e02578–16. doi: 10.1128/AAC.02578-16
28. Di Luca MC, Skaare D, Aasnaes B, Sundsfjord A, Samuelsen Ø. Identification of a novel IMI carbapenemase variant (IMI-9) in Enterobacter cloacae complex. Int J Antimicrob Agents. (2016) 48:764–5. doi: 10.1016/j.ijantimicag.2016.09.004
29. Nicoletti AG, Marcondes MFM, Martins WMBS, Almeida LGP, Nicolás MF, Vasconcelos ATR, et al. Characterization of BKC-1 class A carbapenemase from Klebsiella pneumoniae clinical isolates in Brazil. Antimicrob Agents Chemother. (2015) 59:5159–64. doi: 10.1128/AAC.00158-15
30. Partridge SR. Mobilization of blaBKC-1 by ISKpn23? Antimicrob Agents Chemother. (2016) 60:5102–4. doi: 10.1128/AAC.00785-16
31. Poirel L, Lartigue M-F, Decousser J-W, Nordmann P. ISEcp1B-mediated transposition of blaCTX-M in Escherichia coli. Antimicrob Agents Chemother. (2005) 49:447–50. doi: 10.1128/AAC.49.1.447-450.2005
32. Martins WMBS, Seco BMS, Sampaio JM, Sands K, Toleman MA, Gales AC. Detection of BKC-1 in Citrobacter freundii: a clue of mobilization in an IncQ1 plasmid carrying blaBKC-1. Int J Antimicrob Agents. (2020) 2020:106042. doi: 10.1016/j.ijantimicag.2020.106042
33. Martins WMBS, Nicoletti AG, Santos SR, Sampaio JLM, Gales AC. Frequency of BKC-1-producing Klebsiella species isolates. Antimicrob Agents Chemother. (2016) 60:5044–6. doi: 10.1128/AAC.00470-16
34. Poirel L, Bonnin RA, Nordmann P. Genetic support and diversity of acquired extended-spectrum β-lactamases in Gram-negative rods. Infect Genet Evol. (2012) 12:883–93. doi: 10.1016/j.meegid.2012.02.008
35. Poirel L, Héritier C, Podglajen I, Sougakoff W, Gutmann L, Nordmann P. Emergence in Klebsiella pneumoniae of a chromosome-encoded SHV β-lactamase that compromises the efficacy of imipenem. Antimicrob Agents Chemother. (2003) 47:755–8. doi: 10.1128/AAC.47.2.755-758.2003
36. Tollentino FM, Polotto M, Nogueira ML, Lincopan N, Neves P, Mamizuka EM, et al. High prevalence of bla(CTX-M) extended spectrum β-lactamase genes in Klebsiella pneumoniae isolates from a tertiary care hospital: first report of bla(SHV-12), bla(SHV-31), bla(SHV-38), and bla(CTX-M-15) in Brazil. Microb Drug Resist. (2011) 17:7–16. doi: 10.1089/mdr.2010.0055
37. Mondal AH, Siddiqui MT, Sultan I, Haq QMR. Prevalence and diversity of blaTEM, blaSHV and blaCTX-M variants among multidrug resistant Klebsiella spp. from an urban riverine environment in India. Int J Environ Health Res. (2019) 29:117–29. doi: 10.1080/09603123.2018.1515425
38. Dziri R, Talmoudi A, Barguellil F, Ouzari H-I, El Asli MS, Klibi N. Huge diversity of TEM and SHV β-lactamases types among CTX-M-15-producing Enterobacteriaceae species in Tunisia. Microb Drug Resist. (2019) 25:1149–54. doi: 10.1089/mdr.2018.0445
39. Bonnet R. Growing group of extended-spectrum β-lactamases: the CTX-M enzymes. Antimicrob Agents Chemother. (2004) 48:1–14. doi: 10.1128/AAC.48.1.1-14.2004
40. Girlich D, Poirel L, Nordmann P. Do CTX-M β-lactamases hydrolyse ertapenem? J Antimicrob Chemother. (2008) 62:1155–6. doi: 10.1093/jac/dkn317
41. Girlich D, Poirel L, Nordmann P. CTX-M expression and selection of ertapenem resistance in Klebsiella pneumoniae and Escherichia coli. Antimicrob Agents Chemother. (2009) 53:832–4. doi: 10.1128/AAC.01007-08
42. Poirel L, Ortiz de la Rosa J-M, Richard A, Aires-de-Sousa M, Nordmann P. CTX-M-33, a CTX-M-15 derivative conferring reduced susceptibility to carbapenems. Antimicrob Agents Chemother. (2019) 63:e01515–19. doi: 10.1128/AAC.01515-19
43. Galani I, Souli M, Chryssouli Z, Giamarellou H. Detection of CTX-M-15 and CTX-M-33, a novel variant of CTX-M-15, in clinical Escherichia coli isolates in Greece. Int J Antimicrob Agents. (2007) 29:598–600. doi: 10.1016/j.ijantimicag.2006.11.010
44. Aires-de-Sousa M, Ortiz de la Rosa JM, Goncalves ML, Costa A, Nordmann P, Poirel L. Occurrence of NDM-1-producing Morganella morganii and Proteus mirabilis in a single patient in Portugal: probable in vivo transfer by conjugation. J Antimicrob Chemother. (2020) 75:903–6. doi: 10.1093/jac/dkz542
45. Poirel L, Le Thomas I, Naas T, Karim A, Nordmann P. Biochemical sequence analyses of GES-1, a novel class A extended-spectrum β-lactamase, and the class 1 integron In52 from Klebsiella pneumoniae. Antimicrob Agents Chemother. (2000) 44:622–32. doi: 10.1128/AAC.44.3.622-632.2000
46. Poirel L, Weldhagen GF, Naas T, De Champs C, Dove MG, Nordmann P. GES-2, a class A β-lactamase from Pseudomonas aeruginosa with increased hydrolysis of imipenem. Antimicrob Agents Chemother. (2001) 45:2598–603. doi: 10.1128/AAC.45.9.2598-2603.2001
47. Kotsakis SD, Miriagou V, Tzelepi E, Tzouvelekis LS. Comparative biochemical and computational study of the role of naturally occurring mutations at ambler positions 104 and 170 in GES β-lactamases. Antimicrob Agents Chemother. (2010) 54:4864–71. doi: 10.1128/AAC.00771-10
48. Poirel L, Brinas L, Fortineau N, Nordmann P. Integron-encoded GES-type extended-spectrum β-lactamase with increased activity toward aztreonam in Pseudomonas aeruginosa. Antimicrob Agents Chemother. (2005) 49:3593–7. doi: 10.1128/AAC.49.8.3593-3597.2005
49. Vourli S, Giakkoupi P, Miriagou V, Tzelepi E, Vatopoulos AC, Tzouvelekis LS. Novel GES/IBC extended-spectrum β-lactamase variants with carbapenemase activity in clinical enterobacteria. FEMS Microbiol Lett. (2004) 234:209–13. doi: 10.1111/j.1574-6968.2004.tb09535.x
50. Wachino J, Doi Y, Yamane K, Shibata N, Yagi T, Kubota T, et al. Nosocomial spread of ceftazidime-resistant Klebsiella pneumoniae strains producing a novel class a β-lactamase, GES-3, in a neonatal intensive care unit in Japan. Antimicrob Agents Chemother. (2004) 48:1960–7. doi: 10.1128/AAC.48.6.1960-1967.2004
51. Ryoo NH, Kim E-C, Hong SG, Park YJ, Lee K, Bae IK, et al. Dissemination of SHV-12 and CTX-M-type extended-spectrum β-lactamases among clinical isolates of Escherichia coli and Klebsiella pneumoniae and emergence of GES-3 in Korea. J Antimicrob Chemother. (2005) 56:698–702. doi: 10.1093/jac/dki324
52. Wachino J, Doi Y, Yamane K, Shibata N, Yagi T, Kubota T, et al. Molecular characterization of a cephamycin-hydrolyzing and inhibitor-resistant class A β-lactamase, GES-4, possessing a Single G170S substitution in the Ω-Loop. Antimicrob Agents Chemother. (2004) 48:2905–10. doi: 10.1128/AAC.48.8.2905-2910.2004
53. Aires-de-Sousa M, Ortiz de la Rosa JM, Gonçalves ML, Pereira AL, Nordmann P, Poirel L. Epidemiology of carbapenemase-producing Klebsiella pneumoniae in a Hospital, Portugal. Emerging Infect Dis. (2019) 25:1632–8. doi: 10.3201/eid2509.190656
54. Bonnin RA, Jousset AB, Urvoy N, Gauthier L, Tlili L, Creton E, et al. Detection of GES-5 Carbapenemase in Klebsiella pneumoniae, a Newcomer in France. Antimicrob Agents Chemother. (2017) 61: doi: 10.1128/AAC.02263-16
55. Moura Q, Cerdeira L, Fernandes MR, Vianello MA, Lincopan N. Novel class 1 integron (In1390) harboring blaGES-5 in a Morganella morganii strain recovered from a remote community. Diagn Microbiol Infect Dis. (2018) 91:345–7. doi: 10.1016/j.diagmicrobio.2018.03.006
56. Girlich D, Poirel L, Szczepanowski R, Schlüter A, Nordmann P. Carbapenem-hydrolyzing GES-5-encoding gene on different plasmid types recovered from a bacterial community in a sewage treatment plant. Appl Environ Microbiol. (2012) 78:1292–5. doi: 10.1128/AEM.06841-11
57. Picão RC, Santos AF, Nicoletti AG, Furtado GH, Gales AC. Detection of GES-5-producing Klebsiella pneumoniae in Brazil. J Antimicrob Chemother. (2010) 65:796–7. doi: 10.1093/jac/dkq024
58. Jeong SH, Bae IK, Kim D, Hong SG, Song JS, Lee JH, et al. First outbreak of Klebsiella pneumoniae clinical isolates producing GES-5 and SHV-12 extended-spectrum β-lactamases in Korea. Antimicrob Agents Chemother. (2005) 49:4809–10. doi: 10.1128/AAC.49.11.4809-4810.2005
59. Cuzon G, Bogaerts P, Bauraing C, Huang T-D, Bonnin RA, Glupczynski Y, et al. Spread of plasmids carrying multiple GES variants. Antimicrob Agents Chemother. (2016) 60:5040–3. doi: 10.1128/AAC.00360-16
60. Gomi R, Matsuda T, Yamamoto M, Chou P-H, Tanaka M, Ichiyama S, et al. Characteristics of carbapenemase-producing enterobacteriaceae in wastewater revealed by genomic analysis. Antimicrob Agents Chemother. (2018) 62:e02501–17. doi: 10.1128/AAC.02501-17
61. Streling AP, Barbosa PP, Marcondes MF, Nicoletti AG, Picão RC, Pinto EC, et al. Genetic and biochemical characterization of GES-16, a new GES-type β-lactamase with carbapenemase activity in Serratia marcescens. Diagn Microbiol Infect Dis. (2018) 92:147–51. doi: 10.1016/j.diagmicrobio.2018.05.003
62. Literacka E, Izdebski R, Urbanowicz P, Zabicka D, Klepacka J, Sowa-Sierant I, Zak I, Garus-Jakubowska A, et al. Spread of Klebsiella pneumoniae ST45 producing GES-5 carbapenemase or GES-1 extended-spectrum β-lactamase in newborns and infants. Antimicrob Agents Chemother. (2020) 64:e00595–20. doi: 10.1128/AAC.00595-20
63. Ellington MJ, Davies F, Jauneikaite E, Hopkins KL, Turton JF, Adams G, et al. A multi-species cluster of GES-5 carbapenemase producing Enterobacterales linked by a geographically disseminated plasmid. Clin Infect Dis. (2019) 71:2553–60. doi: 10.1093/cid/ciz1130
64. Henriques I, Moura A, Alves A, Saavedra MJ, Correia A. Molecular characterization of a carbapenem-hydrolyzing class A β-lactamase, SFC-1, from Serratia fonticola UTAD54. Antimicrob Agents Chemother. (2004) 48:2321–4. doi: 10.1128/AAC.48.6.2321-2324.2004
65. Fonseca F, Sarmento AC, Henriques I, Samyn B, van Beeumen J, Domingues P, et al. Biochemical characterization of SFC-1, a class A carbapenem-hydrolyzing β-lactamase. Antimicrob Agents Chemother. (2007) 51:4512–4. doi: 10.1128/AAC.00491-07
66. Naas T, Vandel L, Sougakoff W, Livermore DM, Nordmann P. Cloning and sequence analysis of the gene for a carbapenem-hydrolyzing class A β-lactamase, Sme-1, from Serratia marcescens S6. Antimicrob Agents Chemother. (1994) 38:1262–70. doi: 10.1128/AAC.38.6.1262
67. Yang YJ, Wu PJ, Livermore DM. Biochemical characterization of a β-lactamase that hydrolyzes penems and carbapenems from two Serratia marcescens isolates. Antimicrob Agents Chemother. (1990) 34:755–8. doi: 10.1128/AAC.34.5.755
68. Queenan AM, Torres-Viera C, Gold HS, Carmeli Y, Eliopoulos GM, Moellering RC, et al. SME-type carbapenem-hydrolyzing class A β-lactamases from geographically diverse Serratia marcescens strains. Antimicrob Agents Chemother. (2000) 44:3035–9. doi: 10.1128/AAC.44.11.3035-3039.2000
69. Gales AC, Biedenbach DJ, Winokur P, Hacek DM, Pfaller MA, Jones RN. Carbapenem-resistant Serratia marcescens isolates producing Bush group 2f β-lactamase (SME-1) in the United States: results from the MYSTIC Programme. Diagn Microbiol Infect Dis. (2001) 39:125–7. doi: 10.1016/S0732-8893(00)00222-4
70. Hemarajata P, Amick T, Yang S, Gregson A, Holzmeyer C, Bush K, et al. Selection of hyperproduction of AmpC and SME-1 in a carbapenem-resistant Serratia marcescens isolate during antibiotic therapy. J Antimicrob Chemother. (2018) 73:1256–62. doi: 10.1093/jac/dky028
71. Fairfax MR, Queenan AM, Lephart PR, Kaye KS, Dror M, Arnous N, et al. Detection of 2 SME-1 carbapenemase-producing Serratia marcescens in Detroit. Diagn Microbiol Infect Dis. (2011) 71:325–6. doi: 10.1016/j.diagmicrobio.2011.06.007
72. Carrër A, Poirel L, Pitout JD, Church D, Nordmann P. Occurrence of an SME-2-producing Serratia marcescens isolate in Canada. Int J Antimicrob Agents. (2008) 31:181–2. doi: 10.1016/j.ijantimicag.2007.10.007
73. Poirel L, Wenger A, Bille J, Bernabeu S, Naas T, Nordmann P. SME-2-producing Serratia marcescens isolate from Switzerland. Antimicrob Agents Chemother. (2007) 51:2282–3. doi: 10.1128/AAC.00309-07
74. Queenan AM, Shang W, Schreckenberger P, Lolans K, Bush K, Quinn J. SME-3, a novel member of the Serratia marcescens SME family of carbapenem-hydrolyzing β-lactamases. Antimicrob Agents Chemother. (2006) 50:3485–7. doi: 10.1128/AAC.00363-06
75. Cayô R, Leme RCP, Streling AP, Matos AP, Nodari CS, Chaves JRE, et al. Serratia marcescens harboring SME-4 in Brazil: a silent threat. Diagn Microbiol Infect Dis. (2017) 87:357–8. doi: 10.1016/j.diagmicrobio.2017.01.008
76. Dabos L, Patiño-Navarrete R, Nastro M, Famiglietti A, Glaser P, Rodriguez CH, et al. SME-4-producing Serratia marcescens from Argentina belonging to clade 2 of the S. marcescens phylogeny. J Antimicrob Chemother. (2019) 74:1836–41. doi: 10.1093/jac/dkz115
77. Castanheira M, Doyle TB, Kantro V, Mendes RE, Shortridge D. Meropenem-vaborbactam activity against carbapenem-resistant enterobacterales isolates collected in U.S. Hospitals during 2016 to 2018. Antimicrob Agents Chemother. (2020) 64:e01951–19. doi: 10.1128/AAC.00305-20
78. Naas T, Livermore DM, Nordmann P. Characterization of an LysR family protein, SmeR from Serratia marcescens S6, its effect on expression of the carbapenem-hydrolyzing β-lactamase Sme-1, and comparison of this regulator with other β-lactamase regulators. Antimicrob Agents Chemother. (1995) 39:629–37. doi: 10.1128/AAC.39.3.629
79. Mataseje LF, Boyd DA, Delport J, Hoang L, Imperial M, Lefebvre B, et al. Serratia marcescens harbouring SME-type class A carbapenemases in Canada and the presence of blaSME on a novel genomic island, SmarGI1-1. J Antimicrob Chemother. (2014) 69:1825–9. doi: 10.1093/jac/dku040
80. Dortet L, Poirel L, Abbas S, Oueslati S, Nordmann P. Genetic and biochemical characterization of FRI-1, a carbapenem-hydrolyzing class A β-lactamase from Enterobacter cloacae. Antimicrob Agents Chemother. (2015) 59:7420–5. doi: 10.1128/AAC.01636-15
81. Meunier D, Findlay J, Doumith M, Godoy D, Perry C, Pike R, et al. FRI-2 carbapenemase-producing Enterobacter cloacae complex in the UK. J Antimicrob Chemother. (2017) 72:2478–82. doi: 10.1093/jac/dkx173
82. Schauer J, Gatermann SG, Marschal M, Pfennigwerth N. Genetic and biochemical characterization of FRI-3, a novel variant of the Ambler class A carbapenemase FRI-1. J Antimicrob Chemother. (2019) 74:2891–4. doi: 10.1093/jac/dkz295
83. Kubota H, Uwamino Y, Matsui M, Sekizuka T, Suzuki Y, Okuno R, et al. FRI-4 carbapenemase-producing Enterobacter cloacae complex isolated in Tokyo, Japan. J Antimicrob Chemother. (2018) 73:2969–72. doi: 10.1093/jac/dky291
84. Boyd DA, Lefebvre B, Mataseje LF, Gagnon S, Roger M, Savard P, et al. Enterobacter sp. N18-03635 harbouring blaFRI-6 class A carbapenemase, Canada. J Antimicrob Chemother. (2020) 75:486–8. doi: 10.1093/jac/dkz438
85. Castanheira M, Toleman MA, Jones RN, Schmidt FJ, Walsh TR. Molecular characterization of a β-Lactamase Gene, blaGIM-1, encoding a new subclass of metallo-β-Lactamase. Antimicrob Agents Chemother. (2004) 48:4654–61. doi: 10.1128/AAC.48.12.4654-4661.2004
86. Borra PS, Samuelsen Ø, Spencer J, Walsh TR, Lorentzen MS, Leiros H-KS. Crystal structures of Pseudomonas aeruginosa GIM-1: active-site plasticity in Metallo-β-Lactamases. Antimicrob Agents Chemother. (2013) 57:848–54. doi: 10.1128/AAC.02227-12
87. Rieber H, Frontzek A, Pfeifer Y. Emergence of Metallo-β-Lactamase GIM-1 in a clinical isolate of Serratia marcescens. Antimicrob Agents Chemother. (2012) 56:4945–7. doi: 10.1128/AAC.00405-12
88. Hamprecht A, Poirel L, Göttig S, Seifert H, Kaase M, Nordmann P. Detection of the carbapenemase GIM-1 in Enterobacter cloacae in Germany. J Antimicrob Chemother. (2013) 68:558–61. doi: 10.1093/jac/dks447
89. Wendel AF, Brodner AHB, Wydra S, Ressina S, Henrich B, Pfeffer K, et al. Genetic characterization and emergence of the metallo-β-lactamase GIM-1 in Pseudomonas spp. and Enterobacteriaceae during a long-term outbreak. Antimicrob Agents Chemother. (2013) 57:5162–5. doi: 10.1128/AAC.00118-13
90. Wendel AF, Kaase M, Autenrieth IB, Peter S, Oberhettinger P, Rieber H, et al. Protracted regional dissemination of GIM-1-producing Serratia marcescens in Western Germany. Antimicrob Agents Chemother. (2017) 61. doi: 10.1128/AAC.01880-16
91. Wendel AF, Meyer S, Deenen R, Köhrer K, Kolbe-Busch S, Pfeffer K, et al. Long-term, low-frequency cluster of a German-imipenemase-1-producing Enterobacter hormaechei ssp. steigerwaltii ST89 in a Tertiary Care Hospital in Germany. Microb Drug Resist. (2018) 24:1305–15. doi: 10.1089/mdr.2017.0433
92. Wendel AF, Ressina S, Kolbe-Busch S, Pfeffer K, MacKenzie CR. Species diversity of environmental GIM-1-producing bacteria collected during a long-term outbreak. Appl Environ Microbiol. (2016) 82:3605–10. doi: 10.1128/AEM.00424-16
93. Wendel AF, MacKenzie CR. Characterization of a novel metallo-β-lactamase variant, GIM-2, from a clinical isolate of Enterobacter cloacae in Germany. Antimicrob Agents Chemother. (2015) 59:1824–5. doi: 10.1128/AAC.05062-14
94. Sekiguchi J, Morita K, Kitao T, Watanabe N, Okazaki M, Miyoshi-Akiyama T, et al. KHM-1, a novel plasmid-mediated metallo-β-lactamase from a Citrobacter freundii clinical isolate. Antimicrob Agents Chemother. (2008) 52:4194–7. doi: 10.1128/AAC.01337-07
95. Umeda K, Nakamura H, Fukuda A, Yamaguchi T, Matsumoto Y, Motooka D, et al. Molecular characterization of blaKHM-1 encoding plasmid in an Enterobacter hormaechei subsp. hoffmannii isolate from blood culture. PLoS ONE. (2020) 15:e0227605. doi: 10.1371/journal.pone.0227605
96. Suzuki Y, Ida M, Kubota H, Ariyoshi T, Murakami K, Kobayashi M, et al. Multiple β-lactam resistance gene-carrying plasmid harbored by Klebsiella quasipneumoniae isolated from Urban Sewage in Japan. mSphere. (2019) 4:e00391–19. doi: 10.1128/mSphere.00391-19
97. El Salabi A, Borra PS, Toleman MA, Samuelsen Ø, Walsh TR. Genetic and biochemical characterization of a novel metallo-β-lactamase, TMB-1, from an Achromobacter xylosoxidans strain isolated in Tripoli, Libya. Antimicrob Agents Chemother. (2012) 56:2241–5. doi: 10.1128/AAC.05640-11
98. Kayama S, Shigemoto N, Shimizu W, Kuwahara R, Ikeda M, Ikebe K, et al. Tripoli metallo-β-lactamase-1 (TMB-1)-producing Acinetobacter spp. with decreased resistance to imipenem in Japan. Antimicrob Agents Chemother. (2014) 58:2477–8. doi: 10.1128/AAC.01790-13
99. Suzuki S, Matsui M, Suzuki M, Sugita A, Kosuge Y, Kodama N, et al. Detection of tripoli metallo-β-lactamase 2 (TMB-2), a variant of blaTMB-1, in clinical isolates of Acinetobacter spp. in Japan. J Antimicrob Chemother. (2013) 68:1441–2. doi: 10.1093/jac/dkt031
100. Gauthier L, Dortet L, Jousset AB, Mihaila L, Golse N, Naas T, et al. Molecular characterization of plasmid-encoded Tripoli MBL 1 (TMB-1) in Enterobacteriaceae. J Antimicrob Chemother. (2019) 74:42–7. doi: 10.1093/jac/dky372
101. Saavedra MJ, Peixe L, Sousa JC, Henriques I, Alves A, Correia A. Sfh-I, a subclass B2 metallo-β-lactamase from a Serratia fonticola environmental isolate. Antimicrob Agents Chemother. (2003) 47:2330–3. doi: 10.1128/AAC.47.7.2330-2333.2003
102. Fonseca F, Arthur CJ, Bromley EHC, Samyn B, Moerman P, Saavedra MJ, et al. Biochemical characterization of Sfh-I, a subclass B2 metallo-β-lactamase from Serratia fonticola UTAD54. Antimicrob Agents Chemother. (2011) 55:5392–5. doi: 10.1128/AAC.00429-11
103. Yong D, Toleman MA, Bell J, Ritchie B, Pratt R, Ryley H, et al. Genetic and biochemical characterization of an acquired subgroup B3 metallo-β-lactamase gene, blaAIM-1, and its unique genetic context in Pseudomonas aeruginosa from Australia. Antimicrob Agents Chemother. (2012) 56:6154–9. doi: 10.1128/AAC.05654-11
104. Zhou H, Guo W, Zhang J, Li Y, Zheng P, Zhang H. Draft genome sequence of a metallo-β-lactamase (blaAIM-1)-producing Klebsiella pneumoniae ST1916 isolated from a patient with chronic diarrhoea. J Glob Antimicrob Resist. (2019) 16:165–7. doi: 10.1016/j.jgar.2019.01.010
105. Bougnom BP, Zongo C, McNally A, Ricci V, Etoa FX, Thiele-Bruhn S, et al. Wastewater used for urban agriculture in West Africa as a reservoir for antibacterial resistance dissemination. Environ Res. (2019) 168:14–24. doi: 10.1016/j.envres.2018.09.022
106. Leiros H-KS, Borra PS, Brandsdal BO, Edvardsen KSW, Spencer J, Walsh TR, et al. Crystal structure of the mobile metallo-β-lactamase AIM-1 from Pseudomonas aeruginosa: insights into antibiotic binding and the role of Gln157. Antimicrob Agents Chemother. (2012) 56:4341–53. doi: 10.1128/AAC.00448-12
107. Lange F, Pfennigwerth N, Hartl R, Kerschner H, Achleitner D, Gatermann SG, et al. LMB-1, a novel family of class B3 MBLs from an isolate of Enterobacter cloacae. J Antimicrob Chemother. (2018) 73:2331–5. doi: 10.1093/jac/dky215
108. Dabos L, Rodriguez CH, Nastro M, Dortet L, Bonnin RA, Famiglietti A, et al. LMB-1 producing Citrobacter freundii from Argentina, a novel player in the field of MBLs. Int J Antimicrob Agents. (2020) 55:105857. doi: 10.1016/j.ijantimicag.2019.11.014
109. Kim JY, Jung HI, An YJ, Lee JH, Kim SJ, Jeong SH, et al. Structural basis for the extended substrate spectrum of CMY-10, a plasmid-encoded class C β-lactamase. Mol Microbiol. (2006) 60:907–16. doi: 10.1111/j.1365-2958.2006.05146.x
110. Jousset AB, Oueslati S, Bernabeu S, Takissian J, Creton E, Vogel A, et al. False-positive carbapenem-hydrolyzing confirmatory tests due to ACT-28, a chromosomally encoded AmpC with weak carbapenemase activity from Enterobacter kobei. Antimicrob Agents Chemother. (2019) 63:e02388–18. doi: 10.1128/AAC.02388-18
111. Lee SH, Jeong SH, Park Y-M. Characterization of blaCMY-10 a novel, plasmid-encoded AmpC-type β-lactamase gene in a clinical isolate of Enterobacter aerogenes. J Appl Microbiol. (2003) 95:744–52. doi: 10.1046/j.1365-2672.2003.02040.x
112. Song JS, Jang SJ, Lee JJ, Lee JH, Bae IK, Jeong BC, et al. Association of the bla(CMY-10) gene with a novel complex class 1 integron carrying an ISCR1 element in clinical isolates from Korea. Clin Microbiol Infect. (2010) 16:1013–7. doi: 10.1111/j.1469-0691.2009.03002.x
113. Lee JH, Jung HI, Jung JH, Park JS, Ahn JB, Jeong SH, et al. Dissemination of transferable AmpC-type β-lactamase (CMY-10) in a Korean hospital. Microb Drug Resist. (2004) 10:224–30. doi: 10.1089/mdr.2004.10.224
114. Livermore DM, Mushtaq S, Barker K, Hope R, Warner M, Woodford N. Characterization of β-lactamase and porin mutants of Enterobacteriaceae selected with ceftaroline + avibactam (NXL104). J Antimicrob Chemother. (2012) 67:1354–8. doi: 10.1093/jac/dks079
115. Na J-H, An YJ, Cha S-S. GMP and IMP Are competitive inhibitors of CMY-10, an extended-spectrum class C β-lactamase. Antimicrob Agents Chemother. (2017) 61:e00098–17. doi: 10.1128/AAC.00098-17
116. Bradford PA, Urban C, Mariano N, Projan SJ, Rahal JJ, Bush K. Imipenem resistance in Klebsiella pneumoniae is associated with the combination of ACT-1, a plasmid-mediated AmpC β-lactamase, and the foss of an outer membrane protein. Antimicrob Agents Chemother. (1997) 41:563–9. doi: 10.1128/AAC.41.3.563
117. Rottman M, Benzerara Y, Hanau-Berçot B, Bizet C, Philippon A, Arlet G. Chromosomal ampC genes in Enterobacter species other than Enterobacter cloacae, and ancestral association of the ACT-1 plasmid-encoded cephalosporinase to Enterobacter asburiae. FEMS Microbiol Lett. (2002) 210:87–92. doi: 10.1111/j.1574-6968.2002.tb11164.x
118. Bonnin RA, Nordmann P, Poirel L. Screening and deciphering antibiotic resistance in Acinetobacter baumannii: a state of the art. Expert Rev Anti Infect Ther. (2013) 11:571–83. doi: 10.1586/eri.13.38
119. Towner KJ, Evans B, Villa L, Levi K, Hamouda A, Amyes SGB, et al. Distribution of intrinsic plasmid replicase genes and their association with carbapenem-hydrolyzing class D β-lactamase genes in European clinical isolates of Acinetobacter baumannii. Antimicrob Agents Chemother. (2011) 55:2154–9. doi: 10.1128/AAC.01661-10
120. Wang X, Zong Z, Lü X. Tn2008 is a major vehicle carrying bla(OXA-23) in Acinetobacter baumannii from China. Diagn Microbiol Infect Dis. (2011) 69:218–22. doi: 10.1016/j.diagmicrobio.2010.10.018
121. Corvec S, Poirel L, Naas T, Drugeon H, Nordmann P. Genetics and expression of the carbapenem-hydrolyzing oxacillinase gene blaOXA-23 in Acinetobacter baumannii. Antimicrob Agents Chemother. (2007) 51:1530–3. doi: 10.1128/AAC.01132-06
122. Bonnet R, Marchandin H, Chanal C, Sirot D, Labia R, De Champs C, et al. Chromosome-encoded class D β-lactamase OXA-23 in Proteus mirabilis. Antimicrob Agents Chemother. (2002) 46:2004–6. doi: 10.1128/AAC.46.6.2004-2006.2002
123. Österblad M, Karah N, Halkilahti J, Sarkkinen H, Uhlin BE, Jalava J. Rare detection of the acinetobacter class D carbapenemase blaOXA-23 gene in Proteus mirabilis. Antimicrob Agents Chemother. (2016) 60:3243–5. doi: 10.1128/AAC.03119-15
124. Potron A, Hocquet D, Triponney P, Plésiat P, Bertrand X, Valot B. Carbapenem-susceptible OXA-23-producing Proteus mirabilis in the French community. Antimicrob Agents Chemother. (2019) 63:e00191-19. doi: 10.1128/AAC.00191-19
125. Octavia S, Xu W, Ng OT, Marimuthu K, Venkatachalam I, Cheng B, et al. Identification of AbaR4 Acinetobacter baumannii resistance island in clinical isolates of blaOXA-23-positive Proteus mirabilis. J Antimicrob Chemother. (2020) 75:521–5. doi: 10.1093/jac/dkz472
126. Bonnin RA, Girlich D, Jousset AB, Gauthier L, Cuzon G, Bogaerts P, et al. A single Proteus mirabilis lineage from human and animal sources: a hidden reservoir of OXA-23 or OXA-58 carbapenemases in Enterobacterales. Sci Rep. (2020) 10:9160. doi: 10.1038/s41598-020-66161-z
127. Osterblad M, Hakanen AJ, Jalava J. Evaluation of the Carba NP test for carbapenemase detection. Antimicrob Agents Chemother. (2014) 58:7553–6. doi: 10.1128/AAC.02761-13
128. Nigro SJ, Hall RM. Structure and context of Acinetobacter transposons carrying the oxa23 carbapenemase gene. J Antimicrob Chemother. (2016) 71:1135–47. doi: 10.1093/jac/dkv440
129. Girlich D, Bonnin RA, Dortet L, Naas T. Genetics of acquired antibiotic resistance genes in Proteus spp. Front Microbiol. (2020) 11:256. doi: 10.3389/fmicb.2020.00256
130. Peleg AY, Seifert H, Paterson DL. Acinetobacter baumannii: emergence of a successful pathogen. Clin Microbiol Rev. (2008) 21:538–82. doi: 10.1128/CMR.00058-07
131. Leulmi Z, Kandouli C, Mihoubi I, Benlabed K, Lezzar A, Rolain J-M. First report of blaOXA-24 carbapenemase gene, armA methyltransferase and aac(6')-Ib-cr among multidrug-resistant clinical isolates of Proteus mirabilis in Algeria. J Glob Antimicrob Resist. (2019) 16:125–9. doi: 10.1016/j.jgar.2018.08.019
132. Poirel L, Marqué S, Héritier C, Segonds C, Chabanon G, Nordmann P. OXA-58, a novel class D β-lactamase involved in resistance to carbapenems in Acinetobacter baumannii. Antimicrob Agents Chemother. (2005) 49:202–8. doi: 10.1128/AAC.49.1.202-208.2005
133. Leski TA, Bangura U, Jimmy DH, Ansumana R, Lizewski SE, Li RW, et al. Identification of blaOXA-51-like, blaOXA-58, blaDIM-1, and blaVIM carbapenemase genes in hospital Enterobacteriaceae isolates from Sierra Leone. J Clin Microbiol. (2013) 51:2435–8. doi: 10.1128/JCM.00832-13
134. Girlich D, Bonnin RA, Bogaerts P, De Laveleye M, Huang DT, Dortet L, et al. Chromosomal amplification of the blaOXA-58 carbapenemase gene in a Proteus mirabilis clinical isolate. Antimicrob Agents Chemother. (2016) 61:e01697–16. doi: 10.1128/AAC.01697-16
135. Literacka E, Izdebski R, Baraniak A, Zabicka D, Schneider A, Urbanowicz P, Herda M, et al. Proteus mirabilis producing the OXA-58 carbapenemase in Poland. Antimicrob Agents Chemother. (2019) 63. doi: 10.1128/AAC.00106-19
136. Lange F, Pfennigwerth N, Gerigk S, Gohlke F, Oberdorfer K, Purr I, et al. Dissemination of blaOXA-58 in Proteus mirabilis isolates from Germany. J Antimicrob Chemother. (2017) 72:1334–9. doi: 10.1093/jac/dkw566
137. Poirel L, Nordmann P. Genetic structures at the origin of acquisition and expression of the carbapenem-hydrolyzing oxacillinase gene blaOXA-58 in Acinetobacter baumannii. Antimicrob Agents Chemother. (2006) 50:1442–8. doi: 10.1128/AAC.50.4.1442-1448.2006
138. El Garch F, Bogaerts P, Bebrone C, Galleni M, Glupczynski Y. OXA-198, an acquired carbapenem-hydrolyzing class D β-lactamase from Pseudomonas aeruginosa. Antimicrob Agents Chemother. (2011) 55:4828–33. doi: 10.1128/AAC.00522-11
139. Bonnin RA, Jousset AB, Gauthier L, Emeraud C, Girlich D, Sauvadet A, et al. First occurrence of the OXA-198 carbapenemase in Enterobacterales. Antimicrob Agents Chemother. (2020) doi: 10.1128/AAC.01471-19
140. Bonnin RA, Bogaerts P, Girlich D, Huang T-D, Dortet L, Glupczynski Y, et al. Molecular characterization of OXA-198 carbapenemase-producing Pseudomonas aeruginosa clinical isolates. Antimicrob Agents Chemother. (2018) 62:e02496-17. doi: 10.1128/AAC.02496-17
141. Antonelli A, D'Andrea MM, Vaggelli G, Docquier J-D, Rossolini GM. OXA-372, a novel carbapenem-hydrolysing class D β-lactamase from a Citrobacter freundii isolated from a hospital wastewater plant. J Antimicrob Chemother. (2015) 70:2749–56. doi: 10.1093/jac/dkv181
142. Bogaerts P, Naas T, Saegeman V, Bonnin RA, Schuermans A, Evrard S, et al. OXA-427, a new plasmid-borne carbapenem-hydrolysing class D β-lactamase in Enterobacteriaceae. J Antimicrob Chemother. (2017) 72:2469–77. doi: 10.1093/jac/dkx184
143. Desmet S, Nepal S, van Dijl JM, Van Ranst M, Chlebowicz MA, Rossen JW, et al. Antibiotic resistance plasmids cointegrated into a megaplasmid harboring the blaOXA-427 carbapenemase gene. Antimicrob Agents Chemother. (2018) 62:e01448-17. doi: 10.1128/AAC.01448-17
144. Frère J-M, Bogaerts P, Huang T-D, Stefanic P, Moray J, Bouillenne F, et al. Interactions between avibactam and ceftazidime-hydrolyzing class D β-lactamases. Biomolecules. (2020) 10:483. doi: 10.3390/biom10030483
145. French CE, Coope C, Conway L, Higgins JPT, McCulloch J, Okoli G, et al. Control of carbapenemase-producing Enterobacteriaceae outbreaks in acute settings: an evidence review. J Hosp Infect. (2017) 95:3–45. doi: 10.1016/j.jhin.2016.10.006
146. Bassetti M, Righi E, Carnelutti A, Graziano E, Russo A. Multidrug-resistant Klebsiella pneumoniae: challenges for treatment, prevention and infection control. Expert Rev Anti Infect Ther. (2018) 16:749–61. doi: 10.1080/14787210.2018.1522249
147. Hilliquin D, Lomont A, Zahar J-R. Cohorting for preventing the nosocomial spread of carbapenemase-producing Enterobacterales, in non-epidemic settings: is it mandatory? J Hosp Infect. (2020) 105:534–45. doi: 10.1016/j.jhin.2020.04.022
148. Bogaerts P, Oueslati S, Meunier D, Nonhoff C, Yunus S, Massart M, et al. Multicentre evaluation of the BYG Carba v2.0 test, a simplified electrochemical assay for the rapid laboratory detection of carbapenemase-producing Enterobacteriaceae. Sci Rep. (2017) 7:9937. doi: 10.1038/s41598-017-09820-y
149. Nordmann P, Poirel L, Dortet L. Rapid detection of carbapenemase-producing Enterobacteriaceae. Emerging Infect Dis. (2012) 18:1503–7. doi: 10.3201/eid1809.120355
150. McMullen AR, Wallace MA, LaBombardi V, Hindler J, Campeau S, Humphries R, et al. Multicenter evaluation of the RAPIDEC® CARBA NP assay for the detection of carbapenemase production in clinical isolates of Enterobacterales and Pseudomonas aeruginosa. Eur J Clin Microbiol Infect Dis. (2020) 39:2037–44. doi: 10.1007/s10096-020-03937-1
151. Bernabeu S, Dortet L, Naas T. Evaluation of the β-CARBATM test, a colorimetric test for the rapid detection of carbapenemase activity in Gram-negative bacilli. J Antimicrob Chemother. (2017) 72:1646–58. doi: 10.1093/jac/dkx061
152. Compain F, Gallah S, Eckert C, Arlet G, Ramahefasolo A, Decré D, et al. Assessment of carbapenem resistance in enterobacteriaceae with the rapid and easy-to-use chromogenic β carba test. J Clin Microbiol. (2016) 54:3065–8. doi: 10.1128/JCM.01912-16
153. Mancini S, Kieffer N, Poirel L, Nordmann P. Evaluation of the RAPIDEC® CARBA NP and β-CARBA® tests for rapid detection of carbapenemase-producing Enterobacteriaceae. Diagn Microbiol Infect Dis. (2017) 88:293–7. doi: 10.1016/j.diagmicrobio.2017.05.006
154. Hrabák J, Walková R, Studentová V, Chudácková E, Bergerová T. Carbapenemase activity detection by matrix-assisted laser desorption ionization-time of flight mass spectrometry. J Clin Microbiol. (2011) 49:3222–7. doi: 10.1128/JCM.00984-11
155. Dortet L, Tandé D, de Briel D, Bernabeu S, Lasserre C, Gregorowicz G, et al. MALDI-TOF for the rapid detection of carbapenemase-producing Enterobacteriaceae: comparison of the commercialized MBT STAR®-Carba IVD Kit with two in-house MALDI-TOF techniques and the RAPIDEC® CARBA NP. J Antimicrob Chemother. (2018) 73:2352–9. doi: 10.1093/jac/dky209
156. Carvalhaes CG, Picão RC, Nicoletti AG, Xavier DE, Gales AC. Cloverleaf test (modified Hodge test) for detecting carbapenemase production in Klebsiella pneumoniae: be aware of false positive results. J Antimicrob Chemother. (2010) 65:249–51. doi: 10.1093/jac/dkp431
157. Girlich D, Poirel L, Nordmann P. Value of the modified hodge test for detection of emerging carbapenemases in Enterobacteriaceae. J Clin Microbiol. (2012) 50:477–9. doi: 10.1128/JCM.05247-11
158. Pasteran F, Gonzalez LJ, Albornoz E, Bahr G, Vila AJ, Corso A. Triton Hodge Test: improved protocol for Modified Hodge Test for enhanced detection of NDM and other carbapenemase producers. J Clin Microbiol. (2016) 54:640–9. doi: 10.1128/JCM.01298-15
159. van der Zwaluw K, de Haan A, Pluister GN, Bootsma HJ, de Neeling AJ, Schouls LM. The carbapenem inactivation method (CIM), a simple and low-cost alternative for the Carba NP test to assess phenotypic carbapenemase activity in gram-negative rods. PLoS ONE. (2015) 10:e0123690. doi: 10.1371/journal.pone.0123690
160. Gauthier L, Bonnin RA, Dortet L, Naas T. Retrospective and prospective evaluation of the Carbapenem inactivation method for the detection of carbapenemase-producing Enterobacteriaceae. PLoS ONE. (2017) 12:e0170769. doi: 10.1371/journal.pone.0170769
161. Pierce VM, Simner PJ, Lonsway DR, Roe-Carpenter DE, Johnson JK, Brasso WB, et al. Modified carbapenem inactivation method for phenotypic detection of carbapenemase production among Enterobacteriaceae. J Clin Microbiol. (2017) 55:2321–33. doi: 10.1128/JCM.00193-17
162. Muntean M-M, Muntean A-A, Gauthier L, Creton E, Cotellon G, Popa MI, et al. Evaluation of the rapid carbapenem inactivation method (rCIM): a phenotypic screening test for carbapenemase-producing Enterobacteriaceae. J Antimicrob Chemother. (2018) 73:900–8. doi: 10.1093/jac/dkx519
163. Saito R, Koyano S, Dorin M, Higurashi Y, Misawa Y, Nagano N, et al. Evaluation of a simple phenotypic method for the detection of carbapenemase-producing Enterobacteriaceae. J Microbiol Methods. (2015) 108:45–8. doi: 10.1016/j.mimet.2014.11.008
164. Dortet L, Bernabeu S, Gonzalez C, Naas T. Evaluation of the Carbapenem Detection SetTM for the detection and characterization of carbapenemase-producing Enterobacteriaceae. Diagn Microbiol Infect Dis. (2018) 91:220–5. doi: 10.1016/j.diagmicrobio.2018.02.012
165. Rösner S, Kamalanabhaiah S, Küsters U, Kolbert M, Pfennigwerth N, Mack D. Evaluation of a novel immunochromatographic lateral flow assay for rapid detection of OXA-48, NDM, KPC and VIM carbapenemases in multidrug-resistant Enterobacteriaceae. J Med Microbiol. (2019) 68:379–81. doi: 10.1099/jmm.0.000925
166. Boutal H, Vogel A, Bernabeu S, Devilliers K, Creton E, Cotellon G, et al. A multiplex lateral flow immunoassay for the rapid identification of NDM-, KPC-, IMP- and VIM-type and OXA-48-like carbapenemase-producing Enterobacteriaceae. J Antimicrob Chemother. (2018) 73:909–15. doi: 10.1093/jac/dkx521
167. Volland H, Girlich D, Laguide M, Gonzalez C, Paris V, Laroche M, et al. Improvement of the immunochromatographic NG-Test Carba 5 assay for the detection of IMP variants previously undetected. Antimicrob Agents Chemother. (2019) 64: doi: 10.1128/AAC.01940-19
168. Cointe A, Bonacorsi S, Truong J, Hobson C, Doit C, Monjault A, et al. Detection of carbapenemase-producing enterobacteriaceae in positive blood culture using an immunochromatographic RESIST-4 O.K.N.V. Assay. Antimicrob Agents Chemother. (2018) 62:e01828–18. doi: 10.1128/AAC.01828-18
169. Takissian J, Bonnin RA, Naas T, Dortet L. NG-Test Carba 5 for rapid detection of carbapenemase-producing enterobacterales from positive blood cultures. Antimicrob Agents Chemother. (2019) 63:e00011-19. doi: 10.1128/AAC.00011-19
170. Riccobono E, Bogaerts P, Antonelli A, Evrard S, Giani T, Rossolini GM, et al. Evaluation of the OXA-23 K-SeT® immunochromatographic assay for the rapid detection of OXA-23-like carbapenemase-producing Acinetobacter spp. J Antimicrob Chemother. (2019) 74:1455–7. doi: 10.1093/jac/dkz001
171. Tenover FC, Canton R, Kop J, Chan R, Ryan J, Weir F, et al. Detection of colonization by carbapenemase-producing Gram-negative Bacilli in patients by use of the Xpert MDRO assay. J Clin Microbiol. (2013) 51:3780–7. doi: 10.1128/JCM.01092-13
172. Cointe A, Walewski V, Hobson CA, Doit C, Bidet P, Dortet L, et al. Rapid carbapenemase detection with Xpert Carba-R V2 directly on positive blood vials. Infect Drug Resist. (2019) 12:3311–6. doi: 10.2147/IDR.S204436
173. Lucena Baeza L, Hamprecht A. A profile of the GenePOC Carba C assay for the detection and differentiation of gene sequences associated with carbapenem-non-susceptibility. Expert Rev Mol Diagn. (2020) 1–13. doi: 10.1080/14737159.2020.1785287
174. Girlich D, Bernabeu S, Grosperrin V, Langlois I, Begasse C, Arangia N, et al. Evaluation of the Amplidiag CarbaR+MCR Kit for accurate detection of carbapenemase-producing and colistin-resistant bacteria. J Clin Microbiol. (2019) 57:e01800-18. doi: 10.1128/JCM.01800-18
175. Ceyssens P-J, Garcia-Graells C, Fux F, Botteldoorn N, Mattheus W, Wuyts V, et al. Development of a Luminex xTAG® assay for cost-effective multiplex detection of β-lactamases in Gram-negative bacteria. J Antimicrob Chemother. (2016) 71:2479–83. doi: 10.1093/jac/dkw201
176. Bogaerts P, Cuzon G, Evrard S, Hoebeke M, Naas T, Glupczynski Y. Evaluation of a DNA microarray for rapid detection of the most prevalent extended-spectrum β-lactamases, plasmid-mediated cephalosporinases and carbapenemases in Enterobacteriaceae, Pseudomonas and Acinetobacter. Int J Antimicrob Agents. (2016) 48:189–93. doi: 10.1016/j.ijantimicag.2016.05.006
177. Girlich D, Bernabeu S, Fortineau N, Dortet L, Naas T. Evaluation of the CRE and ESBL ELITe MGB® kits for the accurate detection of carbapenemase- or CTX-M-producing bacteria. Diagn Microbiol Infect Dis. (2018) 92:1–7. doi: 10.1016/j.diagmicrobio.2018.02.001
178. Angers-Loustau A, Petrillo M, Bengtsson-Palme J, Berendonk T, Blais B, Chan K-G, et al. The challenges of designing a benchmark strategy for bioinformatics pipelines in the identification of antimicrobial resistance determinants using next generation sequencing technologies. F1000Res. (2018) 7:ISCB Comm J-459. doi: 10.12688/f1000research.14509.1
179. Zankari E, Hasman H, Cosentino S, Vestergaard M, Rasmussen S, Lund O, et al. Identification of acquired antimicrobial resistance genes. J Antimicrob Chemother. (2012) 67:2640–4. doi: 10.1093/jac/dks261
180. Alcock BP, Raphenya AR, Lau TTY, Tsang KK, Bouchard M, Edalatmand A, et al. CARD 2020: antibiotic resistome surveillance with the comprehensive antibiotic resistance database. Nucleic Acids Res. (2020) 48:D517–25. doi: 10.1093/nar/gkz935
181. Drawz SM, Bonomo RA. Three decades of β-lactamase inhibitors. Clin Microbiol Rev. (2010) 23:160–201. doi: 10.1128/CMR.00037-09
182. Karaiskos I, Galani I, Souli M, Giamarellou H. Novel β-lactam-β-lactamase inhibitor combinations: expectations for the treatment of carbapenem-resistant Gram-negative pathogens. Expert Opin Drug Metab Toxicol. (2019) 15:133–49. doi: 10.1080/17425255.2019.1563071
183. Yahav D, Giske CG, Grāmatniece A, Abodakpi H, Tam VH, Leibovici L. New β-Lactam-β-lactamase inhibitor combinations. Clin Microbiol Rev. (2020) 34:e00115-20. doi: 10.1128/CMR.00115-20
184. Sherry NL, Baines SL, Howden BP. Ceftazidime/avibactam susceptibility by three different susceptibility testing methods in carbapenemase-producing Gram-negative bacteria from Australia. Int J Antimicrob Agents. (2018) 52:82–5. doi: 10.1016/j.ijantimicag.2018.02.017
185. Livermore DM, Mushtaq S, Warner M, Vickers A, Woodford N. In vitro activity of cefepime/zidebactam (WCK 5222) against Gram-negative bacteria. J Antimicrob Chemother. (2017) 72:1373–85. doi: 10.1093/jac/dkw593
186. Papp-Wallace KM, Bethel CR, Caillon J, Barnes MD, Potel G, Bajaksouzian S, et al. Beyond piperacillin-tazobactam: cefepime and AAI101 as a potent β-Lactam-β-lactamase inhibitor combination. Antimicrob Agents Chemother. (2019) 63:e00105-19. doi: 10.1128/AAC.00105-19
187. Liu B, Trout REL, Chu G-H, McGarry D, Jackson RW, Hamrick JC, et al. Discovery of Taniborbactam (VNRX-5133): a broad-spectrum serine- and metallo-β-lactamase inhibitor for carbapenem-resistant bacterial infections. J Med Chem. (2020) 63:2789–801. doi: 10.1021/acs.jmedchem.9b01518
188. Mushtaq S, Vickers A, Woodford N, Haldimann A, Livermore DM. Activity of nacubactam (RG6080/OP0595) combinations against MBL-producing Enterobacteriaceae. J Antimicrob Chemother. (2019) 74:953–60. doi: 10.1093/jac/dky522
189. Lasko MJ, Nicolau DP. Carbapenem-resistant enterobacterales: considerations for treatment in the era of new antimicrobials and evolving enzymology. Curr Infect Dis Rep. (2020) 22:6. doi: 10.1007/s11908-020-0716-3
190. Hackel MA, Lomovskaya O, Dudley MN, Karlowsky JA, Sahm DF. In vitro activity of meropenem-vaborbactam against clinical isolates of KPC-positive Enterobacteriaceae. Antimicrob Agents Chemother. (2017) 62:e01904-17. doi: 10.1128/AAC.01904-17
191. Pfaller MA, Huband MD, Mendes RE, Flamm RK, Castanheira M. In vitro activity of meropenem/vaborbactam and characterisation of carbapenem resistance mechanisms among carbapenem-resistant Enterobacteriaceae from the 2015 meropenem/vaborbactam surveillance programme. Int J Antimicrob Agents. (2018) 52:144–50. doi: 10.1016/j.ijantimicag.2018.02.021
192. Biagi M, Shajee A, Vialichka A, Jurkovic M, Tan X, Wenzler E. Activity of imipenem-relebactam and meropenem-vaborbactam against carbapenem-resistant, SME-producing Serratia marcescens. Antimicrob Agents Chemother. (2020) 64:e02255-19. doi: 10.1128/AAC.02255-19
193. Lob SH, Hackel MA, Kazmierczak KM, Young K, Motyl MR, Karlowsky JA, et al. In vitro activity of imipenem-relebactam against gram-negative ESKAPE pathogens isolated by Clinical Laboratories in the United States in 2015 (Results from the SMART Global Surveillance Program). Antimicrob Agents Chemother. (2017) 61:e02209-16. doi: 10.1128/AAC.02209-16
194. Sader HS, Rhomberg PR, Flamm RK, Jones RN, Castanheira M. WCK 5222 (cefepime/zidebactam) antimicrobial activity tested against Gram-negative organisms producing clinically relevant β-lactamases. J Antimicrob Chemother. (2017) 72:1696–703. doi: 10.1093/jac/dkx050
195. Hamrick JC, Docquier J-D, Uehara T, Myers CL, Six DA, Chatwin CL, et al. VNRX-5133 (Taniborbactam), a broad-spectrum inhibitor of serine- and metallo-β-lactamases, restores activity of cefepime in Enterobacterales and Pseudomonas aeruginosa. Antimicrob Agents Chemother. (2020) 64:e01963-19. doi: 10.1128/AAC.01963-19
Keywords: CHDL, carbapenem, transposon, insertion sequence, antibiotic resistance, detection
Citation: Bonnin RA, Jousset AB, Emeraud C, Oueslati S, Dortet L and Naas T (2021) Genetic Diversity, Biochemical Properties, and Detection Methods of Minor Carbapenemases in Enterobacterales. Front. Med. 7:616490. doi: 10.3389/fmed.2020.616490
Received: 12 October 2020; Accepted: 09 December 2020;
Published: 20 January 2021.
Edited by:
Raffaele Zarrilli, University of Naples Federico II, ItalyReviewed by:
Sanda Sardelic, University Hospital Split, CroatiaSophia Vourli, University General Hospital Attikon, Greece
Copyright © 2021 Bonnin, Jousset, Emeraud, Oueslati, Dortet and Naas. This is an open-access article distributed under the terms of the Creative Commons Attribution License (CC BY). The use, distribution or reproduction in other forums is permitted, provided the original author(s) and the copyright owner(s) are credited and that the original publication in this journal is cited, in accordance with accepted academic practice. No use, distribution or reproduction is permitted which does not comply with these terms.
*Correspondence: Rémy A. Bonnin, remy.bonnin@u-psud.fr