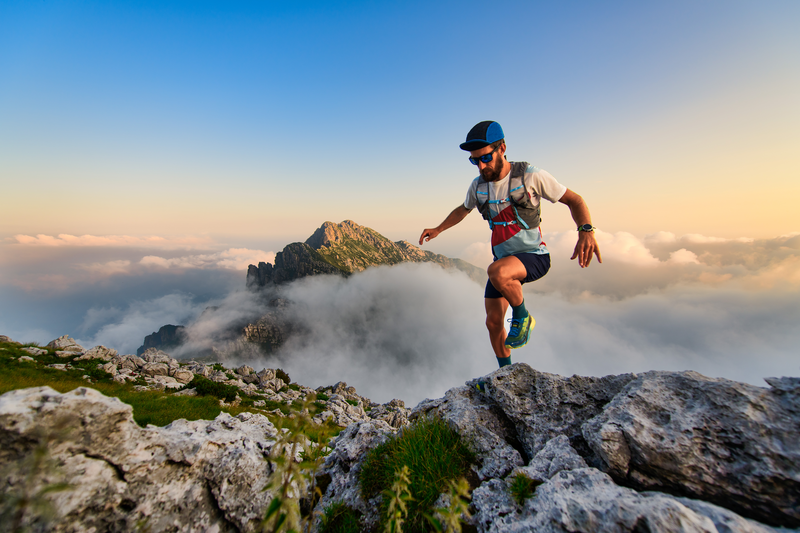
95% of researchers rate our articles as excellent or good
Learn more about the work of our research integrity team to safeguard the quality of each article we publish.
Find out more
REVIEW article
Front. Med. , 06 August 2020
Sec. Nephrology
Volume 7 - 2020 | https://doi.org/10.3389/fmed.2020.00393
Anemia is a complication of chronic kidney disease (CKD), primarily due to insufficient secretion of erythropoietin (EPO) by the kidney. Erythropoiesis-stimulating agents (ESAs) are used to treat anemia associated with chronic kidney disease. A poor response to ESAs has been associated with inflammation. Inflammation can affect erythrocytes and its production in many ways, but mainly through the inflammatory cytokine IL-6 to stimulate the synthesis of hepcidin in the liver. Hepcidin causes iron insufficiency, which causes erythrocytes to fail to mature normally. In addition, inhibition of bone marrow erythroid precursor cells by inflammatory cytokines such as IL-1 and TNF-α also affects bone marrow hematopoiesis. These cytokines are also important factors leading to EPO resistance. Roxadustat is a new drug for the treatment of renal anemia. In addition to promoting the production of EPO, clinical trials have shown that it can significantly reduce hepcidin and can potentially be used for the treatment of inflammation-induced anemia in CKD.
Anemia is one of the major complications of chronic kidney disease (1, 2), which seriously influences the prognosis of patients. The more severe the anemia, the higher the risk of mortality, cardiovascular events and hospitalization (3, 4). The main cause of renal anemia is renal damage, which affects the secretion of erythropoietin. Therefore, when ESAs appeared, the treatment of renal anemia has been improved, with improved quality of life of patients and improved patient survival.
Approximately 5 to 10% of patients with renal anemia have EPO resistance (5), which leads to the need for higher doses of EPO to achieve hemoglobin levels, with cardiovascular events, mortality and other adverse event gradually increasing (6–8). In earlier studies, plasma C-reactive protein (CRP) was usually higher in patients with ESA resistance (9, 10), and many inflammatory cytokines such as IL-6 and tumors necrosis factor-α (TNF-α) were elevated in plasma and highly related to with ESA resistance (11–14). Inflammation may be an important reasons of ESA resistance (15, 16).
Inflammation is another cause of renal anemia (1, 2). Cross-sectional data from 7,389 adult outpatients suggested that an increase in plasma CRP concentration was associated with a decrease in hemoglobin (Hb), and as CKD progresses, inflammation may gradually increase (17). Inflammation has been found in ~35 to 65% of hemodialysis patients (18). Costa et al. found serum CRP and neutrophils were significantly elevated in hemodialysis patients with CKD (19). Inflammatory anemia is a common feature of patients in patients with advanced chronic kidney disease and an established risk factor for end-stage renal disease (20). In patients with inflammatory anemia, ESA do not achieve satisfactory results, and an alternative therapy is needed, especially in patients with advanced chronic kidney disease.
Roxadustat is a new oral anti-renal anemia medication developed by FibroGen. It was approved for marketing in China in December 2018 and China became the first country in the world to use it for the treatment of renal anemia (21). It promotes the production of endogenous erythropoietin, is effective in the treatment of renal anemia, is well-tolerated and safe. This article reviews the related research of Roxadustat, focusing on roxadustat for the treatment of inflammatory anemia of chronic kidney disease.
Under normal circumstances, EPO in plasma promotes the differentiation of bone marrow hematopoietic stem cells into erythroid progenitor cells, which then gradually form mature new red blood cells, thereby continuously renewing the body's blood. In this process, plasma transferrin and serum iron are transported to the surface of the red blood cell membrane to bind the transferrin receptor, and enter the cell to release iron through the effect of puffing. Intracellular iron combines with iron porphyrins in mitochondria to form hemoglobin, and complete the maturation of red blood cells.
During the process of maintaining serum iron homeostasis, reticuloendothelial cells swallow senescent red blood cells to reabsorb iron in hemoglobin, and transport intracellular iron to the serum to supplement serum iron with ferroportin (FPN) (22). In addition to the human duodenum, divalent metal transporter 1 (DMT1) and duodenal cytochrome b (DcytB) are important components of the intestinal absorption of iron, especially when body are deficient in iron, which coordinate with each other to absorb the iron in food, and then transports it into the duodenal cells. The iron in the intestinal cells outputs the iron to the blood circulation through FPN to maintain the steady state of the iron in the body. Therefore, increased iron absorption in the intestine is closely related to DMT1, DcytB, and FPN protein levels (23).
Like many chronic diseases, chronic kidney disease is largely considered an inflammatory disease (24). Inflammation can affect hematopoietic function. Inflammation promotes hepatic secretion of hepcidin through the interleukin 6 (IL-6) -STAT3 pathway (25–29) and the bone morphogenetic protein (BMP) -SMAD pathway (30). Hepcidin increases binding to iron transporters on monocyte phagocytic and duodenal cell membranes, thereby promoting the internalization and degradation of FPN (31), resulting in obstructed iron output in monocyte macrophages and duodenal cells (32). At the same time, it was found that hepcidin can also inhibit the secretion of DMT1 and DcytB to affect the absorption of intestinal iron (33–36). These factors lead to insufficient plasma iron, which cannot meet the iron required for erythropoiesis to lead to anemia, so hepcidin is the key to causing anemia and ESA resistance (37). A cross-sectional study of serum Hepcidin-25 levels and anemia in non-diabetic chronic kidney disease patients in Japan showed that serum hepcidin was negatively correlated with hemoglobin and gradually leaded to the development of chronic kidney disease and gradually increases (38).
Second, inflammatory cytokines play an important role in many patients with renal anemia. Inflammatory cytokines IL-1, TNF-a, and IFN-α inhibited the proliferation and differentiation of erythroid precursors in the bone marrow, especially erythroid burst forming units and erythroid colony forming units. In early in vitro experiments, IL-1 and TNF-α were found to inhibit erythropoietin expression in isolated rat kidney and human liver cancer cells (39–41), but it has not been confirmed in humans whether inflammatory factors inhibit EPO production. Increased oxidative stress under inflammation leads to lipid peroxidation of erythrocyte membrane (42, 43), at the same time, inflammatory cytokines promote phagocytosis of macrophages (44), which together lead to shortened red blood cell lifespan. Khalil et al. used anti-oxidant vitamin C in hemodialysis patients with inflammatory anemia, and the results showed that they can reduce the EPO resistance of hemodialysis patients and reduce the dose required for EPO (45). Therefore, inflammation can lead to anemia in patients with chronic kidney disease through many ways. Figure 1 summarized the mechanisms by which inflammation causes anemia and EPO resistance.
Figure 1. Inflammation leads to anemia and EPO resistance of mechanisms in CKD. IL-6, interleukin-6; IL-1, interleukin-1; IFN-α, -β, -γ, interferon-α, -β, -γ; TNF-α, tumor necrosis factor-α; EPO, erythropoietin; EBFU, erythroid burst-forming units; ECFO, erythroid colony-forming units; ↑, strengthen or increase; ↓, decrease or inhibit; FNP, ferroportin; DMT1, divalent metal transporter 1; CKD, chronic kidney disease.
Roxadustat (FG-4592) is a hypoxia-inducible factor prolyl hydroxylase inhibitor (PHI). The main mechanism of action is to inhibit the activity of prolyl hydroxylase domain protein (PHD) under the normoxia. Hypoxia-inducible factor α (HIF-α) has three subtypes 1, 2, and 3, and HIF-β has only one subtypes. In the case where PHD is inhibited, the HIF-α will be not hydroxylated by PHD, nor will it be ubiquitinated by the von Hippel-Lindau tumor suppressor (VHL), It will not be degraded by the proteasome. Therefore, more stable HIF-α enters the nucleus and forms heterodimers with HIF-β (46, 47), which activates target gene expression, such as erythropoietin, glycolysis, and angiogenic factors (48, 49). Therefore, besides being used to treat anemia, roxadustat may also be used to treat other diseases.
Until now, more than 9,000 patients with renal anemia have participated in phase 2 and phase 3 clinical studies, including non-dialysis patients, hemodialysis patients, and peritoneal dialysis patients. Clinical trials have shown that roxadustat corrected anemia by stimulating the expression of endogenous erythropoietin, which promoted erythropoiesisb and was then non-inferior and superior to placebo and epoetin. No serious drug-related adverse events had occurred in clinical trials to date. Tables 1, 2 were the summary of roxadustat in clinical trials, respectively. Table 3 showed the effects of inflammatory on roxadustat in clinical trials.
The purpose of this phase III clinical study was to evaluate the efficacy and safety of roxadustat in the treatment of anemia in dialysis patient. Three hundred and five patients with end-stage renal disease undergoing dialysis were randomly assigned to roxadustat group and epoetin alfa group. The results showed that roxadustat increased hemoglobin statistically significant non-inferiority relative to epoetin alfa [difference: 0.2 ± 1.2 g/dl; 95% confidence interval (CI): −0.02 to 0.5], The level of hepcidin in the roxadustat group and the epoetin group decreased by 30.2 ng/ml (95% CI: −64.8 to 13.6), and 2.3 ng/ml (95% CI: −51.6 to 6.2), respectively. In addition, patients with elevated CRP levels had similar CRP mean hemoglobin levels and comparable dose in roxadustat group, however, although the dose of epoetin alfa was increased in epoetin group. In the elevated CRP, the hemoglobin level of roxadustat group was larger than that of epoetin alfa group (0.9 ± 1.0 g/dl vs. 0.3 ± 1.1 g/dl) (53).
The purpose of this phase III clinical study was to evaluate the efficacy and safety of roxadustat. One hundred and fifty four patients who did not receive dialysis were randomized to 2:1 to the roxadustat and placebo groups. The results showed a hemoglobin increase of 1.9 ± 1.2 g/dl in roxadustat group and 0.4 ± 0.8 g/dl in the placebo group (P < 0.001). The decrease in hepcidin in the roxadustat group was 56.14 ± 63.40 ng/ml and 15.10 ± 48.06 ng/ml, respectively (54).
Inflammation interferes with the body's iron homeostasis through hepcidin. Therefore, reducing hepcidin is the key to treating inflammatory anemia (58). Hepcidin antibodies now a new strategy for treating inflammatory anemia (59–61). However, it is well-known that under low oxygen conditions, serum hepcidin in the human body can effectively reduce (62, 63). At the beginning, people thought that it was hypoxia to stabilize HIF which directly inhibited hepatocyte synthesis of hepcidin at the gene level. However, in a hypoxic treatment of HepG2 cells, knockdown of HIF-1α or HIF-2α did not reverse the decline of Hepcidin mRNA, which was not a direct target of HIF-1α or HIF-2α (64, 65).
In experiments conducted by Peyssonnaux et al. mice showed elevated levels of serum inflammatory cytokines IL-6 and IL-1 due to hepatic inflammation and steatosis, whereas decreased levels of hepcidin and increased expression of FNP in mice with VHL gene deletion. Experiments have shown that inhibition of VHL or PHD activity can be used to treat chronic inflammatory anemia (66). Therefore, increased hepcidin caused by inflammation is reduced by inhibiting the activity of VHL and PHD.
Recently, studies have found that hypoxia-lowering hepcidin appeared to be related to platelet derived growth factor (PDGF-B) in bone marrow (27, 67). When PDGF-B or its receptor was inactivated, inhibition of hepcidin was relieved (67, 68), and PDGF-B is a target gene of HIF-1α (69). Or HIF may be an expression of hepcidin controlled by an indirect way (70). Chaston et al. found that hypoxia inhibited the expression of hepcidin by BMP-SMAD pathway (71), but there was no clear explanation for how hypoxia inhibits hepcidin expression.
Roxadustat is a PHI that works under conditions that simulate hypoxia. The study found hepcidin levels was significantly greater in receiving roxadustat than in receiving placebo for CKD of patients (37.5 vs. 4.8 ng/mL, respectively, P < 0.0001). Hepcidin level was a dose-dependent decrease fir roxadustat (50). Many clinical studies have shown that roxadustat could significantly reduce hepcidin levels in patients with CKD (50, 51, 53–57). Even though plasma CRP was above the upper limit of normal, roxadustat still reduced plasma hepcidin.
Roxadustat can reduce plasma hepcidin to restore the intestinal absorption of dietary iron. In addition, it has been found that hypoxia could enhance the absorption of dietary iron by the intestines (72). It has been shown that stable HIF-2 could promote the expression of DcytB, DMT1, and FPN at the gene level (73–75), while HIF-1 can promote transferrin expression. In the second phase of the Roxadustat trial conducted by Besarab et al. dialysis patients can significantly increase hemoglobin levels regardless of iron supplementation or iron supplementation. Elevated hemoglobin levels were almost the same for oral iron and intravenous iron, and was not influenced by CRP (57). Therefore, roxadustat can stabilize HIF by inhibiting PHD to promote the expression of DcytB, DMT1, and FPN in mucosal cells, accelerate the absorption of intestinal iron, and promote the release of iron from macrophages of FPN, which is beneficial to supplement plasma iron to meet the demand for iron in erythropoiesis. Figure 2 showed that roxadustat can promote intestinal iron absorption.
Figure 2. Metabolisms of HIF in the cell without roxadustat, Iron is normally absorbed in the intestines and roxadustat promotes iron absorption. HIF-α, hypoxia-inducible factor α; HIF-β, hypoxia-inducible factor β; PHD, prolyl hypoxia domain protein; FG-4592, roxadustat; FPN, ferroportin; DcytB, duodenal cytochrome B; DMT1, divalent metal transporter 1; VHL, von Hipple-Lindau tumor suppressor; red, activation; white, inactivation; Normoxia, normal oxygen level in the body.
Inflammation and inflammatory cytokines affected erythrocyte, and were also responsible for EPO resistance. It has long been known that early ischemic preconditioning of organs that were ischemia-reperfusion would reduce the damage to organs, which seemed to be related to the hypoxic protection mechanism of the body. In a trial conducted by Cheng et al. early intermittent chronic hypoxic preconditioning of skeletal muscle in rats with ischemia-reperfusion resulted in decreased signs of inflammation such as tumor necrosis factor and macrophage (76), and the hypoxic protective mechanism appeared to have Inhibition of inflammation reduces the effects of ischemia-reperfusion injury (77).
HIF can promote adenosine gene expression and assist adenosine to exert anti-inflammatory effects (78–80). Many studies have confirmed that HIF had anti-inflammatory and promoted the function of inflammation regression (78, 81, 82). Kobayashi et al. demonstrated that myeloid-specific HIF reduces renal inflammation associated with chronic kidney injury, and that overall loss of HIF or myeloid-specific inactivation promotes inflammation, and Long-term hypoxia in an unimpaired kidney inhibits the expression of multiple inflammatory molecules (83). In addition, it has been found that T cells or dendritic cells lacking HIF-1 cause aggravation of intestinal inflammation (84), especially in inflammatory bowel diseases, and the treatment of inflammatory bowel disease with PHIs has become a new approach (85). In addition, in the kidney injury induced by cisplatin alone, the inflammatory cytokines such as TNF-α, IL-1β, IL-6 were significantly increased, but after treatment with roxadustat and cisplatin, these inflammatory factors were much reduced. The trial confirmed that roxadustat had a potential anti-inflammatory effect and was associated with HIF (86). In the roxadustat phase 3 trial in China, the dose of roxadustat decreased slightly in the CRP above the upper limit group as the treatment time decreased. However, epoetin alpha increased the dose over time, and it was difficult for Hb to reach the target value. From a molecular perspective, roxadustat can stabilize HIF against inflammation and inhibit the production of inflammatory cytokines, but more clinical studies are needed to confirm this possibility. Figure 3 showed the mechanisms of roxadustat in the treatment of inflammatory anemia.
Figure 3. Roxadustat treats inflammatory anemia in CKD. Inflammatory cytokines promote the production of hepcidin to inhibit iron absorption and iron transport and iron release. Inflammatory factors can also interfere with bone marrow hematopoiesis. In addition, inflammatory factors may inhibit the production of EPO. In summary, inflammation causes anemia and EPO resistance through these causes. Roxadustat can inhibit the increase of hepcidin caused by inflammation and promote the production of EPO, and may also inhibit the secretion of inflammatory cytokines. Roxadustat treats inflammatory anemia by these factors. Black: inflammation path; red: roxadustat path.
A case of association between roxadustat and pulmonary hypertension was reported in Poland. The investigators believed that roxadustat stabilized HIF-2α and affected the production of pulmonary hypertension induced by vascular remodeling. Although the association between roxadustat and pulmonary artery was not clear (87). PHI inhibits PHD from a molecular mechanism, then stabilizes HIF-2α to up-regulate Notch3 and transform growth factor β, and promote the conversion of pericytes into myofibroblasts or vascular smooth muscle cells. This is related to the formation of pulmonary hypertension. Extensive research evidence suggested that pulmonary hypertension was associated with poor long-term viability and increased mortality in patients with advanced kidney disease (88, 89). Therefore, the association between roxadustat and pulmonary hypertension needs further research.
Fortunately, the Phase 2 and Phase 3 clinical trials were currently announced, and roxadustat was well-tolerated, there were no deaths in roxadustat-treated patients. Of course, drug-related adverse events mainly including infection (upper respiratory tract infection, urinary tract infection), hyperkalemia, metabolic acidosis, abnormal liver function, Gastrointestinal disorders (such as diarrhea and vomiting, etc.), Gastrointestinal disorders (Such as diarrhea and vomiting, etc.), the serious adverse events in roxadustat-related subjects included vascular access complications, however, in general, fewer drug-related adverse events occurred (50–57).
All the above studies show that roxadustat can up-regulate erythropoietin, reduce hepcidin, and promote intestinal absorption of iron by stabilizing HIF. Therefore, it can correct iron metabolism disorders due to inflammation in the body. It may be a new choice for the treatment of chronic kidney disease inflammatory anemia. Of course, roxadustat anti-inflammatory and inhibitory inflammatory cytokine also require more clinical studies to confirm.
ZY: conceptualization, software, writing–original draft, investigation, data curation and visualization, and formal analysis. GX: supervision, project administration, and writing–review and editing. All authors contributed to the article and approved the submitted version.
This work was supported by the National Natural Science Foundation of China (No. 81970583), Joint Project of the Natural Science Foundation of Jiangxi Province in 2020, and the Nature Science Foundation of Jiangxi Province (No. 20181BAB205016).
The authors declare that the research was conducted in the absence of any commercial or financial relationships that could be construed as a potential conflict of interest.
EPO, erythropoietin; ESAs, erythropoiesis-stimulating agents; CRP, C-reactive protein; hs-CRP, high sensitivity CRP; CKD, chronic kidney disease; DMT1, divalent metal transporter 1; FPN, ferroportin; DcytB, duodenal cytochrome B; HIF, hypoxia-inducible factor; VHL, von Hipple-Lindau tumor suppressor; PHD, prolyl hydroxylase domain protein; PDGF-B, platelet-derived growth factor B; IL-6, interleukin 6; BMP, bone morphogenetic protein; Hb, hemoglobin; HD, hemodialysis; TNF, tumor necrosis factor; CI, confidence interval.
1. Babitt JL, Lin HY. Mechanisms of anemia in CKD. J Am Soc Nephrol. (2012) 23:1631–4. doi: 10.1681/ASN.2011111078
2. Nangaku M, Eckardt KU. Pathogenesis of renal anemia. Semin Nephrol. (2006) 26:261–8. doi: 10.1016/j.semnephrol.2006.06.001
3. Locatelli F, Pisoni RL, Akizawa T, Cruz JM, DeOreo PB, Lameire NH, et al. Anemia management for hemodialysis patients: Kidney Disease Outcomes Quality Initiative (K/DOQI) guidelines and Dialysis Outcomes and Practice Patterns Study (DOPPS) findings. Am J Kidney Dis. (2004) 44(Suppl. 2):27–33. doi: 10.1053/j.ajkd.2004.08.008
4. Volkova N, Arab L. Evidence-based systematic literature review of hemoglobin/hematocrit and all-cause mortality in dialysis patients. Am J Kidney Dis. (2006) 47:24–36. doi: 10.1053/j.ajkd.2005.09.007
5. Drueke T. Hyporesponsiveness to recombinant human erythropoietin. Nephrol Dial Transplant. (2001) 16(Suppl. 7):25–8. doi: 10.1093/ndt/16.suppl_7.25
6. Bradbury BD, Wang O, Critchlow CW, Rothman KJ, Heagerty P, Keen M, et al. Exploring relative mortality and epoetin alfa dose among hemodialysis patients. Am J Kidney Dis. (2008) 51:62–70. doi: 10.1053/j.ajkd.2007.09.015
7. Kilpatrick RD, Critchlow CW, Fishbane S, Besarab A, Stehman-Breen C, Krishnan M, et al. Greater epoetin alfa responsiveness is associated with improved survival in hemodialysis patients. Clin J Am Soc Nephrol. (2008) 3:1077–83. doi: 10.2215/CJN.04601007
8. Minutolo R, Conte G, Cianciaruso B, Bellizzi V, Camocardi A, De Paola L, et al. Hyporesponsiveness to erythropoiesis-stimulating agents and renal survival in non-dialysis CKD patients. Nephrol Dial Transplant. (2012) 27:2880–6. doi: 10.1093/ndt/gfs007
9. Costa E, Lima M, Alves JM, Rocha S, Rocha-Pereira P, Castro E, et al. Inflammation, T-cell phenotype, and inflammatory cytokines in chronic kidney disease patients under hemodialysis and its relationship to resistance to recombinant human erythropoietin therapy. J Clin Immunol. (2008) 28:268–75. doi: 10.1007/s10875-007-9168-x
10. Szczech LA, Barnhart HX, Inrig JK, Reddan DN, Sapp S, Califf RM, et al. Secondary analysis of the CHOIR trial epoetin-α dose and achieved hemoglobin outcomes. Kidney Int. (2008) 74:791–8. doi: 10.1038/ki.2008.295
11. Barany P. Inflammation, serum C-reactive protein, and erythropoietin resistance. Nephrol Dial Transplant. (2001) 16:224–7. doi: 10.1093/ndt/16.2.224
12. Bradbury BD, Critchlow CW, Weir MR, Stewart R, Krishnan M, Hakim RH. Impact of elevated C-reactive protein levels on erythropoiesis- stimulating agent (ESA) dose and responsiveness in hemodialysis patients. Nephrol Dial Transplant. (2009) 24:919–25. doi: 10.1093/ndt/gfn543
13. Cooper AC, Mikhail A, Lethbridge MW, Kemeny DM, Macdougall IC. Increased expression of erythropoiesis inhibiting cytokines (IFN-gamma, TNF-alpha, IL-10, and IL-13) by T cells in patients exhibiting a poor response to erythropoietin therapy. J Am Soc Nephrol. (2003) 14:1776–84. doi: 10.1097/01.ASN.0000071514.36428.61
14. Kalantar-Zadeh K, McAllister CJ, Lehn RS, Lee GH, Nissenson AR, Kopple JD. Effect of malnutrition-inflammation complex syndrome on EPO hyporesponsiveness in maintenance hemodialysis patients. Am J Kidney Dis. (2003) 42:761–73. doi: 10.1016/S0272-6386(03)00915-6
15. Macdougall IC, Cooper AC. Erythropoietin resistance: the role of inflammation and pro-inflammatory cytokines. Nephrol Dial Transplant. (2002) 17(Suppl. 11):39–43. doi: 10.1093/ndt/17.suppl_11.39
16. Rattanasompattikul M, Molnar MZ, Zaritsky JJ, Hatamizadeh P, Jing J, Norris KC, et al. Association of malnutrition-inflammation complex and responsiveness to erythropoiesis-stimulating agents in long-term hemodialysis patients. Nephrol Dial Transplant. (2013) 28:1936–45. doi: 10.1093/ndt/gfs368
17. Chonchol M, Lippi G, Montagnana M, Muggeo M, Targher G. Association of inflammation with anaemia in patients with chronic kidney disease not requiring chronic dialysis. Nephrol Dial Transplant. (2008) 23:2879–83. doi: 10.1093/ndt/gfn109
18. Del Vecchio L, Pozzoni P, Andrulli S, Locatelli F. Inflammation and resistance to treatment with recombinant human erythropoietin. J Renal Nutr. (2005) 15:137–41. doi: 10.1053/j.jrn.2004.09.024
19. Costa E, Rocha S, Rocha-Pereira P, Nascimento H, Castro E, Miranda V, et al. Neutrophil activation and resistance to recombinant human erythropoietin therapy in hemodialysis patients. Am J Nephrol. (2008) 28:935–40. doi: 10.1159/000142147
20. Stenvinkel P. The role of inflammation in the anaemia of end-stage renal disease. Nephrol Dial Transplant. (2001) 16(Suppl. 7):36–40. doi: 10.1093/ndt/16.suppl_7.36
21. Dhillon S. Roxadustat: first global approval. Drugs. (2019) 79:563–72. doi: 10.1007/s40265-019-01077-1
22. Bergamaschi G, Di Sabatino A, Pasini A, Ubezio C, Costanzo F, Grataroli D, et al. Intestinal expression of genes implicated in iron absorption and their regulation by hepcidin. Clin Nutr. (2017) 36:1427–33. doi: 10.1016/j.clnu.2016.09.021
23. Latunde-Dada GO, Xiang L, Simpson RJ, McKie AT. Duodenal cytochrome b (Cybrd 1) and HIF-2alpha expression during acute hypoxic exposure in mice. Eur J Nutr. (2011) 50:699–704. doi: 10.1007/s00394-011-0175-6
24. Weiss G, Goodnough LT. Anemia of chronic disease. N Engl J Med. (2005) 352:1011–23. doi: 10.1056/NEJMra041809
25. Gordeuk VR, Miasnikova GY, Sergueeva AI, Niu X, Nouraie M, Okhotin DJ, et al. Chuvash polycythemia VHLR200W mutation is associated with down-regulation of hepcidin expression. Blood. (2011) 118:5278–82. doi: 10.1182/blood-2011-03-345512
26. Reichert CO, da Cunha J, Levy D, Maselli LMF, Bydlowski SP, Spada C. Hepcidin: homeostasis and diseases related to iron metabolism. Acta Haematol. (2017) 137:220–36. doi: 10.1159/000471838
27. Rishi G, Subramaniam VN. Signaling pathways regulating hepcidin. Vitam Horm. (2019) 110:47–70. doi: 10.1016/bs.vh.2019.01.003
28. Verga Falzacappa MV, Vujic Spasic M, Kessler R, Stolte J, Hentze MW, Muckenthaler MU. STAT3 mediates hepatic hepcidin expression and its inflammatory stimulation. Blood. (2007) 109:353–8. doi: 10.1182/blood-2006-07-033969
29. Wrighting DM, Andrews NC. Interleukin-6 induces hepcidin expression through STAT3. Blood. (2006) 108:3204–9. doi: 10.1182/blood-2006-06-027631
30. Steinbicker AU, Sachidanandan C, Vonner AJ, Yusuf RZ, Deng DY, Lai CS, et al. Inhibition of bone morphogenetic protein signaling attenuates anemia associated with inflammation. Blood. (2011) 117:4915–23. doi: 10.1182/blood-2010-10-313064
31. Ganz T. Hepcidin and iron regulation, 10 years later. Blood. (2011) 117:4425–33. doi: 10.1182/blood-2011-01-258467
32. Drakesmith H, Nemeth E, Ganz T. Ironing out Ferroportin. Cell Metab. (2015) 22:777–87. doi: 10.1016/j.cmet.2015.09.006
33. Fraenkel PG. Anemia of inflammation: a review. Med Clin North Am. (2017) 101:285–96. doi: 10.1016/j.mcna.2016.09.005
34. Latunde-Dada GO, Simpson RJ, McKie AT. Duodenal cytochrome B expression stimulates iron uptake by human intestinal epithelial cells. J Nutr. (2008) 138:991–5. doi: 10.1093/jn/138.6.991
35. Mastrogiannaki M, Matak P, Peyssonnaux C. The gut in iron homeostasis: role of HIF-2 under normal and pathological conditions. Blood. (2013) 122:885–92. doi: 10.1182/blood-2012-11-427765
36. Sheikh N, Dudas J, Ramadori G. Changes of gene expression of iron regulatory proteins during turpentine oil-induced acute-phase response in the rat. Lab Invest. (2007) 87:713–25. doi: 10.1038/labinvest.3700553
37. Zhang AS, Enns CA. Molecular mechanisms of normal iron homeostasis. Hematology Am Soc Hematol Educ Prog. (2009) 1:207–14. doi: 10.1182/asheducation-2009.1.207
38. Uehata T, Tomosugi N, Shoji T, Sakaguchi Y, Suzuki A, Kaneko T, et al. Serum hepcidin-25 levels and anemia in non-dialysis chronic kidney disease patients: a cross-sectional study. Nephrol Dial Transplant. (2012) 27:1076–83. doi: 10.1093/ndt/gfr431
39. Faquin WC, Schneider TJ, Goldberg MA. Effect of inflammatory cytokines on hypoxia-induced erythropoietin production. Blood. (1992) 79:1987–94. doi: 10.1182/blood.V79.8.1987.bloodjournal7981987
40. Jelkmann W. Proinflammatory cytokines lowering erythropoietin production. J Interferon Cytok Res. (1998) 18:555–9. doi: 10.1089/jir.1998.18.555
41. Leng HM, Kidson SH, Keraan MM, Randall GW, Folb PI. Cytokine-mediated inhibition of erythropoietin synthesis by dexamethasone. J Pharm Pharmacol. (1996) 48:971–4. doi: 10.1111/j.2042-7158.1996.tb06015.x
42. Bamgbola OF. Pattern of resistance to erythropoietin-stimulating agents in chronic kidney disease. Kidney Int. (2011) 80:464–74. doi: 10.1038/ki.2011.179
43. Stenvinkel P, Barany P. Anaemia, rHuEPO resistance, and cardiovascular disease in end-stage renal failure; links to inflammation and oxidative stress. Nephrol Dial Transplant. (2002) 17(Suppl. 5):32–7. doi: 10.1093/ndt/17.suppl_5.32
45. Khalil SK, Amer HA, El Behairy AM, Warda M. Oxidative stress during erythropoietin hyporesponsiveness anemia at end stage renal disease: molecular and biochemical studies. J Adv Res. (2016) 7:348–58. doi: 10.1016/j.jare.2016.02.004
46. Haase VH. Regulation of erythropoiesis by hypoxia-inducible factors. Blood Rev. (2013) 27:41–53. doi: 10.1016/j.blre.2012.12.003
47. Haase VH. HIF-prolyl hydroxylases as therapeutic targets in erythropoiesis and iron metabolism. Hemodial Int. (2017) 21(Suppl. 1):S110–24. doi: 10.1111/hdi.12567
48. Bogdanovski DA, DiFazio LT, Bogdanovski AK, Csoka B, Jordan GB, Paul ER, et al. Hypoxia-inducible-factor-1 in trauma and critical care. J Crit Care. (2017) 42:207–12. doi: 10.1016/j.jcrc.2017.07.029
49. Semenza GL. Hydroxylation of HIF-1: oxygen sensing at the molecular level. Physiology. (2004) 19:176–82. doi: 10.1152/physiol.00001.2004
50. Chen N, Qian J, Chen J, Yu X, Mei C, Hao C, et al. Phase 2 studies of oral hypoxia-inducible factor prolyl hydroxylase inhibitor FG-4592 for treatment of anemia in China. Nephrol Dial Transplant. (2017) 32:1373–86. doi: 10.1093/ndt/gfx011
51. Akizawa T, Iwasaki M, Otsuka T, Reusch M, Misumi T. Roxadustat treatment of chronic kidney disease-associated anemia in Japanese patients not on dialysis: a phase 2, randomized, double-blind, placebo-controlled trial. Adv Ther. (2019) 36:1438–54. doi: 10.1007/s12325-019-00943-4
52. Besarab A, Provenzano R, Hertel J, Zabaneh R, Klaus SJ, Lee T, et al. Randomized placebo-controlled dose-ranging and pharmacodynamics study of roxadustat (FG-4592) to treat anemia in nondialysis-dependent chronic kidney disease (NDD-CKD) patients. Nephrol Dial Transplant. (2015) 30:1665–73. doi: 10.1093/ndt/gfv302
53. Chen N, Hao C, Liu BC, Lin H, Wang C, Xing C, et al. Roxadustat treatment for anemia in patients undergoing long-term dialysis. N Engl J Med. (2019) 381:1011–22. doi: 10.1056/NEJMoa1901713
54. Chen N, Hao C, Peng X, Lin H, Yin A, Hao L, et al. Roxadustat for anemia in patients with kidney disease not receiving dialysis. N Engl J Med. (2019) 381:1001–10. doi: 10.1056/NEJMoa1813599
55. Provenzano R, Besarab A, Wright S, Dua S, Zeig S, Nguyen P, et al. Roxadustat (FG-4592) versus epoetin alfa for anemia in patients receiving maintenance hemodialysis: a phase 2, randomized, 6- to 19-week, open-label, active-comparator, dose-ranging, safety and exploratory efficacy study. Am J Kidney Dis. (2016) 67:912–24. doi: 10.1053/j.ajkd.2015.12.020
56. Provenzano R, Besarab A, Sun CH, Diamond SA, Durham JH, Cangiano JL, et al. Oral hypoxia-inducible factor prolyl hydroxylase inhibitor roxadustat (FG-4592) for the treatment of anemia in patients with CKD. Clin J Am Soc Nephrol. (2016) 11:982–91. doi: 10.2215/CJN.06890615
57. Besarab A, Chernyavskaya E, Motylev I, Shutov E, Kumbar LM, Gurevich K, et al. Roxadustat (FG-4592): correction of anemia in incident dialysis patients. J Am Soc Nephrol. (2016) 27:1225–33. doi: 10.1681/ASN.2015030241
58. Tsuchiya K, Nitta K. Hepcidin is a potential regulator of iron status in chronic kidney disease. Ther Apher Dial. (2013) 17:1–8. doi: 10.1111/1744-9987.12001
59. Coyne DW. Hepcidin: clinical utility as a diagnostic tool and therapeutic target. Kidney Int. (2011) 80:240–4. doi: 10.1038/ki.2011.141
60. Ganz T. Hepcidin, a key regulator of iron metabolism and mediator of anemia of inflammation. Blood. (2003) 102:783–8. doi: 10.1182/blood-2003-03-0672
61. Sasu BJ, Cooke KS, Arvedson TL, Plewa C, Ellison AR, Sheng J, et al. Antihepcidin antibody treatment modulates iron metabolism and is effective in a mouse model of inflammation-induced anemia. Blood. (2010) 115:3616–24. doi: 10.1182/blood-2009-09-245977
62. Braliou GG, Verga Falzacappa MV, Chachami G, Casanovas G, Muckenthaler MU, Simos G. 2-Oxoglutarate-dependent oxygenases control hepcidin gene expression. J Hepatol. (2008) 48:801–10. doi: 10.1016/j.jhep.2007.12.021
63. Nicolas G, Chauvet C, Viatte L, Danan JL, Bigard X, Devaux I, et al. The gene encoding the iron regulatory peptide hepcidin is regulated by anemia, hypoxia, and inflammation. J Clin Investig. (2002) 110:1037–44. doi: 10.1172/JCI0215686
64. Mastrogiannaki M, Matak P, Mathieu JR, Delga S, Mayeux P, Vaulont S, et al. Hepatic hypoxia-inducible factor-2 down-regulates hepcidin expression in mice through an erythropoietin-mediated increase in erythropoiesis. Haematologica. (2012) 97:827–34. doi: 10.3324/haematol.2011.056119
65. Volke M, Gale DP, Maegdefrau U, Schley G, Klanke B, Bosserhoff AK, et al. Evidence for a lack of a direct transcriptional suppression of the iron regulatory peptide hepcidin by hypoxia-inducible factors. PLoS ONE. (2009) 4:e7875. doi: 10.1371/journal.pone.0007875
66. Peyssonnaux C, Zinkernagel AS, Schuepbach RA, Rankin E, Vaulont S, Haase VH, et al. Regulation of iron homeostasis by the hypoxia-inducible transcription factors (HIFs). J Clin Invest. (2007) 117:1926–32. doi: 10.1172/JCI31370
67. Sonnweber T, Nachbaur D, Schroll A, Nairz M, Seifert M, Demetz E, et al. Hypoxia induced downregulation of hepcidin is mediated by platelet derived growth factor BB. Gut. (2014) 63:1951–9. doi: 10.1136/gutjnl-2013-305317
68. Ravasi G, Pelucchi S, Buoli Comani G, Greni F, Mariani R, Pelloni I, et al. Hepcidin regulation in a mouse model of acute hypoxia. Eur J Haematol. (2018) 100:636–43. doi: 10.1111/ejh.13062
69. Schito L, Rey S, Tafani M, Zhang H, Wong CC, Russo A, et al. Hypoxia-inducible factor 1-dependent expression of platelet-derived growth factor B promotes lymphatic metastasis of hypoxic breast cancer cells. Proc Natl Acad Sci USA. (2012) 109:E2707–16. doi: 10.1073/pnas.1214019109
70. Peyssonnaux C, Nizet V, Johnson RS. Role of the hypoxia inducible factors HIF in iron metabolism. Cell Cycle. (2008) 7:28–32. doi: 10.4161/cc.7.1.5145
71. Chaston TB, Matak P, Pourvali K, Srai SK, McKie AT, Sharp PA. Hypoxia inhibits hepcidin expression in HuH7 hepatoma cells via decreased SMAD4 signaling. Am J Physiol Cell Physiol. (2011) 300:C888–95. doi: 10.1152/ajpcell.00121.2010
72. D'Anna MC, Roque ME. Physiological focus on the erythropoietin-hepcidin-ferroportin axis. Can J Physiol Pharmacol. (2013) 91:338–45. doi: 10.1139/cjpp-2012-0214
73. Muckenthaler MU, Rivella S, Hentze MW, Galy B. A red carpet for iron metabolism. Cell. (2017) 168:344–61. doi: 10.1016/j.cell.2016.12.034
74. Shah YM, Matsubara T, Ito S, Yim SH, Gonzalez FJ. Intestinal hypoxia-inducible transcription factors are essential for iron absorption following iron deficiency. Cell Metab. (2009) 9:152–64. doi: 10.1016/j.cmet.2008.12.012
75. Taylor M, Qu A, Anderson ER, Matsubara T, Martin A, Gonzalez FJ, et al. Hypoxia-inducible factor-2alpha mediates the adaptive increase of intestinal ferroportin during iron deficiency in mice. Gastroenterology. (2011) 140:2044–55. doi: 10.1053/j.gastro.2011.03.007
76. Cheng WJ, Liu X, Zhang L, Guo XQ, Wang FW, Zhang Y, et al. Chronic intermittent hypobaric hypoxia attenuates skeletal muscle ischemia-reperfusion injury in mice. Life Sci. (2019) 231:116533. doi: 10.1016/j.lfs.2019.06.008
77. Colgan SP, Campbell EL, Kominsky DJ. Hypoxia and mucosal inflammation. Annu Rev Pathol. (2016) 11:77–100. doi: 10.1146/annurev-pathol-012615-044231
78. Bartels K, Grenz A, Eltzschig HK. Hypoxia and inflammation are two sides of the same coin. Proc Natl Acad Sci USA. (2013) 110:18351–2. doi: 10.1073/pnas.1318345110
79. Bowser JL, Lee JW, Yuan X, Eltzschig HK. The hypoxia-adenosine link during inflammation. J Appl Physiol. (2017). 123:1303–20. doi: 10.1152/japplphysiol.00101.2017
80. Eltzschig HK, Carmeliet P. Hypoxia and inflammation. N Engl J Med. (2011) 364:656–65. doi: 10.1056/NEJMra0910283
81. Kiers HD, Scheffer GJ, van der Hoeven JG, Eltzschig HK, Pickkers P, Kox M. Immunologic consequences of hypoxia during critical illness. Anesthesiology. (2016) 125:237–49. doi: 10.1097/ALN.0000000000001163
82. Scholz CC, Taylor CT. Targeting the HIF pathway in inflammation and immunity. Curr Opin Pharmacol. (2013) 13:646–53. doi: 10.1016/j.coph.2013.04.009
83. Kobayashi H, Gilbert V, Liu Q, Kapitsinou PP, Unger TL, Rha J, et al. Myeloid cell-derived hypoxia-inducible factor attenuates inflammation in unilateral ureteral obstruction-induced kidney injury. J Immunol. (2012) 188:5106–15. doi: 10.4049/jimmunol.1103377
84. Higashiyama M, Hokari R, Hozumi H, Kurihara C, Ueda T, Watanabe C, et al. HIF-1 in T cells ameliorated dextran sodium sulfate-induced murine colitis. J Leukoc Biol. (2012) 91:901–9. doi: 10.1189/jlb.1011518
85. Cummins EP, Seeballuck F, Keely SJ, Mangan NE, Callanan JJ, Fallon PG, et al. The hydroxylase inhibitor dimethyloxalylglycine is protective in a murine model of colitis. Gastroenterology. (2008) 134:156–65. doi: 10.1053/j.gastro.2007.10.012
86. Yang Y, Yu X, Zhang Y, Ding G, Zhu C, Huang S, et al. Hypoxia-inducible factor prolyl hydroxylase inhibitor roxadustat (FG-4592) protects against cisplatin-induced acute kidney injury. Clin Sci. (2018) 132:825–38. doi: 10.1042/CS20171625
87. Cygulska K, Wejner-Mik P, Plewka M, Figiel L, Chrzanowski L, Kasprzak JD. Roxadustat: another drug that causes pulmonary hypertension? Report of first human case. Pol Arch Intern Med. (2019) 129:344–5. doi: 10.20452/pamw.4445
88. Agarwal R. Prevalence, determinants and prognosis of pulmonary hypertension among hemodialysis patients. Nephrol Dial Transplant. (2012) 27:3908–14. doi: 10.1093/ndt/gfr661
Keywords: chronic kidney disease, roxadustat, inflammation, hypoxia inducible factor, erythropoietin
Citation: Yan Z and Xu G (2020) A Novel Choice to Correct Inflammation-Induced Anemia in CKD: Oral Hypoxia-Inducible Factor Prolyl Hydroxylase Inhibitor Roxadustat. Front. Med. 7:393. doi: 10.3389/fmed.2020.00393
Received: 18 December 2019; Accepted: 23 June 2020;
Published: 06 August 2020.
Edited by:
Michael L. Moritz, University of Pittsburgh, United StatesReviewed by:
Francesco Locatelli, Alessandro Manzoni Hospital, ItalyCopyright © 2020 Yan and Xu. This is an open-access article distributed under the terms of the Creative Commons Attribution License (CC BY). The use, distribution or reproduction in other forums is permitted, provided the original author(s) and the copyright owner(s) are credited and that the original publication in this journal is cited, in accordance with accepted academic practice. No use, distribution or reproduction is permitted which does not comply with these terms.
*Correspondence: Gaosi Xu, Z2Fvc2l4dUAxNjMuY29t
Disclaimer: All claims expressed in this article are solely those of the authors and do not necessarily represent those of their affiliated organizations, or those of the publisher, the editors and the reviewers. Any product that may be evaluated in this article or claim that may be made by its manufacturer is not guaranteed or endorsed by the publisher.
Research integrity at Frontiers
Learn more about the work of our research integrity team to safeguard the quality of each article we publish.