- 1Department of Pediatrics, University of British Columbia, Vancouver, BC, Canada
- 2Istituti Clinici Scientifici Maugeri, Istituto di Ricerca e Cura a Carattere Scientifico, Tradate, Italy
- 3Faculty of Medicine, Institute of Biomedical Sciences and Institute of Immunology and Immunotherapy, University of Chile, Santiago, Chile
- 4Vanderbilt University Medical Center, Nashville, TN, United States
- 5Respiratory and Intensive Care Unit, Hospital Clinic of Barcelona, University of Barcelona, Barcelona, Spain
- 6Faculty of Medicine and Health, School of Pharmacy, University of Sydney, Sydney, NSW, Australia
- 7Westmead Hospital, Sydney, NSW, Australia
- 8Marie Bashir Institute of Infectious Diseases and Biosecurity, University of Sydney, Sydney, NSW, Australia
- 9Department of Clinical Pharmacy and Pharmacology, University Medical Center Groningen, University of Groningen, Groningen, Netherlands
- 10Public Health Consulting Group, Lugano, Switzerland
- 11University of Tasmania, Monash University, RMIT University, Hobart, Australia
- 12Queen Mary Hospital, Hong Kong, China
- 13Geriatric Clinic Unit, Department of Medicine and Surgery, University-Hospital of Parma, University of Parma, Parma, Italy
- 14Hong Kong Tuberculosis, Chest and Heart Diseases Association, Hong Kong, China
- 15Faculty of Medicine and Health Sciences, Vaccine and Infectious Diseases Institute, University of Antwerp, Antwerp, Belgium
- 16St. Mary's Hospital, London, United Kingdom
- 17Data Science Institute, Hasselt University, Hasselt, Belgium
- 18Centre for Health Economic Research and Modelling Infectious Diseases, Vaccine and Infectious Disease Institute, University of Antwerp, Antwerp, Belgium
- 19Universitair Medisch Centrum Groningen, Groningen, Netherlands
- 20University of Veterinary Medicine, Hanover, Germany
- 21Department of Infectious Diseases, Galliera Hospital, Genoa, Italy
- 22Università degli Studi di Milano, Milan, Italy
- 23Universidade Federal do Rio Grande do Sul (UFRGS), Porto Alegre, Brazil
- 24Department of Internal Medicine (Infectious Diseases), Yale School of Medicine, New Haven, CT, United States
- 25Department of Epidemiology of Microbial Diseases, Yale School of Public Health, New Haven, CT, United States
- 26Radiology Unit, Department of Medicine and Surgery, University of Parma, Parma, Italy
- 27Feinberg School of Medicine, Ann & Robert H. Lurie Children's Hospital of Chicago, Northwestern University, Evanston, IL, United States
- 28Department of Pediatrics and Pediatric Surgery, Faculty of Medicine, Dr. Luis Calvo Mackenna Hospital, University of Chile, Santiago, Chile
- 29Pediatric Clinic, Department of Medicine and Surgery, Pietro Barilla Children's Hospital, University of Parma, Parma, Italy
Coronavirus disease 2019 (COVID-19) is a rapidly evolving, highly transmissible, and potentially lethal pandemic caused by a novel coronavirus, severe acute respiratory syndrome coronavirus 2 (SARS-CoV-2). As of June 11 2020, more than 7,000,000 COVID-19 cases have been reported worldwide, and more than 400,000 patients have died, affecting at least 188 countries. While literature on the disease is rapidly accumulating, an integrated, multinational perspective on clinical manifestations, immunological effects, diagnosis, prevention, and treatment of COVID-19 can be of global benefit. We aimed to synthesize the most relevant literature and experiences in different parts of the world through our global consortium of experts to provide a consensus-based document at this early stage of the pandemic.
Introduction
In December 2019, a cluster of pneumonia cases of an unknown cause was reported in Wuhan city, the capital of Hubei province in China (1). The novel coronavirus, subsequently named severe acute respiratory syndrome coronavirus 2 (SARS-CoV-2) was identified via deep sequencing of patients' respiratory tract samples (2); the disease was designated in February 2020 by the World Health Organization (WHO) as coronavirus disease 2019 (COVID-19) (3). The original cluster of cases was linked to a seafood market with presumed zoonotic transmission, followed by efficient person-to-person transmission (4). Since the initial reports, COVID-19 has rapidly spread from Wuhan to the rest of the world with cases and fatalities increasing rapidly. The WHO declared COVID-19 as a pandemic on March 11 2020.
CoVs are large enveloped non-segmented positive-sense single-stranded RNA viruses, and COVID-19 is the third known zoonotic coronavirus disease after severe acute respiratory syndrome (SARS) and the Middle East respiratory syndrome (MERS) (5). While all three of these known zoonotic CoV belong to the β-coronavirus genera (6), SARS-CoV-2 is a distinct new β-coronavirus belonging to the subgenus botulinum of Coronaviridae (2). As COVID-19 is a new and rapidly evolving pandemic, knowledge on the disease pathogenesis, clinical manifestations and diagnosis, optimal treatment, and preventative strategies are evolving. Our goal was to rapidly synthesize the accumulating data through our global consortium of experts and to provide an overall overview on COVID-19 disease.
SARS-CoV-2 Characteristics, Viral Shedding, and Diagnostic Testing
SARS-CoV-2 has more than 80% homology to SARS-CoV and 50% to MERS-CoV (7). Although the pathogenesis is not yet precisely defined, the virus enters human cells through the ACE2 receptor (8, 9). Replicating strains have evolved, as many mutations and deletions in coding and non-coding regions of SARS-CoV-2 have been detected (10). There is variation in SARS-CoV-2 detection in body compartments (Figure 1) (11–15). Consistent with other human CoV (hCoV) (16), SARS-CoV-2 has been shown to be shed by asymptomatic subjects to a yet unknown extent (17–19), and it has been suggested that infectiousness might peak on or before symptom onset (20).
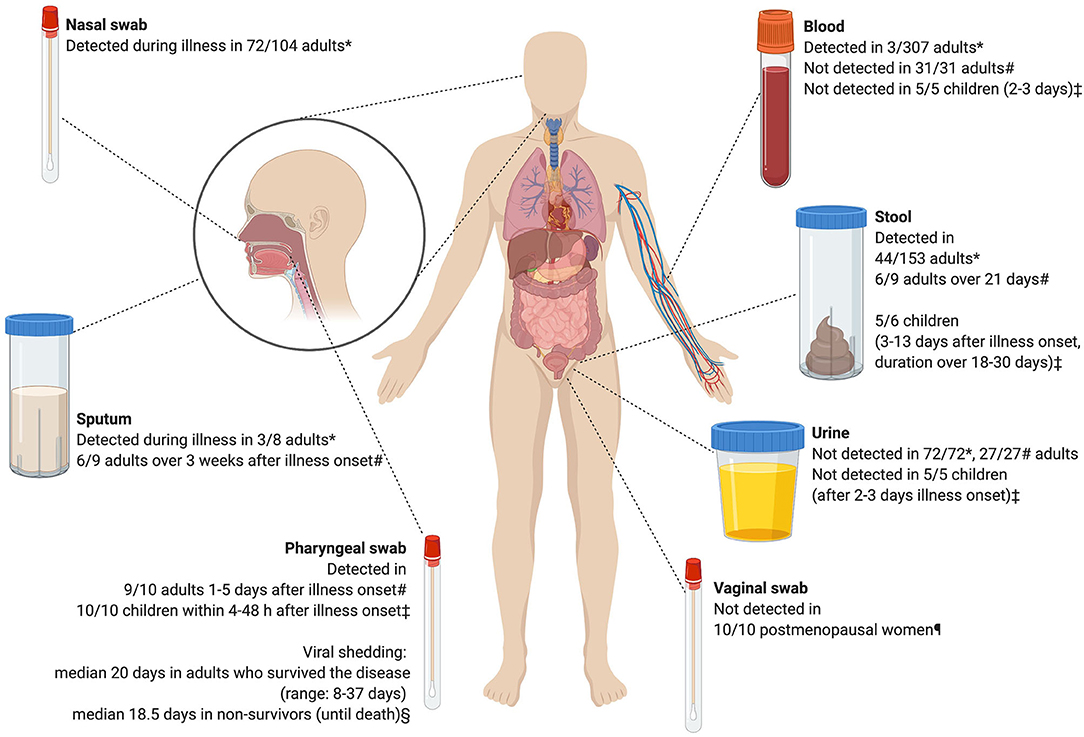
Figure 1. Detection of severe acute respiratory syndrome coronavirus 2 (SARS-CoV-2) by polymerase chain reaction after illness onset. Based on *11, §12, ‡13, #14, ¶15. The figure was created with Biorender.com.
SARS-CoV-2 can remain viable in aerosols and on surfaces. In studies of experimentally induced aerosols SARS-CoV2 was detected for at least 3 h in aerosols in one study (21) and for 16 h in another study (22), which also detected viable virus. SARS-CoV-2 was still detected after 72 and 48 h on plastic and stainless steel, respectively. On copper and cardboard, no viable virus was apparent after 4 and 8 h, respectively (21). In addition to droplet transmission, outbreaks of SARS-CoV-2 that are related to indoor crowded spaces have also suggested aerosol transmission (23–25). The recent WHO statement on the transmission of SARS-CoV-2 still concludes that transmission occurs mainly through direct, indirect, or close contact with infected persons through infected secretions (saliva, respiratory secretions, or respiratory droplets) (26).
Nucleic acid amplification tests (NAAT) of SARS-CoV-2 are currently the gold standard for COVID-19 laboratory diagnosis (27). Limitations in testing include the availability of tests, the need for appropriate swabbing, reduced sensitivity later in the course of the disease (14, 28, 29), and a lag time between obtaining testing and receiving the results, leading to a delay in patient-related actions. The use of oropharyngeal saliva with good sampling have matched the sensitivity of a nasopharyngeal swab in the diagnosis of COVID-19, and yet are able to reduce the workload and protective equipment consumption of health care workers (28). Rapid tests reduce this lag time allowing for more immediate detection (30) and are based on isothermal RNA amplification (31), detection of SARS-CoV-2 antigen in the nasopharynx, and detection of antibodies in blood (32). Two rapid NAATs, developed by Luminex and Abbott, providing results in <1 h have been licensed (32). Rapid antigen detection tests would be a suitable alternative when PCR is not readily available, and it has the advantage of low-cost and short time to results (29, 33) [e.g., Sona Nanotech (Halifax, Canada)].
Anti-SARS-CoV-2 antibodies (IgM/IgG) appear 4–5 days after infection (34, 35), and the seropositivity rate is 50 and 100% after 7, and 14 days of infection, respectively (14). Serology can thus confirm infection in cases that are negative by PCR/antigen detection in patients presenting after 2 weeks from symptoms onset, and in symptomatic contacts of a confirmed case, where contact happened more than 7 days before testing (28).
Immune Response to SARS-CoV-2
The innate immune system provides the first line of defense against viral attacks. However, evidence emerging from in vitro, ex vivo, and in vivo animal models and human studies suggest that SARS-CoV-2 drives an inappropriate innate inflammatory response characterized by low levels of IFN I and III interferon alongside high-inflammatory cytokines, particularly IL-1RA, and IL-6 (36, 37). COVID-19 patients with mild-moderate disease experience a low-grade innate response (38), while those with severe disease have high plasma levels of pro-inflammatory cytokines and chemokines such as IL-2, IL6, IL-7, TNF-α, G-CSF, MCP-1, MIP-1α, and IP-10 (39–41). Furthermore, upregulated chemoattractant chemokines cause the local trafficking of multiple inflammatory cells, including macrophages, natural killer (NK) cells, neutrophils, and T cells, all of which contribute to immunopathology (36). This also accounts for the well-described association between high neutrophil count and disease severity (42).
Neutralizing antibodies (nAbs) against SARS-CoV-2 are thought to be a key component of adaptive protective immunity, yet many patients who recover from COVID-19 only develop low levels of nAbs, while those with severe disease experience an early rise, suggesting a more nuanced role for nAbs, and a possible contribution to immunopathology (43). In addition to neutralization, antibody-induced complement mediated cytotoxicity is also thought to contribute to COVID-19 disease severity (43). Antibodies to both SARS-CoV and MERS-CoV wane with time, it will be important to know whether SARS-CoV-2 antibodies confer long-lasting immunity and protection. Infection with hCoVs other than SARS-CoV and MERS-CoV are common and also induce coronavirus-specific antibodies, some of which are cross-reactive with SARS-CoV-2 but of different functional quality (44). Poor or non-nAbs antibodies may drive the antibody dependent enhancement (ADE) of disease, leading to greater disease severity on subsequent contact with the virus (45). This could hinder the development of safe and effective SARS-CoV-2-specific vaccines (46), however, ADE has not yet been described in patients suffering from COVID-19 (43).
CD4+ and CD8+ T cells are important in controlling viral infections, including SARS-CoV and MERS-CoV (47–50). Our understanding of the role of T-cell mediated immunity (CMI) in COVID-19 is only just being teased out, but a number of studies report virus-specific CD4+ and CD8+ T cells in COVID-19 individuals, particularly CD8+ T cells. These cells are mostly of an activated, and in some reports more exhausted, phenotype (51). It has been suggested that dysregulated T cell function may contribute to the immunopathology observed in COVID-19. While those with mild-moderate disease maintain their lymphocyte counts and have more polyfunctional T cells, studies variously report lower or higher cytotoxicity of CD8+ T cells in those with severe disease (51). The lymphopenia that accompanies severe SARS-CoV-2 infection (52, 53) might suggest viral-induced suppression of CMI, although this could also be due to lymphocyte trafficking to the site of infection (51).
Clinical Manifestations and Prognosis
Children
Several reports of COVID-19 in children have been published (13, 54–59). Children aged <18 years compromised 1 and 1.7% of US (60) and Italian COVID-19 cases (61). In a review of 171 children with COVID-19 from China, fever was present in 41.5% (54), nearly 16% were asymptomatic, and 7% had radiologic features of pneumonia with no symptoms. Although three patients required invasive mechanical ventilation, all had coexisting medical conditions and all patients recovered. In another study from China of 2,135 children with COVID-19 (34% laboratory-confirmed, 66% had suspected disease), 90% were asymptomatic or had mild-moderate disease (59), and one child died. Other smaller case series reported that most children with COVID-19 presented with fever, cough, sore throat, and a small percentage had vomiting and diarrhea (13, 55–58). The virus may persist in the stool of children but whether this is transmissible has not been shown (62). Data on 2,527 pediatric patients reported to the US CDC showed that 73% of 293 children (with data on symptoms) had fever, cough, or shortness of breath. Of 745 children in the US series with information on hospitalization, 147 (20%) were hospitalized and 15 (2%) were admitted to the ICU. Of 345 patients with information on comorbidities, 80 (23%) had at least one comorbidity with chronic lung, and cardiovascular disease most common. Three patients died (60).
Recent data from one New York City pediatric hospital revealed that 16/50 (32%) of those admitted required mechanical ventilation, comorbidity was found in 33/50 (66%), and that the most common comorbidity was obesity reported in 11/50 (22%) patients (63). Only one fatality was reported from sudden cardiac arrest that followed a period of severe hypoxemia. In this cohort, infants, and immune-compromised children did not suffer from severe disease, but the numbers were small (63). Another study on hospitalized children admitted to a tertiary care center in New York City revealed that 14/46 (30.4%) were obese, but this comorbidity was not associated with admission to the PICU, and one patient died (CFR of 2%) (64). A recent study of 46 Canadian and US pediatric intensive care units reported on 48 patients admitted during a 3-week period. Notably, only 35% of the 46 hospitals reported admissions of children with COVID-19 to the PICU, which further emphasizes that severe disease is relatively less frequent in children. In this small cohort, comorbidity was noted in 40/48 (83%), 18/48 (38%) required invasive ventilation, and the overall CFR was 4.2% (65).
A newly described inflammatory disease related to SARS-CoV-2 has recently been reported in children. It has been termed Pediatric Multisystem Inflammatory Syndrome (PMIS) or Multisystem Inflammatory Syndrome in Children (MIS-C). Reports from Europe and the U.S. describe critically ill children with fever, rash, conjunctivitis, abdominal complaints, shock, and significant cardiac dysfunction (66–71). Several of the children described appeared to have had a history of prior SARS-CoV-2 infection several weeks earlier or have anti-SARS-CoV-2 antibodies detected. Case definitions have been developed to better characterize these patients (72). Empiric treatment has generally involved high-dose intravenous immunoglobulin (2 g/kg), steroids, and rarely more targeted anti-inflammatory medications such as anakinra (68–71).
Pregnancy
Small case series described the clinical features in pregnant women with COVID-19 (73–75). Signs and symptoms in pregnant women were similar to non-pregnant individuals (76). Chen et al. (73) reported that all 9 women in their report with COVID-19 had cesarean sections, 2 for fetal distress, 2 for preterm premature rupture of the membranes, and one for preeclampsia. Overall, premature delivery is reported in 47% (15/32) of COVID-19 cases in pregnancy (77). Recently, a 2nd trimester miscarriage in a pregnant woman with COVID-19 was reported, with SARS-CoV-2 detected by PCR in the placenta (78).
Vertical transmission of SARS-CoV-2 to the infant is a potential concern (79–81). Some reports have not documented vertical transmission of SARS-CoV-2 (73–75, 82), others have described potential transmission (83). A report of 10 sick neonates born to women with COVID-19 had fetal distress, premature labor, respiratory symptoms, and one died, but vertical transmission was not documented (82). Small case series reported on the presence of anti-SARS-CoV-2 IgM at birth or early life in asymptomatic newborns of women with COVID-19 (84, 85). The presence of IgM in newborns suggests that it is of fetal origin. Out of 33 newborns of women infected with SARS-CoV-2 during pregnancy, three had early-onset SARS-CoV-2 infection, but their outcome was favorable and it is unclear whether they were infected in-utero or after birth (86, 87). Overall, growing evidence suggests that vertical transmission is not to be expected (88, 89).
The UK Royal College of Obstetricians and Gynecologists most recent statement published April 17 2020 recommends that antenatal care to be continued routinely, and attention for the hypercoagulable state of a pregnant woman in view of COVID-19 hypercoagulability, has to be considered, as well as the mental health of pregnant women (90). Although it was suggested that neonates should be isolated when infected (91), the WHO and several national bodies recommend isolation together with the mother. SARS-CoV-2 has not been detected in human milk and thus breast-feeding should be encouraged, although all the measures required to avoid transmission from the mother are needed.
Adults
Early in the disease course, adults infected with SARS-CoV-2 may present with fever, alterations in taste and/or smell and mild respiratory or gastrointestinal symptoms (12, 92, 93). Later during disease, a fraction of patients may develop shortness of breath, chest tightness, and palpitations leading to hospitalization (94). Cohorts may differ for age and presence of comorbidities [mainly hypertension, diabetes mellitus, chronic obstructive pulmonary disease [COPD], coronary heart disease, cerebrovascular disease, and malignancy] leading to variable outcomes. In fact, while a cohort of 1,099 adults with COVID-19 from China, the median age was 47 years and 25% had underlying chronic illness, a cohort of 5,700 patients (median age 63 years) from New York (USA) presented a chronic comorbidity in more than 60% and an Italian one with 1,591 patients (median age 63 years) presented at least one comorbidity in 68% (94–96). While 80% of patients of the Chinese cohort had mild disease, 15.7% had severe disease, the majority of these patients were older than 65 years and those with coexisting morbidities, and 5% were critical, requiring ventilatory or extracorporeal membrane oxygenation (ECMO) support. Another study from China concentrated on a more severe cohort of patients, of whom 54 out of 191 died (12). Nearly half of the patients had underlying comorbidities (30% of the entire cohort had hypertension and 19% had diabetes). Death was associated with older age, higher disease severity score (SOFA), and elevated blood d-dimer on admission. These findings may help to identify patients who will go on to have severe disease. Using data from 169 hospitals in Asia, Europe, and North America, independent risk factors for death were age > 65 years, coronary artery disease, heart failure, cardiac arrhythmia, and COPD (97), a finding that is supported by a recent multicenter US study (98). It has been reported that the prevalence of asthma in patients with COVID-19 is lower than in the geography-matched adults population, and it has been suggested that respiratory allergies might be associated with reduced ACE2 expression in airway cells (41, 99). Initial data suggested that gender has also been shown to differentially affect the outcome of COVID-19 patients. A small study from China found that men with COVID-19 are more at risk for worse outcomes and death, independent of their age (100), a finding later confirmed by an interim meta-analysis (101).
Recently, it was reported that 12/38 adult patients with COVID-19 had ocular manifestations (e.g., conjunctival hyperemia, chemosis, epiphora, or increased secretions) (102). Guillian-Barre syndrome was also associated with SARS-CoV-2 infection in 5 out of 1,000–1,200 admitted patients in 3 hospitals in Italy after an interval of 5–10 days after illness onset (103). However, the causal relationship remains to be investigated. Large vessel stroke has also been reported to be a presenting symptom of COVID-19 in a small case series in young adults (104).
Elderly
COVID-19 is severe in older individuals. Death rates have been reported as higher in Italy, Spain, and France in comparison to China, perhaps related to older populations in Europe. These countries differ in the percentages of population over 65 (the age-group most afflicted by infection, 23% in Italy) and life expectancy (e.g., 83.4 years in Italy vs. 76.7 years in China) (105). These demographic differences could partially explain why Italy has a higher overall case-fatality rate CFR (7.2%) compared with China (2.3%). Interestingly, the CFR in Italy and China are similar for age groups 0–69 years, but higher in Italy among >80 years old patients (52% of deaths, 20% CFR), and especially >90 years old (22.7% CFR) (105). However, CFR should be interpreted with caution as it is affected by testing strategy and capacity and the number tested.
Aging is accompanied by immune senescence and the enhanced tendency to inflammation (106). The chronic increase in inflammatory cytokines may explain the higher tendency for pulmonary fibrosis and clotting dysfunction following infection with SARS-CoV-2, especially in older patients with multiple comorbidities (39, 107), which affect >60% of people >65 years of age (108). Data from 355/2003 (17.7%) Italian patients who died from COVID-19 showed that nearly 50% had ≥3 comorbidities (105). Cardio-respiratory and metabolic diseases were associated with poor prognosis (39). The use of multiple medications and the potential drug-drug interaction might increase the risk of adverse drug effects and thus require a careful evaluation.
Comorbidities, anti-viral and concomitant medication, and COVID-19 appear to be associated with hyperactive delirium, especially in hospitalized patients with pre-existing cognitive impairment (109). As suggested by NICE rapid guidelines and the Canadian Frailty Network, the assessment of all adults for frailty is highly recommended especially at hospital admission, which can guide clinicians in the decision to admit to ICU and in selecting therapeutic choices (110). A grading system has also been reported for US hospitals to provide a framework for making allocation decisions (111). However, the European Geriatric Medicine Society has stated that advanced age should not be a criterion for excluding patients from care (112).
Radiographic Features
Although the diagnosis of COVID-19 is based on the identification of SARS-CoV-2 by PCR, radiological findings are useful complements in the diagnosis and management of COVID-19 pneumonia. However, there is still no consensus for the use of chest radiography or computed tomography (CT) for evaluating patients with suspected COVID-19 pneumonia. The British Society of Thoracic Imaging considers chest radiography as a key decision tool for suspected COVID-19 pneumonia (113). As the predominant pattern seen in COVID-19 pneumonia is ground-glass opacification, detecting COVID-19 pneumonia on chest radiography is likely to be challenging, and is complicated by the presence of comorbidities. The Chinese experience indicates that chest CT is the preferred diagnostic modality for COVID-19 pneumonia (114). The most common CT findings of the COVID-19 pneumonia, ground glass opacification and/or consolidation, mainly reflect diffuse alveolar damage and/or organizing pneumonia, which overlap with non-COVID-19 etiologies (Figure 2) (114). Specificity for COVID-19 pneumonia can be increased if its peripheral distribution, fine reticular opacity, and vascular thickening is included (115). There is a correlation between the severity of pulmonary findings on CT and patients outcome (116). Thus, even if not specific, it has been suggested that chest CT could be used as a helpful diagnostic test in the emergency work up of COVID-19, complementing PCR. Chest CT had higher sensitivity for early diagnosis of COVID-19 compared with PCR (117), however, it should be reserved for patients who are not improving or who show worsening respiratory symptoms. It should be noted that 11–15% (sporadically up to ~50%) of patients may have normal chest CT scans up to 2 days after the onset of symptoms (118). The latter findings and the necessary cleaning procedures of both the CT room and personal protection equipment may challenge its integration in the routine work up of COVID-19.
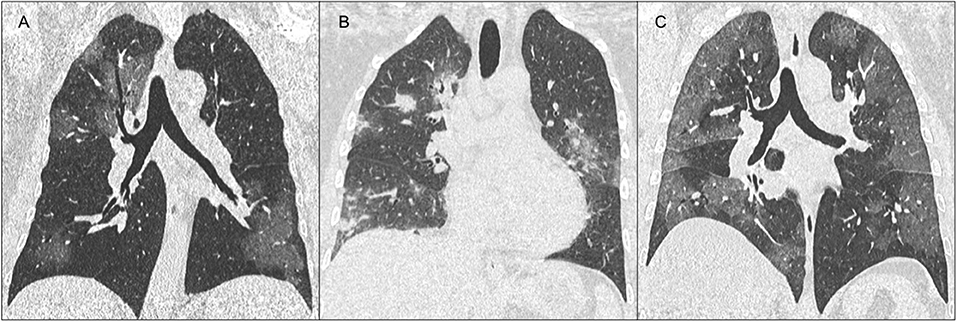
Figure 2. Representative computed tomography (CT) images of various manifestations of the COVID-19. (A) Coronal chest CT images show patchy ground-glass opacities involving both lungs. (B) Ground glass may also appear widespread, confluent, and peripherally distributed. (C) Consolidation and rounded nodules may be also observed in association with ground glass opacities.
Therapeutic Approach and Intensive Care Management
Pharmacological Treatment
Pharmacological treatment includes drugs targeting key components of the virus entry to alveolar epithelial cells or their reproduction or the host immune system (Figures 3, 4). Lopinavir/ritonavir evaluated in an open label randomized controlled trial in severe cases and late-presenters (median 13 days from symptoms onset), failed to show significant improvement in virologic or clinical response compared to standard of care (140). Gastrointestinal adverse effects including nausea and diarrhea, and drug-drug interaction limit its use in older patients with polypharmacy.
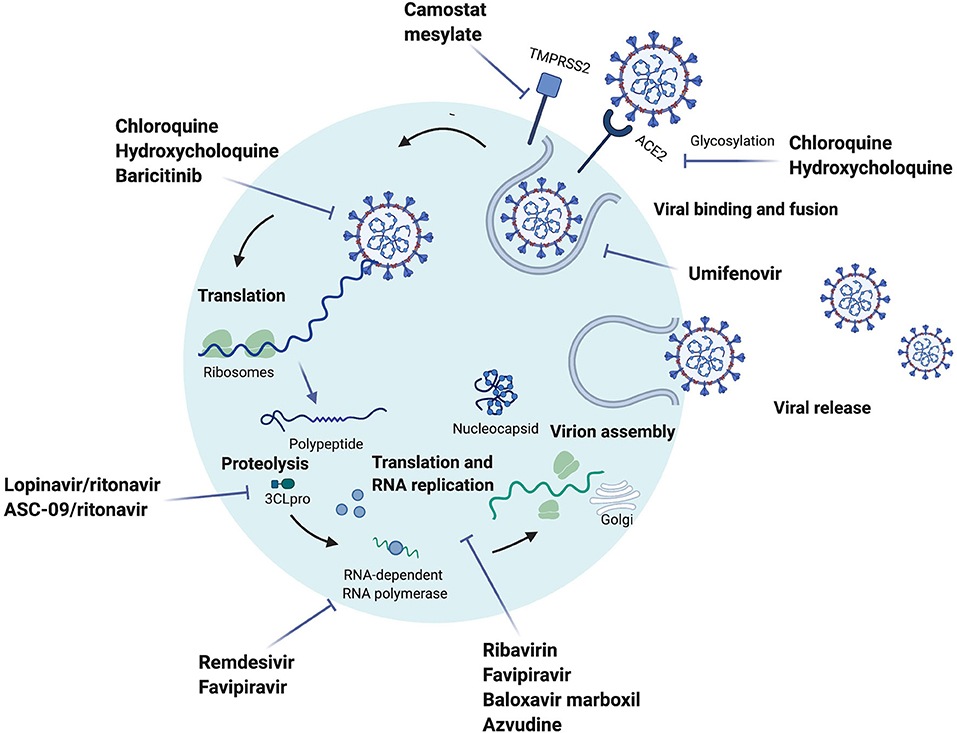
Figure 3. Drugs under investigation for potential use for Coronavirus disease-19 targeting SARS-CoV-2 and their proposed mechanism of action. Umifenovir inhibits the fusion of the virus to the cell (119, 120). Camostat mesylate inhibits the cellular serine protease TMPRSS2, which has been suggested to be a potential entry of the virus (8, 119). Chloroquine (CQ)/hydrochloroquine (HCQ) mechanism of action is still unclear, however it has been suggested that the drug inhibits the glycosylation of ACE2, and disrupts the late stages of viral entry (119, 121–123). Baricitinib is suggested to have an effect on the endocytosis due to the inhibition of AP-2-associated protein kinase 1 (119, 124). Lopinavir/ritonavir and ASC-09/ritonavir are protease inhibitors, lopinavir/ritonavir is inhibiting the 3CLpro proteinase, which is translating the polypeptide from the genomic RNA (125). Remdesivir is an adenosine analog that moves into the viral RNA and inhibits the RNA-dependent RNA polymerase, which stops the RNA synthesis (119, 126). Baloxavir marboxil, in the influenza virus, inhibits the protein cap-dependent endonuclease, which results in inhibiting viral transcription (127). Azvudine is a nucleoside reverse transcriptase inhibitor that potentially affects the replication of SARS-CoV-2 (128). A proposed mechanism of action for favipiravir is the inhibition of the viral RNA synthesis due to its wide anti-RNA virus activity, it is known to also inhibit the RNA-dependent RNA polymerase (129, 130). Ribavirin has a broad antiviral activity, it is suggested to have an indirect effect on the RNA replication (131). The figure was created with Biorender.com.
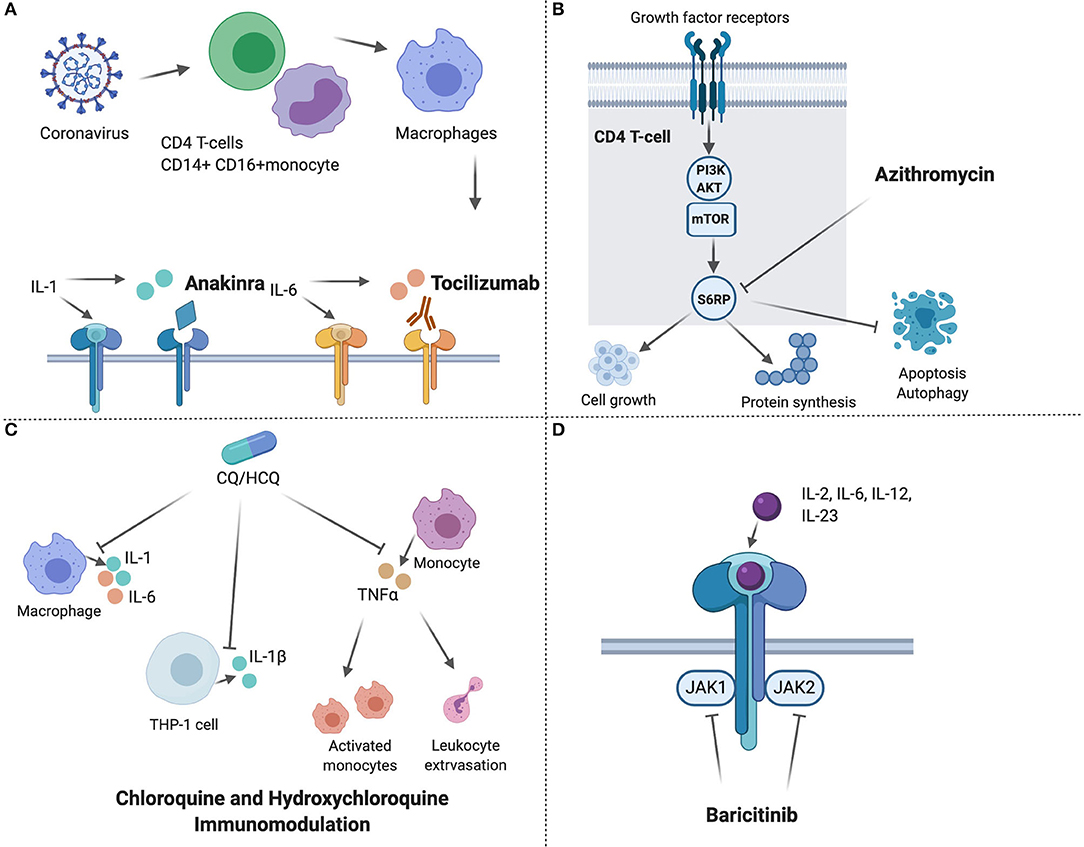
Figure 4. Host targeted drugs with potential use for Coronavirus disease-19 and their proposed mechanism of action. (A) Anakinra, is a human Interleukin 1 receptor antagonist, and tocilizumab is a monoclonal antibody that binds to IL-6 receptors (132, 133); (B) Azithromycin inhibits phosphorylation of S6RP in the mTOR pathway in T cells, which leads to reduced cell growth, protein synthesis, and increased apoptosis and autophagy of T cells (134); (C) Immunomodulating effects of chloroquine and hydroxychloroquine, which inhibits the production of IL-1, IL-1β, IL-6, and TNFα (121, 135, 136); (D) Baricitinib inhibits the activation of different interleukins and growth factors through inhibiting the JAK1 and JAK2 on the cytokine receptor (137–139). The figure was created with Biorender.com.
Studies have suggested that the SARS-CoV-2 induces low levels of IFN I and III (36, 37). Recently, a phase-2 open label randomized controlled trial showed that early treatment (median of 4 days from symptoms onset) with the triple combination of interferon beta-1b, lopinavir-ritonavir, and ribavirin was safe and highly effective in shortening the duration of viral shedding, alleviating symptoms, and reducing cytokine responses, when compared to lopinavir-ritonavir alone in mild to moderate cases (141).
Chloroquine and its alternative hydroxychloroquine showed in vitro activity (142). However, available clinical data failed to show a clinical benefit of hydroxychloroquine either as treatment or prophylaxis (143–145) which was confirmed in a recent meta-analysis (146). Cardiotoxicity (e.g., prolongation of QT leading to torsades de pointes) is a well-known adverse effect of these drugs and should be balanced (147–150). Promising results of a recent phase 2 study exploring the efficacy of a combination of interferon beta-ib, lopinavir-ritonavir, and ribavirin warrant further evaluation (141).
Remdesivir is a broad-spectrum antiviral with potent activity against RNA viruses (151, 152). It reduced viral loads in SARS-CoV-infected mice (151) and has potent in vitro activity against SARS-CoV-2 (53). A 10-day course has been shown to be associated with clinical improvement in 36 out of 53 COVID-19 patients (153), but the lack of control group challenges the interpretation of such data. In a recently published RCT, Remdesivir use was not associated with a statistically significant difference in time to clinical improvement compared with placebo among patients with symptom duration of 12 days or less. However, the study was stopped before reaching the pre-specified sample size challenging any definite conclusions (154). A phase 3 study did not show a difference between a 5-day course and a 10-day course but unfortunately lacked a placebo arm to determine its benefit compared to standard of care (155). However, when compared to placebo it was able to show a shortening of time to recovery (156). Tolerability is expected to be good based on its high viral selectivity. Remdesivir has been issued emergency use authorization by the FDA and is being evaluated by the EMA for a conditional marketing authorization.
Interleukin receptor inhibitors like tocilizumab and anakinra have been suggested to curb the cytokine storm (157–159). Drugs like ribavirin, favipiravir, umifenovir, nitazoxanide, darunavir/cobicistat, and IFN-beta are being investigated (157, 160).
The use COVID-19 pharmacological therapies is recommended in the context of clinical trials (143). When designing drug trials it is important to use physiologically-based pharmacokinetic (PBPK) models to select the most appropriate dose likely to be successful (142). Drugs eligible for further evaluation against COVID-19 drug lung concentrations should at least exceed in vitro EC90 values (161). In addition, the timing of drug administration is another important consideration. WHO is leading a multi-country, randomized trial comparing standard of care with remdesevir, lopinavir/ritonavir, lopinavir/ritonavir, and IFN-beta1a, or chloroquine/hydroxychloroquine (solidarity trial).
Intensive Care Management
The COVID-19 pandemic is having a highly significant impact on ICUs (162). Early data from China (163) reported that 5% of all COVID-19 patients required ICU admission and the CFR of patients with ARDS was 54% (164). Patients admitted to the ICUs with ARDS present with a severe form of the disease and require mechanical ventilation and 5% required ECMO. Data from Italy on 1,591 patients admitted to the ICU in the Lombardy region showed that of 1,403 patients with available data on comorbidity, 709 (68%) had at least one comorbidity and 509 (49%) had hypertension. Patients older than 64 years old had a higher mortality rate than patients younger than 63 years old, 36 vs. 15%, respectively (96).
The Surviving Sepsis Campaign recommendations provide guidance on the management of adults with COVID-19 in the ICU and these guidelines grade the evidence and provide best practice statements where evidence is lacking (165), such as: the use of fit-tested FFP2 respirators for personal protection of healthcare workers, the use of negative pressure rooms for patients having aerosol generating procedures, and the performance of endotracheal intubation by experienced personnel to avoid nosocomial spread of SARS-CoV-2. Areas where no recommendation was made include the use of helmet non-invasive ventilation or the therapeutic use of antivirals, chloroquine or hydroxychloroquine, interferon gamma, anakinra, and tocilizumab. One important area of controversy is the use of corticosteroids for ARDS. Although steroids have been recommended for patients with ARDS (165), their use should be individualized and is preferable in settings with clinical trial capacity (143, 166). ARDSNet guidelines recommend against the routine use of corticosteroids in patients with ARDS. However, the recent SSC COVID-19 guidelines recommend their use in COVID-19 patients with ARDS (weak evidence) although without full agreement from all panel members. Guidance for the management of critically ill adults with COVID-19 is outlined (Panel 1).
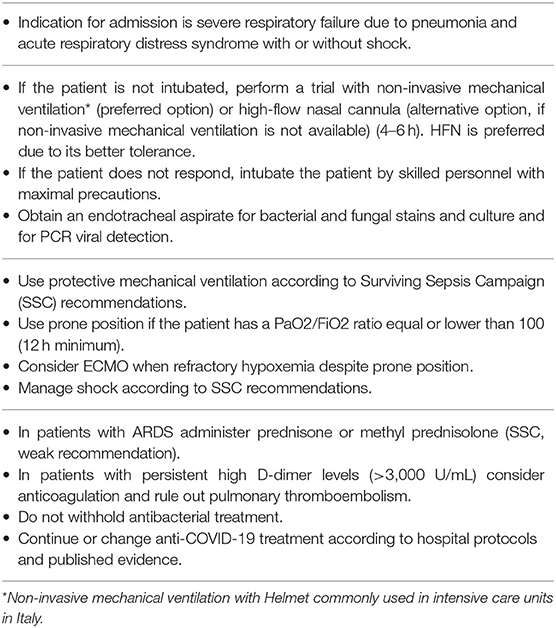
Panel 1. Recommended management of patients admitted to intensive care unit with Coronavirus disease-19.
Public Health Response
Case Definition
The initial WHO case definitions for COVID-19 consider suspected, probable, and confirmed cases and requests national authorities to report both probable and confirmed cases (Panel 2). Case-based reporting is done daily, and aggregated data are sent to the WHO on a weekly basis. Selected health conditions, which may predispose people to COVID-19 (e.g., pregnancy, cardiovascular diseases, and immunodeficiency) are reported, and whether the patient is a health care worker.
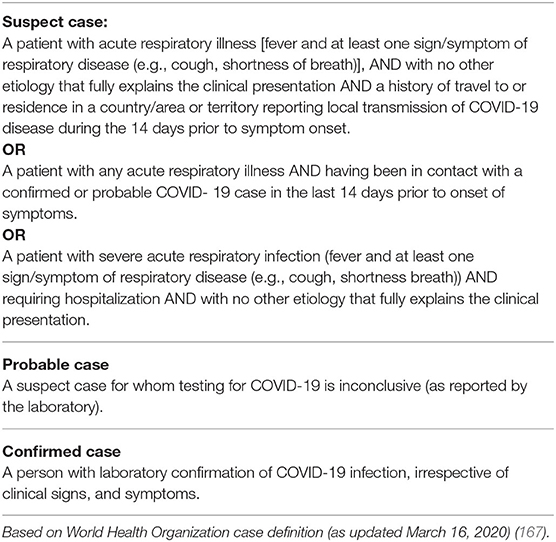
Panel 2. World Health Organization Coronavirus disease-19 (COVID-19) case definitions (as updated March 16, 2020).
The case definitions are established to verify that individuals with the highest risk of acquiring the disease are tested. This helps in providing early isolation and avoids further transmission of SARS-CoV-2. History of exposure is reported to document transmission patterns in the communities and 14 days is considered as the incubation period to cover a relatively large confidence interval (168). Therefore, history of travel in the last 14 days and the list of country/countries where the individual is traveling from are also reported. Once community transmission has been documented, case definitions require modification and should be based on the most common symptoms. It was recently shown that the prevalence of SARS-CoV-2 among patients that would have missed risk-based testing was ~5% among adults with flu-like symptoms in California (169).
Infection Control
Traditionally, infection prevention and control principles are based on a hierarchy of administrative, environmental, and personal protective measures (masks for infectious patients and respirators for airborne agents to protect health care workers and visitors) (170). This approach has been well-summarized for tuberculosis (170), but has also been suggested for COVID-19 (171, 172). While N95/N99/FFP2/FFP3 masks are recommended to protect health care workers and other exposed individuals in the workplace, there is debate on the use of surgical masks (173, 174). Although there is agreement on the use of surgical masks to limit the spread of droplet nuclei for symptomatic patients under isolation, there is an ongoing dialogue for the potential mass use of surgical masks to limit the community spread of COVID-19 in early stages infection and from asymptomatic individuals (171–175). Arguments against this have been raised, based on the potential false sense of protection this can generate and the potential risks of moisture retention, long mask re-use, and limited filtration capacity (176). Studies performed on influenza confirm that surgical masks are up to 3 times more effective in reducing droplet transmission than home-made masks (176, 177). The interim WHO guidance (5 June 2020) on the use of masks in the context of COVID-19 states that the use of masks by healthy people in the community is not supported by high quality evidence. However, governments should encourage the general public to wear masks in specific situations and settings (178). Specific infection control considerations for COVID-19 are detailed in Panel 3.
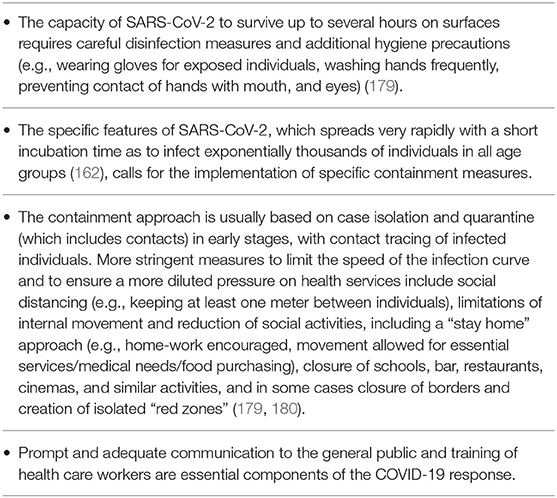
Panel 3. Infection control and containment measures considerations specific for SARS-CoV-2 infection.
Country-Specific Responses
On March 18 2020, the WHO Regional Office for Europe issued a statement (181) summarizing the situation of Europe as under the “Four Cs” scenarios of the outbreak: (1) no case; (2) first case; (3) first cluster; and (4) first evidence of community transmission. The pandemic is progressing at different speeds and at different times in different countries, depending on demographics and other factors (e.g., population mixing, migration, and international travel). However, the basic actions to be undertaken under each scenario are the same. These include strict measures to interrupt human-to-human transmission including active case-finding followed by rapid diagnosis and isolation with immediate physical distancing and travel-related (e.g., travel restrictions and border closure) measures (182, 183).
Surveillance is critical in understanding the progression of the pandemic. Rapidly establishing sensitive surveillance and widespread testing ensures that cases are identified promptly and effective contact tracing is in place in the early stages when there are only a small number of cases. As the outbreak progresses, seroprevalence studies can help in estimating infections in communities, the extent of spread of asymptomatic transmission, the role different age groups might be playing in enhancing transmission, and the acquisition of population immunity.
The WHO recommended that countries: (1) prepare and be ready; (2) detect, protect, and treat; (3) reduce transmission; and (4) innovate and learn, while protecting vulnerable people. Herein, we describe public health responses and lessons learned from several countries identified because of the caseload, the strategic importance, and direct experience within the writing committee.
China
After the major outbreak was recognized, the city of Wuhan was cordoned off on January 23 2020 (184). However, around 5 million people had already left Wuhan during the peak transport period before the Chinese New Year. An extreme form of social distancing and compulsory mask-wearing in public places were undertaken all over the country to block human-to-human transmission (173, 185). These measures appeared to eliminate most of the transmissions with unclear links in the community, and many of the subsequently observed cases then appeared in clusters, mostly involving families (76). Intensive case-finding and isolation were undertaken together with contact tracing and quarantine of contacts and other high-risk groups using big data and artificial intelligence. The spread of COVID-19 was rapidly brought under control outside the province of Hubei, allowing for the staged resumption of essential economic activities with modification of the work process and environment to minimize person-to-person contact.
More than 1,800 health care workers were infected in Hubei, mostly occurring early in the overwhelmed hospitals with severe shortages of personal protective equipment in Wuhan (76). Successful confinement of massive outbreaks of COVID-19 to Wuhan and other cities of Hubei allowed for the timely channeling of disaster response capacity of the country to these seriously affected areas. Hospital capacity was rapidly expanded with reinforced manpower and personal protective equipment to accommodate all patients with severe disease. New intermediate care facilities were rapidly constructed and manned by rescue teams from other parts of the country to care for the much larger number of patients with milder disease. Effective triage of patients according to their treatment needs maximized the health care capacity and throughput to accommodate all subsequent patients in an environment safer for themselves, their families, the health care staff, and the community.
Italy
The epidemic in Northern Italy took place about 4 weeks after that in China, while other European countries followed Italy with a delay of 7–10 days. Italy started creating a closed “red zone” around the municipalities initially experiencing the outbreaks. The “red zone” was then extended to entire Regions (Lombardia, Veneto, part of Emilia Romagna) and then to the entire country. Movement restrictions, closure of schools, and other social aggregation sites were implemented early, although people's compliance was suboptimal initially. An extraordinary effort was conducted to increase the number of ICU beds and to procure masks, respirators, and ventilators, which, in the early phases, were lacking. The response was coordinated by the Civil Protection which is well-organized in Italy to ensure a rapid response to earthquakes. The situation has improved significantly since the end of March 2020.
Several countries followed this approach while others followed slightly different ones (Table 1).
Other Experiences
The UK response has been based on (186) (1) contain (detect early cases, follow-up close contacts); (2) delay (slow the spread, lower the peak impact); (3) research; and (4) mitigate (provide the best care for cases, support hospitals to maintain essential services, ensure ongoing support for ill people in the community, and minimize the impact of the disease on society, public services, and economy). Early on it was thought that by “slowing spread” rather than “suppression,” the peak could be pushed into the summer when there is less pressure on the health service. However, it became clear that this approach was not going to be successful given the level of spread already within the population. Thus, the government rapidly moved to a strategy of “suppression” in line with the responses of other countries. The study that predicted that the National Health Service would be overwhelmed if a mitigation strategy continued was pivotal in the change of the UK approach (187). This approach raised discussions in the UK and beyond (188).
In Sweden, travels were discouraged but not prohibited, schools partially closed, bars and restaurants continued to operate. Sweden has chosen one of the most liberal approaches seen in Europe, with many measures being of a suggestive, recommended, or non-compulsory character. This approach differs from that of other countries in Europe, and was associated with a relatively high cumulative case incidence and mortality. After 4 months from the epidemic onset, the overall mortality rates are approaching normal levels in most of the affected countries, following a period of a substantial excess mortality, which was also observed in UK, Italy, Spain, and Belgium.
In the US, testing became free for all on March 16 2020 but, initially, there were limited numbers of tests available. Movement of individuals was discouraged while domestic travel was still allowed. Massive testing was performed in the US and strict quarantines were imposed in large cities with different approaches from State to State (Table 1).
Future Perspectives
The future pandemic course will depend on the early implementation, massive diagnosis, and contact tracing/isolation in addition to broad restrictions leading to significant and prolonged physical distancing. Nevertheless, considering that sufficient population immunity will be required to definitely control the virus in the upcoming years (unless it were to mutate to a less virulent, competing virus), which will require several years of manageable levels of viral infection, introducing, and retreating social restrictions proving most effective, can be envisioned for the near future (187). Fast track drug development and repurposing of available drugs should be informed initially by in vitro data to support activity, PBPK data to inform dose selection, and well-designed, prospective randomized-controlled trials powered to detect a clinically meaningful outcome.
Safe and effective vaccines would be the most suitable solution generating the required population immunity to stop virus circulation. Vaccine development is in progress, both by pharmaceutical companies and research institutions. On the website of the WHO, a draft landscape of a growing number of COVID-19 candidate vaccines can be found. Most vaccines are still in the preclinical phases of the development, but some of them are already in Phase I clinical trials (189, 190). Previous preclinical studies with vaccine candidates for SARS and MERS demonstrated the induction of neutralizing antibodies, but a possibility for enhanced disease had been seen in some vaccinated animals after challenge with the wild-type virus (191), and in experimental models (191, 192). Potential vaccines will need to be carefully studied, the duration of immunity assessed, and the potential for enhanced vaccine disease will need to be carefully evaluated over time. Importantly, from here on, the inevitable threat of new emerging coronaviruses (193) will have to be confronted earlier. China acted well, but too late, and the virus spread in weeks to the rest of the world, which in turn also acted too late, with few exceptions; and some countries continue to do so. Preparing for new events will require coordinated efforts between epidemiologists, clinical and basic science researchers, and artificial intelligence experts; the time is now. Hot spots for new coronavirus emergence need to be monitored continuously to detect severe human cases very early on. In this new post COVID-19 era, transparency and collaboration will be critical to confront future pandemic threats.
Author Contributions
SE, BA-R, and GM conceived the project and designed the outline. All authors searched the scientific literature and contributed to the writing of the different sections of the first draft. All authors reviewed and edited the manuscript at the different stages of development and approved the final version.
Funding
A-GM was funded by Marie Skłodowska-Curie Actions [Grant agreement no. 529 713660—PRONKJEWAIL—H2020-MSCA-COFUND-2015]. AT was supported by IDIBAPS, CIBERES, and ICREA.
Conflict of Interest
BA-R was supported by the Canadian Health and Research institute Vanier Canada scholarship.
The remaining authors declare that the research was conducted in the absence of any commercial or financial relationships that could be construed as a potential conflict of interest.
Acknowledgments
The article is part of the activities of the Global Tuberculosis Network (GTN), and of the WHO Collaborating Centre for Tuberculosis and Lung Diseases, Tradate, ITA-80, 2017-2020- GBM/RC/LDA).
References
1. Report of clustering pneumonia of unknown etiology in Wuhan City. Wuhan Municipal Health Commission. (2019) Available online at: http://wjw.wuhan.gov.cn/front/web/showDetail/2019123108989 (accessed October 16, 2020).
2. Zhu N, Zhang D, Wang W, Li X, Yang B, Song J, et al. A novel coronavirus from patients with pneumonia in China, 2019. N Engl J Med. (2020) 382:727–33. doi: 10.1056/NEJMoa2001017
3. World Health Organization. Naming the Coronavirus Disease (COVID-19) and the Virus that Causes it. Available online at: https://www.who.int/emergencies/diseases/novel-coronavirus-2019/technical-guidance/naming-the-coronavirus-disease-(covid-2019)-and-the-virus-that-causes-it (accessed October 16, 2020).
4. Li Q, Guan X, Wu P, Wang X, Zhou L, Tong Y, et al. Early transmission dynamics in Wuhan, China, of novel coronavirus-infected pneumonia. N Engl J Med. (2020) 382:1199–207. doi: 10.1056/NEJMoa2001316
5. Memish ZA, Perlman S, Van Kerkhove MD, Zumla A. Middle east respiratory syndrome. Lancet. (2020) 395:1063–77. doi: 10.1016/S0140-6736(19)33221-0
6. Chen Y, Liu Q, Guo D. Emerging coronaviruses: genome structure, replication, and pathogenesis. J Med Virol. (2020) 92:418–23. doi: 10.1002/jmv.25681
7. Hui DS, I Azhar E, Madani TA, Ntoumi F, Kock R, Dar O, et al. The continuing 2019-nCoV epidemic threat of novel coronaviruses to global health - the latest 2019 novel coronavirus outbreak in Wuhan, China. Int J Infect Dis. (2020) 91:264–6. doi: 10.1016/j.ijid.2020.01.009
8. Hoffmann M, Kleine-Weber H, Schroeder S, Krüger N, Herrler T, Erichsen S, et al. SARS-CoV-2 cell entry depends on ACE2 and TMPRSS2 and is blocked by a clinically proven protease inhibitor. Cell. (2020) 181:271–80.e8. doi: 10.1016/j.cell.2020.02.052
9. Aleksova A, Ferro F, Gagno G, Cappelletto C, Santon D, Rossi M, et al. COVID-19 and Renin-Angiotensin system inhibition - role of angiotensin converting enzyme 2 (ACE2) - Is there any scientific evidence for controversy? J Intern Med. (2020) 8:10.111/joim.13101. doi: 10.1111/joim.13101
10. Phan T. Genetic diversity and evolution of SARS-CoV-2. Infect Genet Evol. (2020) 81:104260. doi: 10.1016/j.meegid.2020.104260
11. Wang W, Xu Y, Gao R, Lu R, Han K, Wu G, et al. Detection of SARS-CoV-2 in different types of clinical specimens. JAMA. (2020) 323:1843–4. doi: 10.1001/jama.2020.3786
12. Zhou F, Yu T, Du R, Fan G, Liu Y, Liu Z, et al. Clinical course and risk factors for mortality of adult inpatients with COVID-19 in Wuhan, China: a retrospective cohort study. Lancet. (2020) 395:1054–62. doi: 10.1016/S0140-6736(20)30566-3
13. Cai J, Xu J, Lin D, Yang Z, Xu L, Qu Z, et al. A case series of children with 2019 novel coronavirus infection: clinical and epidemiological features. Clin Infect Dis. (2020) 28:ciaa198. doi: 10.1093/cid/ciaa198
14. Wölfel R, Corman VM, Guggemos W, Seilmaier M, Zange S, Müller MA, et al. Virological assessment of hospitalized patients with COVID-2019. Nature. (2020) 581:465–9. doi: 10.1038/s41586-020-2196-x
15. Qiu L, Liu X, Xiao M, Xie J, Cao W, Liu Z, et al. SARS-CoV-2 is not detectable in the vaginal fluid of women with severe COVID-19 infection. Clin Infect Dis. (2020) 71:813–7. doi: 10.1093/cid/ciaa375
16. Heimdal I, Moe N, Krokstad S, Christensen A, Skanke LH, Nordbø SA, et al. Human coronavirus in hospitalized children with respiratory tract infections: a 9-year population-based study from Norway. J Infect Dis. (2019) 219:1198–206. doi: 10.1093/infdis/jiy646
17. Li C, Ji F, Wang L, Hao J, Dai M, Liu Y, et al. Asymptomatic and human-to-human transmission of SARS-CoV-2 in a 2-family cluster, Xuzhou, China. Emerg Infect Dis. (2020) 26:1626–8. doi: 10.3201/eid2607.200718
18. Kimball A, Hatfield KM, Arons M, James A, Taylor J, Spicer K, et al. Asymptomatic and presymptomatic SARS-CoV-2 infections in residents of a long-term care skilled nursing facility - King County, Washington, March (2020). MMWR Morb Mortal Wkly Rep. (2020) 69:377–81. doi: 10.15585/mmwr.mm6913e1
19. Arons MM, Hatfield KM, Reddy SC, Kimball A, James A, Jacobs JR, et al. Presymptomatic SARS-CoV-2 infections and transmission in a skilled nursing facility. N Engl J Med. (2020) 382:2081–90. doi: 10.1056/NEJMoa2008457
20. He X, Lau EHY, Wu P, Deng X, Wang J, Hao X, et al. Temporal dynamics in viral shedding and transmissibility of COVID-19. Nat Med. (2020) 26:672–75. doi: 10.1101/2020.03.15.20036707
21. van Doremalen N, Bushmaker T, Morris DH, Holbrook MG, Gamble A, Williamson BN, et al. Aerosol and surface stability of SARS-CoV-2 as compared with SARS-CoV-1. N Engl J Med. (2020) 382:1564–7. doi: 10.1101/2020.03.09.20033217
22. Fears AC, Klimstra WB, Duprex P, Hartman A, Weaver SC, Plante KS, et al. Persistence of severe acute respiratory syndrome coronavirus 2 in aerosol suspensions. Emerg Infect Dis. (2020) 26:2168–71. doi: 10.3201/eid2609.201806
23. Hamner L, Dubbel P, Capron I, Ross A, Jordan A, Lee J, et al. High SARS-CoV-2 attack rate following exposure at a choir practice - Skagit County, Washington, March (2020). MMWR Morb Mortal Wkly Rep. (2020) 69:606–10. doi: 10.15585/mmwr.mm6919e6
24. Lu J, Gu J, Li K, Xu C, Su W, Lai Z, et al. COVID-19 outbreak associated with air conditioning in restaurant, Guangzhou, China, (2020). Emerg Infect Dis. (2020) 26:1628–31. doi: 10.3201/eid2607.200764
25. Jang S, Han SH, Rhee JY. Cluster of coronavirus disease associated with fitness dance classes, South Korea. Emerg Infect Dis. (2020) 26:1917–20. doi: 10.3201/eid2608.200633
26. World Health Organization. Modes of Transmission of Virus Causing COVID-19: Implications for IPC Precaution Recommendations. Available online at: https://www.who.int/news-room/commentaries/detail/transmission-of-sars-cov-2-implications-for-infection-prevention-precautions (accessed September 30, 2020).
27. Corman VM, Landt O, Kaiser M, Molenkamp R, Meijer A, Chu DKW, et al. Detection of 2019 novel coronavirus (2019-nCoV) by real-time RT-PCR. Euro Surveill. (2020) 25:2000045. doi: 10.2807/1560-7917.ES.2020.25.3.2000045
28. To KK, Tsang OT, Leung WS, Tam AR, Wu TC, Lung DC, et al. Temporal profiles of viral load in posterior oropharyngeal saliva samples and serum antibody responses during infection by SARS-CoV-2: an observational cohort study. Lancet Infect Dis. (2020) 20:565–74. doi: 10.1016/S1473-3099(20)30196-1
29. Loeffelholz MJ, Tang YW. Laboratory diagnosis of emerging human coronavirus infections - the state of the art. Emerg Microbes Infect. (2020) 9:747–56. doi: 10.1080/22221751.2020.1745095
30. He F, Deng Y, Li W. Coronavirus disease 2019: what we know? J Med Virol. (2020) 92:719–25. doi: 10.1002/jmv.25766
31. Shen M, Zhou Y, Ye J, AL-maskri AA, Kang Y, Zeng S, et al. Recent advances and perspectives of nucleic acid detection for coronavirus. J Pharm Anal. (2020) 10:97–101. doi: 10.1016/j.jpha.2020.02.010
32. Sheridan C. Fast, portable tests come online to curb coronavirus pandemic. Nat Biotechnol. (2020) 38:515–18. doi: 10.1038/d41587-020-00010-2
33. World Health Organization (WHO). Laboratory Testing for 2019 Novel Coronavirus (2019-nCoV) in Suspected Human Cases. Geneva: WHO (2020). Available online at: https://www.who.int/publications/i/item/10665-331501 (accessed April 3, 2020).
34. Guo L, Ren L, Yang S, Xiao M, Chang, Yang F, et al. Profiling early humoral response to diagnose novel coronavirus disease (COVID-19). Clin Infect Dis. (2020) 71:778–85. doi: 10.1093/cid/ciaa310
35. Xiang F, Wang X, He X, Peng Z, Yang B, Zhang J, et al. Antibody detection and dynamic characteristics in patients with COVID-19. Clin Infect Dis. (2020). doi: 10.1093/cid/ciaa461. [Epub ahead of print].
36. Blanco-Melo D, Nilsson-Payant BE, Liu WC, Uhl S, Hoagland D, Møller R, et al. Imbalanced host response to SARS-CoV-2 drives development of COVID-19. Cell. (2020) 181:1036–45.e9. doi: 10.1016/j.cell.2020.04.026
37. Chu H, Chan JF, Wang Y, Yuen TT, Chai Y, Hou Y, et al. Comparative replication and immune activation profiles of SARS-CoV-2 and SARS-CoV in human lungs: an ex vivo study with implications for the pathogenesis of COVID-19. Clin Infect Dis. (2020) 71:1400–9. doi: 10.1093/cid/ciaa410
38. Thevarajan I, Nguyen TH, Koutsakos M, Druce J, Caly L, van de Sandt CE, et al. Breadth of concomitant immune responses prior to patient recovery: a case report of non-severe COVID-19. Nat Med. (2020) 26:453–5. doi: 10.1038/s41591-020-0819-2
39. Huang C, Wang Y, Li X, Ren L, Zhao J, Hu Y, et al. Clinical features of patients infected with 2019 novel coronavirus in Wuhan, China. Lancet. (2020) 395:497–506. doi: 10.1016/S0140-6736(20)30183-5
40. Yang Y, Shen C, Li J, Yuan J, Wei J, Huang F, et al. Plasma IP-10 and MCP-3 levels are highly associated with disease severity and predict the progression of COVID-19. J Allergy Clin Immunol. (2020) 146:119–27.e4. doi: 10.1016/j.jaci.2020.04.027
41. Li X, Xu S, Yu M, Wang K, Tao Y, Zhou Y, et al. Risk factors for severity and mortality in adult COVID-19 inpatients in Wuhan. J Allergy Clin Immunol. (2020) 146:110–18. doi: 10.1016/j.jaci.2020.04.006
42. Wu F, Zhao S, Yu B, Chen YM, Wang W, Song ZG, et al. A new coronavirus associated with human respiratory disease in China. Nature. (2020) 579:265–9. doi: 10.1038/s41586-020-2008-3
43. Zohar T, Alter G. Dissecting antibody-mediated protection against SARS-CoV-2. Nat Rev Immunol. (2020) 20:392–4. doi: 10.1038/s41577-020-0359-5
44. Selva KJ, van de Sandt CE, Lemke MM, Lee CY, Shoffner SK, Chua BY. Distinct systems serology features in children, elderly COVID patients. medRxiv. (2020). 05.11.20098459;
45. Tetro JA. Is COVID-19 receiving ADE from other coronaviruses? Microbes Infect. (2020) 22:72–3. doi: 10.1016/j.micinf.2020.02.006
46. Jiang S. Don't rush to deploy COVID-19 vaccines and drugs without sufficient safety guarantees. Nature. (2020) 579:321. doi: 10.1038/d41586-020-00751-9
47. Zhao J, Mangalam AK, Channappanavar R, Fett C, Meyerholz DK, Agnihothram S, et al. Airway memory CD4+ T cells mediate protective immunity against emerging respiratory coronaviruses. Immunity. (2016) 44:1379–91. doi: 10.1016/j.immuni.2016.05.006
48. Coleman CM, Sisk JM, Halasz G, Zhong J, Beck SE, Matthews KL, et al. CD8+ T cells and macrophages regulate pathogenesis in a mouse model of middle east respiratory syndrome. J Virol. (2017) 91:e01825–16. doi: 10.1128/JVI.01825-16
49. Zhao J, Alshukairi AN, Baharoon SA, Ahmed WA, Bokhari AA, Nehdi AM, et al. Recovery from the middle east respiratory syndrome is associated with antibody and T-cell responses. Sci Immunol. (2017) 2:eaan5393. doi: 10.1126/sciimmunol.aan5393
50. Wang SF, Chen KH, Chen M, Li WY, Chen YJ, Tsao CH, et al. Human-leukocyte antigen class I Cw 1502 and class II DR 0301 genotypes are associated with resistance to severe acute respiratory syndrome (SARS) infection. Viral Immunol. (2011) 24:421–6. doi: 10.1089/vim.2011.0024
51. Vabret N, Britton GJ, Gruber C, Hegde S, Kim J, Kuksin M, et al. Immunology of COVID-19: current state of the science. Immunity. (2020) 52:910–41. doi: 10.1016/j.immuni.2020.05.002
52. Zhang JJ, Dong X, Cao YY, Yuan YD, Yang YB, Yan YQ, et al. Clinical characteristics of 140 patients infected with SARS-CoV-2 in Wuhan, China. Allergy. (2020) 75:1730–41. doi: 10.1111/all.14238
53. Wang M, Cao R, Zhang L, Yang X, Liu J, Xu M, et al. Remdesivir and chloroquine effectively inhibit the recently emerged novel coronavirus (2019-nCoV) in vitro. Cell Res. (2020) 30:269–71. doi: 10.1038/s41422-020-0282-0
54. Lu X, Zhang L, Du H, Zhang J, Li YY, Qu J, et al. SARS-CoV-2 infection in Children. N Engl J Med. (2020) 282:1663–5. doi: 10.1056/NEJMc2005073
55. Ji LN, Chao S, Wang YJ, Li XJ, Mu XD, Lin MG, et al. Clinical features of pediatric patients with COVID-19: a report of two family cluster cases. World J Pediatr. (2020) 16:267–70. doi: 10.1007/s12519-020-00356-2
56. Cui Y, Tian M, Huang D, Wang X, Huang Y, Fan L, et al. A 55-day-old female infant infected with COVID 19: presenting with pneumonia, liver injury, and heart damage. J Infect Dis. (2020) 221:1775–81. doi: 10.1093/infdis/jiaa265
57. Liu W, Zhang Q, Chen J, Xiang R, Song H, Shu S, et al. Detection of covid-19 in children in early january 2020 in Wuhan, China. N Engl J Med. (2020) 382:1370–1. doi: 10.1056/NEJMc2003717
58. Shen K, Yang Y, Wang T, Zhao D, Jiang Y, Jin R, et al. Diagnosis, treatment, and prevention of 2019 novel coronavirus infection in children: experts' consensus statement. World J Pediatr. (2020) 16:223–31. doi: 10.1007/s12519-020-00344-6
59. Dong Y, Mo X, Hu Y, Qi X, Jiang F, Jiang Z, et al. Epidemiology of COVID-19 among children in China. Pediatrics. (2020) 145:e20200702. doi: 10.1542/peds.2020-0702
60. CDC COVID-19 Response Team. Coronavirus Disease 2019 in Children — United States, February 12–April 2,2020. MMWR Morb Mortal Wkly Rep. (2020) 69:422–6. doi: 10.15585/mmwr.mm6914e4
61. Parri N, Lenge M, Buonsenso D, Group CIiPEDCR. Children with covid-19 in pediatric emergency departments in Italy. N Engl J Med. (2020) 383:187–90. doi: 10.1056/NEJMc2007617
62. Tang A, Tong ZD, Wang HL, Dai YX, Li KF, Liu JN, et al. Detection of novel coronavirus by RT-PCR in stool specimen from asymptomatic child, China. Emerg Infect Dis. (2020) 26:1337–9. doi: 10.3201/eid2606.20.0301
63. Zachariah P, Johnson CL, Halabi KC, Ahn D, Sen AI, Fischer A, et al. Epidemiology, clinical features, and disease severity in patients with coronavirus disease 2019 (COVID-19) in a children's hospital in New York City, New York. JAMA Pediatr. 2020:e202430. doi: 10.1001/jamapediatrics.2020.2430
64. Chao JY, Derespina KR, Herold BC, Goldman DL, Aldrich M, Weingarten J, et al. Clinical characteristics and outcomes of hospitalized and critically Ill children and adolescents with coronavirus disease 2019 (COVID-19) at a tertiary care medical center in New York City. J Pediatr. (2020) 223:14–9.e2. doi: 10.1016/j.jpeds.2020.05.006
65. Shekerdemian LS, Mahmood NR, Wolfe KK, Riggs BJ, Ross CE, McKiernan CA, et al. Characteristics and outcomes of children with coronavirus disease 2019 (COVID-19) infection admitted to us and canadian pediatric intensive care units. JAMA Pediatr. (2020) 174:1–6. doi: 10.1001/jamapediatrics.2020.1948
66. Toubiana J, Poirault C, Corsia A, Bajolle F, Fourgeaud J, Angoulvant F, et al. Kawasaki-like multisystem inflammatory syndrome in children during the covid-19 pandemic in Paris, France: prospective observational study. BMJ. (2020) 369:m2094. doi: 10.1136/bmj.m2094
67. Chiotos K, Bassiri H, Behrens EM, Blatz AM, Chang J, Diorio C, et al. Multisystem inflammatory syndrome in children during the COVID-19 pandemic: a case series. J Pediatric Infect Dis Soc. (2020) 9:393–8. doi: 10.1093/jpids/piaa069
68. Deza Leon MP, Redzepi A, McGrath E, Abdel-Haq N, Shawaqfeh A, Sethuraman U, et al. COVID-19 associated pediatric multi-system inflammatory syndrome. J Pediatric Infect Dis Soc. (2020) 9:407–8. doi: 10.1093/jpids/piaa061
69. Verdoni L, Mazza A, Gervasoni A, Martelli L, Ruggeri M, Ciuffreda M, et al. An outbreak of severe Kawasaki-like disease at the Italian epicentre of the SARS-CoV-2 epidemic: an observational cohort study. Lancet. (2020) 395:1771–8. doi: 10.1016/S0140-6736(20)31103-X
70. Belhadjer Z, Méot M, Bajolle F, Khraiche D, Legendre A, Abakka S, et al. Acute heart failure in multisystem inflammatory syndrome in children (MIS-C) in the context of global SARS-CoV-2 pandemic. Circulation. (2020). doi: 10.1161/CIRCULATIONAHA.120.048360. [Epub ahead of print].
71. Riphagen S, Gomez X, Gonzalez-Martinez C, Wilkinson N, Theocharis P. Hyperinflammatory shock in children during COVID-19 pandemic. Lancet. (2020) 395:1607–8. doi: 10.1016/S0140-6736(20)31094-1
72. Centers for Disease Control and Prevention. Multisystem Inflammatory Syndrome in Children (MIS-C). Information for Healthcare Providers about Multisystem Inflammatory Syndrome in Children (MIS-C). Available online at: https://www.cdc.gov/mis-c/hcp/ (accessed June 11, 2020).
73. Chen H, Guo J, Wang C, Luo F, Yu X, Zhang W, et al. Clinical characteristics and intrauterine vertical transmission potential of COVID-19 infection in nine pregnant women: a retrospective review of medical records. Lancet. (2020) 395:809–15. doi: 10.1016/S0140-6736(20)30360-3
74. Fan C, Lei D, Fang C, Li C, Wang M, Liu Y, et al. Perinatal transmission of COVID-19 associated SARS-CoV-2: should we worry? Clin Infect Dis. (2020). doi: 10.1093/cid/ciaa226. [Epub ahead of print].
75. Liu Y, Chen H, Tang K, Guo Y. Clinical manifestations and outcome of SARS-CoV-2 infection during pregnancy. J Infect. (2020). doi: 10.1016/j.jinf.2020.02.028. [Epub ahead of print].
76. WHO. Report of the WHO-China Joint Mission on Coronavirus Disease 2019. (COVID-19) (2020). Available online at: https://www.who.int/docs/default-source/coronaviruse/who-china-joint-mission-on-covid-19-final-report.pdf (accessed March 22, 2020).
77. Mullins E, Evans D, Viner RM, O'Brien P, Morris E. Coronavirus in pregnancy and delivery: rapid review. Ultrasound Obstet Gynecol. (2020) 55:586–92. doi: 10.1101/2020.03.06.20032144
78. Baud D, Greub G, Favre G, Gengler C, Jaton K, Dubruc E, et al. Second-trimester miscarriage in a pregnant woman with SARS-CoV-2 infection. JAMA. (2020) 323:2198–200. doi: 10.1001/jama.2020.7233
79. Gagneur A, Dirson E, Audebert S, Vallet S, Legrand-Quillien MC, Laurent Y, et al. Materno-fetal transmission of human coronaviruses: a prospective pilot study. Eur J Clin Microbiol Infect Dis. (2008) 27:863–6. doi: 10.1007/s10096-008-0505-7
80. Wong SF, Chow KM, Leung TN, Ng WF, Ng TK, Shek CC, et al. Pregnancy and perinatal outcomes of women with severe acute respiratory syndrome. Am J Obstet Gynecol. (2004) 191:292–7. doi: 10.1016/j.ajog.2003.11.019
81. Alfaraj SH, Al-Tawfiq JA, Memish ZA. Middle east respiratory syndrome coronavirus (MERS-CoV) infection during pregnancy: report of two cases & review of the literature. J Microbiol Immunol Infect. (2019) 52:501–3. doi: 10.1016/j.jmii.2018.04.005
82. Zhu H, Wang L, Fang C, Peng S, Zhang L, Chang G, et al. Clinical analysis of 10 neonates born to mothers with 2019-nCoV pneumonia. Transl Pediatr. (2020) 9:51–60. doi: 10.21037/tp.2020.02.06
83. Wang L, Shi Y, Xiao T, Fu J, Feng X, Mu D, et al. Chinese expert consensus on the perinatal and neonatal management for the prevention and control of the 2019 novel coronavirus infection (First edition). Ann Transl Med. (2020) 8:47. doi: 10.21037/atm.2020.02.20
84. Zeng H, Xu C, Fan J, Tang Y, Deng Q, Zhang W, et al. Antibodies in infants born to mothers with COVID-19 pneumonia. JAMA. (2020) 323:1848–9. doi: 10.1001/jama.2020.4861
85. Dong L, Tian J, He S, Zhu C, Wang J, Liu C, et al. Possible vertical transmission of SARS-CoV-2 from an infected mother to her newborn. JAMA. (2020) 323:1846–8. doi: 10.1001/jama.2020.4621
86. Zeng L, Xia S, Yuan W, Yan K, Xiao F, Shao J, et al. Neonatal early-onset infection with SARS-CoV-2 in 33 neonates born to mothers with COVID-19 in Wuhan, China. JAMA Pediatr. (2020) 174:722–25. doi: 10.1001/jamapediatrics.2020.0878
87. Abu-Raya B, Giles M, Sadarangani M. Vertical transmission of SARS-CoV-2 from the mother to the infant: intrauterine vs. post-natal infection. JAMA Pediatrics. (2020). 37:769–72. doi: 10.1055/s-0040-1712457
88. Dashraath P, Wong JLJ, Lim MXK, Lim LM, Li S, Biswas A, et al. Coronavirus disease 2019 (COVID-19) pandemic and pregnancy. Am J Obstet Gynecol. (2020) 222:521–31. doi: 10.1016/j.ajog.2020.03.021
89. Schwartz DA. An analysis of 38 pregnant women with COVID-19, their newborn infants, and maternal-fetal transmission of SARS-CoV-2: maternal coronavirus infections and pregnancy outcomes. Arch Pathol Lab Med. (2020). doi: 10.5858/arpa.2020-0901-SA. [Epub ahead of print].
91. Lu Q, Shi Y. Coronavirus disease (COVID-19) and neonate: what neonatologist need to know. J Med Virol. (2020) 92:564–7. doi: 10.1002/jmv.25740
92. Vaira LA, Salzano G, Deiana G, De Riu G. Anosmia and ageusia: common findings in COVID-19 patients. Laryngoscope. (2020) 15:10.1002/lary.28753. doi: 10.1002/lary.28753
93. Lechien JR, Chiesa-Estomba CM, Place S, Van Laethem Y, Cabaraux P, Mat Q, et al. Clinical and epidemiological characteristics of 1,420 European patients with mild-to-moderate coronavirus disease 2019. J Intern Med. (2020) 30:10.1111/joim.13086. doi: 10.1111/joim.13089
94. Richardson S, Hirsch JS, Narasimhan M, Crawford JM, McGinn T, Davidson KW, et al. Presenting characteristics, comorbidities, and outcomes among 5700 patients hospitalized with COVID-19 in the New York city area. JAMA. (2020) 323:2052–59. doi: 10.1001/jama.2020.6775
95. Guan WJ, Ni ZY, Hu Y, Liang WH, Ou CQ, He JX, et al. Clinical characteristics of coronavirus disease 2019 in China. N Engl J Med. (2020) 80:656–65. doi: 10.1101/2020.02.06.20020974
96. Grasselli G, Zangrillo A, Zanella A, Antonelli M, Cabrini L, Castelli A, et al. Baseline characteristics and outcomes of 1591 patients infected with SARS-CoV-2 admitted to ICUs of the lombardy region, Italy. JAMA. (2020) 323:1574–81. doi: 10.1001/jama.2020.5394
97. Mehra MR, Desai SS, Kuy S, Henry TD, Patel AN. Cardiovascular disease, drug therapy, and mortality in covid-19. N Engl J Med. (2020) 382:2582. doi: 10.1056/NEJMoa2007621
98. Imam Z, Odish F, Gill I, O'Connor D, Armstrong J, Vanood A, et al. Older age and comorbidity are independent mortality predictors in a large cohort of 1305. COVID-19 patients in Michigan, United States. J Intern Med. (2020) 288:469–76. doi: 10.1111/joim.13119
99. Jackson DJ, Busse WW, Bacharier LB, Kattan M, O'Connor GT, Wood RA, et al. Association of respiratory allergy, asthma and expression of the SARS-CoV-2 receptor, ACE2. J Allergy Clin Immunol. (2020) 146:203–6.e3. doi: 10.1016/j.jaci.2020.04.009
100. Jin JM, Bai P, He W, Wu F, Liu XF, Han DM, et al. Gender differences in patients with COVID-19: focus on severity and mortality. Front Public Health. (2020) 8:152. doi: 10.3389/fpubh.2020.00152
101. Pérez-López F, Tajada M, Savirón-Cornudella R, Sánchez-Prieto M, Chedraui P, Terán E. Coronavirus disease 2019 and gender-related mortality in European countries: a meta-analysis. Maturitas. (2020) 141:59–62. doi: 10.1016/j.maturitas.2020.06.017
102. Wu P, Duan F, Luo C, Liu Q, Qu X, Liang L, et al. Characteristics of ocular findings of patients with coronavirus disease 2019 (COVID-19) in Hubei Province, China. JAMA Ophthalmol. (2020) 138:575–8. doi: 10.1001/jamaophthalmol.2020.1291
103. Toscano G, Palmerini F, Ravaglia S, Ruiz L, Invernizzi P, Cuzzoni MG, et al. Guillain-Barré Syndrome Associated with SARS-CoV-2. N Engl J Med. (2020) 17:NEJMc2009191. doi: 10.1056/NEJMc2009191
104. Oxley TJ, Mocco J, Majidi S, Kellner CP, Shoirah H, Singh IP, et al. Large-vessel stroke as a presenting feature of covid-19 in the young. N Engl J Med. (2020) 382:e60. doi: 10.1056/NEJMc2009787
105. Onder G, Rezza G, Brusaferro S. Case-fatality rate and characteristics of patients dying in relation to COVID-19 in Italy. JAMA. (2020) 323:1775–6. doi: 10.1001/jama.2020.4683
106. Ferrucci L, Corsi A, Lauretani F, Bandinelli S, Bartali B, Taub DD, et al. The origins of age-related proinflammatory state. Blood. (2005) 105:2294–9. doi: 10.1182/blood-2004-07-2599
107. Giwa AL, Desai A, Duca A. Novel 2019 coronavirus SARS-CoV-2 (COVID-19): an updated overview for emergency clinicians. Emerg Med Pract. (2020) 21:1–28.
108. Palmer K, Marengoni A, Forjaz MJ, Jureviciene E, Laatikainen T, Mammarella F, et al. Multimorbidity care model: recommendations from the consensus meeting of the joint action on chronic diseases and promoting healthy ageing across the life cycle (JA-CHRODIS). Health Policy. (2018) 122:4–11. doi: 10.1016/j.healthpol.2017.09.006
109. Morandi A, Davis D, Bellelli G, Arora RC, Caplan GA, Kamholz B, et al. The diagnosis of delirium superimposed on dementia: an emerging challenge. J Am Med Dir Assoc. (2017) 18:12–8. doi: 10.1016/j.jamda.2016.07.014
110. COVID-19 rapid guideline: critical care NICE guideline published: 20 March 2020 Available online at: http://www.nice.org.uk/guidance/ng159 (accessed March 25, 2020).
111. White DB, Lo B. A framework for rationing ventilators and critical care beds during the COVID-19 pandemic. JAMA. (2020) 323:1773–4. doi: 10.1001/jama.2020.5046
112. Statement of the EuGMS Executive Board on the COVID-19 epidemic. EUGMS Website. Available online at: https://www.eugms.org/news/read/article/489.html (accessed March 25, 2020).
113. BSTI NHSE COVID-19 Radiology Decision Support Tool. Available online at: https://www.bsti.org.uk/covid-19-resources/covid-19-nhse-bsti-imaging-decision-tool/ (accessed March 23, 2020).
114. Shi H, Han X, Jiang N, Cao Y, Alwalid O, Gu J, et al. Radiological findings from 81 patients with COVID-19 pneumonia in Wuhan, China: a descriptive study. Lancet Infect Dis. (2020) 20:425–34. doi: 10.1016/S1473-3099(20)30086-4
115. Bai HX, Hsieh B, Xiong Z, Halsey K, Choi JW, Tran TML, et al. Performance of radiologists in differentiating COVID-19 from viral pneumonia on chest CT. Radiology. (2020) 296:E46–54. doi: 10.1148/radiol.2020200823
116. Wu J, Wu X, Zeng W, Guo D, Fang Z, Chen L, et al. Chest CT findings in patients with corona virus disease 2019 and its relationship with clinical features. Invest Radiol. (2020) 55:257–61. doi: 10.1097/RLI.0000000000000670
117. Ai T, Yang Z, Hou H, Zhan C, Chen C, Lv W, et al. Correlation of chest CT and RT-PCR testing in coronavirus disease 2019 (COVID-19) in China: a report of 1014 cases. Radiology. (2020) 296:E32–E40. doi: 10.1148/radiol.2020200642
118. Huang P, Liu T, Huang L, Liu H, Lei M, Xu W, et al. Use of chest CT in combination with negative RT-PCR assay for the 2019 novel coronavirus but high clinical suspicion. Radiology. (2020) 295:22–3. doi: 10.1148/radiol.2020200330
119. Li H, Zhou Y, Zhang M, Wang H, Zhao Q, Liu J. Updated approaches against SARS-CoV-2. Antimicrob Agents Chemother. (2020) 64:e00483–20. doi: 10.1128/AAC.00483-20
120. Blaising J, Polyak SJ, Pécheur EI. Arbidol as a broad-spectrum antiviral: an update. Antiviral Res. (2014) 107:84–94. doi: 10.1016/j.antiviral.2014.04.006
121. Savarino A, Boelaert JR, Cassone A, Majori G, Cauda R. Effects of chloroquine on viral infections: an old drug against today's diseases? Lancet Infect Dis. (2003) 3:722–7. doi: 10.1016/S1473-3099(03)00806-5
122. Zhan X, Dowell S, Shen Y, Lee DL. Chloroquine to fight COVID-19: a consideration of mechanisms and adverse effects? Heliyon. (2020) 6:e04900. doi: 10.1016/j.heliyon.2020.e04900
123. Satarker S, Ahuja T, Banerjee M, E VB, Dogra S, Agarwal T, et al. Hydroxychloroquine in COVID-19: potential mechanism of action against SARS-CoV-2. Curr Pharmacol Rep. (2020) 24:1–9. doi: 10.1007/s40495-020-00231-8
124. Richardson P, Griffin I, Tucker C, Smith D, Oechsle O, Phelan A, et al. Baricitinib as potential treatment for 2019-nCoV acute respiratory disease. Lancet. (2020) 395:e30–1. doi: 10.1016/S0140-6736(20)30304-4
125. Morse JS, Lalonde T, Xu S, Liu WR. Learning from the past: possible urgent prevention and treatment options for severe acute respiratory infections caused by 2019-nCoV. Chembiochem. (2020) 21:730–8. doi: 10.1002/cbic.202000047
126. Gordon CJ, Tchesnokov EP, Feng JY, Porter DP, Gotte M. The antiviral compound remdesivir potently inhibits RNA-dependent RNA polymerase from middle east respiratory syndrome coronavirus. J Biol Chem. (2020) 295:4773–9. doi: 10.1074/jbc.AC120.013056
127. Abraham GM, Morton JB, Saravolatz LD. Baloxavir: a novel antiviral agent in the treatment of influenza. Clin Infect Dis. (2020) ciaa107. doi: 10.1093/cid/ciaa107
128. Wang RR, Yang QH, Luo RH, Peng YM, Dai SX, Zhang XJ, et al. Azvudine, a novel nucleoside reverse transcriptase inhibitor showed good drug combination features and better inhibition on drug-resistant strains than lamivudine in vitro. PLoS ONE. (2014) 9:e105617. doi: 10.1371/journal.pone.0105617
129. Shiraki K, Daikoku T. Favipiravir, an anti-influenza drug against life-threatening RNA virus infections. Pharmacol Ther. (2020) 209:107512. doi: 10.1016/j.pharmthera.2020.107512
130. Furuta Y, Gowen BB, Takahashi K, Shiraki K, Smee DF, Barnard DL. Favipiravir (T-705), a novel viral RNA polymerase inhibitor. Antiviral Res. (2013) 100:446–54. doi: 10.1016/j.antiviral.2013.09.015
131. Jordan PC, Stevens SK, Deval J. Nucleosides for the treatment of respiratory RNA virus infections. Antivir Chem Chemother. (2018) 26:2040206618764483. doi: 10.1177/2040206618764483
132. Cavalli G, Dinarello CA. Anakinra therapy for non-cancer inflammatory diseases. Front Pharmacol. (2018) 9:1157. doi: 10.3389/fphar.2018.01157
133. Hashizume M, Tan SL, Takano J, Ohsawa K, Hasada I, Hanasaki A, et al. Tocilizumab, a humanized anti-IL-6R antibody, as an emerging therapeutic option for rheumatoid arthritis: molecular and cellular mechanistic insights. Int Rev Immunol. (2015) 34:265–79. doi: 10.3109/08830185.2014.938325
134. Weng D, Wu Q, Chen XQ, Du YK, Chen T, Li H, et al. Azithromycin treats diffuse panbronchiolitis by targeting T cells via inhibition of mTOR pathway. Biomed Pharmacother. (2019) 110:440–8. doi: 10.1016/j.biopha.2018.11.090
135. Ben-Zvi I, Kivity S, Langevitz P, Shoenfeld Y. Hydroxychloroquine: from malaria to autoimmunity. Clin Rev Allergy Immunol. (2012) 42:145–53. doi: 10.1007/s12016-010-8243-x
136. Jang CH, Choi JH, Byun MS, Jue DM. Chloroquine inhibits production of TNF-alpha, IL-1beta and IL-6 from lipopolysaccharide-stimulated human monocytes/macrophages by different modes. Rheumatology. (2006) 45:703–10. doi: 10.1093/rheumatology/kei282
137. Jamilloux Y, El Jammal T, Vuitton L, Gerfaud-Valentin M, Kerever S, Sève P. JAK inhibitors for the treatment of autoimmune and inflammatory diseases. Autoimmun Rev. (2019) 18:102390. doi: 10.1016/j.autrev.2019.102390
138. Kubo S, Nakayamada S, Tanaka Y. Baricitinib for the treatment of rheumatoid arthritis. Expert Rev Clin Immunol. (2016) 12:911–9. doi: 10.1080/1744666X.2016.1214576
139. Kubo S, Nakayamada S, Tanaka Y. Baricitinib for the treatment of rheumatoid arthritis and systemic lupus erythematosus: a 2019 update. Expert Rev Clin Immunol. (2019) 15:693–700. doi: 10.1080/1744666X.2019.1608821
140. Cao B, Wang Y, Wen D, Liu W, Wang J, Fan G, et al. A trial of lopinavir-ritonavir in adults hospitalized with severe covid-19. N Engl J Med. (2020) 382:1787–99. doi: 10.1056/NEJMoa2001282
141. Hung IF, Lung KC, Tso EY, Liu R, Chung TW, Chu MY, et al. Triple combination of interferon beta-1b, lopinavir-ritonavir, and ribavirin in the treatment of patients admitted to hospital with COVID-19: an open-label, randomised, phase 2 trial. Lancet. (2020) 395:1695–704. doi: 10.1016/S0140-6736(20)31042-4
142. Yao X, Ye F, Zhang M, Cui C, Huang B, Niu P, et al. In vitro antiviral activity and projection of optimized dosing design of hydroxychloroquine for the treatment of severe acute respiratory syndrome coronavirus 2 (SARS-CoV-2). Clin Infect Dis. (2020) 9:ciaa237. doi: 10.1093/cid/ciaa237
143. Infectious Diseases Society of America. Infectious Diseases Society of America Guidelines on the Treatment and Management of Patients with COVID-19 Infection. Available online at: https://www.idsociety.org/COVID19guidelines (accessed October 16, 2020).
144. Geleris J, Sun Y, Platt J, Zucker J, Baldwin M, Hripcsak G, et al. Observational study of hydroxychloroquine in hospitalized patients with covid-19. N Engl J Med. (2020) 382:2411–18. doi: 10.1056/NEJMoa2012410
145. Boulware DR, Pullen MF, Bangdiwala AS, Pastick KA, Lofgren SM, Okafor EC, et al. A randomized trial of hydroxychloroquine as postexposure prophylaxis for covid-19. N Engl J Med. (2020) 383:517–25. doi: 10.1056/NEJMoa2016638
146. Zhang JJY, Lee KS, Ang LW, Leo YS, Young BE. Risk factors of severe disease and efficacy of treatment in patients infected with COVID-19: a systematic review, meta-analysis and meta-regression analysis. Clin Infect Dis. (2020) ciaa576. doi: 10.1093/cid/ciaa576
147. Gabriels J, Saleh M, Chang D, Epstein LM. Inpatient use of mobile continuous telemetry for COVID-19 patients treated with hydroxychloroquine and azithromycin. HeartRhythm Case Rep. (2020) 6:241–3. doi: 10.1016/j.hrcr.2020.03.017
148. Mercuro NJ, Yen CF, Shim DJ, Maher TR, McCoy CM, Zimetbaum PJ, et al. Risk of QT interval prolongation associated with use of hydroxychloroquine with or without concomitant azithromycin among hospitalized patients testing positive for coronavirus disease 2019 (COVID-19). JAMA Cardiol. (2020) 5:1036–41. doi: 10.1001/jamacardio.2020.1834
149. Bessière F, Roccia H, Delinière A, Charrière R, Chevalier P, Argaud L, et al. Assessment of QT intervals in a case series of patients with coronavirus disease 2019 (COVID-19) infection treated with hydroxychloroquine alone or in combination with azithromycin in an intensive care unit. JAMA Cardiol. (2020). doi: 10.1001/jamacardio.2020.1787. [Epub ahead of print].
150. Borba MGS, Val FFA, Sampaio VS, Alexandre MAA, Melo GC, Brito M, et al. Effect of high vs low doses of chloroquine diphosphate as adjunctive therapy for patients hospitalized with severe acute respiratory syndrome coronavirus 2 (SARS-CoV-2) infection: a randomized clinical trial. JAMA Netw Open. (2020) 3:e208857. doi: 10.1001/jamanetworkopen.2020.8857
151. Sheahan TP, Sims AC, Graham RL, Menachery VD, Gralinski LE, Case JB, et al. Broad-spectrum antiviral GS-5734 inhibits both epidemic and zoonotic coronaviruses. Sci Transl Med. (2017) 9:eaal3653. doi: 10.1126/scitranslmed.aal3653
152. Lo MK, Jordan R, Arvey A, Sudhamsu J, Shrivastava-Ranjan P, Hotard AL, et al. GS-5734 and its parent nucleoside analog inhibit Filo-, Pneumo-, and paramyxoviruses. Sci Rep. (2017) 7:43395. doi: 10.1038/srep43395
153. Grein J, Ohmagari N, Shin D, Diaz G, Asperges E, Castagna A, et al. Compassionate use of remdesivir for patients with severe covid-19. N Engl J Med. (2020) 382:2327–36. doi: 10.1056/NEJMoa2007016
154. Wang Y, Zhang D, Du G, Du R, Zhao J, Jin Y, et al. Remdesivir in adults with severe COVID-19: a randomised, double-blind, placebo-controlled, multicentre trial. Lancet. (2020) 395:1569–78. doi: 10.1016/S0140-6736(20)31022-9
155. Goldman JD, Lye DCB, Hui DS, Marks KM, Bruno R, Montejano R, et al. Remdesivir for 5 or 10 days in patients with severe covid-19. N Engl J Med. (2020). doi: 10.1056/NEJMoa2015301. [Epub ahead of print].
156. Beigel JH, Tomashek KM, Dodd LE, Mehta AK, Zingman BS, Kalil AC, et al. Remdesivir for the treatment of covid-19 - preliminary report. N Engl J Med. (2020). doi: 10.1056/NEJMoa2007764. [Epub ahead of print].
157. McCreary EK, Pogue JM. Coronavirus disease 2019 treatment: a review of early and emerging options. Open Forum Infect Dis. (2020) 7:ofaa105. doi: 10.1093/ofid/ofaa105
158. Pontali E, Volpi S, Antonucci G, Castellaneta M, Buzzi D, Tricerri F, et al. Safety and efficacy of early high-dose IV anakinra in severe COVID-19 lung disease. J Allergy Clin Immunol. (2020) 146:213–15. doi: 10.1016/j.jaci.2020.05.002
159. Scala S, Pacelli R. Fighting the host reaction to SARS-COv-2 in critically III patients: the possible contribution of off-label drugs. Front Immunol. (2020) 11:1201. doi: 10.3389/fimmu.2020.01201
160. Li G, De Clercq E. Therapeutic options for the 2019 novel coronavirus (2019-nCoV). Nat Rev Drug Discov. (2020) 19:149–50. doi: 10.1038/d41573-020-00016-0
161. Arshad U, Pertinez H, Box H, Tatham L, Rajoli RK, Curley P, et al. Prioritisation of anti-SARS-Cov-2 drug repurposing opportunities based on plasma and target site concentrations derived from their established human pharmacokinetics. Clin Pharmacol Ther. (2020) 108:775–90. doi: 10.1101/2020.04.16.20068379
162. Dara M, Sotgiu G, Reichler MR, Chiang CY, Chee CBE, Migliori BG. New diseases and old threats: lessons from tuberculosis for the COVID-19 response. Int J Tuberc Lung Dis. (2020) 24:544–5. doi: 10.5588/ijtld.20.0151
163. Wu Z, McGoogan JM. Characteristics of and important lessons from the coronavirus disease 2019 (Covid-19) outbreak in china: summary of a report of 72 314 cases from the Chinese center for disease control and prevention. JAMA. (2020) 323:1239–42. doi: 10.1001/jama.2020.2648
164. Wu C, Chen X, Cai Y, Xia J, Zhou X, Xu S, et al. Risk factors associated with acute respiratory distress syndrome and death in patients with coronavirus disease 2019 pneumonia in Wuhan, China. JAMA Intern Med. (2020) 180:1–11. doi: 10.1001/jamainternmed.2020.0994
165. Alhazzani W, Hylander Møller M, Arabi YM, Loeb M, Ng Gong M, Fan E, et al. Surviving Sepsis Campaign: Guidelines on the Management of Critically III Adults with Coronavirus Disease 2019 (COVID-19). Available online at: https://www.esicm.org/ssc-covid19-guidelines/ (accessed October 16, 2020).
166. Clinical management of severe acute respiratory infection when novel coronavirus (nCoV) infection is suspected. Available online at: https://www.who.int/publications-detail/clinical-management-of-severe-acute-respiratory-infection-when-novel-coronavirus-(ncov)-infection-is-suspected (accessed March 26, 2020).
167. World Health Organization. Coronavirus Disease 2019 (COVID-19) Situation Report – 56. Available online at: https://www.who.int/docs/default-source/coronaviruse/situation-reports/20200316-sitrep-56-covid-19.pdf?sfvrsn=9fda7db2_2 (accessed April 7, 2020).
168. Linton NM, Kobayashi T, Yang Y, Hayashi K, Akhmetzhanov AR, Jung SM, et al. Incubation period and other epidemiological characteristics of 2019. Novel coronavirus infections with right truncation: a statistical analysis of publicly available case data. J Clin Med. (2020) 9:538. doi: 10.1101/2020.01.26.20018754
169. Spellberg B, Haddix M, Lee R, Butler-Wu S, Holtom P, Yee H, et al. Community prevalence of SARS-CoV-2 among patients with influenzalike illnesses presenting to a los angeles medical center in march 2020. JAMA. (2020) 323:1966–7. doi: 10.1001/jama.2020.4958
170. Migliori GB, Nardell E, Yedilbayev A, D'Ambrosio L, Centis R, Tadolini M, et al. Reducing tuberculosis transmission: a consensus document from the world health organization regional office for Europe. Eur Respir J. (2019) 53:1900391. doi: 10.1183/13993003.00391-2019
171. Bai Y, Yao L, Wei T, Tian F, Jin DY, Chen L, et al. Presumed asymptomatic carrier transmission of COVID-19. JAMA. (2020) 323:1406–7. doi: 10.1001/jama.2020.2565
172. Zou L, Ruan F, Huang M, Liang L, Huang H, Hong Z, et al. SARS-CoV-2 viral load in upper respiratory specimens of infected patients. N Engl J Med. (2020) 382:1177–9. doi: 10.1056/NEJMc2001737
173. Leung CC, Lam TH, Cheng KK. Mass masking in the COVID-19 epidemic: people need guidance. Lancet. (2020) 395:945. doi: 10.1016/S0140-6736(20)30520-1
174. Leung CC, Lam TH, Cheng KK. Let us not forget the mask in our attempts to stall the spread of COVID-19. Int J Tuberc Lung Dis. (2020) 24:364–6. doi: 10.5588/ijtld.20.0124
175. THE NATIONAL ACADEMIC PRESS. Rapid Expert Consultation Update on SARS-CoV-2 Surface Stability and Incubation for the COVID-19 Pandemic (March 27, 2020). Available online at: https://www.nap.edu/catalog/25763/rapid-expert-consultation-update-on-sars-cov-2-surface-stability-and-incubation-for-the-covid-19-pandemic-march-27-2020 (accessed April 6, 2020).
176. MacIntyre CR, Seale H, Dung TC, Hien NT, Nga PT, Chughtai AA, et al. A cluster randomised trial of cloth masks compared with medical masks in healthcare workers. BMJ Open. (2015) 5:e006577. doi: 10.1136/bmjopen-2014-006577
177. Davies A, Thompson KA, Giri K, Kafatos G, Walker J, Bennett A. Testing the efficacy of homemade masks: would they protect in an influenza pandemic? Disaster Med Public Health Prep. (2013) 7:413–8. doi: 10.1017/dmp.2013.43
178. World Health Organization. Advice on the Use of Masks in the Context of COVID-19: Interim Guidance, 5 June 2020. Available online at: https://apps.who.int/iris/handle/10665/332293 (accessed June 12, 2020).
179. World Health Organization. Country and Technical Guidance -Coronavirus Disease (COVID-19). Available online at: https://www.who.int/emergencies/diseases/novel-coronavirus-2019/technical-guidance (accessed April 7, 2020).
180. DECRETO-LEGGE 25 marzo 2020. Misure Urgenti per Fronteggiare l'emergenza Epidemiologica da COVID-19. (20G00035) Available online at: https://www.gazzettaufficiale.it/eli/id/2020/03/25/20G00035/sg (accessed April 7, 2020).
181. Hans Kluge, WHO website. Statement – Every Country Needs to Take Boldest Actions to Stop COVID-19. Available online at: http://www.euro.who.int/en/about-us/regional-director/statements/statement-every-country-needs-to-take-boldest-actions-to-stop-covid-19?utm_source=WHO%2FEurope+mailing+list&utm_campaign=93bb011baf-EMAIL_CAMPAIGN_2020_03_16_10_53&utm_medium=email&utm_term=0_60241f4736-93bb011baf-100418457 (accessed March 18, 2020).
182. Non-Pharmaceutical Public Health Measures for Mitigating the Risk and Impact of Epidemic and Pandemic Influenza. WHO (2019).
184. Chen S, Yang J, Yang W, Wang C, Bärnighausen T. COVID-19 control in China during mass population movements at New Year. Lancet. (2020) 395:764–6. doi: 10.1016/S0140-6736(20)30421-9
185. Prem K, Liu Y, Russell TW, Kucharski AJ, Eggo RM, Davies N, et al. The effect of control strategies to reduce social mixing on outcomes of the COVID-19 epidemic in Wuhan, China: a modelling study. Lancet Public Health. (2020) 5:261–70. doi: 10.1101/2020.03.09.20033050
186. Policy Paper: Coronavirus Action Plan: A Guide to What You Can Expect Across the UK. (2020) Available online at: https://www.gov.uk/government/publications/coronavirus-action-plan/coronavirus-action-plan-a-guide-to-what-you-can-expect-across-the-uk (accessed March 24, 2020).
187. Ferguson NM, Laydon D, Nedjati-Gilani G, Imai N, Ainslie K, Baguelin M, et al. Impact of Non-Pharmaceutical Interventions (NPIs) to Reduce COVID- 19 Mortality and Healthcare Demand. London: Imperial College COVID-19 Response Team (2020).
188. Editorial. COVID-19: Learning from experience. Lancet. (2020) 395:1011. doi: 10.1016/S0140-6736(20)30686-3
189. Draft Landscape of COVID-19 Candidate Vaccines. Available online at: https://www.who.int/who-documents-detail/draft-landscapeof-covid-19-candidate-vaccines (accessed May 26, 2020).
190. Zhu FC, Li YH, Guan XH, Hou LH, Wang WJ, Li JX, et al. Safety, tolerability, and immunogenicity of a recombinant adenovirus type-5 vectored COVID-19 vaccine: a dose-escalation, open-label, non-randomised, first-in-human trial. Lancet. (2020) 395:1845–54. doi: 10.1016/S0140-6736(20)31208-3
191. Roper RL, Rehm KE. SARS vaccines: where are we? Expert Rev Vaccines. (2009) 8:887–98. doi: 10.1586/erv.09.43
192. Yip MS, Leung HL, Li PH, Cheung CY, Dutry I, Li D, et al. Antibody-dependent enhancement of SARS coronavirus infection and its role in the pathogenesis of SARS. Hong Kong Med J. (2016) 22:25–31.
Keywords: COVID-19, coronavirus, intensive care management, prevention, workplace safety, infection control, SARS-CoV-2, physical distancing
Citation: Abu-Raya B, Migliori GB, O'Ryan M, Edwards K, Torres A, Alffenaar J-W, Märtson A-G, Centis R, D'Ambrosio L, Flanagan K, Hung I, Lauretani F, Leung CC, Leuridan E, Maertens K, Maggio MG, Nadel S, Hens N, Niesters H, Osterhaus A, Pontali E, Principi N, Rossato Silva D, Omer S, Spanevello A, Sverzellati N, Tan T, Torres-Torreti JP, Visca D and Esposito S (2020) Coronavirus Disease-19: An Interim Evidence Synthesis of the World Association for Infectious Diseases and Immunological Disorders (Waidid). Front. Med. 7:572485. doi: 10.3389/fmed.2020.572485
Received: 02 July 2020; Accepted: 12 October 2020;
Published: 30 October 2020.
Edited by:
Zisis Kozlakidis, International Agency for Research on Cancer (IARC), FranceReviewed by:
Xiaodong Zhang, Jilin University, ChinaIo Cheong, Shanghai Jiao Tong University, China
Copyright © 2020 Abu-Raya, Migliori, O'Ryan, Edwards, Torres, Alffenaar, Märtson, Centis, D'Ambrosio, Flanagan, Hung, Lauretani, Leung, Leuridan, Maertens, Maggio, Nadel, Hens, Niesters, Osterhaus, Pontali, Principi, Rossato Silva, Omer, Spanevello, Sverzellati, Tan, Torres-Torreti, Visca and Esposito. This is an open-access article distributed under the terms of the Creative Commons Attribution License (CC BY). The use, distribution or reproduction in other forums is permitted, provided the original author(s) and the copyright owner(s) are credited and that the original publication in this journal is cited, in accordance with accepted academic practice. No use, distribution or reproduction is permitted which does not comply with these terms.
*Correspondence: Susanna Esposito, c3VzYW5uYS5lc3Bvc2l0byYjeDAwMDQwO3VuaW1pLml0
†These authors share first authorship