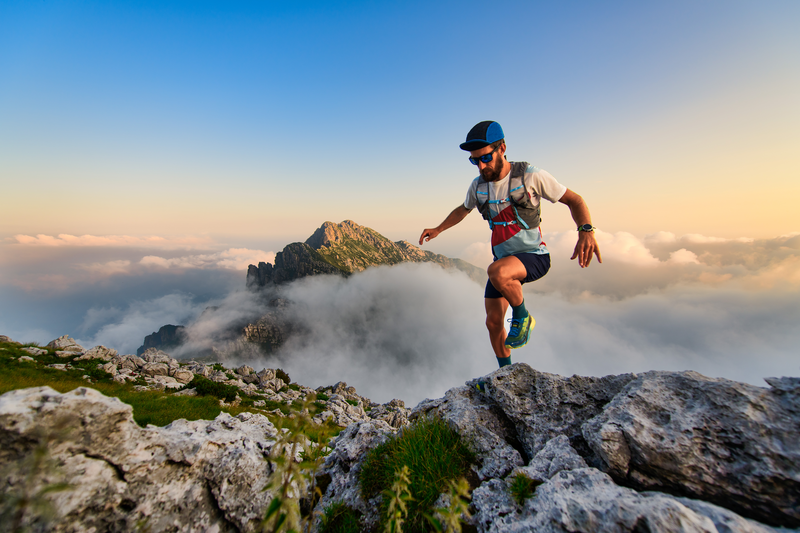
94% of researchers rate our articles as excellent or good
Learn more about the work of our research integrity team to safeguard the quality of each article we publish.
Find out more
MINI REVIEW article
Front. Med. , 22 May 2020
Sec. Pulmonary Medicine
Volume 7 - 2020 | https://doi.org/10.3389/fmed.2020.00248
This article is part of the Research Topic Coronavirus Disease (COVID-19): Pathophysiology, Epidemiology, Clinical Management and Public Health Response View all 400 articles
Angiotensin-converting enzyme 2 (ACE2) is the receptor for COVID-19 (SARs-CoV-2). ACE2 protects the lung and heart from acute respiratory distress syndrome (ARDS) and acute myocarditis and arrhythmias, because it breaks down Angiotensin II, which has inflammatory effects in the lung and heart as well as in the kidney. When SARS-CoV-2 binds to ACE2, it suppresses it, so this protective action of ACE2 is lost. Death from COVID-19 is due to ARDS and also heart failure and acute cardiac injury. Drugs that prevent the inflammatory actions of Angiotensin II (i.e., Angiotensin receptor blockers, ARBs) prevent acute lung injury caused by SARS-CoV. Clinical trials are underway to test the risks and benefits of ARBs and angiotensin-converting enzyme inhibitors (ACEIs) in COVID-19 patients requiring hospitalization. Other potential treatments are also discussed.
This article explains how the renin-angiotensin system (RAS) interacts with the severe acute respiratory syndrome coronavirus (SARS-CoV) and also with the novel coronavirus, SARS-CoV-2, which causes infection and subsequent acute lung and probably heart injury [COVID-19 (1–3)]. As well as identifying potential therapeutic strategies for treating acute lung injury and myocarditis in COVID-19 [see also (4–6)], this article provides a background to the management of patients with essential hypertension in accordance with recommendations made in the joint statement issued by the Heart Failure Society of America, the American College of Cardiology and the American Heart Association (7) that patients who are using drugs that block the RAS should continue to use them during this pandemic. It also describes why the use of angiotensin-converting enzyme inhibitors (ACEIs) and angiotensin receptor blockers (ARBs) is associated with less severe COVID-19 infections (8, 9).
There are two major arms of the RAS, one arm, the Angiotensin II (Ang II)-Ang II type 1 receptor (AT1R) pathway is pro-inflammatory and can cause acute lung injury (10, 11). The other arm, the angiotensin-converting enzyme 2 (ACE2)-Ang–(1-7)-Mas receptor (MasR) pathway is anti-inflammatory because ACE2 metabolizes Ang II, thus reducing its levels and converting it to the anti-inflammatory peptide, Ang–(1-7) [Figure 1A, (2, 12)].
Figure 1. (A) The effects of angiotensin peptides on proliferation, angiogenesis, vasoconstriction, inflammation and fibrosis. Note the effects of Angiotensin II via the Angiotensin II type I receptor (AT1R) are blocked by its interaction with the Angiotensin II type II receptor (AT2R) and by its metabolism to Ang–(1-7) acting via the Mas receptor (MasR). (B) SARS-CoV-2, by inhibiting ACE2, blocks the metabolism of Angiotensin II to Ang–(1-7) so blocking the anti-inflammatory pathway and causing high levels of Angiotensin II. Angiotensin converting enzyme inhibitors (ACEIs) prevent the formation of Angiotensin II and angiotensin receptor blocking drugs (ARBs) prevent Angiotensin II from causing inflammation and fibrosis.
ACE2 is the receptor for coronaviruses, including SARS-CoV-2 (4). As a result of SARS-CoV-2 binding to ACE2, the enzyme is no longer functional (13). Thus, the pro-inflammatory Ang II-AT1R is no longer blocked by the ACE2-Ang–(1-7)-MasR pathway and it is this imbalance that causes acute lung injury (13). A multi-centered double blind clinical trial has recently been established to test the efficacy of treating patients suffering from COVID-19 with an ARB (https://clinicaltrials.gov/ct2/show/NCT04312009). A second is investigating the outcomes of treatment of COVID-19 patients with ACEIs or ARBs (https://clinicaltrials.gov/ct2/show/NCT04331574).
Coronaviruses are a group of viruses that have in recent years caused epidemics of acute respiratory syndromes. The first major epidemic was SARS (Severe Acute Respiratory Syndrome)-CoV in 2003 which was responsible for 8,000 deaths; the second was MERS (Middle East Respiratory Syndrome), which occurred in 2012. The most recent is SARS-CoV-2 which causes COVID-19. It was first recognized in China in December 2019 and is now sweeping the world. SARS-CoV-2 is already responsible for more cases of infection and also more deaths than the two previous coronavirus epidemics. There are also a number of other coronaviruses that cause upper respiratory tract infections, in particular HCoV-NL63.
SARS-CoV and SARS-CoV-2 both enter the cell by binding to ACE2 (13, 14), as does HCoV-NL63 (15). HCoV-NL63, like SARS-CoV-2, can cause mild respiratory infections, but most commonly affects the young (16). It has, however, been associated with bronchiolitis and croup but does not cause the acute respiratory distress syndrome (ARDS) characteristic of SARS-CoV-2 infection. The differences in the severity of illness caused by these two viruses could be related to a differing affinity of the viruses to ACE2 (17) or the fact that entry of HCoV-NL63 into cells requires intracellular acidification while SARS-CoV entry can occur independent of intracellular acidification (18). MERS-CoV binds to a different receptor, Dipeptidyldipeptidase4 (DPP4) (19), and another human coronavirus, HCoV-229E, uses aminopeptidase N (APN) as its receptor (20). These receptors are membrane-bound proteases, and all can affect the production or metabolism of angiotensin peptides (21, 22).
Zhang et al. in a clinical study of 140 patients found that hypertension and diabetes were the two most common comorbidities in patients with COVID-19 (23). The increase in the prevalence of these two comorbidities in patients with severe disease was not significant when compared with those with infections that were not severe (23). In a study by Guan et al. 261 patients (23.7%) had a co-existing disorder and 21.5% of them reached the composite end-point (admission to ICU, use of mechanical ventilation or death) (24). Of the 15% that had hypertension, and 7.4% that had diabetes, 13.1 and 6.1% (respectively), of these patients reached the composite end-point. It should be noted that hypertension and diabetes are very common comorbidities, no other co-existing disorders were as prevalent in this cohort.
There has been considerable debate about the use of drugs that block the RAS in the treatment of hypertensive patients who have COVID-19 (25). This debate has not adequately considered the roles of local pulmonary and circulating RASs in the pathogenesis of COVID-19. Put simply, ACE2, the receptor for entry of SARS-CoV and SARS-CoV-2, also activates a RAS pathway that prevents acute lung injury (1, 2). These ‘Ying' and ‘Yang' actions of ACE2 have caused an apparent dilemma concerning the use of RAS blocking drugs in the treatment of hypertension and diabetes. This is because while they upregulate the SARS-CoV-2 receptor (ACE2) (a means for viral entry into cells), they also protect tissues from the pro-inflammatory actions of Ang II and could be an effective therapeutic strategy to manage COVID-19 induced lung injury [see also (4, 5)]. This seems to be the case in the light of recent clinical studies (8, 9).
Figure 1A shows the major arms of the RAS that are involved in the pathogenesis of coronavirus-induced acute lung injury. We have avoided including a number of other RAS pathways involved in the metabolism of Ang II for simplicity. A more thorough description of these pathways can be obtained elsewhere (12). As seen in Figure 1B, there are two major pathways involved in the pathogenesis of coronavirus-induced infections. Both pathways involve the formation of Ang II from Ang I, a peptide produced by the action of renin on angiotensinogen. The octapeptide, Ang II, is formed by the action of angiotensin converting enzyme (ACE) on Ang I. Ang II can bind to two receptors, the AT1R and the AT2R, or it can be broken down by number of proteases to smaller peptides that have a variety of biological actions.
The most important pathway for Ang II break down is via the removal of one amino acid from its C-terminus by a zinc-dependent carboxy peptidase, ACE2, to generate the peptide, Ang–(1-7), which acts on another receptor known as the MasR. There are other pathways that can also generate Ang–(1-7) (12). These two RAS pathways (Ang II-AT1R) and ACE2-Ang–(1-7)-MasR have opposing actions. In addition, Ang II can act via the AT2R to produce effects similar to those generated by the ACE2-Ang (1-7)-MasR pathway (12).
The Ang II-AT1R axis is well-known because it is responsible for hypertension; it raises blood pressure both through actions in the brain on the sympathetic nervous system, and in peripheral blood vessels causing vasoconstriction. This axis also controls sodium reabsorption partly through its own actions in the kidney but also because it stimulates the release of the sodium retaining hormone, aldosterone, from the adrenal gland.
There are other actions of this pathway that are pro-inflammatory, and which stimulate fibrosis. The ACE2-Ang–(1-7)-MasR pathway on the other hand lowers blood pressure, possibly through production of nitric oxide. Furthermore, the ACE2-Ang–(1-7)-MasR pathway is anti-inflammatory. Thus, ACE2 not only breaks down Ang II but also produces a vasodilator anti-inflammatory molecule, Ang–(1-7) (26).
The circulating RAS is an endocrine system capable of reaching the brain, the heart and the lungs but there are also local tissue RASs, in many organs, such as the heart, the kidney, the female reproductive tract, and the brain. There is also a local lung RAS (27) that has been implicated in the etiology of pulmonary fibrosis (11, 28).
Through a combination of circulating and tissue systems, the RAS can have powerful effects in the lungs. Renin is released from the kidneys into venous blood and renal lymphatics. It produces Ang I from angiotensinogen, which is produced by the liver. Ang I in the venous blood returning to the heart and lungs is converted in the lung by ACE to Ang II. Thus, the lung is exposed to high levels of Ang II.
The gene for ACE2 is located on Xp22 and contains 18 exons, many of which are similar to the ACE gene. It is a Zn carboxypeptidase with only one catalytic site, and it has 40% homology with ACE. It is an ectoenzyme with its N-terminus and catalytic site facing the extracellular space so it can metabolize circulating peptides (29). ACE inhibitors (ACEIs) do not affect its activity.
The spike (S) protein on the surface of the SARS-CoV-2 (COVID-19) mediates receptor recognition and membrane fusion. The spike protein is trimeric and, on fusion, separates into S1 and S2 subunits. S1 contains the receptor binding domain that directly binds to the catalytic site of ACE2. When this occurs a cleavage site on the S2 protein develops which is acted on by host proteases resulting in membrane fusion (14). The S protein of SARS-CoV-2 binds to the catalytic site of ACE2 more efficiently than does the SARS-CoV (30); unfortunately antibodies that recognize the SARS-CoV receptor binding domain (RBD) do not recognize the SARS-CoV-2 RBD (30). However, sera from convalescent SARS patients cross neutralized the SARS-spike protein (S)-driven entry into cells (31) and polyclonal murine antibodies directed against the SARS-CoV spike protein (S) potently inhibit SARS-CoV-2 S cell entry (32).
There is a widespread distribution of ACE2 throughout the body (33), including the lungs (11), heart (34, 35), and kidney (36).
Coronaviruses gain access to the body via the respiratory tract and it has recently been shown that nasal goblet cells, type II pneumocytes and ileal enterocytes all possess the necessary combination of ACE2 and TMPRSS2 for viral entry to be successful (31, 37). HCoV-NL63 binds to human airway epithelial cells in vitro (38). In the lung, ACE2 is widely distributed throughout the bronchial and pulmonary epithelium and the pulmonary capillaries (39). ACE2 protects the lung from the pro-inflammatory and pro-fibrotic actions of circulating Ang II by metabolizing Ang II to Ang–(1-7), which, acting via the MasR, inhibits Ang II-AT1R pro-inflammatory pathways [Figure 1A, (4)].
ACE2 levels vary with age, being highest in young animals and lowest in older animals, levels in older males and females being 78 and 67% lower, respectively (40). This suggests that young people are more likely to get the SARS coronavirus infection than older people, as appears to be the case. On the other hand, decreased production of ACE2 in the elderly could be one reason why coronaviruses cause more serious complications in older persons. It has to be said however, that there has not been a systematic study of the relationships between age and ACE2 expression in human tissues and, as explained below, there may be species specific differences. In the lungs (1, 2), the heart (35, 41), and the kidney, ACE2 (36) protects against the pro-inflammatory actions of Ang II acting via the AT1R.
Figure 1B shows two sites of action of drugs that block the activity of the RAS mediated by the interaction of Ang II with the AT1R. One of the two classes of drugs commonly used in clinical practice, blocks the activity of the RAS by blocking the formation of Ang II; it inhibits the activity of ACE. ACE is a Zn containing carboxypeptidase that removes a dipeptide from the C-terminal end of the decapeptide, Ang I, thus generating the active peptide responsible for most actions of the RAS, Ang II. Drugs that block this enzyme are known as ACE inhibitors (ACEIs). They have no direct effect on the activity of ACE2, except by limiting the amount of Ang II that is produced.
The second group of drugs are known as angiotensin receptor blockers (ARBs); they block the interaction of Ang II with the AT1R. ARBs do not reduce Ang II levels.
The use of these drugs could result in two significant consequences:
1. ARBs cause a rise in Ang II levels. This increase in Ang II results from blocking Ang II's effects on blood pressure and sodium and water balance and indirectly causing positive feedback on renin release. High levels of Ang II will result in increased conversion of Ang II to Ang–(1-7) by ACE2 and increased interaction of Ang II with the AT2R. This means that not only are the pro-inflammatory effects of Ang II-AT1R prevented but the anti-inflammatory effects mediated by the ACE2-Ang–(1-7)-MasR axis are enhanced and Ang II-AT2R's anti-inflammatory effects are also sustained. As well, it has been shown in the heart that ARBs cause upregulation of ACE2 because they prevent Ang II-AT1R mediated reductions in ACE2 activity (42). It is not known however, if this up-regulation of ACE2 occurs in the lungs.
2. ACEIs block the formation of Ang II, so there is no associated enhancement of any anti-inflammatory effects mediated by the ACE2-Ang–(1-7)-MasR axis and the AT2R nor would there be up-regulation of ACE2. There is only withdrawal of Ang II-AT1R's pro-inflammatory actions.
Lung ACE2 is the receptor for both SARS-CoV and SARS-CoV-2 (13, 31). The spike (S) protein binds to ACE2 and enters the cell where it is modified by a serine protease (TMPRSS2). This protease is essential for cell entry of the virus (31). The binding of coronaviruses to ACE2 and the modification of its spike protein by TMPRSS2 are essential for infection.
Binding of the SARS-CoV spike protein to ACE2 results in reduced ACE2 protein levels (13). Kuba et al. (13) showed that ACE2-/- knockout mice did not get infected with SARS-CoV and did not get acute lung inflammation (13). Thus, it would appear that low levels of pulmonary ACE2 protect against coronavirus infection. There is however a sinister side effect to the loss of pulmonary ACE2 because it plays a critical role in preventing acute lung injury.
Xie et al. suggest that there is a greater prevalence of SARS-CoV in young people because they have higher ACE2 levels (40). Yet there is a paradox, because if young people, like other mammals, have high levels of pulmonary ACE2, they should be more susceptible to symptomatic infection with coronaviruses. The prevalence of infection is however based on the appearance of symptoms and in the young, the disease is usually so mild that infection rates appear to be low. In fact, the milder nature of the disease in the young compared to the old could be a consequence of the age-dependent nature of ACE2 expression in the lung (see above). On the other hand it is possible that the animal data on ACE2 expression and age is misleading as some human studies have shown that the older the patient the higher the level of circulating ACE2 (43, 44). As stated above, there is no systematic study exploring the effects of age on ACE2 expression by human tissues. The differences between animal-based studies and human data may well be related to the short life span of animals used and the fact that they are housed in environments that protect them from infections and pollution etc.
It has also been claimed that ARBs stimulate the expression of ACE2 in the lung and that this accounts for a higher morbidity in hypertensive patients suffering from COVID-19 (25). As stated above, there is no evidence that ARBs have this effect on pulmonary ACE2, but ARBs upregulate cardiac ACE2. Ang II levels are likely to be elevated in patients treated with an ARB. As Ang II is the major substrate from which ACE2 produces Ang–(1-7) (1), it might be expected that high levels of Ang II would upregulate ACE2. However, Ang II and Ang–(1-7) have counter regulatory actions on ACE2 expression via MAP kinase/phosphatase pathways (45). Furthermore, Ang II downregulates ACE2 activity in cardiac myocytes and fibroblasts (42). Therefore, the overall effects of ARBs on pulmonary ACE2 could be modified by counteracting the effects of Ang–(1-7).
Hypertension and diabetes are the most common comorbidities found in patients suffering from COVID-19. In one study, there was no significant difference in the existence of either comorbidity between less severe and more severe cases (23). However, in a study comparing 113 patients who died with COVID-19 infections, hypertension and cardiovascular disease were more common than in patients who recovered (161). Furthermore, acute cardiac injury and heart failure, like acute respiratory distress syndrome and respiratory failure, contributed to the critical nature of the illness (46).
ACE2 protects the lung from acute lung injury because it reduces levels of Ang II by converting it to Ang–(1-7). In 2005, Imai et al. (1) showed that ACE2 played a critical role in the prevention of lung injury. Briefly, they induced acute lung injury in mice by sepsis, lipopolysaccharide (LPS) endotoxin or by acid aspiration. These treatments all caused severe lung inflammation in ACE2-/- knockout mice, which was mitigated by intraperitoneal injections of recombinant human ACE2 (1). In a second publication in 2005, Kuba et al. (13) not only showed that ACE2 was the definitive receptor for SARS-CoV, but that loss of ACE2 from the lung caused by the binding of viral spike protein was responsible for the acute lung injury caused by coronavirus infections. They also showed, as would be expected from the known actions of ACE2, that this viral infection was associated with raised Ang II levels, which caused acute lung injury via the Ang II-AT1R pathway. Treatment with the ARB, losartan, prevented SARS-CoV-induced lung injury (13).
We suggest that pulmonary ACE2 plays a critical role in protecting the lung from Ang II-AT1R induced inflammation because not only is there a local pulmonary RAS but the lung is also the major site for conversion of Ang I (which is inactive) to Ang II (47). Therefore, loss of ACE2, by binding of SARS-CoV-2, not only exposes the pulmonary epithelium to locally formed Ang II but also to Ang II formed in the lung from circulating Ang I.
Recently, a SARS-CoV-2 infected patient presented with acute heart failure a week after experiencing “flu-like symptoms” and was diagnosed with acute myocarditis without any interstitial pneumonitis (48). It is known that SARS binds to myocardial ACE2 and downregulates myocardial ACE2 protein (3). The protective effects of ACE2 in the heart are well-described (41, 49) and it is the major metabolic pathway for breakdown of Ang II in the heart (50). ACE2 protects the heart from Ang II-AT1R signaling induced injury (34).
To summarize; the data described above demonstrate that there could be a problem in treating coronavirus infections. While it is most advisable to prevent viral infection and reduce the viral load, prevention of coronavirus-associated lung and cardiac injury saves lives. The dual role of ACE2 as a receptor for the virus in the lungs and heart but a “protector” of the lungs and heart from coronavirus-induced injury has led to debate concerning the use of anti-hypertensive drugs that inhibit the RAS because they upregulate ACE2 in the lung, increase receptor availability and therefore may increase viral load.
There is no doubt, however, that Ang II-acting via the AT1R causes acute lung injury and probably cardiac injury when the ACE2-Ang–(1-7)-Mas receptor pathway is blocked by downregulation of ACE2. This pathway also protects from other forms of acute lung injury as well as SARS-CoV induced injury. ARBs would prevent downregulation of this pathway once infection has occurred and ameliorate any unopposed pro-inflammatory effects of Ang II mediated via its AT1R. ACEIs on the other hand may not upregulate ACE2 but would prevent Ang II-induced inhibition of ACE2 activity.
Since there is an approved serine protease inhibitor that could be used to block TMPRSS2, it is possible that this could be used to treat COVID-19. Another potential alternative could be to saturate the airways with recombinant ACE2 or soluble ACE2 so that viral particles are “mopped up” leaving bronchial pulmonary ACE2 intact. Treatment with recombinant human ACE2 could have the added beneficial effect of protecting the lung against lung injury.
The major problem facing treatment of coronavirus-induced lung and probably cardiac injury is that there is reduced ACE2 caused by the virus binding to ACE2. This means that Ang II is no longer metabolized by ACE2, and the anti-inflammatory Ang–(1-7) pathway is lost. Thus, lung and cardiac Ang II-AT1R pro-inflammatory pathways are activated and unopposed by the protective arm of the RAS. As suggested by Kuba et al. (13), ARBs are an appropriate adjunct therapy for treating coronavirus-induced lung injury. Recent analysis of clinical data from patients with COVID-19 support the use of RAS blocking drugs in the treatment of this infection (8, 9). Other alternatives, as suggested by Imai et al., include injection of recombinant ACE2. Haschke et al. (51) have shown the rhACE2 is well-tolerated by healthy human subjects.
The publication of the first clinical trials to test the efficacy of ARBs and ACEIs in the treatment of acute lung injury induced by SARS-CoV-2 is welcome news because these drugs are widely used clinically. If the results of these trials support the clinical data already obtained and improve the outcome of coronavirus infections, they would be of immeasurable benefit in the clinical management of this pandemic.
EL wrote the first draft of the manuscript. All authors contributed to manuscript revision, read and approved the submitted version.
The authors declare that the research was conducted in the absence of any commercial or financial relationships that could be construed as a potential conflict of interest.
We would like to thank Laureate Professor Roger Smith for his support.
1. Imai Y, Kuba K, Rao S, Huan Y, Guo F, Guan B, et al. Angiotensin-converting enzyme 2 protects from severe acute lung failure. Nature. (2005) 436:112–6. doi: 10.1038/nature03712
2. Jia H. Pulmonary angiotensin-converting enzyme 2 (ACE2) and inflammatory lung disease. Shock. (2016) 46:239–48. doi: 10.1097/SHK.0000000000000633
3. Oudit GY, Kassiri Z, Jiang C, Liu PP, Poutanen SM, Penninger JM, et al. SARS-coronavirus modulation of myocardial ACE2 expression and inflammation in patients with SARS. Eur J Clin Invest. (2009) 39:618–25. doi: 10.1111/j.1365-2362.2009.02153.x
4. Zhang H, Penninger JM, Li Y, Zhong N, Slutsky AS. Angiotensin-converting enzyme 2 (ACE2) as a SARS-CoV-2 receptor: molecular mechanisms and potential therapeutic target. Intensive Care Med. (2020) 46:586–90. doi: 10.1007/s00134-020-05985-9
5. Gurwitz D. Angiotensin receptor blockers as tentative SARS-CoV-2 therapeutics. Drug Dev Res. (2020). doi: 10.1002/ddr.21656. [Epub ahead of print].
6. Hanff TC, Harhay MO, Brown TS, Cohen JB, Mohareb AM. Is there an association between COVID-19 mortality and the renin-angiotensin system-a call for epidemiologic investigations. Clin Infect Dis. (2020) ciaa329. doi: 10.1093/cid/ciaa329. [Epub ahead of print].
7. American Heart Association The Heart Failure Society of America The American College of Cardiology. Patients Taking ACE-i and ARBs Who Contract COVID-19 Should Continue Treatment, Unless Otherwise Advised by Their Physician. (2020). Available online at: https://newsroom.heart.org/news/patients-taking-ace-i-and-arbs-who-contract-covid-19-should-continue-treatment-unless-otherwise-advised-by-their-physician.
8. Zhang P, Zhu L, Cai J, Lei F, Qin JJ, Xie J, et al. Association of inpatient use of angiotensin converting enzyme inhibitors and angiotensin ii receptor blockers with mortality among patients with hypertension hospitalized with COVID-19. Circ Res. (2020). doi: 10.1161/CIRCRESAHA.120.317134. [Epub ahead of print].
9. Ghosal S, Mukherjee JJ, Sinha B, Gangopadhyay KK. The effect of angiotensin converting enzyme inhibitors and angiotensin receptor blockers on death and severity of disease in patients with coronavirus disease 2019 (COVID-19): a meta-analysis. medRxiv [Preprint]. (2020). doi: 10.1101/2020.04.23.20076661
10. Uhal BD, Abdul-Hafez A. Angiotensin II in apoptotic lung injury: potential role in meconium aspiration syndrome. J Perinatol. (2008) 28(Suppl. 3):S108–12. doi: 10.1038/jp.2008.149
11. Uhal BD, Li X, Piasecki CC, Molina-Molina M. Angiotensin signalling in pulmonary fibrosis. Int J Biochem Cell Biol. (2012) 44:465–8. doi: 10.1016/j.biocel.2011.11.019
12. Li XC, Zhang J, Zhuo JL. The vasoprotective axes of the renin-angiotensin system: Physiological relevance and therapeutic implications in cardiovascular, hypertensive and kidney diseases. Pharmacol Res. (2017) 125:21–38. doi: 10.1016/j.phrs.2017.06.005
13. Kuba K, Imai Y, Rao S, Gao H, Guo F, Guan B, et al. A crucial role of angiotensin converting enzyme 2 (ACE2) in SARS coronavirus-induced lung injury. Nat Med. (2005) 11:875–9. doi: 10.1038/nm1267
14. Yan R, Zhang Y, Li Y, Xia L, Guo Y, Zhou Q. Structural basis for the recognition of the SARS-CoV-2 by full-length human ACE2. Science. (2020) 367:1444–8. doi: 10.1101/2020.02.19.956946
15. Wu K, Li W, Peng G, Li F. Crystal structure of NL63 respiratory coronavirus receptor-binding domain complexed with its human receptor. Proc Natl Acad Sci USA. (2009) 106:19970–4. doi: 10.1073/pnas.0908837106
16. Abdul-Rasool S, Fielding BC. Understanding human coronavirus HCoV-NL63. Open Virol J. (2010) 4:76–84. doi: 10.2174/1874357901004010076
17. Mathewson AC, Bishop A, Yao Y, Kemp F, Ren J, Chen H, et al. Interaction of severe acute respiratory syndrome-coronavirus and NL63 coronavirus spike proteins with angiotensin converting enzyme-2. J Gen Virol. (2008) 89:2741–5. doi: 10.1099/vir.0.2008/003962-0
18. Simmons G, Reeves JD, Rennekamp AJ, Amberg SM, Piefer AJ, Bates P. Characterization of severe acute respiratory syndrome-associated coronavirus (SARS-CoV) spike glycoprotein-mediated viral entry. Proc Natl Acad Sci USA. (2004) 101:4240–5. doi: 10.1073/pnas.0306446101
19. Wang N, Shi X, Jiang L, Zhang S, Wang D, Tong P, et al. Structure of MERS-CoV spike receptor-binding domain complexed with human receptor DPP4. Cell Res. (2013) 23:986–93. doi: 10.1038/cr.2013.92
20. Yeager CL, Ashmun RA, Williams RK, Cardellichio CB, Shapiro LH, Look AT, et al. Human aminopeptidase N is a receptor for human coronavirus 229E. Nature. (1992) 357:420–2. doi: 10.1038/357420a0
21. Wilson JR, Brown NJ. Examining EXAMINE for an interaction with angiotensin-converting enzyme inhibition. Hypertension. (2016) 68:549–51. doi: 10.1161/HYPERTENSIONAHA.116.07884
22. Wevers BA, van der Hoek L. Renin-angiotensin system in human coronavirus pathogenesis. Future Virol. (2010) 5:145–61. doi: 10.2217/fvl.10.4
23. Zhang JJ, Dong X, Cao YY, Yuan YD, Yang YB, Yan YQ, et al. Clinical characteristics of 140 patients infected with SARS-CoV-2 in Wuhan, China. Allergy. (2020). doi: 10.1111/all.14238. [Epub ahead of print].
24. Guan WJ, Ni ZY, Hu Y, Liang WH, Ou CQ, He JX, et al. Clinical characteristics of coronavirus disease 2019 in China. N Engl J Med. (2020) 382:1708–20. doi: 10.1101/2020.02.06.20020974
25. Diaz JH. Hypothesis: angiotensin-converting enzyme inhibitors and angiotensin receptor blockers may increase the risk of severe COVID-19. J Travel Med. (2020) taaa041. doi: 10.1093/jtm/taaa041. [Epub ahead of print].
26. Zambelli V, Bellani G, Borsa R, Pozzi F, Grassi A, Scanziani M, et al. Angiotensin-(1-7) improves oxygenation, while reducing cellular infiltrate and fibrosis in experimental acute respiratory distress syndrome. Intensive Care Med Exp. (2015) 3:44. doi: 10.1186/s40635-015-0044-3
27. Marshall RP. The pulmonary renin-angiotensin system. Curr Pharm Des. (2003) 9:715–22. doi: 10.2174/1381612033455431
28. Uhal BD, Dang M, Dang V, Llatos R, Cano E, Abdul-Hafez A, et al. Cell cycle dependence of ACE-2 explains downregulation in idiopathic pulmonary fibrosis. Eur Respir J. (2013) 42:198–210. doi: 10.1183/09031936.00015612
29. Hamming I, Cooper ME, Haagmans BL, Hooper NM, Korstanje R, Osterhaus AD, et al. The emerging role of ACE2 in physiology and disease. J Pathol. (2007) 212:1–11. doi: 10.1002/path.2162
30. Wrapp D, Wang N, Corbett KS, Goldsmith JA, Hsieh CL, Abiona O, et al. Cryo-EM structure of the 2019-nCoV spike in the prefusion conformation. Science. (2020) 367:1260–3. doi: 10.1126/science.abb2507
31. Hoffmann M, Kleine-Weber H, Schroeder S, Kruger N, Herrler T, Erichsen S, et al. SARS-CoV-2 cell entry depends on ACE2 and TMPRSS2 and Is blocked by a clinically proven protease inhibitor. Cell. (2020) 181:271–80 e8. doi: 10.1016/j.cell.2020.02.052
32. Walls AC, Park YJ, Tortorici MA, Wall A, McGuire AT, Veesler D. Structure, function, and antigenicity of the SARS-CoV-2 spike glycoprotein. Cell. (2020) 181:281–92 e6. doi: 10.1016/j.cell.2020.02.058
33. Hamming I, Timens W, Bulthuis ML, Lely AT, Navis G, van Goor H. Tissue distribution of ACE2 protein, the functional receptor for SARS coronavirus. A first step in understanding SARS pathogenesis. J Pathol. (2004) 203:631–7. doi: 10.1002/path.1570
34. Yamamoto K, Ohishi M, Katsuya T, Ito N, Ikushima M, Kaibe M, et al. Deletion of angiotensin-converting enzyme 2 accelerates pressure overload-induced cardiac dysfunction by increasing local angiotensin II. Hypertension. (2006) 47:718–26. doi: 10.1161/01.HYP.0000205833.89478.5b
35. Oudit GY, Kassiri Z, Patel MP, Chappell M, Butany J, Backx PH, et al. Angiotensin II-mediated oxidative stress and inflammation mediate the age-dependent cardiomyopathy in ACE2 null mice. Cardiovasc Res. (2007) 75:29–39. doi: 10.1016/j.cardiores.2007.04.007
36. Mori J, Patel VB, Ramprasath T, Alrob OA, DesAulniers J, Scholey JW, et al. Angiotensin 1-7 mediates renoprotection against diabetic nephropathy by reducing oxidative stress, inflammation, and lipotoxicity. Am J Physiol Renal Physiol. (2014) 306:F812–21. doi: 10.1152/ajprenal.00655.2013
37. Ziegler CGK, Allon SJ, Nyquist SK, Mbano IM, Miao VN, Tzouanas CN, et al. SARS-CoV-2 receptor ACE2 is an interferon-stimulated gene in human airway epithelial cells and is detected in specific cell subsets across tissues. Cell. (2020). doi: 10.1016/j.cell.2020.04.035. [Epub ahead of print].
38. Banach SB, Orenstein JM, Fox LM, Randell SH, Rowley AH, Baker SC. Human airway epithelial cell culture to identify new respiratory viruses: coronavirus NL63 as a model. J Virol Methods. (2009) 156:19–26. doi: 10.1016/j.jviromet.2008.10.022
39. Jia HP, Look DC, Shi L, Hickey M, Pewe L, Netland J, et al. ACE2 receptor expression and severe acute respiratory syndrome coronavirus infection depend on differentiation of human airway epithelia. J Virol. (2005) 79:14614–21. doi: 10.1128/JVI.79.23.14614-14621.2005
40. Xie X, Chen J, Wang X, Zhang F, Liu Y. Age- and gender-related difference of ACE2 expression in rat lung. Life Sci. (2006) 78:2166–71. doi: 10.1016/j.lfs.2005.09.038
41. Crackower MA, Sarao R, Oudit GY, Yagil C, Kozieradzki I, Scanga SE, et al. Angiotensin-converting enzyme 2 is an essential regulator of heart function. Nature. (2002) 417:822–8. doi: 10.1038/nature00786
42. Gallagher PE, Ferrario CM, Tallant EA. Regulation of ACE2 in cardiac myocytes and fibroblasts. Am J Physiol Heart Circ Physiol. (2008) 295:H2373–9. doi: 10.1152/ajpheart.00426.2008
43. Chen YY, Zhang P, Zhou XM, Liu D, Zhong JC, Zhang CJ, et al. Relationship between genetic variants of ACE2 gene and circulating levels of ACE2 and its metabolites. J Clin Pharm Ther. (2018) 43:189–95. doi: 10.1111/jcpt.12625
44. Soro-Paavonen A, Gordin D, Forsblom C, Rosengard-Barlund M, Waden J, Thorn L, et al. Circulating ACE2 activity is increased in patients with type 1 diabetes and vascular complications. J Hypertens. (2012) 30:375–83. doi: 10.1097/HJH.0b013e32834f04b6
45. Gallagher PE, Ferrario CM, Tallant EA. MAP kinase/phosphatase pathway mediates the regulation of ACE2 by angiotensin peptides. Am J Physiol Cell Physiol. (2008) 295:C1169–74. doi: 10.1152/ajpcell.00145.2008
46. Chen T, Wu D, Chen H, Yan W, Yang D, Chen G, et al. Clinical characteristics of 113 deceased patients with coronavirus disease 2019: retrospective study. BMJ. (2020) 368:m1091. doi: 10.1136/bmj.m1091
47. Ng KK, Vane JR. Conversion of angiotensin I to angiotensin II. Nature. (1967) 216:762–6. doi: 10.1038/216762a0
48. Inciardi RM, Lupi L, Zaccone G, Italia L, Raffo M, Tomasoni D, et al. Cardiac involvement in a patient with coronavirus disease 2019 (COVID-19). JAMA Cardiol. (2020). doi: 10.1001/jamacardio.2020.1096. [Epub ahead of print].
49. Ferrario CM, Jessup J, Chappell MC, Averill DB, Brosnihan KB, Tallant EA, et al. Effect of angiotensin-converting enzyme inhibition and angiotensin II receptor blockers on cardiac angiotensin-converting enzyme 2. Circulation. (2005) 111:2605–10. doi: 10.1161/CIRCULATIONAHA.104.510461
50. Zisman LS, Meixell GE, Bristow MR, Canver CC. Angiotensin-(1-7) formation in the intact human heart: in vivo dependence on angiotensin II as substrate. Circulation. (2003) 108:1679–81. doi: 10.1161/01.CIR.0000094733.61689.D4
Keywords: renin-angiotensin system (RAS), ACE2, lung injury, cardiovascular disease, COVID-19, SARS- CoV-2
Citation: Lumbers ER, Delforce SJ, Pringle KG and Smith GR (2020) The Lung, the Heart, the Novel Coronavirus, and the Renin-Angiotensin System; The Need for Clinical Trials. Front. Med. 7:248. doi: 10.3389/fmed.2020.00248
Received: 02 April 2020; Accepted: 11 May 2020;
Published: 22 May 2020.
Edited by:
Kian Fan Chung, Imperial College London, United KingdomReviewed by:
Koshika Yadava, University of Oxford, United KingdomCopyright © 2020 Lumbers, Delforce, Pringle and Smith. This is an open-access article distributed under the terms of the Creative Commons Attribution License (CC BY). The use, distribution or reproduction in other forums is permitted, provided the original author(s) and the copyright owner(s) are credited and that the original publication in this journal is cited, in accordance with accepted academic practice. No use, distribution or reproduction is permitted which does not comply with these terms.
*Correspondence: Eugenie R. Lumbers, ZXVnZW5pZS5sdW1iZXJzQG5ld2Nhc3RsZS5lZHUuYXU=
Disclaimer: All claims expressed in this article are solely those of the authors and do not necessarily represent those of their affiliated organizations, or those of the publisher, the editors and the reviewers. Any product that may be evaluated in this article or claim that may be made by its manufacturer is not guaranteed or endorsed by the publisher.
Research integrity at Frontiers
Learn more about the work of our research integrity team to safeguard the quality of each article we publish.