- 1Vascular Medicine Department and Biosurgical Research Lab (Carpentier Foundation), AP-HP, Georges Pompidou European Hospital, Université de Paris, Paris, France
- 2PARCC, INSERM, Université de Paris, Paris, France
- 3Emergency Department, AP-HP, Georges Pompidou European Hospital, Université de Paris, Paris, France
- 4Intensive Care Unit, AP-HP, Georges Pompidou European Hospital, Université de Paris, Paris, France
- 5Innovative Therapies in Haemostasis, INSERM, Université de Paris, Paris, France
- 6Haematology Department and Biosurgical Research Lab (Carpentier Foundation), AP-HP, Georges Pompidou European Hospital, Université de Paris, Paris, France
- 7Plastic Surgery Department, AP-HP, Georges Pompidou European Hospital, Université de Paris, Paris, France
- 8Biochemistry Department, AP-HP, Georges Pompidou European Hospital, Université de Paris, Paris, France
- 9Haematology Department, AP-HP, Georges Pompidou European Hospital, Université de Paris, Paris, France
- 10Respiratory Medicine Department and Biosurgical Research Lab (Carpentier Foundation), AP-HP, Georges Pompidou European Hospital, Université de Paris, Paris, France
- 11Virology Department, AP-HP, Georges Pompidou European Hospital, Université de Paris, Paris, France
- 12Centre de Recherche des Cordeliers, Functional Genomics of Solid Tumors, INSERM, Université de Paris, Paris, France
- 13Intensive Care Unit and Biosurgical Research Lab (Carpentier Foundation), AP-HP, Georges Pompidou European Hospital, Université de Paris, Paris, France
Background: Coronavirus disease 2019 (COVID-19) has been associated with cardiovascular complications and coagulation disorders.
Objectives: To explore clinical and biological parameters of COVID-19 patients with hospitalization criteria that could predict referral to intensive care unit (ICU).
Methods: Analyzing the clinical and biological profiles of COVID-19 patients at admission.
Results: Among 99 consecutive patients that fulfilled criteria for hospitalization, 48 were hospitalized in the medicine department, 21 were first admitted to the medicine ward department and referred later to ICU, and 30 were directly admitted to ICU from the emergency department. At admission, patients requiring ICU were more likely to have lymphopenia, decreased SpO2, a D-dimer level above 1,000 ng/mL, and a higher high-sensitivity cardiac troponin (Hs-cTnI) level. A receiver operating characteristic curve analysis identified Hs-cTnI above 9.75 pg/mL as the best predictive criteria for ICU referral [area under the curve (AUC), 86.4; 95% CI, 76.6–96.2]. This cutoff for Hs-cTnI was confirmed in univariate [odds ratio (OR), 22.8; 95% CI, 6.0–116.2] and multivariate analysis after adjustment for D-dimer level (adjusted OR, 20.85; 95% CI, 4.76–128.4). Transthoracic echocardiography parameters subsequently measured in 72 patients showed an increased right ventricular (RV) afterload correlated with Hs-cTnI (r = 0.42, p = 0.010) and D-dimer (r = 0.18, p = 0.047).
Conclusion: Hs-cTnI appears to be the best relevant predictive factor for referring COVID-19 patients to ICU. This result associated with the correlation of D-dimer with RV dilatation probably reflects a myocardial injury due to an increased RV wall tension. This reinforces the hypothesis of a COVID-19-associated microvascular thrombosis inducing a higher RV afterload.
1. What is known about this topic?
COVID-19 is associated with cardiovascular complications and coagulation disorders.
Predictive markers of severity and intensive care unit (ICU) referral are required upon hospital admission.
2. What does this paper add?
D-Dimer and high sensitivity cardiac troponin (Hs-cTnI) at hospital admission are prognosis biomarkers for ICU referral.
Hs-cTnI appears the best relevant predictive factor for ICU referral in COVID-19 patients.
D-Dimer and Hs-cTnI elevation are correlated with the increase of right ventricular afterload observed in COVID-19.
Introduction
Severe acute respiratory syndrome coronavirus 2 (SARS-CoV-2) infection can be asymptomatic or lead to the coronavirus disease 2019 (COVID-19), which has not only a very large pattern of respiratory manifestations but also other non-specific symptoms including fever, headache, hemoptysis, nausea, vomiting, and diarrhea also previously described in other coronavirus infections (1, 2). In terms of respiratory symptoms, COVID-19 is characterized by a large spectrum of infectious signs from dry cough and pulmonary edema to acute respiratory distress syndrome (ARDS), requiring hospitalization in intensive care unit (ICU) and leading to death in the most severe cases (2). While patients with cardiovascular comorbidities are described to have the higher mortality rate in published data (3), it is however challenging to provide a risk stratification of disease progression at admission for COVID-19 patients. Respiratory condition can worsen rapidly and is currently difficult to predict. From the very first consultation of the patient, biological markers could help to predict COVID-19 systemic consequences that would require ICU referral.
Several biomarkers of COVID-19 have been associated with the disease severity and progression. COVID-19-associated coagulopathy was found more frequent in fatal COVID-19 cases, and a high D-dimer level has been associated with poor prognosis and in-hospital mortality (4–6). The hypothesis of the presence of microthrombi in lungs and kidneys was suggested after autopsy case series (7–9). In COVID-19, microvascular thrombosis is observed during an inflammatory storm and could participate in damaging capillary endothelium and disrupting the thrombo-protective state of endothelial cells (10–12). SARS-CoV-2 has been shown to infect blood vessels and induce vascular damage in vitro and in vivo (13–15). SARS-CoV-2 can infect cells via the angiotensin-converting enzyme 2 (ACE2) receptor, which is ubiquitous but largely expressed in endothelial cells (16). Endotheliitis could be at the origin of impaired microcirculatory function affecting the lungs and kidneys (15, 17, 18). Furthermore, we previously described the endothelial lesion in patients with hospitalization criteria as a marker of COVID-19 severity at hospital admission (19). Acute myocardial injuries were also largely reported, such as numerous acute coronary syndromes or myocarditis (20). Cardiac troponin is increased in the case of community-acquired pneumonia, in the context of myocardial oxygen supply–demand mismatch and supply (21), and seems to correlate with the severity of respiratory impairment. Yet, few data are currently available on troponin level at the time of COVID-19 diagnosis (20, 22–24). Mechanisms of myocardial and tissue injury as well as coagulopathy associated with COVID-19 could be linked to the cytokine storm (25).
In this study, we aimed at identifying, in COVID-19 patients with criteria for hospitalization, biological and clinical markers at admission that could predict future referral in ICU and help anticipating a worsening of the patient's condition. We also investigated correlations between biological markers and cardiac function, assessed by ultrasound.
Methods
Study Design and Population
This study is an observational cohort study conducted at the Georges Pompidou European Hospital in Paris, France. We prospectively included consecutive patients with confirmed SARS-CoV-2 infection. Inclusion criteria were patients over 18 years of age, with COVID-19, who consulted the emergency department with hospitalization criteria. Primary endpoint was ICU transfer, according to the usual criteria of ICU requirement, described in Table 1, and was kept unchanged throughout the study. All patients were confirmed with SARS-CoV-2 infection by nasopharyngeal swabs. For each patient included, clinical evaluation and computed tomography (CT) scan and biological evaluation were performed. For all patients, baseline characteristics (demographics, treatment, clinical examination, cardiovascular risk factors, and body mass index) and biological data were retrieved from the medical records using a standardized data collection.
Routine Blood Examinations
All samples were collected in ethylenediaminetetraacetic acid (EDTA), sodium heparin, and 0.129 M trisodium citrate tubes (9NC BD Vacutainer©). Routine lab tests included complete blood count, creatinine, C-reactive protein (CRP), and high-sensitivity cardiac troponin (Hs-cTnI, Beckman) on a DXI analyzer (26, 27). Coagulation tests were prothrombin time (PT) ratio, fibrinogen, and soluble fibrin monomer level (STA-Liatest FM® Diagnostica Stago©) explored on a STA-R® Max coagulometer (Stago©) as previously described (28). D-Dimer concentrations were determined using the Vidas D-Dimer assay (BioMérieux©) according to the manufacturer's instructions.
Transthoracic Cardiac Ultrasound and ICU Respiratory Parameters Evaluation
Cardiac ultrasound was performed on 72 additional consecutive COVID-19 patients, in the medicine department (n = 32) and ICU (n = 40). Transthoracic echocardiography (TTE) was performed using commercially available equipment CX50®, S5-1 probe (1–5 MHz; 80 elements) (Philips Medical Systems©, Andover, Massachusetts), according to the guidelines of the American Society of Echocardiography, during the first 24 h after hospital admission (29). At the time of echocardiographic examination, heart rate (HR), systolic, diastolic, and mean blood pressure were recorded. The examination included standard parasternal view to assess the size of the left ventricle (LV) [LV end diastolic diameter, (LVEDD); LV end systolic diameter (LVESD)]. LV ejection fraction was determined using the Simpson's method. Velocity time integral (VTI, cm) of the LV outflow tract and cardiac output were measured on an apical five-chamber view. Using the apical four-chamber view, mitral inflow was recorded by pulse-wave Doppler. We assessed the early diastole (E, cm/s) and the atrial contraction (A, cm/s). Early diastole (e′, cm/s) velocity of the lateral mitral annulus was measured by tissue Doppler imaging. We then calculated the E/A and E/e′ ratios. Using the same view, we carried out an evaluation of the right ventricle (RV): tricuspid annular plane systolic excursion (TAPSE) with TM mode, S wave velocity with tissue Doppler imaging, peak systolic tricuspid insufficiency velocity, and ratio of basal diameters of the right and left ventricle (RV/LV). The trans-tricuspid pressure difference was estimated from the peak velocity of tricuspid regurgitant jet (TR Vmax) by applying the simplified Bernoulli equation. The systolic pulmonary arterial pressure (sPAP) was then derived by adding estimated right atrium pressure based on the inspiratory changes in the dimension of inferior vena cava (30).
We also collected respiratory characteristics [FiO2, positive end-expiratory pressure (PEEP), plateau pressure (Pplat), respiratory system compliance] and hemodynamic parameters [mean arterial pressure (MAP), HR, catecholamine dose] at the time of TTE assessment. The blood gas samples were collected just before the TTE.
Statistical Analysis
Continuous data were expressed as median [interquartile range (IQR)] and categorical data as proportion. Patients were compared according to patients' care pathway divided into three groups: patient hospitalized in the medical department, patients hospitalized in the medical department and then referred to ICU following respiratory worsening, and patients directly admitted to the ICU. In this univariate analysis, continuous variables were compared using Kruskal–Wallis test, and categorical variables were compared using Cochran–Armitage test for trend (multiple group). Patients were also compared according to the Hs-cTnI at admission. In this univariate analysis, we determined the differences in median using the unpaired t-test (Mann–Whitney U test) for continuous variable, and differences in proportions were assessed with the chi-square test or Fisher's exact test if necessary. We generated receiver operating characteristics (ROC) curves with four different regression models that included variables with significant difference in the univariate analysis (31, 32). Models included (i) plasma level of D-dimer (over a cutoff value of 1,000 ng/mL only), (ii) the Hs-cTnI only, (iii) the Hs-cTnI adjusted on gender, the presence of pneumonia on CT scan and the plasma level of D-dimer (over a cutoff value of 1,000 ng/mL), and (iv) the gender, the presence of pneumonia on CT scan, and the plasma level of D-dimer (over a cutoff value of 1,000 ng/mL). The four models helped assess the extent to which the level of D-dimer and Hs-cTnI influenced the predictability of hospitalization in ICU. We calculated the area under the curve (AUC) for the different logistic regression model (32, 33). We used logistic regression to determine whether the level of D-dimer (as a categorical dependent variable dichotomized according to the cutoff of 1,000 ng/mL) and the level of Hs-cTnI (as a categorical dependent variable dichotomized according to the cutoff of 9.75 pg/mL) were associated with the ICU referral (34, 35). The model included only these two variables (D-dimer and Hs-cTnI), and to take into account any potential interaction, we performed the model with and without interaction term. The correlation between biological parameters (Hs-cTnI and D-dimer) at hospital admission and ultrasound characteristics of patients who were hospitalized in ICU with invasive mechanical ventilation was assessed using the Kendall coefficient correlation test. All analyses were two-sided and a p < 0.05 was considered statistically significant. Statistical analysis was performed using R studio software (R© Development Core Team, 2019).
Results
D-Dimer and Hs-cTnI Levels at Hospital Admission Are Discriminant Biomarkers to Predict ICU Referral
The cohort study was composed of 99 consecutive patients who presented to the emergency department and were diagnosed with COVID-19 in March and April 2020. They were divided into three groups: patients hospitalized in the medical department (n = 48), patients first hospitalized in the medicine department (mean of 3.0 ± 1.4 days) then referred to ICU due to respiratory degradation (n = 21), and patients admitted to ICU after admission to the emergency department (n = 30).
These three groups were strictly comparable in terms of age, body mass index, cardiovascular risk factors, treatments, and time from illness onset to hospitalization (Table 2). However, COVID-19 patients directly admitted to ICU had more often history of coronary heart disease and were more likely to have dyspnea at admission (p < 0.001), decreased SpO2 (p < 0.001), pneumonia on the CT scan (p = 0.002), ARDS (p < 0.001), and increased respiratory rate–breath per minute (p < 0.001). In terms of biological features, patients directly requiring ICU admission and those referred to ICU after conventional hospitalization had a significantly higher white blood cell count and granulocytes count (respectively, p = 0.03 and 0.003) with more severe lymphopenia and monocytopenia (respectively, p = 0.002 and 0.005) than patients hospitalized in the medicine department. Regarding coagulation disorders, 70% of COVID-19 patients admitted to ICU had D-dimer level above 1,000 ng/mL at hospital admission (p = 0.0013). The PT ratio was significantly different between groups; however, it remained within normal range. In the whole COVID-19 population, fibrin monomers were negative and associated with hyperfibrinogenemia and without thrombocytopenia. These results were not in favor of a COVID-19-associated disseminated intravascular coagulation (DIC). Lastly, COVID-19 patients admitted to ICU had a significantly higher CRP level (p < 0.001), plasma creatinine (p = 0.002), and Hs-cTnI (p < 0.001). No correlation was found between Hs-cTnI and D-dimer (p = 0.82), Hs-cTnI and creatinine (p = 0.27), or Hs-cTnI and SpO2 (p = 0.13), while a significant association was found between Hs-cTnI and CRP (p = 0.001).
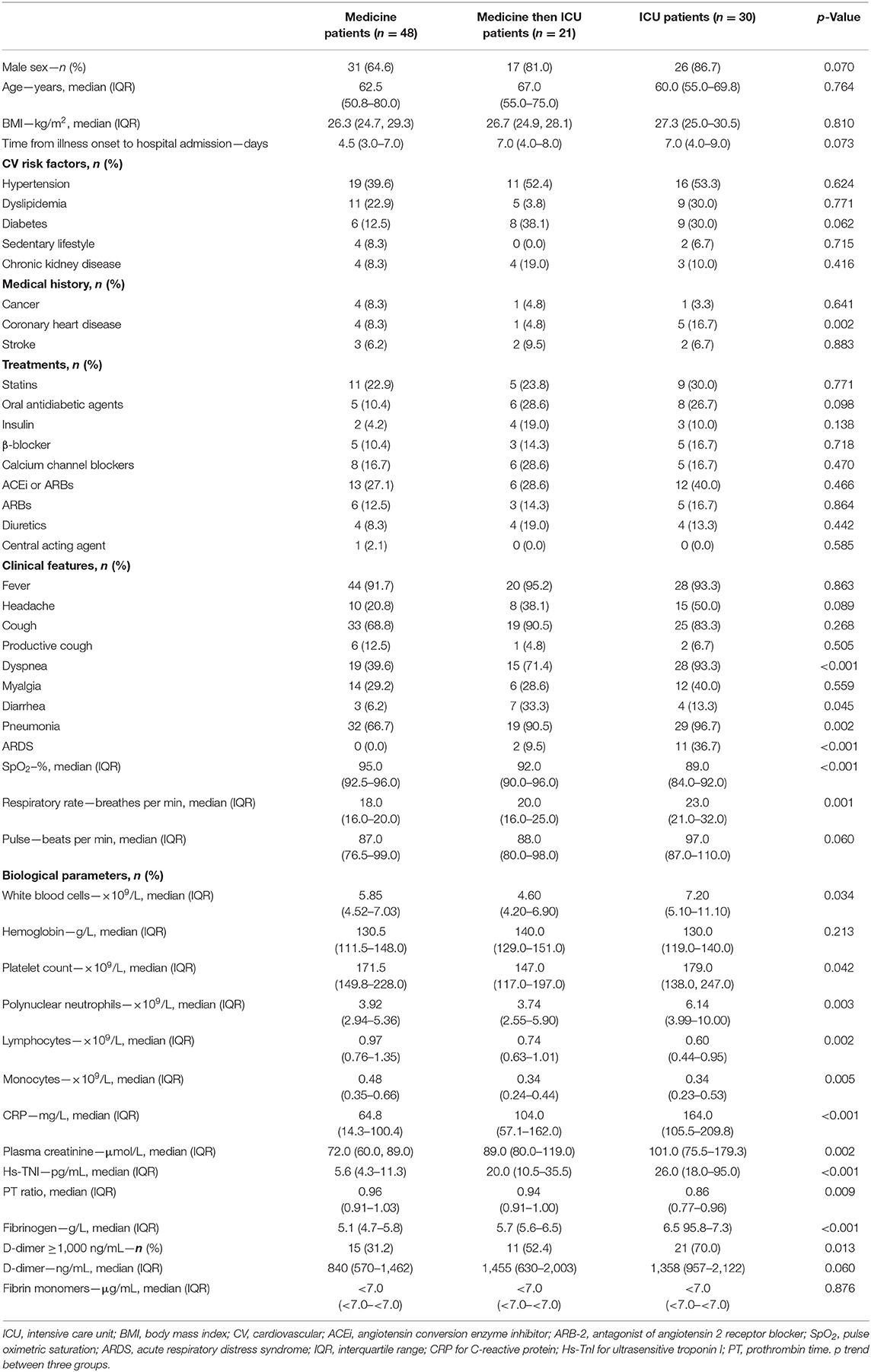
Table 2. Demographic and clinical characteristics of patients at admission according to the addressed department (medical or ICU).
Hs-cTnI Level at Entrance Is the Most Relevant Biomarker to Predict ICU Referral
In the context of coagulopathy and myocardial injury during COVID-19 and given the observation that D-dimer and Hs-cTnI levels at admission were associated with ICU admission, a ROC curve analysis was constructed using these biomarkers (Figure 1). We used different D-dimer level cutoffs (>1,000, >2,000, and >3,000 ng/mL) as potential prognostic criteria for ICU referral. For the cutoff of >1,000 ng/mL, the ROC curve (AUC, 67.2; 95% CI, 51.4–80.2) yielded a sensitivity of 72.4% (95% CI, 50.0–86.0), a specificity of 60.0% (95% CI, 43.0–75.0), a positive predictive value (PPV) of 58.6% (95% CI, 40.0–74.0), and a negative predictive value (NPV) of 74.2% (95% CI, 55.0–87.0%, Table 3). None of the other cutoffs had better prognostic values (Table 3). The D-dimer cutoff of 1,000 ng/mL as a predictive value for ICU referral was improved in a ROC curve analysis, when associated with gender and pneumonia at CT scan (AUC, 79.1; 95% CI, 68.5–89.7, p = 0.04). The addition of Hs-cTnI to this model allowed reaching an AUC of 84.9 (95% CI, 73.9–95.9, p = 0.03). Furthermore, Hs-cTnI alone was the best predictor for ICU outcome with AUC of 86.4 (95% CI, 76.6–96.2). ROC curve identified a cutoff at 9.75 pg/mL yielding a high sensitivity of 89.6% (95% CI, 71.0–97.0), a good specificity of 72.4% (95% CI, 52.0–86.0), a high PPV of 76.5% (95% CI, 58.0–88.0), and a high NPV of 87.5% (95% CI, 66.0–96.0). None of the other Hs-cTnI cutoffs currently used for acute myocardial infarction [11.6 and 19.8 pg/mL, respectively, for men and women; cutoff detection for acute myocardial injury defined as an elevated Hs-cTnI value above the 99th percentile upper reference limit (27)] had a prognostic value better than 9.75 pg/mL (Table 4).
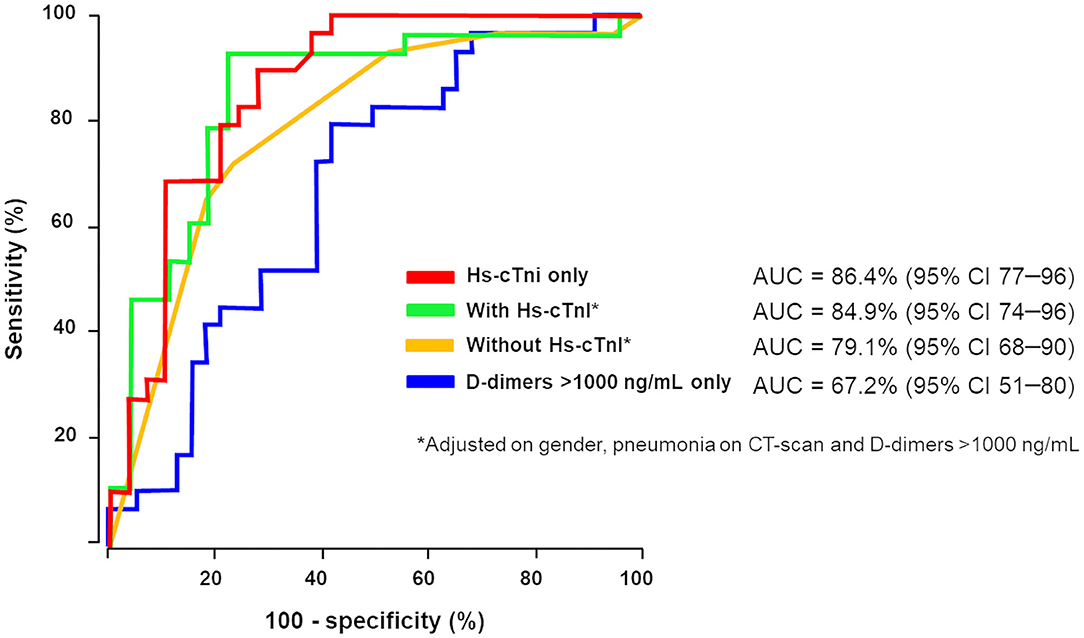
Figure 1. D-Dimer and high-sensitivity cardiac troponin (Hs-cTnI) involvement in intensive care unit (ICU) referral. We used different D-dimer level cutoffs (>1,000 ng/mL, >2,000 ng/mL, >3,000 ng/mL) as potential prognostic criteria for ICU referral. Receiver operating characteristics (ROC) curve analysis associating D-dimer above 1,000 ng/mL, gender and pneumonia at CT scan for ICU transfer (in yellow) increases area under the curve (AUC) in contrast to D-dimer ≥1,000 ng/mL alone (AUC, 79.1; 95% CI, 68–90, p = 0.04). Addition of Hs-cTnI to this model (in green) allowed reaching AUC of 84.9 (95% CI, 74–96, p = 0.03). Hs-cTnI alone was the best predictive ROC curve (in red) for ICU outcome with AUC of 86.4 (95% CI, 77–96).
Table 5 confirms the link between Hs-cTnI and ICU referral using a logistic regression model with the cutoff of 9.75 pg/mL for Hs-cTnI (OR, 22.8; 95% CI, 6.0–116.2, p < 0.001). Strikingly, when adjusted to D-dimer level, the adjusted OR of 20.85 (95% CI, 4.76–128.4, p < 0.001) was not better. Inversely, when a logistic regression model used the cutoff of 1,000 ng/mL for D-dimer, the association between D-dimer and ICU outcome (OR, 4.02; 95% CI, 1.46–11.93, p = 0.009) did not remain significant when adjusted to Hs-cTnI (OR, 1.34; 95% CI, 0.3–5.9, p = 0.7), confirming the higher specificity of Hs-cTnI in predicting ICU referral in hospitalized patients. The addition of the interaction term between D-dimer and Hs-cTnI did not change the associations observed. To confirm the relevance of this proposed Hs-cTnI cutoff of 9.75 pg/mL, we compared clinical and biological results in the study population and found that patients with Hs-cTnI above 9.75 pg/mL were older (p = 0.01) and more likely to get a β-blocker prescription (p = 0.034), angiotensin-converting enzyme inhibitors (ACEi) or angiotensin receptor blockers (ARBs) therapy (respectively, p = 0.005 for ACEi or ARBs and p = 0.021 for ARBs alone), or a history of coronary heart disease (p = 0.016) (Supplementary Table 3). Lastly, we evaluated the link between Hs-cTnI and mortality among the same patients. The ROC curve identified a cutoff at 10.75 pg/mL yielding a sensitivity of 42.6% (95% CI, 29.5–56.8) and a specificity of 100% (95% CI, 80.7–100). We will confirm the relevance of an Hs-cTnI threshold of 9.75 pg/mL in a prospective study.
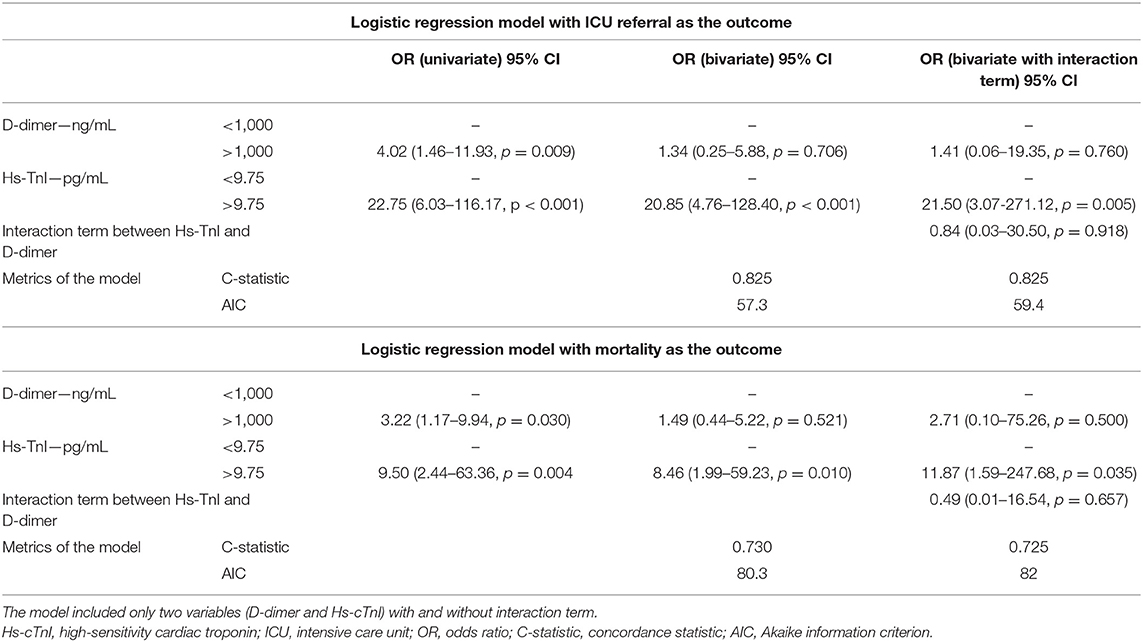
Table 5. Logistic regression model evaluating D-dimer >1,000 ng/mL and high-sensitivity troponin level in ICU referral and mortality.
D-Dimer and Hs-cTnI Levels Are Related to Vascular Obstruction and Increased Right Ventricular Afterload on Transthoracic Cardiac Ultrasound Evaluation
We explored the cardiac function by TTE of 72 consecutive patients tested positive for COVID-19, admitted in April 2020, 32 (44%) patients in the medicine department, and 40 (56%) patients in ICU. Demographic data and respiratory function are reported in Supplementary Tables 1, 2. Regarding patients in ICU, at the time of TTE assessment, median PEEP was 13 cmH2O (5–16), median Pplat was 26 cmH2O (19–28), median respiratory system compliance was 36.30 (mL/cmH2O) (31.1–45.9), and FiO2 50% (44–100). Regarding hemodynamic parameters median MAP was 70 mmHg (60–78), median HR was 105/min (85–116), while median epinephrine dose was 0.0 mg/h (0.0–0.9). Most patients had respiratory acidosis with median pH 7.28 (7.20–7.34), median PaCO2 of 53 mmHg (42–59), median PaO2 of 85 mmHg (68–100), and median lactate of 1.45 mmol/L (1.1–2.0).
As described in Supplementary Table 4, we found no change in the LV ultrasound parameters. No significant abnormalities in the LV geometry [LVEDD at 44.0 (41.9–50) mm or LVESD at 31.0 (26.4–37) mm], LV ejection fraction [60 (55–65)%], or LV filling pressures [E/A ratio at 0.9 (0.7–1.4)], with a median E wave at 75 (59–85.2) cm/s and E/lateral e′ ratio at 6.2 (4.9–8.3), were indeed observed. Regarding the relationship between biological parameters at hospital admission and ultrasound characteristics, Hs-cTnI showed a significant but weak correlation with the LV ejection fraction (r = −0.196, p = 0.039) and no correlation with the E/e′ ratio (p = 0.157). D-Dimer did not correlate with these parameters. We did not find any acute systolic dysfunction associated with COVID-19. Hs-cTnI elevation corresponded rather to a higher rise in heart disease with underlying low LVEF.
Concerning the RV, we found an initial dilatation of the RV diameter [median diameter of 37.8 (33.0–43.3) mm], with an RV/LV ratio of 0.8 (0.7–0.9), without dilatation of the LV. RV function was maintained [s′ tissue Doppler imaging (TDI) at 13.0 (11.5–16.0) cm/s; tricuspid annular plane systolic excursion (TAPSE) at 21.0 (17.8–23.6) mm]; the systolic pulmonary arterial pressure (sPAP) value remained above normal but without major elevation [31.6 (25.1–43.6) mmHg]. Regarding the ultrasound parameters of the RV, the strongest correlation was between Hs-cTnI level and sPAP (r = 0.42; p = 0.01, Table 6). Hs-cTnI was also correlated with an RV systolic dysfunction (correlation with TAPSE, r = −0.24; p = −0.007) but not with an RV dilatation (RV/LV ratio, p = −0.765). D-Dimer levels showed significant correlations with the same parameters, sPAP (r = 0.18, p = 0.046), TAPSE (r = 0.18, p = 0.035), and with the dilatation of the RV (r = 0.23, p = 0.012).
Discussion
In this prospective single-center study, we reported that Hs-cTnI level at admission was the best biomarker to predict ICU transfer and respiratory severity in COVID-19 patients. Moreover, we evidenced the D-dimer involvement in the pathophysiology of COVID-19 and the correlation with a RV afterload, which allows us to confirm pulmonary vascular obstruction as a site of coagulopathy and a source of circulating D-dimer.
D-dimer increase has been widely reported during SARS-CoV-2 infection (2, 17, 36–38). It has been first associated with sepsis-induced coagulopathy and with DIC (37, 39). However, beside a high D-dimer level, the present study evidenced a low level of fibrin monomers, a high fibrinogen level, and no signs of thrombocytopenia, allowing us to exclude a DIC at admission. D-dimer may reflect the consequences of the COVID-19-associated coagulopathy (40, 41), as it probably participates in the respiratory disease through the development of capillary microthrombosis as observed in postmortem studies (42) and attributed to a vascular thickening or vascular congestion (14, 43–45). Moreover, another pulmonary vascular issue in COVID-19 is related to a high incidence of pulmonary embolism (PE) (46, 47) whose exact association still needs to be determined. However, our results show a low prognostic value of D-dimer levels on ICU referral when taken alone. In the same line, this finding could be similar to the use of D-dimer in PE, which has a low predictive performance when using absolute values and needs an age-adjusted cutoff. Interestingly, we previously observed that the of D-dimer level <500 ng/mL associated with female gender and absence of pneumonia at CT scan as potential exclusion criteria for COVID-19 diagnosis (17). Increase in D-dimer level and its evolution is probably a better reflect of COVID-19-associated coagulopathy involvement and progression that might help to choose between a prophylactic or a therapeutic anticoagulation strategy. This hypothesis needs to be confirmed in ongoing prospective randomized clinical trials.
The major finding of the present study is the excellent prognostic value of Hs-cTnI level to predict respiratory worsening, ICU referral, and mortality. It is important to note that the proposed Hs-cTnI cutoff is lower than the thresholds used for myocarditis or myocardial infarction diagnosis (48). The observed elevation of Hs-cTnI may probably be more resulting from microcirculatory damage and myocardial oxygen supply–demand mismatch and supply than a primary pathology of the myocardium, as it was already observed in the case of community-acquired pneumonia (21, 49). In parallel with myocardial inflammation due to SARS-CoV-2 infection, myocardial damage could result from a RV wall tension due to an increased RV afterload with RV dilation and tricuspid valve insufficiency. Yet, the mechanism of troponin release from the myocardium is not fully understood, and various pathophysiological scenarios have been proposed (50). However, the higher the troponin, the higher the risk of ICU referral (51, 52), with no altered LV function probably underlining the burden of endotheliitis and microthrombotic processes in the outcome of patients with COVID-19.
In the present study, Hs-cTnI was clearly correlated with sPAP values as well as with RV longitudinal systolic dysfunction. These results suggest that an increase in pulmonary pressures secondary to capillary microthrombosis may be responsible for right ventricular myocardial distress, which leads to troponin release. This hypothesis is also supported by the results of Szekely et al. showing RV dysfunction at the forefront of cardiac damage associated with COVID-19 and correlated with troponin levels (53). Although troponin was the best prognostic marker of patient worsening, D-dimer levels also have a prognostic role, appearing to correspond to a causal process of pulmonary microthrombosis. Thus, we suggest here a kinetic study of cardiopulmonary-induced lesion in COVID-19 as proposed in Figure 2. Pulmonary endothelium forms a key part of the alveolar–capillary unit, providing an interface for efficient gas exchange between the alveolar space and blood cells within lung capillaries. We previously described an early endothelial lesion that drives prognosis and ICU transfer of patients. This thrombo-inflammatory process in pulmonary vessels is probably the main actor of microthrombosis in lung capillaries (reflected by increased D-dimer) driving consequences in right ventricle. Thus, troponin increase is mainly reflecting RV afterload increase.
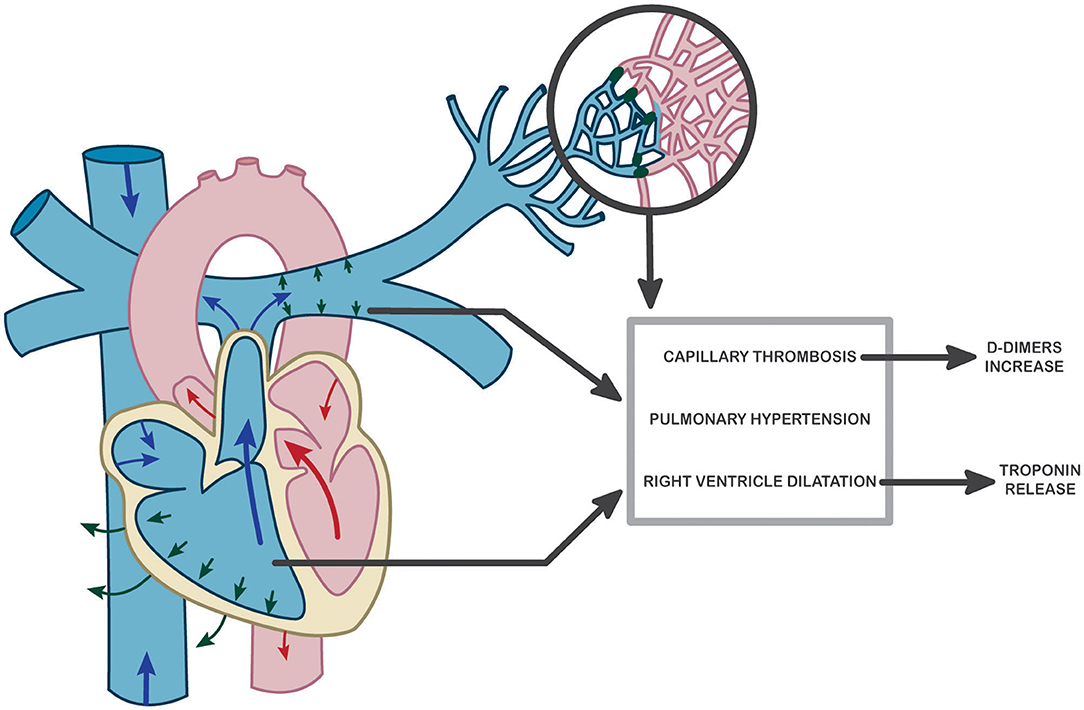
Figure 2. Hypothesis of a potential pathophysiological mechanism explaining pulmonary and cardiac dysfunction in COVID-19 and resulting in troponin and D-dimer increase at admission to the hospital.
Limitations
This is a pilot study on the search for prognostic biomarkers of COVID-19, explaining this single-center work on a limited number of patients. We also do not present troponin kinetics, which were not systematically performed. A longer follow-up will be required to firmly define prognostic biomarkers of COVID-19 severity, and our results should be replicated in a multicenter study. Due to the small simple size and to respect the assumptions required to perform logistic regression, we were authorized to include in the model only these two variables (Hs-cTnI and D-dimer). For this reason, the influence of parameters such as gender or prior anticoagulant treatment (only three patients were treated for atrial fibrillation) could not be reasonably studied in this work. Thus, taking into account all of these limitations, the pathophysiological link between right ventricular afterload, cardiac troponin release, and COVID-19 is first and foremost a hypothesis requiring broader validation.
Despite these limitations, we believe that Hs-cTnI provides important information on the severity of COVID-19, like in pulmonary embolism, even with a cutoff value below the threshold usually used for acute coronary syndrome diagnosis (11.6 pg/mL for women and 19.8 pg/mL for men). Therefore, with the condition that each center adjusts its cutoff according to the intervariability of the method, Hs-cTnI could be considered as a relevant surrogate marker to avoid any delay in COVID-19 patient care and referral to ICU. Despite these limitations, we believe that, like in pulmonary embolism, Hs-cTnI, even at a low value below the threshold usually used (11.6 pg/mL for women and 19.8 pg/mL for men), provides important information on the severity of COVID-19.
In conclusion, it seems consistent to open the way for biomarkers in cardiovascular complications and coagulation disorders in COVID-19 patients at admission to the hospital. Further prospective studies should not only evaluate Hs-cTnI and D-dimer levels utility to predict admission to ICU but also evaluate their prognostic value during follow-up and their relevance in respiratory and thrombotic related disorders.
Data Availability Statement
The raw data supporting the conclusions of this article will be made available by the authors, without undue reservation.
Ethics Statement
The studies involving human participants were reviewed and approved by Comité de protection des personnes. The patients/participants provided their written informed consent to participate in this study.
Author Contributions
DS, TM, and J-LD conceived and supervised the study. GG, J-LA, LK, NG, and BD monitored and analyzed the data. RC analyzed the data and supervised statistical analysis. All authors interpreted the data, drafted and revised the manuscript, and approved the final version.
Funding
This work was funded by grants from the French National Research Agency and the Fondation de France ANR SARCODO.
Conflict of Interest
The authors declare that the research was conducted in the absence of any commercial or financial relationships that could be construed as a potential conflict of interest.
Acknowledgments
We would like to acknowledge all nurses, technicians, and physicians involved in the Vascular Medicine, Respiratory Medicine, Intensive Care, and Hematology Departments at the Georges Pompidou European Hospital for their help in taking care of the patients and including them in the study. We thank the AP-HP for promoting the project SARCODO. We thank the unit of clinical research URC HEGP CIC-EC1418 (Natacha Nohile, Pauline Jouany, and Dr. Juliette Djadi-Prat) and Helene Cart-Grandjean from AP-HP for their involvement with the project SARCODO.
Supplementary Material
The Supplementary Material for this article can be found online at: https://www.frontiersin.org/articles/10.3389/fmed.2020.586307/full#supplementary-material
References
1. D'Amico F, Baumgart DC, Danese S, Peyrin-Biroulet L. Diarrhea during COVID-19 infection: pathogenesis, epidemiology, prevention and management. Clin Gastroenterol Hepatol. (2020) 18:1663–72. doi: 10.1016/j.cgh.2020.04.001
2. Zhou F, Yu T, Du R, Fan G, Liu Y, Liu Z, et al. Clinical course and risk factors for mortality of adult inpatients with COVID-19 in Wuhan, China: a retrospective cohort study. Lancet. (2020) 395:1054–62. doi: 10.1016/S0140-6736(20)30566-3
3. Zheng YY, Ma YT, Zhang JY, Xie X. COVID-19 and the cardiovascular system. Nat Rev Cardiol. (2020) 17:259–60. doi: 10.1038/s41569-020-0360-5
4. Liu J, Zhang S, Wu Z, Shang Y, Dong X, Li G, et al. Clinical outcomes of COVID-19 in Wuhan, China: a large cohort study. Ann Intensive Care. (2020) 10:99. doi: 10.1186/s13613-020-00706-3
5. Thachil J, Tang N, Gando S, Falanga A, Cattaneo M, Levi M, et al. ISTH interim guidance on recognition and management of coagulopathy in COVID-19. J Thromb Haemost. (2020) 18:1023–6. doi: 10.1111/jth.14810
6. Zhang L, Yan X, Fan Q, Liu H, Liu X, Liu Z, et al. D-dimer levels on admission to predict in-hospital mortality in patients with Covid-19. J Thromb Haemost. (2020) 18:1324–9. doi: 10.1111/jth.14859
7. Bösmüller H, Traxler S, Bitzer M, Häberle H, Raiser W, Nann D, et al. The evolution of pulmonary pathology in fatal COVID-19 disease: an autopsy study with clinical correlation. Virchows Arch. (2020) 477:349–57. doi: 10.1007/s00428-020-02881-x
8. Cheng Y, Luo R, Wang K, Zhang M, Wang Z, Dong L, et al. Kidney disease is associated with in-hospital death of patients with COVID-19. Kidney Int. (2020) 97:829–38. doi: 10.1016/j.kint.2020.03.005
9. Zhang H, Zhou P, Wei Y, Yue H, Wang Y, Hu M, et al. Histopathologic changes and SARS-COV-2 immunostaining in the lung of a patient with coviD-19. Ann Intern Med. (2020) 172:629–32. doi: 10.7326/M20-0533
10. Ciceri F, Beretta L, Scandroglio AM, Colombo S, Landoni G, Ruggeri A, et al. Microvascular COVID-19 lung vessels obstructive thromboinflammatory syndrome (MicroCLOTS): an atypical acute respiratory distress syndrome working hypothesis. Crit Care Resusc. (2020) 22:95–7.
11. Magro C, Mulvey JJ, Berlin D, Nuovo G, Salvatore S, Harp J, et al. Complement associated microvascular injury and thrombosis in the pathogenesis of severe COVID-19 infection: a report of five cases. Transl Res. (2020) 220:1–13. doi: 10.1016/j.trsl.2020.04.007
12. McGonagle D, O'Donnell JS, Sharif K, Emery P, Bridgewood C. Immune mechanisms of pulmonary intravascular coagulopathy in COVID-19 pneumonia. Lancet Rheumatol. (2020) 2:e460–1. doi: 10.1016/S2665-9913(20)30121-1
13. Monteil V, Kwon H, Prado P, Hagelkrüys A, Wimmer RA, Stahl M, et al. Inhibition of SARS-CoV-2 infections in engineered human tissues using clinical-grade soluble human ACE2. Cell. (2020) 181:905–913.e7. doi: 10.1016/j.cell.2020.04.004
14. Qanadli SD, Beigelman-Aubry C, Rotzinger DC. Vascular changes detected with thoracic CT in coronavirus disease (COVID-19) might be significant determinants for accurate diagnosis and optimal patient management. AJR Am J Roentgenol. (2020) 215:W15. doi: 10.2214/AJR.20.23185
15. Varga Z, Flammer AJ, Steiger P, Haberecker M, Andermatt R, Zinkernagel AS, et al. Endothelial cell infection and endotheliitis in COVID-19. Lancet. (2020) 395:1417–8. doi: 10.1016/S0140-6736(20)30937-5
16. Ferrario CM, Jessup J, Chappell MC, Averill DB, Brosnihan KB, Tallant EA, et al. Effect of angiotensin-converting enzyme inhibition and angiotensin II receptor blockers on cardiac angiotensin-converting enzyme 2. Circulation. (2005) 111:2605–10. doi: 10.1161/CIRCULATIONAHA.104.510461
17. Khider L, Gendron N, Goudot G, Chocron R, Hauw-Berlemont C, Cheng C, et al. Curative anticoagulation prevents endothelial lesion in COVID-19 patients. J Thromb Haemost. (2020). doi: 10.1111/jth.14968. [Epub ahead of print].
18. Mauri T, Spinelli E, Scotti E, Colussi G, Basile MC, Crotti S, et al. Potential for lung recruitment and ventilation-perfusion mismatch in patients with the acute respiratory distress syndrome from coronavirus disease 2019. Crit Care Med. (2020) 48:1129–34. doi: 10.1097/CCM.00000000000043861
19. Smadja DM, Guerin CL, Chocron R, Yatim N, Boussier J, Gendron N, et al. Angiopoietin-2 as a marker of endothelial activation is a good predictor factor for intensive care unit admission of COVID-19 patients. Angiogenesis. (2020) 23:611–20. doi: 10.1007/s10456-020-09730-0
20. Chapman AR, Bularga A, Mills NL. High-sensitivity cardiac troponin can be an ally in the fight against COVID-19. Circulation. (2020) 141: 1733–5. doi: 10.1161/circulationaha.120.047008
21. Frencken JF, van Baal L, Kappen TH, Donker DW, Horn J, van der Poll T, et al. Myocardial injury in critically ill patients with community-acquired pneumonia a cohort study. Ann Am Thorac Soc. (2019) 16:606–12. doi: 10.1513/AnnalsATS.201804-286OC
22. Raad M, Dabbagh M, Gorgis S, Yan J, Chehab O, Dagher C, et al. Cardiac injury patterns and inpatient outcomes among patients admitted with COVID-19. Am J Cardiol. (2020) 133:154–61. doi: 10.1016/j.amjcard.2020.07.040
23. Stefanini GG, Chiarito M, Ferrante G, Cannata F, Azzolini E, Viggiani G, et al. Early detection of elevated cardiac biomarkers to optimise risk stratification in patients with COVID-19. Heart. (2020) 106:1512–8. doi: 10.1136/heartjnl-2020-317322
24. Toraih EA, Elshazli RM, Hussein MH, Elgaml A, Amin MN, El-Mowafy M, et al. Association of cardiac biomarkers and comorbidities with increased mortality, severity, and cardiac injury in COVID-19 patients: a meta-regression and decision tree analysis. J Med Virol. (2020) 92:2473–88. doi: 10.1002/jmv.26166
25. Qin C, Zhou L, Hu Z, Zhang S, Yang S, Tao Y, et al. Dysregulation of immune response in patients with COVID-19 in Wuhan, China. Clin Infect Dis. (2020) 71:762–8. doi: 10.1093/cid/ciaa248
26. Apple FS, Ler R, Murakami MAM. Determination of 19 cardiac troponin I and T assay 99th percentile values from a common presumably healthy population. Clin Chem. (2012) 58:1574–81. doi: 10.1373/clinchem.2012.192716
27. Thygesen K, Alpert JS, Jaffe AS, Chaitman BR, Bax JJ, Morrow DA, et al. Fourth universal definition of myocardial infarction (2018). Circulation. (2018) 138:e618–51. doi: 10.1161/CIR.0000000000000617
28. Latrémouille C, Carpentier A, Leprince P, Roussel JC, Cholley B, Boissier E, et al. A bioprosthetic total artificial heart for end-stage heart failure: results from a pilot study. J Hear Lung Transplant. (2018) 37:33–7. doi: 10.1016/j.healun.2017.09.002
29. Rudski LG, Lai WW, Afilalo J, Hua L, Handschumacher MD, Chandrasekaran K, et al. Guidelines for the echocardiographic assessment of the right heart in adults: a report from the American Society of Echocardiography endorsed by the European Association of Echocardiography, a registered branch of the European Society of Cardiology. J Am Soc Echocardiogr. (2010) 23:685–713. doi: 10.1016/j.echo.2010.05.010
30. Galiè N, Humbert M, Vachiery JL, Gibbs S, Lang I, Torbicki A, et al. 2015 ESC/ERS guidelines for the diagnosis and treatment of pulmonary hypertension. Eur Heart J. (2016) 37:67–119. doi: 10.1093/eurheartj/ehv317
31. Hajian-Tilaki K. Receiver operating characteristic (ROC) curve analysis for medical diagnostic test evaluation. Casp J Intern Med. (2013) 4:627–35.
32. Park SH, Goo JM, Jo CH. Receiver operating characteristic (ROC) curve: practical review for radiologists. Korean J Radiol. (2004) 5:11–8. doi: 10.3348/kjr.2004.5.1.11
33. DeLong ER, DeLong DM, Clarke-Pearson DL. Comparing the areas under two or more correlated receiver operating characteristic curves: a nonparametric approach. Biometrics. (1988) 44:837. doi: 10.2307/2531595
34. Reichenheim ME, Coutinho ES. Measures and models for causal inference in cross-sectional studies: arguments for the appropriateness of the prevalence odds ratio and related logistic regression. BMC Med Res Methodol. (2010) 10:66. doi: 10.1186/1471-2288-10-66
35. Sedgwick P. Bias in observational study designs: cross sectional studies. BMJ. (2015) 350: h1286. doi: 10.1136/bmj.h1286
36. Han H, Yang L, Liu R, Liu F, Wu KL, Li J, et al. Prominent changes in blood coagulation of patients with SARS-CoV-2 infection. Clin Chem Lab Med. (2020) 58:1116–20. doi: 10.1515/cclm-2020-0188
37. Tang N, Li D, Wang X, Sun Z. Abnormal coagulation parameters are associated with poor prognosis in patients with novel coronavirus pneumonia. J Thromb Haemost. (2020) 18:844–7. doi: 10.1111/jth.14768
38. Yin S, Huang M, Li D, Tang N. Difference of coagulation features between severe pneumonia induced by SARS-CoV2 and non-SARS-CoV2. J Thromb Thrombolysis. (2020). doi: 10.1007/s11239-020-02105-8. [Epub ahead of print].
39. Tang N, Bai H, Chen X, Gong J, Li D, Sun Z. Anticoagulant treatment is associated with decreased mortality in severe coronavirus disease 2019 patients with coagulopathy. J Thromb Haemost. (2020) 18:1094–9. doi: 10.1111/jth.14817
40. Helms J, Severac F, Merdji H, Anglés-Cano E, Meziani F. Prothrombotic phenotype in COVID-19 severe patients. Intensive Care Med. (2020) 1–2. doi: 10.1007/s00134-020-06082-7
41. Klok FA, Kruip MJHA, van der Meer NJM, Arbous MS, Gommers DAMPJ, Kant KM, et al. Incidence of thrombotic complications in critically ill ICU patients with COVID-19. Thromb Res. (2020) 191:145–7. doi: 10.1016/j.thromres.2020.04.013
42. Xie X, Zhong Z, Zhao W, Zheng C, Wang F, Liu J. Chest CT for typical 2019-nCoV pneumonia: relationship to negative RT-PCR testing. Radiology. (2020) 296:E41–5. doi: 10.1148/radiol.2020200343
43. Klok FA, Kruip MJHA, van der Meer NJM, Arbous MS, Gommers D, Kant KM, et al. Confirmation of the high cumulative incidence of thrombotic complications in critically ill ICU patients with COVID-19: An updated analysis. Thromb Res. (2020) 191:148–50. doi: 10.1016/j.thromres.2020.04.041
44. Middeldorp S, Coppens M, van Haaps TF, Foppen M, Vlaar AP, Müller MCA, et al. Incidence of venous thromboembolism in hospitalized patients with COVID-19. J Thromb Haemost. (2020) 18:1995–2002. doi: 10.1111/jth.14888
45. Wang J, Hajizadeh N, Moore EE, McIntyre RC, Moore PK, Veress LA, et al. Tissue plasminogen activator (tPA) treatment for COVID-19 associated acute respiratory distress syndrome (ARDS): a case series. J Thromb Haemost. (2020) 18:1752–5. doi: 10.1111/jth.14828
46. Danzi GB, Loffi M, Galeazzi G, Gherbesi E. Acute pulmonary embolism and COVID-19 pneumonia: a random association? Eur Heart J. (2020) 41:1858. doi: 10.1093/eurheartj/ehaa254
47. Lodigiani C, Iapichino G, Carenzo L, Cecconi M, Ferrazzi P, Sebastian T, et al. Venous and arterial thromboembolic complications in COVID-19 patients admitted to an academic hospital in Milan, Italy. Thromb Res. (2020) 191:9–14. doi: 10.1016/j.thromres.2020.04.024
48. Zeng J-HH, Liu Y-XX, Yuan J, Wang F-XX, Wu W-BB, Li J-XX, et al. First case of COVID-19 complicated with fulminant myocarditis: a case report and insights. Infection. (2020) 48:773–7. doi: 10.1007/s15010-020-01424-5
49. Moammar MQ, Ali MI, Mahmood NA, DeBari VA, Khan MA. Cardiac troponin I levels and alveolar-arterial oxygen gradient in patients with community-acquired pneumonia. Hear Lung Circ. (2010) 19:90–2. doi: 10.1016/j.hlc.2009.08.009
50. Takasu O, Gaut JP, Watanabe E, To K, Fagley RE, Sato B, et al. Mechanisms of cardiac and renal dysfunction in patients dying of sepsis. Am J Respir Crit Care Med. (2013) 187:509–17. doi: 10.1164/rccm.201211-1983OC
51. Lala A, Johnson KW, Januzzi JL, Russak AJ, Paranjpe I, Richter F, et al. Prevalence and impact of myocardial injury in patients hospitalized with COVID-19 infection. J Am Coll Cardiol. (2020) 76:533–46. doi: 10.1016/j.jacc.2020.06.007
52. Lippi G, Lavie CJ, Sanchis-Gomar F. Cardiac troponin I in patients with coronavirus disease 2019 (COVID-19): evidence from a meta-analysis. Prog Cardiovasc Dis. (2020) 63:390–1. doi: 10.1016/j.pcad.2020.03.001
Keywords: COVID-19, troponin, D-dimer (DD), echocardiograghy, thrombosis, right ventricle
Citation: Goudot G, Chocron R, Augy J-L, Gendron N, Khider L, Debuc B, Aissaoui N, Peron N, Hauw-Berlemont C, Vedie B, Cheng C, Mohamedi N, Krzisch D, Philippe A, Puscas T, Hermann B, Brichet J, Juvin P, Planquette B, Messas E, Pere H, Veyer D, Gaussem P, Sanchez O, Diehl J-L, Mirault T and Smadja DM (2020) Predictive Factor for COVID-19 Worsening: Insights for High-Sensitivity Troponin and D-Dimer and Correlation With Right Ventricular Afterload. Front. Med. 7:586307. doi: 10.3389/fmed.2020.586307
Received: 22 July 2020; Accepted: 29 September 2020;
Published: 12 November 2020.
Edited by:
Alessandra Bura Riviere, Centre Hospitalier Universitaire de Toulouse, FranceReviewed by:
Wei Li, Marshall University, United StatesLong-Sheng Lu, Taipei Medical University, Taiwan
Copyright © 2020 Goudot, Chocron, Augy, Gendron, Khider, Debuc, Aissaoui, Peron, Hauw-Berlemont, Vedie, Cheng, Mohamedi, Krzisch, Philippe, Puscas, Hermann, Brichet, Juvin, Planquette, Messas, Pere, Veyer, Gaussem, Sanchez, Diehl, Mirault and Smadja. This is an open-access article distributed under the terms of the Creative Commons Attribution License (CC BY). The use, distribution or reproduction in other forums is permitted, provided the original author(s) and the copyright owner(s) are credited and that the original publication in this journal is cited, in accordance with accepted academic practice. No use, distribution or reproduction is permitted which does not comply with these terms.
*Correspondence: David M. Smadja, ZGF2aWQuc21hZGphQGFwaHAuZnI=; orcid.org/0000-0001-7731-9202
†These authors have contributed equally to this work