- 1Department of Hepatology and Gastroenterology, Aarhus University Hospital, Aarhus, Denmark
- 2Institute for Liver and Digestive Health, University College London, London, United Kingdom
- 3Storr Liver Centre, Westmead Institute for Medical Research, University of Sydney and Westmead Hospital, Westmead, NSW, Australia
- 4Department of Clinical Biochemistry, Aarhus University Hospital, Aarhus, Denmark
Macrophages are essential components of the human host immune system, which upon activation facilitates a broad pallet of immunomodulatory events including release of pro- or anti-inflammatory cytokines and chemokines, restoration of immune homeostasis and/or wound healing. Moreover, some macrophage phenotypes are crucially involved in fibrogenesis through stimulation of myofibroblasts, while others promote fibrolysis. During the last decades, the role of resident liver macrophages viz. Kupffer cells and recruited monocytes/macrophages in acute and chronic liver diseases has gained interest and been extensively investigated. Specifically, the scavenger receptors CD163 and mannose receptor (CD206), expressed by macrophages, are of utmost interest since activation by various stimuli induce their shedding to the circulation. Thus, quantifying concentrations of these soluble biomarkers may be of promising clinical relevance in estimating the severity of inflammation and fibrosis and to predict outcomes such as survival. Here, we review the existing literature on soluble CD163 and soluble mannose receptor in liver diseases with a particular focus on their relationship to hepatic fibrosis in metabolic associated fatty liver disease, as well as in chronic hepatitis B and C.
Introduction
The progression course from low grade hepatic inflammation and early stage fibrosis to manifest cirrhosis differs depending on disease etiology but also between subgroups within the same disease (1). With established cirrhosis follow substantial reductions in quality of life and survival, especially when decompensation develops with variceal bleeding, hepatic encephalopathy, ascites and/or hepatorenal syndrome or the development of liver cancer (2–4).
The pathomechanisms orchestrating disease progression and maintenance of inflammation and fibrosis are regulated through a complex interplay between immune cells. Resident liver macrophages viz. Kupffer cells along with recruited monocytes/macrophages are essential in the development and progression of liver diseases (5). Macrophages are key players of human innate immunity displaying an extensive array of membrane receptors, which mediate crucial immunomodulatory responses upon macrophage activation with both pro- and anti-inflammatory effects (6, 7). We review the value of two biomarkers of macrophage activation in liver diseases and their relationship to the degree of fibrosis, ultimately concluding with the potential clinical utility of these biomarkers. We briefly comment on macrophage-related therapeutic options.
Macrophages and Liver Fibrosis
Kupffer cells and recruited macrophages possess the ability to exert omnipotent immunomodulation, evident by both pro-fibrotic and anti-fibrotic effects in the liver (8). Traditionally, macrophages have been divided in two subgroups with either a “pro-inflammatory” M1 or “immune-regulatory” M2 phenotype. However, this dichotomous classification is a too simplified division of a highly heterogeneous group of differently activated and functioning immune cells as recently affirmed by advanced molecular sequencing techniques (8–11).
Pathogens reaching the hepatic immune environment initiate a pro-inflammatory response with Kupffer cells as essential partakers and a major source of inflammatory signaling molecules (12). Macrophage activation by pathogen-associated molecular patterns (PAMPs) through pattern-recognizing receptors (PRRs) including Toll-like-receptors is of utmost relevance in the immunological processes during chronic inflammation. Similarly, macrophages may be activated by damage-associated molecular patterns (DAMPs) (Figure 1) (13). The cytokines interleukin-12 (IL-12) and interferon-γ (IFN-γ) are known to initiate a pro-inflammatory response in macrophages, resulting in the release of tumor necrosis factor (TNF), IL-1β, IL-6, IL-12 and reactive oxygen species (7–9). Furthermore, the presence of a IFNL3-IFNL4 haplotype resulting in production of IFN-γ3 is recognized as a promoter of hepatic inflammation and fibrosis progression (14). Through the release of pro-inflammatory cytokines and chemokines, macrophages interplay with other immune cells including T cells, B cells, natural killer T cells and neutrophils (8, 15). Conversely, the release of modulatory cytokines such as IL-10, transforming growth factor β (TGF-β), IL-4 and IL-13, induced by adaptive and innate signals, is of key importance in dampening inflammation and promoting wound healing (7–9, 16). In this view, TGF-β potently stimulates hepatic stellate cell differentiation into myofibroblasts (17) responsible for fibrogenesis during bouts of hepatic inflammation. Myofibroblasts undergo persistent proliferation contributing to disease progression by synthesis of extracellular matrix components and potentiation of ongoing inflammation with the release of chemokines, cytokines and fibrogenic mediators (18–20). Upon dampening of liver injury, histoarchitecture restorative events become dominating with deactivation of myofibroblasts by apoptosis or phenotypic switch and activation of protective or restorative macrophages, which promote fibrosis resolution and tissue remodeling (8, 18, 21), although the mechanisms underlying this phenotypic switch have not been fully elucidated.
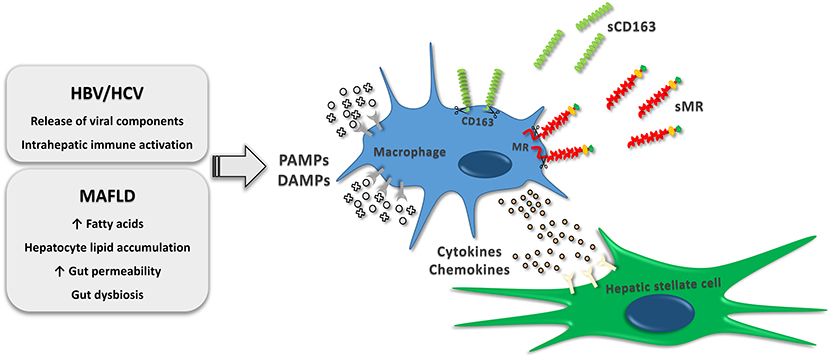
Figure 1. Macrophage activation by immunomodulatory events occurring in MAFLD and chronic HBV or HCV infections. The activation increase receptor shedding, which results in release of soluble CD163 and soluble MR to the circulation. Furthermore, cytokines and chemokines released by resident and recruited liver macrophages stimulate hepatic stellate cell differentiation into myofibroblasts consequently causing synthesis of extracellular matrix components. HBV, hepatitis B virus; HCV, hepatitis C virus; MAFLD, metabolic associated fatty liver disease; PAMPs, pathogen-associated molecular patterns; DAMPs, damage-associated molecular patterns; sCD163, soluble cluster of differentiation 163; sMR, soluble mannose receptor.
Macrophage Activation Markers
As discussed above, macrophages play a crucial role in both hepatic fibrogenesis and resolution of fibrosis, and several macrophage-released molecules are involved in these processes. Thus, plasma concentrations of such molecules may reflect the degree of fibrosis in liver diseases and help clinicians determine disease stage, prognosis, and response to intervention.
Scavenger receptors are expressed by macrophages as part of a large receptor panel accountable for regulation of endocytosis, phagocytosis, adhesion, and signaling. Some scavenger receptors are promising indicators of the inflammatory load in liver diseases and have a potential prognostic value (22). The most intensively studied proteins are cluster of differentiation 163 (CD163) and the mannose receptor (MR/CD206). CD163, a hemoglobin-haptoglobin scavenger receptor, is exclusively expressed by monocytes and macrophages (23, 24) and exerts its main biological function through elimination of hemoglobin-haptoglobin complexes during hemolysis (25). A soluble form (sCD163) is present in the plasma (26), and enzymatic receptor cleavage of CD163 by the TACE/ADAM17 system is highly upregulated in response to inflammatory stimuli, including the PAMP lipopolysaccharide (LPS) (27–30). The mannose receptor is primarily expressed by macrophages, dendritic cells and endothelial cells (31) and is involved in scavenging events including endogenous molecule clearance besides antigen presentation (32). Similar to sCD163, soluble mannose receptor (sMR) is present in plasma, and shedding by proteases is induced by PAMPs (Figure 1) (28, 33). Even though sCD163 and sMR share similarities regarding expression and stimuli leading to shedding, and their concentrations inter-correlate indicating concurrent shedding from activated macrophages, the biomarkers possess different immunogenic roles and may be differently regulated with fluctuations of serum concentrations in diverse immunological conditions (22). Furthermore, the very shedding intensity induced by various stimuli may differ between the two biomarkers (34).
Macrophage Activation Markers in Liver Diseases
The dynamic processes of hepatic inflammation have been extensively studied over the last decades with increasing focus on macrophage activation markers and their utility in staging the degree of ongoing inflammation and fibrosis, as well as predicting disease outcome. In this review, when discussing non-alcoholic fatty liver disease (NAFLD), we will use the recently introduced and more appropriate consensus nomenclature of metabolic associated fatty liver disease (MAFLD) (35, 36). As previously summarized and illustrated in (22, 37, 38) plasma levels of sCD163 are moderately elevated in MAFLD (39), chronic hepatitis B virus (HBV) and chronic hepatitis C virus (HCV) infections (40), Wilson's disease (41, 42), and primary biliary cholangitis (43), and clearly reflects disease severity in MAFLD, HBV and HCV (39, 40, 44–47). In further support of a correlation between sCD163 concentrations and liver disease severity sCD163 levels show significant reductions after lifestyle interventions in MAFLD (48, 49) and after antiviral therapy in HBV and HCV (46, 50, 51). In manifest cirrhosis, sCD163 levels are even higher with a dramatic stepwise increase in parallel with Child-Pugh- and MELD-scores (52). Moreover, sCD163 values predict the degree of portal hypertension (53, 54) and are associated with variceal bleeding and prognosis (55, 56). In cirrhotic patients with hepatocellular carcinoma, sCD163 levels associate significantly with overall survival (57, 58). Hepatic expression of CD163 is significantly increased in patients with acute viral hepatitis compared with chronic viral hepatitis (59). Accordingly, the most prominent elevations of plasma sCD163 are seen in acute liver injury with intense inflammation including alcoholic hepatitis (60, 61), acute liver failure (62, 63), and acute-on-chronic liver failure (ACLF) (64), where it shows great potential as an independent predictor of short-term mortality.
Similarly, sMR has become of increasing interest as a marker of inflammation in liver disease. In children with MAFLD, sMR is elevated compared with non-overweight controls (65). sMR mimics sCD163 in chronic HBV and HCV, with increasing plasma concentrations in association with incrementing severity of hepatic inflammation (51, 66) and persistent reduction after antiviral therapies (51, 67). sMR is substantially elevated in patients with cirrhosis with significant correlation with Child-Pugh-score (56). Furthermore, it is a predictor of long-term survival and for the occurrence of cirrhosis-associated complications including decompensating events such as ascites, hepatic encephalopathy, and upper gastrointestinal hemorrhage due to portal hypertension within 1 year (56). Lastly, patients suffering from acute liver injury due to acetaminophen overdose, acute cirrhosis decompensation, ACLF, and alcoholic hepatitis have markedly increased plasma levels of sMR with 2.5- to 5-fold higher median values compared with healthy individuals (64, 68, 69). In addition, combining the CLIF-C ACLF score and sCD163 improves the prediction of 90 days mortality, while sMR in addition to the CLIF-C acute decompensation (AD) score improves the prediction of 90 and 180 days mortality (64, 70).
In summary, multiple studies have documented that plasma concentrations of the macrophage activation markers sMR and especially sCD163 are reliable indicators of ongoing hepatic inflammation, and potential useful tools to predict disease outcome including mortality.
sCD163 and sMR in Liver Fibrosis
As outlined above, a pivotal event in the hepatic inflammatory response is activation of resident and recruited macrophages responsible for further signaling events and subsequently resulting in a spectrum of scenarios ranging from resolution of inflammation to maintenance or intensification of the response, activation of hepatic stellate cells with fibrosis development, and/or induction of fibrolysis. There is a solid foundation to consider sCD163 and sMR as important and clinically useful markers of inflammation in liver disease, but they may equally reflect fibrosis severity. The latter will be reviewed below with a specific focus on MAFLD and chronic HBV and HCV where the most robust data is available.
MAFLD
The prevalence of obesity is increasing worldwide leading to a substantially increased health care burden related to diseases associated with obesity. This includes MAFLD and MAFLD with steatohepatitis with or without fibrosis (1, 35, 36). MAFLD is related to insulin resistance, obesity, type 2 diabetes mellitus (71); and suggested to be the hepatic manifestation of the metabolic syndrome (72, 73). Key immunomodulatory events comprise hepatic lipid accumulation and increased translocation of bacterial components from the gut. If the lipid load surpasses the hepatic metabolic capacity, the dysregulated hepatic lipid metabolism will result in toxic lipid intermediates. These intermediates will be recognized as DAMPs by liver macrophages and initiate activation through PRRs. Gut derived bacterial components (e.g., LPS) potentiates this process, further increasing liver inflammation (Figure 1). A recent study of 40 non-diabetic and mostly non-obese patients with biopsy-proven MAFLD and varying severity of hepatic steatosis and fibrosis, indicated a link between adipose tissue insulin resistance and Kupffer cell activation since sCD163 concentrations associated with circulating free fatty acids, lipolysis rate and insulin resistance in adipose tissue (74). Persistent and excessive inflammation entails fibrogenic events including activation of myofibroblasts, which are highly dependent on release of inflammatory and pro-fibrotic cytokines such as TGF-β from activated Kupffer cells and recruited macrophages (74–76). Even though fibrosis progresses slowly in MAFLD (77) ~20% are rapid progressors (78), and there is an urgent need for improved tools to non-invasively diagnose and evaluate liver fibrosis as well as to predict progression risks and treatment effects.
In morbidly obese adults undergoing bariatric surgery and perioperative liver biopsy, sCD163 was significantly associated with the Kleiner fibrosis score. In addition, patients with a high fibrosis score had significantly higher preoperative sCD163 levels (39), an association confirmed in two independent cohorts of patients with biopsy-proven MAFLD (Figure 2A). sCD163 as a sole marker performed well in detecting advanced fibrosis, and the addition of sCD163 to the established and widely applied model NAFLD Fibrosis Score (NAFLD-FS) improved the predictive capacity of the latter (44). sCD163 and NAFLD-FS had comparable sensitivities, 84 and 80%, respectively, for predicting advanced fibrosis (F ≥ 3) using low cut-off values in an Australian cohort. However, in an Italian cohort the sensitivities of sCD163 and NAFLD-FS for predicting advanced fibrosis were much lower (~40%), though still comparable (44). Another research group reported a similar significant association between sCD163 and hepatic fibrosis in obese individuals undergoing bariatric surgery (45). Recently, results from 40 patients with MAFLD and available liver histology and sCD163 quantifications as well as a comprehensive metabolic assessment clearly demonstrated an association between sCD163 and the stage of fibrosis (74).
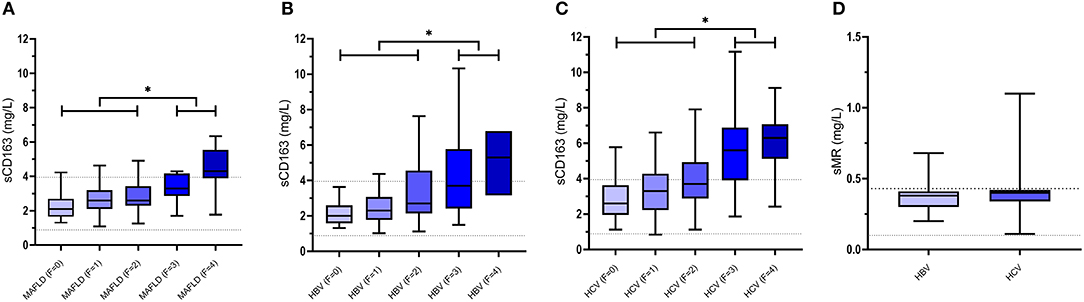
Figure 2. Concentrations of sCD163 in MAFLD (A), chronic HBV infections (B) and chronic HCV infections (C) as well as concentrations of sMR in chronic HBV infections and chronic HCV infections (D) presented by means/medians, interquartile ranges as well as minimum and maximum values. In patients with MAFLD, chronic HBV infections and chronic HCV infections plasma levels of sCD163 increase with increasing fibrosis grade (F0-F4) (40, 44, 51, 67) with significantly higher levels in patients with fibrosis grades F3-F4 compared with patients with fibrosis grades F0-F2 (*p-value <0.001). Dotted lines represent upper and lower reference values for healthy controls (26, 34). MAFLD, metabolic associated fatty liver disease; HBV, hepatitis B virus; HCV, hepatitis C virus; sCD163, soluble cluster of differentiation 163; sMR, soluble mannose receptor.
In obese children undergoing lifestyle interventions, sCD163 was significantly higher at baseline compared with 1-year follow-up in children with a high level of a non-invasive surrogate measure for fibrosis [the Pediatric NAFLD Fibrosis Index (79)]. Following lifestyle intervention, sCD163 levels decreased in association with improvements in metabolic dysfunction (48). However, a recent study of sCD163 and sMR in children with MAFLD reported no significant associations between either sCD163 or sMR and fibrosis assessed from liver biopsies, which was in clear contrast with the results shown in adult cohorts and may suggest different mechanisms in terms of macrophage activation and fibrosis development in children compared with adults with MAFLD (65).
HBV and HCV
Chronic infection with HBV and HCV is maintained by continued release of viral components by infected cells (80, 81), thus interacting with the intrahepatic innate immune system including Kupffer cells and hepatic stellate cells (Figure 1). These are directly activated by HBV and HCV components in addition to an indirect stimulation by differentiated pro-fibrogenic liver macrophages (82, 83). Genetic studies have recently elucidated an association between sCD163 levels and expression of specific gene variants known to promote hepatic inflammation and fibrogenesis in patients with chronic HCV, thus supporting a pivotal role for Kupffer cell activation in the development and progression of hepatic fibrosis (14, 84). Several studies have measured sCD163 and sMR in chronically HBV and HCV infected individuals and related biomarker concentrations to fibrosis scores. In a small population of HCV patients assessed by the non-invasive FibroScan® sCD163 was associated with liver fibrosis (66). Data concerning liver histology and biochemistry from a large treatment-naïve cohort of 513 patients with chronic HCV and 200 patients with chronic HBV were published in 2014 (40). In general, HCV patients had higher sCD163 concentrations for the same fibrosis score as HBV patients. In both HCV and HBV, sCD163 increased in association with histological stages of fibrosis (Figures 2B,C). Supporting a direct association between sCD163 and fibrosis in HCV patients a CD163-HCV-fibrosis-score was superior to the common fibrosis scoring tools, APRI and FIB-4, for predicting significant fibrosis (40). The sCD163-based HCV fibrosis score has been successfully validated (50). A CD163-HBV-fibrosis-score was also presented and evaluated, but was not significantly superior to APRI and FIB-4 (40). Dultz et al. showed that chronic HBV patients with an Ishak fibrosis score ≥2 had significantly higher levels of plasma sCD163 than F0-F1 patients. Thus, sCD163 may be a useful biomarker to discriminate chronic HBV treatment-naïve patients with minimal fibrosis from patients with significant fibrosis (46). Recently, sCD163 and sMR were measured in a cohort of chronic HBV patients before and after nucleoside-analogue treatment (Figure 2D). The two macrophage activation markers showed a weak association with the Ishak fibrosis score (51). In this study, the CD163-HBV-fibrosis-score was validated and performed similarly to APRI and FIB-4 for prediction of significant fibrosis (51). A similar study of sCD163 and sMR in chronic HCV patients reported significant reductions of liver stiffness evaluated by transient elastography (FibroScan® or ARFI scan®) following antiviral treatment (Figure 2D). Concentrations of sCD163 and sMR correlated with liver stiffness at baseline and follow-up, though no consistent conclusion on the predictive value of the markers in relation to fibrosis severity was presented (67). Hence, the high baseline concentrations of sCD163 and sMR may not solely reflect fibrosis, since significantly higher viral load and alanine aminotransferase were present at baseline, indicating a considerable component of active liver inflammation.
In summary, there is a well-documented association between plasma concentrations of sCD163 and hepatic fibrosis in patients with chronic HBV and HCV, with a decline after antiviral treatment. The results for HCV are the most concordant.
Concluding Remarks
Plasma levels of sCD163 and sMR may be clinically useful as they directly reflect macrophage activation in liver diseases. Since macrophages play a significant immunomodulatory role in acute and chronic inflammatory liver disease, including an interplay with hepatic stellate cells, sCD163 and sMR have gained interest as potential markers of hepatic inflammation and fibrosis. Results from several studies in humans indicate usefulness of sCD163 and sMR in estimating the degree of ongoing inflammation in acute and chronic liver diseases in general and in assessing fibrosis severity in patients with MAFLD as well as chronic HBV and HCV infections. Since hepatic inflammation and fibrosis are inter-related events—both being consequences of the continuum of immunological activities in chronic liver diseases—the inflammatory burden must be considered as a possible limitation to the use of sCD163 and sMR as biomarkers of liver fibrosis. Moreover, sCD163 may be elevated in other diseases involving activation of monocytes and macrophages, including Gaucher disease (characterized by excessive macrophage proliferation), hemophagocytic syndrome, infectious diseases, chronic inflammatory diseases, and leukemia, and may be markedly elevated in septic patients (85), limiting its clinical utility. Pulmonary fibrosis may also result in elevations of circulating sCD163 (86). Hence, critical and thorough evaluation of the clinical setting is important before sCD163 and sMR are measured with the purpose of assessing liver fibrosis. However, in the urgent need for improved non-invasive disease scoring tools, especially concerning MAFLD and chronic viral hepatitis B and C, sMR and sCD163 possess great potentials toward being included in fibrosis assessments and may even reflect treatment effects. Further, from a therapeutic perspective, the membrane bound CD163 and perhaps MR may hold promise as entry molecules for liver macrophage-targeted drug delivery (87, 88). An advantage of CD163 is rapid internalization of ligands limiting systemic drug exposure (89) as well as providing a directed action of the applied drug (90). Consequently, intravenous injections of CD163-directed anti-IgG-dexamethasone conjugate in rats on a high fructose diet significantly reduced hepatic inflammation and fibrosis (88). However, future studies in humans are needed to further elucidate the therapeutic potential of macrophage receptors, thereby possibly extending the treatment options for patients with chronic liver diseases.
Author Contributions
RG and HG: conceptualization. RG: writing–original draft. RG, MK, TL, KK, JG, HM, and HG: writing–review and editing. All authors approved the final version of the manuscript.
Funding
HG received research funding from Intercept, Abbvie, NOVO Nordisk Foundation, Arla, and ADS AIPHIA Development Services AG. Advisory board at Ipsen. Speaker Norgine. RG received research funding from ADS AIPHIA Development Services AG.
Conflict of Interest
The authors declare that the research was conducted in the absence of any commercial or financial relationships that could be construed as a potential conflict of interest.
References
1. Asrani SK, Devarbhavi H, Eaton J, Kamath PS. Burden of liver diseases in the world. J Hepatol. (2019) 70:151–71. doi: 10.1016/j.jhep.2018.09.014
2. Ge PS, Runyon BA. Treatment of patients with cirrhosis. N Engl J Med. (2016) 375:767–77. doi: 10.1056/NEJMra1504367
3. Jepsen P, Ott P, Andersen PK, Sørensen HT, Vilstrup H. Clinical course of alcoholic liver cirrhosis: a Danish population-based cohort study. Hepatology. (2010) 51:1675–82. doi: 10.1002/hep.23500
4. Fleming KM, Aithal GP, Card TR, West J. All-cause mortality in people with cirrhosis compared with the general population: a population-based cohort study. Liver Int Off J Int Assoc Study Liver. (2012) 32:79–84. doi: 10.1111/j.1478-3231.2011.02517.x
5. Gao B, Tsukamoto H. Inflammation in alcoholic and nonalcoholic fatty liver disease: friend or foe? Gastroenterology. (2016) 150:1704–9. doi: 10.1053/j.gastro.2016.01.025
6. Gordon S, Martinez-Pomares L. Physiological roles of macrophages. Pflugers Arch. (2017) 469:365–74. doi: 10.1007/s00424-017-1945-7
7. Mosser DM, Edwards JP. Exploring the full spectrum of macrophage activation. Nat Rev Immunol. (2008) 8:958–69. doi: 10.1038/nri2448
8. Tacke F, Zimmermann HW. Macrophage heterogeneity in liver injury and fibrosis. J Hepatol. (2014) 60:1090–6. doi: 10.1016/j.jhep.2013.12.025
9. Porcheray F, Viaud S, Rimaniol AC, Leone C, Samah B, Dereuddre-Bosquet N, et al. Macrophage activation switching: an asset for the resolution of inflammation. Clin Exp Immunol. (2005) 142:481–9. doi: 10.1111/j.1365-2249.2005.02934.x
10. Xiong X, Kuang H, Ansari S, Liu T, Gong J, Wang S, et al. Landscape of intercellular crosstalk in healthy and NASH liver revealed by single-cell secretome gene analysis. Mol Cell. (2019) 75:644–60.e5. doi: 10.1016/j.molcel.2019.07.028
11. Ramachandran P, Dobie R, Wilson-Kanamori JR, Dora EF, Henderson BEP, Luu NT, et al. Resolving the fibrotic niche of human liver cirrhosis at single-cell level. Nature. (2019) 575:512–8. doi: 10.1038/s41586-019-1631-3
12. Wynn TA, Vannella KM. Macrophages in tissue repair, regeneration, and fibrosis. Immunity. (2016) 44:450–62. doi: 10.1016/j.immuni.2016.02.015
13. Medzhitov R. Origin and physiological roles of inflammation. Nature. (2008) 454:428–35. doi: 10.1038/nature07201
14. Eslam M, McLeod D, Kelaeng KS, Mangia A, Berg T, Thabet K, et al. IFN-λ3, not IFN-λ4, likely mediates IFNL3-IFNL4 haplotype-dependent hepatic inflammation and fibrosis. Nat Genet. (2017) 49:795–800. doi: 10.1038/ng.3836
15. Wynn TA, Barron L. Macrophages: master regulators of inflammation and fibrosis. Semin Liver Dis. (2010) 30:245–57. doi: 10.1055/s-0030-1255354
16. Koyama Y, Brenner DA. Liver inflammation and fibrosis. J Clin Invest. (2017) 127:55–64. doi: 10.1172/JCI88881
17. Pradere J-P, Kluwe J, De Minicis S, Jiao J-J, Gwak G-Y, Dapito DH, et al. Hepatic macrophages but not dendritic cells contribute to liver fibrosis by promoting the survival of activated hepatic stellate cells in mice. Hepatology. (2013) 58:1461–73. doi: 10.1002/hep.26429
18. Novo E, Bocca C, Foglia B, Protopapa F, Maggiora M, Parola M, et al. Liver fibrogenesis: un update on established and emerging basic concepts. Arch Biochem Biophys. (2020) 689:108445. doi: 10.1016/j.abb.2020.108445
19. Parola M, Marra F, Pinzani M. Myofibroblast - like cells and liver fibrogenesis: emerging concepts in a rapidly moving scenario. Mol Aspects Med. (2008) 29:58–66. doi: 10.1016/j.mam.2007.09.002
20. Seki E, Schwabe RF. Hepatic inflammation and fibrosis: functional links and key pathways. Hepatology. (2015) 61:1066–79. doi: 10.1002/hep.27332
21. Lee YA, Wallace MC, Friedman SL. Pathobiology of liver fibrosis: a translational success story. Gut. (2015) 64:830–41. doi: 10.1136/gutjnl-2014-306842
22. Nielsen MC, Hvidbjerg Gantzel R, Clària J, Trebicka J, Møller HJ, Grønbæk H. Macrophage activation markers, CD163 and CD206, in acute-on-chronic liver failure. Cells. (2020) 9:1175. doi: 10.3390/cells9051175
23. Pulford K, Micklem K, McCarthy S, Cordell J, Jones M, Mason DY. A monocyte/macrophage antigen recognized by the four antibodies GHI/61, Ber-MAC3, Ki-M8 and SM4. Immunology. (1992) 75:588–95.
24. Backe E, Schwarting R, Gerdes J, Ernst M, Stein H. Ber-MAC3: new monoclonal antibody that defines human monocyte/macrophage differentiation antigen. J Clin Pathol. (1991) 44:936–45. doi: 10.1136/jcp.44.11.936
25. Kristiansen M, Graversen JH, Jacobsen C, Sonne O, Hoffman HJ, Law SK, et al. Identification of the haemoglobin scavenger receptor. Nature. (2001) 409:198–201. doi: 10.1038/35051594
26. Moller HJ, Peterslund NA, Graversen JH, Moestrup SK. Identification of the hemoglobin scavenger receptor/CD163 as a natural soluble protein in plasma. Blood. (2002) 99:378–80. doi: 10.1182/blood.V99.1.378
27. Weaver LK, Hintz-Goldstein KA, Pioli PA, Wardwell K, Qureshi N, Vogel SN, et al. Pivotal advance: activation of cell surface Toll-like receptors causes shedding of the hemoglobin scavenger receptor CD163. J Leukoc Biol. (2006) 80:26–35. doi: 10.1189/jlb.1205756
28. Nielsen MC, Andersen MN, Rittig N, Rodgaard-Hansen S, Gronbaek H, Moestrup SK, et al. The macrophage-related biomarkers sCD163 and sCD206 are released by different shedding mechanisms. J Leukoc Biol. (2019) 106:1129–38. doi: 10.1002/JLB.3A1218-500R
29. Rittig N, Svart M, Jessen N, Møller N, Møller HJ, Grønbæk H. Macrophage activation marker sCD163 correlates with accelerated lipolysis following LPS exposure: a human-randomised clinical trial. Endocr Connect. (2018) 7:107–14. doi: 10.1530/EC-17-0296
30. Svart M, Rittig N, Møller N, Møller HJ, Gronbaek H. Soluble CD163 correlates with lipid metabolic adaptations in type 1 diabetes patients during ketoacidosis. J Diabetes Investig. (2019) 10:67–72. doi: 10.1111/jdi.12869
31. Groger M, Holnthoner W, Maurer D, Lechleitner S, Wolff K, Mayr BB, et al. Dermal microvascular endothelial cells express the 180-kDa macrophage mannose receptor in situ and in vitro. J Immunol. (2000) 165:5428–34. doi: 10.4049/jimmunol.165.10.5428
32. Martinez-Pomares L. The mannose receptor. J Leukoc Biol. (2012) 92:1177–86. doi: 10.1189/jlb.0512231
33. Fraser IP, Takahashi K, Koziel H, Fardin B, Harmsen A, Ezekowitz RA. Pneumocystis carinii enhances soluble mannose receptor production by macrophages. Microbes Infect. (2000) 2:1305–10. doi: 10.1016/S1286-4579(00)01283-1
34. Rodgaard-Hansen S, Rafique A, Christensen PA, Maniecki MB, Sandahl TD, Nexo E, et al. A soluble form of the macrophage-related mannose receptor (MR/CD206) is present in human serum and elevated in critical illness. Clin Chem Lab Med. (2014) 52:453–61. doi: 10.1515/cclm-2013-0451
35. Eslam M, Newsome PN, Sarin SK, Anstee QM, Targher G, Romero-Gomez M, et al. A new definition for metabolic dysfunction-associated fatty liver disease: an international expert consensus statement. J Hepatol. (2020) 73:202–9. doi: 10.1016/j.jhep.2020.07.045
36. Eslam M, Sanyal AJ, George J. MAFLD: a consensus-driven proposed nomenclature for metabolic associated fatty liver disease. Gastroenterology. (2020) 158:1999–2014.e1. doi: 10.1053/j.gastro.2019.11.312
37. Grønbæk H, Gantzel RH, Laursen TL, Kazankov K, Møller HJ. Macrophage markers and innate immunity in cirrhosis. J Hepatol. (2020) 73:1586–8. doi: 10.1016/j.jhep.2020.07.033
38. Møller HJ, Kazankov K, Rødgaard-hansen S, Nielsen MC, Sandahl TD, Vilstrup H. Soluble CD163 (sCD163): biomarker of kupffer cell activation in liver disease. In: Preedy VR, editor. Biomarkers in Liver Disease. Dordrecht: Springer Science+Business Media (2016).
39. Kazankov K, Tordjman J, Moller HJ, Vilstrup H, Poitou C, Bedossa P, et al. Macrophage activation marker soluble CD163 and non-alcoholic fatty liver disease in morbidly obese patients undergoing bariatric surgery. J Gastroenterol Hepatol. (2015) 30:1293–300. doi: 10.1111/jgh.12943
40. Kazankov K, Barrera F, Moller HJ, Bibby BM, Vilstrup H, George J, et al. Soluble CD163, a macrophage activation marker, is independently associated with fibrosis in patients with chronic viral hepatitis B and C. Hepatology. (2014) 60:521–30. doi: 10.1002/hep.27129
41. Björklund J, Laursen TL, Sandahl TD, Møller HJ, Vilstrup H, Ott P, et al. High hepatic macrophage activation and low liver function in stable Wilson patients - a Danish cross-sectional study. Orphanet J Rare Dis. (2018) 13:169. doi: 10.1186/s13023-018-0910-7
42. Glavind E, Gotthardt DN, Pfeiffenberger J, Sandahl TD, Bashlekova T, Willemoe GL, et al. The macrophage activation marker soluble CD163 is elevated and associated with liver disease phenotype in patients with Wilson's disease. Orphanet J Rare Dis. (2020) 15:173. doi: 10.1186/s13023-020-01452-2
43. Bossen L, Rebora P, Bernuzzi F, Jepsen P, Gerussi A, Andreone P, et al. Soluble CD163 and mannose receptor as markers of liver disease severity and prognosis in patients with primary biliary cholangitis. Liver Int Off J Int Assoc Study Liver. (2020) 40:1408–14. doi: 10.1111/liv.14466
44. Kazankov K, Barrera F, Moller HJ, Rosso C, Bugianesi E, David E, et al. The macrophage activation marker sCD163 is associated with morphological disease stages in patients with non-alcoholic fatty liver disease. Liver Int. (2016) 36:1549–57. doi: 10.1111/liv.13150
45. Mueller JL, Feeney ER, Zheng H, Misdraji J, Kruger AJ, Alatrakchi N, et al. Circulating soluble CD163 is associated with steatohepatitis and advanced fibrosis in nonalcoholic fatty liver disease. Clin Transl Gastroenterol. (2015) 6:e114. doi: 10.1038/ctg.2015.36
46. Dultz G, Gerber L, Farnik H, Berger A, Vermehren J, Pleli T, et al. Soluble CD163 is an indicator of liver inflammation and fibrosis in patients chronically infected with the hepatitis B virus. J Viral Hepat. (2015) 22:427–32. doi: 10.1111/jvh.12309
47. Dultz G, Gerber L, Zeuzem S, Sarrazin C, Waidmann O. The macrophage activation marker CD163 is associated with IL28B genotype and hepatic inflammation in chronic hepatitis C virus infected patients. J Viral Hepat. (2016) 23:267–73. doi: 10.1111/jvh.12488
48. Kazankov K, Moller HJ, Lange A, Birkebaek NH, Holland-Fischer P, Solvig J, et al. The macrophage activation marker sCD163 is associated with changes in NAFLD and metabolic profile during lifestyle intervention in obese children. Pediatr Obes. (2015) 10:226–33. doi: 10.1111/ijpo.252
49. Rodgaard-Hansen S, St George A, Kazankov K, Bauman A, George J, Gronbaek H, et al. Effects of lifestyle intervention on soluble CD163, a macrophage activation marker, in patients with non-alcoholic fatty liver disease. Scand J Clin Lab Invest. (2017) 77:498–504. doi: 10.1080/00365513.2017.1346823
50. Lund Laursen T, Brockner Siggard C, Kazankov K, Damgaard Sandahl T, Moller HJ, Ong A, et al. Rapid and persistent decline in soluble CD163 with successful direct-acting antiviral therapy and associations with chronic hepatitis C histology. Scand J Gastroenterol. (2018) 53:986–93. doi: 10.1080/00365521.2018.1481996
51. Laursen TL, Wong GL-H, Kazankov K, Sandahl T, Moller HJ, Hamilton-Dutoit S, et al. Soluble CD163 and mannose receptor associate with chronic hepatitis B activity and fibrosis and decline with treatment. J Gastroenterol Hepatol. (2018) 33:484–91. doi: 10.1111/jgh.13849
52. Rode A, Nicoll A, Moller HJ, Lim L, Angus PW, Kronborg I, et al. Hepatic macrophage activation predicts clinical decompensation in chronic liver disease. Gut. (2013) 62:1231–2. doi: 10.1136/gutjnl-2012-304135
53. Gronbaek H, Sandahl TD, Mortensen C, Vilstrup H, Moller HJ, Moller S. Soluble CD163, a marker of Kupffer cell activation, is related to portal hypertension in patients with liver cirrhosis. Aliment Pharmacol Ther. (2012) 36:173–80. doi: 10.1111/j.1365-2036.2012.05134.x
54. Sandahl TD, McGrail R, Møller HJ, Reverter E, Møller S, Turon F, et al. The macrophage activation marker sCD163 combined with markers of the Enhanced Liver Fibrosis (ELF) score predicts clinically significant portal hypertension in patients with cirrhosis. Aliment Pharmacol Ther. (2016) 43:1222–31. doi: 10.1111/apt.13618
55. Waidmann O, Brunner F, Herrmann E, Zeuzem S, Piiper A, Kronenberger B. Macrophage activation is a prognostic parameter for variceal bleeding and overall survival in patients with liver cirrhosis. J Hepatol. (2013) 58:956–61. doi: 10.1016/j.jhep.2013.01.005
56. Rainer F, Horvath A, Sandahl TD, Leber B, Schmerboeck B, Blesl A, et al. Soluble CD163 and soluble mannose receptor predict survival and decompensation in patients with liver cirrhosis, and correlate with gut permeability and bacterial translocation. Aliment Pharmacol Ther. (2018) 47:657–64. doi: 10.1111/apt.14474
57. Waidmann O, Köberle V, Bettinger D, Trojan J, Zeuzem S, Schultheiß M, et al. Diagnostic and prognostic significance of cell death and macrophage activation markers in patients with hepatocellular carcinoma. J Hepatol. (2013) 59:769–79. doi: 10.1016/j.jhep.2013.06.008
58. Kazankov K, Rode A, Simonsen K, Villadsen GE, Nicoll A, Møller HJ, et al. Macrophage activation marker soluble CD163 may predict disease progression in hepatocellular carcinoma. Scand J Clin Lab Invest. (2016) 76:64–73. doi: 10.3109/00365513.2015.1099722
59. Hiraoka A, Horiike N, Akbar SMF, Michitaka K, Matsuyama T, Onji M. Expression of CD163 in the liver of patients with viral hepatitis. Pathol Res Pract. (2005) 201:379–84. doi: 10.1016/j.prp.2004.10.006
60. Sandahl TD, Gronbaek H, Moller HJ, Stoy S, Thomsen KL, Dige AK, et al. Hepatic macrophage activation and the LPS pathway in patients with alcoholic hepatitis: a prospective cohort study. Am J Gastroenterol. (2014) 109:1749–56. doi: 10.1038/ajg.2014.262
61. Saha B, Tornai D, Kodys K, Adejumo A, Lowe P, McClain C, et al. Biomarkers of macrophage activation and immune danger signals predict clinical outcomes in alcoholic hepatitis. Hepatology. (2019) 70:1134–49. doi: 10.1002/hep.30617
62. Moller HJ, Gronbaek H, Schiodt FV, Holland-Fischer P, Schilsky M, Munoz S, et al. Soluble CD163 from activated macrophages predicts mortality in acute liver failure. J Hepatol. (2007) 47:671–6. doi: 10.1016/j.jhep.2007.05.014
63. Hiraoka A, Horiike N, Akbar SMF, Michitaka K, Matsuyama T, Onji M. Soluble CD163 in patients with liver diseases: very high levels of soluble CD163 in patients with fulminant hepatic failure. J Gastroenterol. (2005) 40:52–6. doi: 10.1007/s00535-004-1493-8
64. Grønb H, Rødgaard-hansen S, Aagaard NK, Arroyo V, Moestrup SK, Garcia E, et al. Macrophage activation markers predict mortality in patients with liver cirrhosis without or with acute-on-chronic liver failure (ACLF). J Hepatol. (2016) 64:813–22. doi: 10.1016/j.jhep.2015.11.021
65. Kazankov K, Alisi A, Møller HJ, De Vito R, Rittig S, Mahler B, et al. Macrophage markers are poorly associated with liver histology in children with nonalcoholic fatty liver disease. J Pediatr Gastroenterol Nutr. (2018) 67:635–42. doi: 10.1097/MPG.0000000000002111
66. Andersen ES, Rodgaard-Hansen S, Moessner B, Christensen PB, Moller HJ, Weis N. Macrophage-related serum biomarkers soluble CD163 (sCD163) and soluble mannose receptor (sMR) to differentiate mild liver fibrosis from cirrhosis in patients with chronic hepatitis C: a pilot study. Eur J Clin Microbiol Infect Dis. (2014) 33:117–22. doi: 10.1007/s10096-013-1936-3
67. Laursen TL, Siggaard CB, Kazankov K, Sandahl TD, Moller HJ, Tarp B, et al. Time-dependent improvement of liver inflammation, fibrosis and metabolic liver function after successful direct-acting antiviral therapy of chronic hepatitis C. J Viral Hepat. (2020) 27:28–35. doi: 10.1111/jvh.13204
68. Siggaard CB, Kazankov K, Rodgaard-Hansen S, Moller HJ, Donnelly MC, Simpson KJ, et al. Macrophage markers soluble CD163 and soluble mannose receptor are associated with liver injury in patients with paracetamol overdose. Scand J Gastroenterol. (2019) 54:623–32. doi: 10.1080/00365521.2019.1608292
69. Sandahl TD, Stoy SH, Laursen TL, Rodgaard-Hansen S, Moller HJ, Moller S, et al. The soluble mannose receptor (sMR) is elevated in alcoholic liver disease and associated with disease severity, portal hypertension, and mortality in cirrhosis patients. PLoS ONE. (2017) 12:e0189345. doi: 10.1371/journal.pone.0189345
70. Grønbaek H, Møller HJ, Saliba F, Zeuzem S, Albillos A, Ariza X, et al. Improved prediction of mortality by combinations of inflammatory markers and standard clinical scores in patients with acute-on-chronic liver failure and acute decompensation. J Gastroenterol Hepatol. (2020). doi: 10.1111/jgh.15125. [Epub ahead of print].
71. Adams LA, Anstee QM, Tilg H, Targher G. Non-alcoholic fatty liver disease and its relationship with cardiovascular disease and other extrahepatic diseases. Gut. (2017) 66:1138–53. doi: 10.1136/gutjnl-2017-313884
72. Marchesini G, Brizi M, Morselli-Labate AM, Bianchi G, Bugianesi E, McCullough AJ, et al. Association of nonalcoholic fatty liver disease with insulin resistance. Am J Med. (1999) 107:450–5. doi: 10.1016/S0002-9343(99)00271-5
73. Marchesini G, Brizi M, Bianchi G, Tomassetti S, Bugianesi E, Lenzi M, et al. Nonalcoholic fatty liver disease: a feature of the metabolic syndrome. Diabetes. (2001) 50:1844–50. doi: 10.2337/diabetes.50.8.1844
74. Rosso C, Kazankov K, Younes R, Esmaili S, Marietti M, Sacco M, et al. Crosstalk between adipose tissue insulin resistance and liver macrophages in non-alcoholic fatty liver disease. J Hepatol. (2019) 71:1012–21. doi: 10.1016/j.jhep.2019.06.031
75. Krenkel O, Tacke F. Macrophages in nonalcoholic fatty liver disease: a role model of pathogenic immunometabolism. Semin Liver Dis. (2017) 37:189–97. doi: 10.1055/s-0037-1604480
76. Baffy G. Kupffer cells in non-alcoholic fatty liver disease: the emerging view. J Hepatol. (2009) 51:212–23. doi: 10.1016/j.jhep.2009.03.008
77. Singh S, Allen AM, Wang Z, Prokop LJ, Murad MH, Loomba R. Fibrosis progression in nonalcoholic fatty liver vs nonalcoholic steatohepatitis: a systematic review and meta-analysis of paired-biopsy studies. Clin Gastroenterol Hepatol Off Clin Pract J Am Gastroenterol Assoc. (2015) 13:640–3. doi: 10.1016/j.cgh.2014.04.014
78. Younossi ZM, Stepanova M, Younossi Y, Golabi P, Mishra A, Rafiq N, et al. Epidemiology of chronic liver diseases in the USA in the past three decades. Gut. (2020) 69:564–8. doi: 10.1136/gutjnl-2019-318813
79. Nobili V, Alisi A, Vania A, Tiribelli C, Pietrobattista A, Bedogni G. The pediatric NAFLD fibrosis index: a predictor of liver fibrosis in children with non-alcoholic fatty liver disease. BMC Med. (2009) 7:21. doi: 10.1186/1741-7015-7-21
80. Ganem D, Prince AM. Hepatitis B virus infection–natural history and clinical consequences. N Engl J Med. (2004) 350:1118–29. doi: 10.1056/NEJMra031087
81. Ashfaq UA, Javed T, Rehman S, Nawaz Z, Riazuddin S. An overview of HCV molecular biology, replication and immune responses. Virol J. (2011) 8:161. doi: 10.1186/1743-422X-8-161
82. Boltjes A, Movita D, Boonstra A, Woltman AM. The role of Kupffer cells in hepatitis B and hepatitis C virus infections. J Hepatol. (2014) 61:660–71. doi: 10.1016/j.jhep.2014.04.026
83. Heydtmann M. Macrophages in hepatitis B and hepatitis C virus infections. J Virol. (2009) 83:2796–802. doi: 10.1128/JVI.00996-08
84. Thabet K, Asimakopoulos A, Shojaei M, Romero-Gomez M, Mangia A, Irving WL, et al. MBOAT7 rs641738 increases risk of liver inflammation and transition to fibrosis in chronic hepatitis C. Nat Commun. (2016) 7:12757. doi: 10.1038/ncomms12757
85. Moller HJ. Soluble CD163. Scand J Clin Lab Invest. (2012) 72:1–13. doi: 10.3109/00365513.2011.626868
86. Shimizu K, Ogawa F, Yoshizaki A, Akiyama Y, Kuwatsuka Y, Okazaki S, et al. Increased serum levels of soluble CD163 in patients with scleroderma. Clin Rheumatol. (2012) 31:1059–64. doi: 10.1007/s10067-012-1972-x
87. Graversen JH, Moestrup SK. Drug trafficking into macrophages via the endocytotic receptor CD163. Membranes (Basel). (2015) 5:228–52. doi: 10.3390/membranes5020228
88. Svendsen P, Graversen JH, Etzerodt A, Hager H, Røge R, Grønbæk H, et al. Antibody-directed glucocorticoid targeting to CD163 in M2-type macrophages attenuates fructose-induced liver inflammatory changes. Mol Ther Methods Clin Dev. (2017) 4:50–61. doi: 10.1016/j.omtm.2016.11.004
89. Granfeldt A, Hvas CL, Graversen JH, Christensen PA, Petersen MD, Anton G, et al. Targeting dexamethasone to macrophages in a porcine endotoxemic model. Crit Care Med. (2013) 41:e309–18. doi: 10.1097/CCM.0b013e31828a45ef
Keywords: liver, fibrosis, macrophages, sCD163, mannose receptor, metabolic associated fatty liver disease, hepatitis B virus, hepatitis C virus
Citation: Gantzel RH, Kjær MB, Laursen TL, Kazankov K, George J, Møller HJ and Grønbæk H (2021) Macrophage Activation Markers, Soluble CD163 and Mannose Receptor, in Liver Fibrosis. Front. Med. 7:615599. doi: 10.3389/fmed.2020.615599
Received: 09 October 2020; Accepted: 11 December 2020;
Published: 08 January 2021.
Edited by:
Rikke Norregaard, Aarhus University, DenmarkReviewed by:
Mark Philipp Kühnel, Hannover Medical School, GermanyEmilia Gore, INSERM U1048 Institut des Maladies Métaboliques et Cardiovasculaires, France
Copyright © 2021 Gantzel, Kjær, Laursen, Kazankov, George, Møller and Grønbæk. This is an open-access article distributed under the terms of the Creative Commons Attribution License (CC BY). The use, distribution or reproduction in other forums is permitted, provided the original author(s) and the copyright owner(s) are credited and that the original publication in this journal is cited, in accordance with accepted academic practice. No use, distribution or reproduction is permitted which does not comply with these terms.
*Correspondence: Henning Grønbæk, henngroe@rm.dk