- 1Department of Dermatology, West China Hospital, Sichuan University, Chengdu, China
- 2State Key Laboratory of Biotherapy, West China Hospital, Sichuan University and Collaborative Innovation Center of Biotherapy, Chengdu, China
Rosacea is a chronic inflammatory cutaneous disorder that adversely affects patient's health and quality of life due to the complex course and the need for repeated treatment. The exact molecular mechanisms of rosacea are unclear. Mast cells are innate immune cells that can be found in virtually all tissues. Recently, increasing evidence has indicated that mast cells have important effects on the pathogenesis of rosacea. In this review article, we describe recent advances of skin mast cells in the development of rosacea. These studies suggested that mast cells can be an important immune cell that connected innate immunity, nerves, and blood vessels in the development of rosacea. Moreover, we review the inhibition of mast cells for the potential treatment of rosacea.
Introduction
Rosacea is an inflammatory skin disease that affects between 0.09 and 22.41% of the general population and 5.46% of adults worldwide (Gether, 2018 #100; Parisi, 2018 #202; Parisi, 2018 #202; Parisi, 2018 #202; Parisi, 2018 #202) (1). According to the National Rosacea Society, the primary clinical manifestations of rosacea include transient/persistent erythema and inflammatory papules/pustules with or without telangiectasia (2). Meanwhile, based on clinical features, subtypes include erythematotelangiectatic rosacea (ETR), papulopustular rosacea (PPR), phymatous rosacea (PhR)/rhinophyma, and ocular rosacea. More recently, the term “phenotype,” describing a person's observable characteristics that can be influenced by environmental or genetic factors, was proposed due to the range of manifestations of the multiple subtypes and progression between subtypes (3, 4). The diverse and overlapping clinical manifestations of rosacea have a significant psychological impact on patients, causing self-abasement, depression, anxiety, and social phobia (5). Moreover, rosacea can be a challenging condition due to its complex pathophysiology and progression among subtypes (3, 6).
The exact pathogenesis of rosacea has yet to be fully determined. Major advances have shown that rosacea results primarily from dysregulation of immune responses (innate and acquired immune responses) and/or neurovascular dysfunction with a genetic component (7, 8). Microbes, ultraviolet (UV) radiation, temperature, stress, and a damaged skin barrier can be triggers, stimulating an augmented innate immune response and/or neurovascular dysregulation (6). Cutaneous receptors, including Toll-like receptor (TLR) 2, nucleotide-binding oligomerization domain (NOD)-like receptor, and transient receptor potential vanilloid (TRPV4), are overexpressed in response to these triggers. Activation of these receptors causes the production of innate immune peptides (e.g., LL-37) and diverse cytokines and chemokines, which induce a variety of proinflammatory reactions (9). Moreover, TLR2 can activate the transcription factor nuclear factor kappa B (NF-κB), a mediator that regulates the inflammatory response (10). These events further positively affect the amplification of inflammation and the dysregulation of adaptive immunity and neurovascular changes, which promote physiological responses, including vasodilation, inflammation, fibrosis, and glandular hyperplasia, and the formation of various skin lesions (6).
Mast cells (MCs), which originate from the bone marrow CD34+ progenitor cells, reside in virtually all tissues throughout the body, particularly in the skin and mucosal surfaces (11). Therefore, MCs are one of the first cells of the immune system that interact with environmental antigens and allergens and invasive pathogens. Furthermore, studies indicate that MCs acquire the ability of an antigen presentation after activation and are associated with adaptive immunity (12, 13). Indeed, the roles of skin MCs in skin inflammation, which is involved in rosacea, have been highlighted (14). However, to our knowledge, the roles of MCs in rosacea have not yet been thoroughly reviewed. This review discusses the possible role of skin MCs during the process of rosacea, and a perspective on their inhibition for the treatment of rosacea will be summarized.
MCS Biology and Activation
MCs are hematopoietic cells and represent potential sources for a series of biologically active secreted products, including different kinds of growth factors and cytokines (15). In humans, MCs are divided into two subtypes based on the protease content of secretory granules: MCT with tryptase in human lung MCs and MCTC with both tryptase and chymase in the skin MCs (16). In mice, mucosal MCs (MMCs) are similar to MCT, and connective tissue MCs (CTMCs) are similar to MCTC (16). Both MMCs and MCT are inducible and transient, while CTMCs and MCTC are constitutive and long-lasting. Moreover, selective expression of Mas-related G protein-coupled receptor X (MRGPRX) 2 is an important characteristic of MCTC and CTMCs (17, 18). In the current review, skin MCs are of primary focus, therefore both MCTC and CTMCs will be referred to as “MCs” (skin MCs) throughout this text.
The differentiation, survival, and migration of MCs require a stem cell factor (SCF), which can be regulated by keratinocytes (19, 20). Moreover, C-X-C motif chemokine ligand 12 (CXCL12) is a chemoattractant and activator of MCs through its receptor, the C-X-C chemokine receptor (CXCR4). The activation and migration of MCs play important roles in regulating homeostasis (21). The classic pathway of MC activation is immunoglobulin E (IgE)-mediated degranulation. MCs express high-affinity IgE receptor (Fc epsilon RI or FcεRI), which can cross-link IgE with specific antigens and form IgE/FcεRI complexes, resulting in MC activation (22, 23). Other pathways also stimulate MCs (24). For example, stimulation via the IgG and Ig-free light chains (FLC) can induce MC activation as can the combination of pathogen and pattern recognition receptors (PRRs) on MCs. These PRRs include surface TLRs, endosomal TLRs, lectin-like receptor, and the nucleotide-binding oligomerization domain-like receptor family pyrin domain containing 3 (NLRP3) inflammasome (25–28). TLR2-mediated activation not only induces MC degranulation but also stimulates MCs to produce cytokines, chemokines, and eicosanoids. Dectin-1 is a member of the lectin-like receptor family and is mainly found on dendritic cells but can also be expressed by MCs. Dectin-1 can help MCs destroy Candida albicans through nitric oxide production in a TLR-2- or dectin-1-dependent pathway. NLRP3 associates with an adaptor protein and apoptosis-associated speck-like protein containing a C-terminal caspase recruitment domain (CARD) to form an inflammasome. After MC exposure to tumor necrosis factor (TNF)-α, the NLRP3 inflammasome induces NF-κB activation (27). In addition, MRGPRX2, complement receptors, neuropeptides (NPs), and neurotransmitter receptors can also participate in the activation of MCs (29, 30). Furthermore, both LL-37 and NPs can activate MCs through MRGPRX2 (31, 32).
Once activated, the MCs can promote the release of different mediators and have a considerable effect on the pathophysiology of diverse inflammatory diseases (24). First, MCs can release histamine, tryptase, and chymase, which are preformed mediators stored in granules (degranulation) that initiate the allergic cascade. Transgranulation, a variant of degranulation, occurs when MCs directly contact other cells and transfer granules into nearby cells by exocytosis (33). Second, effector molecules, including platelet-activating factor and prostaglandins, can be synthesized upon cell stimulation. Furthermore, a series of cytokines, including interleukin (IL)-1, transforming growth factor (TGF)-β, TNF-α, and vascular endothelial growth factor (VEGF), can be released that further activate MCs (34–36). These effectors can participate in numerous inflammatory responses and endothelial dysfunction in the vasculature, altering neural activity and function (37).
Role of MCS in Rosacea
The role of MCs in a broad group of inflammatory or immune-related conditions, such as asthma, allergic rhinitis urticaria, and rheumatic disease, has been reported previously (38). For the last decade and more, the functional characterization of MCs in rosacea is just emerging. A study by Aroni et al. showed that the number of MCs was significantly higher in lesions than in clinically uninvolved skin in patients with ETR and PPR, and there was a positive correlation between MC density and the duration of rosacea (39). A similar study by Schwab et al. Demonstrated, by quantitative analysis of tryptase staining, that the density of MCs was significantly increased in all subtypes of rosacea, especially PPR. Double immunofluorescence staining revealed that sensory nerves were closely associated with the blood vessels and MCs (40). Another study illustrated that quantitative analysis of tryptase staining revealed a significant increase in the ETR group compared with the control group (41). CXCL12 and its receptor C-X-C chemokine receptor 4 (CXCR4) were significantly higher in the ETR group than in the control group (41). Similarly, a study showed that the mRNA expression of chymase and metalloprotease 9 (MMP9), essential markers of MC presence and activation, was significantly elevated in rosacea skin compared to healthy skin specimens (42). Herein, MCs with their functions and molecular mechanisms characterized in rosacea are discussed in detail.
MCs Participate in Innate Immune Responses in Rosacea
Studies have demonstrated that MCs can heighten host defense by initiating inflammation associated with innate immune responses. The host defense function of MCs is mainly mediated by rapid degranulation and neutrophil recruitment. Host defense peptides (HDPs), such as cathelicidin LL-37, can cause degranulation via MRGPRX2 (31). HDPs also result in increased expression of TLR4 on MCs, which may strengthen the ability of MCs to detect invading pathogens. In addition, LL-37 can influence MC activity by inducing chemotaxis and the release of proinflammatory cytokines, such as IL-6 and MMP9. Activated MCs can also release MMP9, further promoting the production of LL-37 (42). Muto et al. performed in vitro co-culture of normal human epidermal keratinocytes (NHEKs) with supernatant from human MCs (huMCs) previously degranulated by compound 48/80. MMP and kallikrein (KLK) activities were significantly enhanced, and LL-37 mRNA levels were significantly increased. In vivo, inflammation was observed in wild-type (WT) mouse skin after the injection of LL-37 (a rosacea mouse model), whereas MC-deficient mice did not show any rosacea-like changes following LL-37 injection. The mRNA expression of MMP9 was significantly lower in MC-deficient mouse skin than in WT mouse skin upon LL-37 injection (42). Similarly, a study by Kim et al. showed that MC number and tryptase and chymase mRNA levels were significantly increased in the skin after the injection of LL-37 compared to the control (43). LL-37 activates MCs, which promote protease production and amplify inflammation, causing an innate immune response that facilitates the development of rosacea.
MCs Participate in Neurogenetic Inflammation in Rosacea
Dermal MCs are closely associated with neurogenic inflammation and mediate the stress–response network in skin inflammation. Stimulation of MCs by NPs, such as 5-hydroxytryptamine (serotonin) receptor 3A (HTR3A), pituitary adenylate cyclase-activating polypeptide (PACAP), substance P (SP), and vasoactive intestinal peptide (VIP), can cause MC degranulation and the release of histamine, tryptase and other mediators (e.g., TNF-α, CXCL9, CXCL10, and CXCL8), thereby promoting inflammation and resulting in itching, flushing, erythema, and/or burning sensations, which are common symptoms of rosacea. In turn, histamine can lead to the release of NPs at the sensory nerve endings, which creates a bidirectional loop between the MCs and sensory nerves. In vivo, tryptase, MMP1a, MMP9, CXCL2, and TNF-α mRNA expression was dramatically increased by NP stimulation in WT but not in MC-deficient mice injected with LL-37 (42). In patients with ETR, the expression levels of HTR3A, PACAP, and VIP were significantly enhanced (40). These results indicated that neurovascular changes and NP release can be translated to rosacea inflammation through the activation of MCs.
TRPV4, one member of the TRPV channels, plays a role in various sensory pathologies (44). Mascarenhas et al. found that TRPV4 was markedly increased in LL-37-injected mice. Immunofluorescence staining also showed a remarkable increase in the co-localization of MC chymase and both TRPV2 and TRPV4. LL-37 directly enhanced TRPV expression in huMCs, and TRPV4 expression is essential for the full degranulation of MCs in rosacea. Moreover, TRPV4 upregulation is dependent on MRGPRX2 in rosacea (45). In addition, the molecular inhibition of TRPV4 for 24 h before irritating cultured MCs with compound 48/80 weakened degranulation, and the knockdown of TRPV4 in huMCs reduced MC degranulation by both compound 48/80 and LL-37 (46). This evidence illustrates that MC activation stimulated by NPs or TRPV4 promotes neurogenetic inflammation associated with rosacea.
MCs Participate in Vasodilation and Angiogenesis in Rosacea
MCs can produce different proangiogenic molecules, including VEGF, fibroblast growth factor (FGF), histamine, and tryptase. First of all, these molecules directly stimulate the migration and/or proliferation of endothelial cells, thus facilitating vascularization and angiogenesis. Besides, FGFs indirectly degrade the connective tissue matrix to provide space for the formation of neovascular sprouts to promote vascularization, angiogenesis, and telangiectasias (14). In addition, histamine and 5-hydroxytryptamine can bind to the vascular receptors, causing vasodilation and increased vascular permeability (47). Vasodilation leads to erythema, and increased vascular permeability causes flushing. Furthermore, histamine causes increased blood flow and disrupts the endothelial barrier (48). These events suggest that MCs also participate in vasodilation and angiogenesis in rosacea.
MCs Participate in Fibrosis in Rosacea
Usually, skin fibrosis is clinically present in only advanced stages, especially in PhR (7). Histamine and tryptase, which are released by MCs, enhance fibrosis in rosacea through their chemotactic function on fibroblasts and MMPs (42). MCs have also been shown to promote the proliferation of fibroblasts through VEGF and basic FGF (49). In turn, fibroblasts release SCF, upregulating the expression and synthesis of monocyte chemoattractant protein-1 (MCP-1) in MCs. Moreover, MCP-1 can also act on fibroblasts, facilitating fibrosis (50). MMP activity can increase the fibrillar extracellular matrix, thereby promoting fibrosis (51). These fibrotic effects induce the clinical manifestation of rhinophyma in rosacea.
Does the Interaction Between MCS and Other Immune Cells Take Part in Rosacea?
Adaptive immunity along with the innate immune system might play a critical role in the pathophysiology of rosacea. Transcriptome analysis on facial biopsies of rosacea patients revealed significant upregulation of macrophages, neutrophils, mast cells, and T helper 1 cell/T helper 17 cell (Th1/Th17)-polarized immune cells (52). Although there are no reports on the interaction between MCs and other immune cells, including macrophages, neutrophils, and T cells in rosacea, many studies have proven that MCs can be activated by other immune cells or can activate neighboring cells through the release of inflammatory mediators (19, 53). Macrophage is an antigen-presenting cell (APC) that is regarded as a significant orchestrator of skin immunity. Neutrophil, typically the first leukocyte to be recruited to an inflammatory site, is capable of eliminating pathogens. Buhl et al. found significant upregulation of macrophage and neutrophils in PPR patients (52). TLR2 and inflammasome complex (IL-1β), accelerating inflammation activation, are expressed on the cellular membrane of macrophages (7). LL-37 and MMP9 are expressed by neutrophils recruited to tissues in response to inflammation (54). On the one hand, macrophages and MCs act as sentinel cells that initiate neutrophil recruitment via inducing increased permeability of local blood vessels and the release of chemokines. One the other hand, MC reconstitution plays an important role in the regulation of neutrophil homeostasis by affecting macrophages (55).
Previous studies revealed that T cells' response takes part in the development of rosacea. CD4+ T cells are highly present in the rosacea skin. Th1 cells along with Th17 cells, differentiated from CD4+ T cells, are increased in the skin tissues of rosacea (52, 56). The release of interferon-γ (IFN-γ) and IL-17 from the T cells stimulates the production of inflammatory lesions and angiogenesis in rosacea (57). The processing and presentation of antigenic peptides on MCs lead to the expansion of CD4+ T cells. In turn, T cells and surface molecules on membrane vesicles secreted can activate MCs, suggesting a bidirectional relationship (58). Based on these evidences in MCs network, we suggest that the possible interaction between MCs and other immune cells especially macrophages, neutrophils, and Th1/Th17 cells may also participate in the pathogenesis of rosacea; although this would require further studies.
Inhibition of MCS For Rosacea Treatment
Cromolyn
Cromolyn sodium can inhibit the release of histamine and other inflammatory mediators from sensitized MCs (59). It is widely used in the management of chronic asthma, mastocytosis, seasonal and perennial allergic rhinitis, allergic conjunctivitis, and vernal keratoconjunctivitis (59, 60). In vivo, rosacea-like inflammation did not occur in mice pretreated with cromolyn and then challenged with LL-37. The mRNA levels of MMP9 and CXCL2 were significantly reduced in the cromolyn-treated mice compared to those injected with LL-37 alone. MMP activity in the skin was also dramatically weakened by cromolyn pretreatment (42). Furthermore, 10 patients with ETR were chosen to topically apply either 4% cromolyn sodium or placebo on their faces. After 8 weeks, only the cromolyn treatment group showed decreased facial erythema. In addition, MMP activity was markedly decreased in the cromolyn treatment group with mildly decreased KLK activity and LL-37 protein levels (42). These results indicate that cromolyn or other MC stabilizers may be a potential therapy for rosacea, especially ETR, through the inhibition of MC activation.
Other Drugs Inhibited the Number or Activity of MCs
Brimonidine, a highly selective α2-adrenergic receptor agonist that binds alpha 2 receptors on the vasculature and causes direct vasoconstriction of both small arteries and veins, is approved for the treatment of rosacea with facial erythema by the Food and Drug Administration (61, 62). A study by Kim et al. showed that clinical manifestations of rosacea induced by LL-37 injection in mice were ameliorated after topical application of brimonidine tartrate 0.33% gel, with a noteworthy histological decrease in the number of MCs. Furthermore, the LL-37-induced increases in the mRNA levels of tryptase and chymase were significantly reduced after the use of brimonidine gel (43). Although the relationship between brimonidine and MCs is unclear, these results suggest that the application of brimonidine may decrease the recruitment of MCs in rosacea.
Botulinum toxin (BoNT) A, which is produced by the bacterium Clostridium botulinum, can prevent the release of acetylcholine from the presynaptic vesicle and modulate SP and VIP, reducing local inflammation around the nerve endings, deactivating the sodium channel, and exhibiting axonal transport (63). Intradermal BoNT A has been regarded as a novel therapy for ETR and PPR (64, 65). Recently, Choi et al. investigated the direct action of BoNT A on MCs in a rosacea mouse model (66). BoNT A reduced MC degranulation both in vivo and in vitro. There was significantly less erythema in mice pretreated with intradermal BoNT A than in control mice; both groups were injected with LL-37. Additionally, the intradermal BoNT A pretreatment group displayed a reduction in dermal MC degranulation in comparison with the control (66). This study indicates that BoNT A can reduce rosacea-associated skin inflammation through direct inhibition of MC degranulation.
Conclusion and Perspective
Rosacea is a complex disorder caused by immune dysfunction and neurovascular dysregulation involving many types of inflammatory cells. In the current review, we describe in detail the roles of MCs in the pathophysiology of rosacea (Figure 1). MCs participate in the pathogenesis of rosacea through innate immune responses, neurogenetic inflammation, angiogenesis, and fibrosis. This review illustrated that MCs can be important immune cells that connect innate immunity, nerves, and blood vessels in the development of rosacea, no matter the subtypes. Although there were no reports on the relationship between MCs and other immune cells, the cellular network indicates that MCs might also play a role in rosacea through interaction with other cells; further studies are needed to focus on this field.
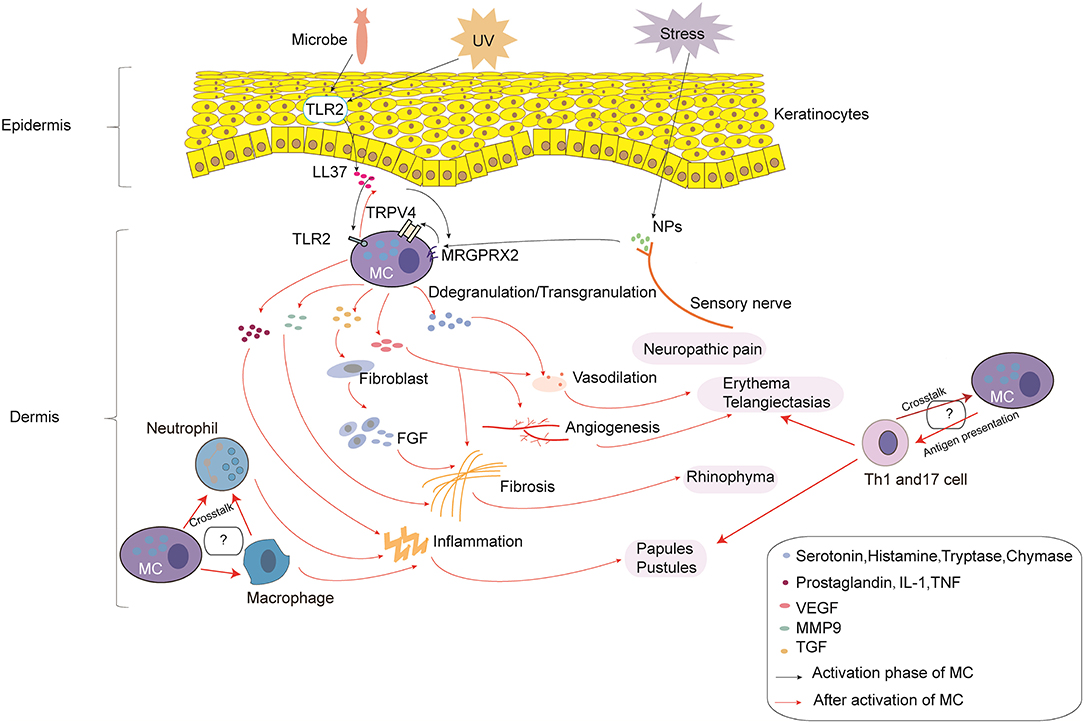
Figure 1. The roles of mast cells (MCs) in the pathophysiology of rosacea. In the process of MC activation, triggers (e.g., microbes and UV) can induce the production of LL-37 by keratinocytes. In addition, stress can induce the production of NPs by sensory nerves. LL-37 promotes the activation of MCs through binding to TLR2 on MCs, which can enhance the production of LL-37. LL-37 and NPs can also promote the activation of MCs through MRGPRX2, which promotes the activation of TRPV4 on MCs. After activation, MC degranulation/transgranulation secretes a series of cytokines and mediators, thereby facilitating vasodilation, inflammation, and angiogenesis, which results in neuropathic pain, erythema, telangiectasias, and the formation of papules and pustules. Moreover, MMP9 produced by MCs directly promotes fibrosis, and cytokines and mediators produced by MCs indirectly promote fibrosis through fibroblasts, which secrete FGF. Furthermore, the cross-talk with macrophages, neutrophils, and Th1/Th17 cells may also take part in the pathophysiology of rosacea, which needs further study.
The waxing and waning course of rosacea has led to the necessary development of novel therapies. Cromolyn sodium or other MC stabilizers affect the release of histamine and other inflammatory mediators from MCs to ameliorate erythema in ETR. Drugs including brimonidine and BoNT can also reduce the recruitment of MCs and inhibit MC degranulation to improve inflammation associated with rosacea. We believe that the important role of MCs makes it a potential target for drug therapy in rosacea; more studies are needed to evaluate the efficacy for its inhibition in the treatment of rosacea.
In conclusion, this work makes a clear summary of the relevant literature and a proposal for a mastocyte-focused therapeutic approach. The limitation is that although we concluded that MCs may interact with other cells to facilitate the development of rosacea, discussion of this mechanism in the broader complex relationships is limited in the diseased skin.
Author Contributions
GH and XJ contributed to determine the content of each section and edited the manuscript. LW, Y-JW, DH, XW, and DD collected the data and wrote each section of the manuscript. All authors read and approved the final manuscript.
Funding
This work was financially supported by the National Natural Science Foundation of China (Grant Nos. 21772131, 81872535, and 81472162).
Conflict of Interest
The authors declare that the research was conducted in the absence of any commercial or financial relationships that could be construed as a potential conflict of interest.
Abbreviations
APC, antigen-presenting cell; BoNT, botulinum toxin; CARD, caspase recruitment domain; CXCL, C-X-C motif chemokine ligand; CXCR, C-X-C chemokine receptor; ETR, erythematotelangiectatic rosacea; FGF, fibroblast growth factor; FLC, Ig-free light chains; FcεRI, Fc epsilon RI; HDPs, host defense peptides; HTR3A, 5-hydroxytryptamine (serotonin) receptor 3A; IFN-γ, interferon-γ; IgE, immunoglobulin E; IL, interleukin; KLK, kallikrein; MC, mast cell; MCP-1, monocyte chemoattractant protein-1; MMP9, metalloprotease 9; MRGPRX, Mas-related G protein-coupled receptor X; NLRP3, the nucleotide-binding oligomerization domain-like receptor (NLR) family pyrin domain containing 3; NF-κB, nuclear factor kappa B; NPs, neuropeptides; PACAP, pituitary adenylate cyclase-activating polypeptide; PhR, phymatous rosacea; PPR, papulopustular rosacea; PRRs, pattern recognition receptors; SCF, stem cell factor; SP, substance P; TGF-β, transforming growth factor; Th1 cell, T helper 1 cell; Th17 cell, T helper 17 cell; TLR, toll-like receptor; TNF-α, tumor necrosis factor-α; TRPV, transient receptor potential vanilloid; UV, ultraviolet; VEGF, vascular endothelial growth factor; VIP, vasoactive intestinal peptide; WT, wild-type.
References
1. Parisi R, Yiu ZZN. The worldwide epidemiology of rosacea. Br J Dermatol. (2018) 179:239–40. doi: 10.1111/bjd.16788
2. Wilkin J, Dahl M, Detmar M, Drake L, Liang MH, Odom R, et al. Standard grading system for rosacea: report of the National Rosacea Society Expert Committee on the classification and staging of rosacea. J Am Acad Dermatol. (2004) 50:907–12. doi: 10.1016/j.jaad.2004.01.048
3. Tan J, Almeida LM, Bewley A, Cribier B, Dlova NC, Gallo R, et al. Updating the diagnosis, classification and assessment of rosacea: recommendations from the global ROSacea COnsensus (ROSCO) panel. Br J Dermatol. (2017) 176:431–8. doi: 10.1111/bjd.15122
4. Schaller M, Almeida LMC, Bewley A, Cribier B, Del Rosso J, Dlova NC, et al. Recommendations for rosacea diagnosis, classification and management: update from the global ROSacea COnsensus 2019 panel. Br J Dermatol. (2019). doi: 10.1111/bjd.18420. [Epub ahead of print].
5. Wu Y, Fu C, Zhang W, Li C, Zhang J. The dermatology life quality index (DLQI) and the hospital anxiety and depression (HADS) in Chinese rosacea patients. Psychol Health Med. (2018) 23:369–74. doi: 10.1080/13548506.2017.1361540
6. Rainer BM, Kang S, Chien AL. Rosacea: epidemiology, pathogenesis, and treatment. Dermatoendocrinology. (2017) 9:e1361574. doi: 10.1080/19381980.2017.1361574
7. Holmes AD, Steinhoff M. Integrative concepts of rosacea pathophysiology, clinical presentation and new therapeutics. Exp Dermatol. (2017) 26:659–67. doi: 10.1111/exd.13143
8. Kulkarni NN, Takahashi T, Sanford JA, Tong Y, Gombart AF, Hinds B, et al. Innate immune dysfunction in rosacea promotes photosensitivity and vascular adhesion molecule expression. J Invest Dermatol. (2019). doi: 10.1016/j.jid.2019.08.436
9. Yamasaki K, Kanada K, Macleod DT, Borkowski AW, Morizane S, Nakatsuji T, et al. TLR2 expression is increased in rosacea and stimulates enhanced serine protease production by keratinocytes. J Invest Dermatol. (2011) 131:688–97. doi: 10.1038/jid.2010.351
10. Baylie RL, Brayden JE. TRPV channels and vascular function. Acta Physiol (Oxf). (2011) 203:99–116. doi: 10.1111/j.1748-1716.2010.02217.x
11. Choi HW, Abraham SN. In vitro and in vivo IgE-/antigen-mediated mast cell activation. Methods Mol Biol. (2018) 1799:71–80. doi: 10.1007/978-1-4939-7896-0_7
12. Cardamone C, Parente R, Feo GD, Triggiani M. Mast cells as effector cells of innate immunity and regulators of adaptive immunity. Immunol Lett. (2016) 178:10–4. doi: 10.1016/j.imlet.2016.07.003
13. Igawa S, Di Nardo A. Skin microbiome and mast cells. Transl Res. (2017) 184:68–76. doi: 10.1016/j.trsl.2017.03.003
14. Choi JE, Di Nardo A. Skin neurogenic inflammation. Semin Immunopathol. (2018) 40:249–59. doi: 10.1007/s00281-018-0675-z
15. Germic N, Frangez Z, Yousefi S, Simon HU. Regulation of the innate immune system by autophagy: neutrophils, eosinophils, mast cells, NK cells. Cell Death Differ. (2019) 26:703–14. doi: 10.1038/s41418-019-0295-8
16. Gurish MF, Austen KF. Developmental origin and functional specialization of mast cell subsets. Immunity. (2012) 37:25–33. doi: 10.1016/j.immuni.2012.07.003
17. Fujisawa D, Kashiwakura J, Kita H, Kikukawa Y, Fujitani Y, Sasaki-Sakamoto T, et al. Expression of Mas-related gene X2 on mast cells is upregulated in the skin of patients with severe chronic urticaria. J Allergy Clin Immunol. (2014) 134:622–33.e9. doi: 10.1016/j.jaci.2014.05.004
18. Dwyer DF, Barrett NA, Austen KF. Expression profiling of constitutive mast cells reveals a unique identity within the immune system. Nat Immunol. (2016) 17:878–87. doi: 10.1038/ni.3445
19. Cho KA, Park M, Kim YH, Woo SY. Th17 cell-mediated immune responses promote mast cell proliferation by triggering stem cell factor in keratinocytes. Biochem Biophys Res Commun. (2017) 487:856–61. doi: 10.1016/j.bbrc.2017.04.141
20. Halova I, Draberova L, Draber P. Mast cell chemotaxis–chemoattractants and signaling pathways. Front Immunol. (2012) 3:119. doi: 10.3389/fimmu.2012.00119
21. Yang HW, Liu XY, Shen ZF, Yao W, Gong XB, Huang HX, et al. An investigation of the distribution and location of mast cells affected by the stiffness of substrates as a mechanical niche. Int J Biol Sci. (2018) 14:1142–52. doi: 10.7150/ijbs.26738
22. Blank U, Madera-Salcedo IK, Danelli L, Claver J, Tiwari N, Sanchez-Miranda E, et al. Vesicular trafficking and signaling for cytokine and chemokine secretion in mast cells. Front Immunol. (2014) 5:453. doi: 10.3389/fimmu.2014.00453
23. Kraft S, Kinet JP. New developments in FcepsilonRI regulation, function and inhibition. Nat Rev Immunol. (2007) 7:365–78. doi: 10.1038/nri2072
24. Redegeld FA, Yu Y, Kumari S, Charles N, Blank U. Non-IgE mediated mast cell activation. Immunol Rev. (2018) 282:87–113. doi: 10.1111/imr.12629
25. Zhang YY, Yu YY, Zhang YR, Zhang W, Yu B. The modulatory effect of TLR2 on LL-37-induced human mast cells activation. Biochem Biophys Res Commun. (2016) 470:368–74. doi: 10.1016/j.bbrc.2016.01.037
26. Kimura Y, Chihara K, Honjoh C, Takeuchi K, Yamauchi S, Yoshiki H, et al. Dectin-1-mediated signaling leads to characteristic gene expressions and cytokine secretion via spleen tyrosine kinase (Syk) in rat mast cells. J Biol Chem. (2014) 289:31565–75. doi: 10.1074/jbc.M114.581322
27. Nakamura Y, Franchi L, Kambe N, Meng G, Strober W, Nunez G. Critical role for mast cells in interleukin-1beta-driven skin inflammation associated with an activating mutation in the nlrp3 protein. Immunity. (2012) 37:85–95. doi: 10.1016/j.immuni.2012.04.013
28. Pinke KH, Lima HG, Cunha FQ, Lara VS. Mast cells phagocyte Candida albicans and produce nitric oxide by mechanisms involving TLR2 and Dectin-1. Immunobiology. (2016) 221:220–7. doi: 10.1016/j.imbio.2015.09.004
29. Subramanian H, Gupta K, Ali H. Roles of Mas-related G protein-coupled receptor X2 on mast cell-mediated host defense, pseudoallergic drug reactions, and chronic inflammatory diseases. J Allergy Clin Immunol. (2016) 138:700–10. doi: 10.1016/j.jaci.2016.04.051
30. Rosa AC, Fantozzi R. The role of histamine in neurogenic inflammation. Br J Pharmacol. (2013) 170:38–45. doi: 10.1111/bph.12266
31. Gupta K, Subramanian H, Ali H. Modulation of host defense peptide-mediated human mast cell activation by LPS. Innate Immun. (2016) 22:21–30. doi: 10.1177/1753425915610643
32. Subramanian H, Gupta K, Guo Q, Price R, Ali H. Mas-related gene X2 (MrgX2) is a novel G protein-coupled receptor for the antimicrobial peptide LL-37 in human mast cells: resistance to receptor phosphorylation, desensitization, and internalization. J Biol Chem. (2011) 286:44739–49. doi: 10.1074/jbc.M111.277152
33. Kunder CA, St John AL, Abraham SN. Mast cell modulation of the vascular and lymphatic endothelium. Blood. (2011) 118:5383–93. doi: 10.1182/blood-2011-07-358432
34. Krystel-Whittemore M, Dileepan KN, Wood JG. Mast cell: a multi-functional master cell. Front Immunol. (2015) 6:620. doi: 10.3389/fimmu.2015.00620
35. Beghdadi W, Madjene LC, Benhamou M, Charles N, Gautier G, Launay P, et al. Mast cells as cellular sensors in inflammation and immunity. Front Immunol. (2011) 2:37. doi: 10.3389/fimmu.2011.00037
36. Taracanova A, Alevizos M, Karagkouni A, Weng Z, Norwitz E, Conti P, et al. SP and IL-33 together markedly enhance TNF synthesis and secretion from human mast cells mediated by the interaction of their receptors. Proc Natl Acad Sci USA. (2017) 114:E4002–9. doi: 10.1073/pnas.1524845114
37. Mittal A, Sagi V, Gupta M, Gupta K. Mast cell neural interactions in health and disease. Front Cell Neurosci. (2019) 13:110. doi: 10.3389/fncel.2019.00110
38. Galli SJ. Mast cells and KIT as potential therapeutic targets in severe asthma. N Engl J Med. (2017) 376:1983–4. doi: 10.1056/NEJMe1702653
39. Aroni K, Tsagroni E, Kavantzas N, Patsouris E, Ioannidis E. A study of the pathogenesis of rosacea: how angiogenesis and mast cells may participate in a complex multifactorial process. Arch Dermatol Res. (2008) 300:125–31. doi: 10.1007/s00403-007-0816-z
40. Schwab VD, Sulk M, Seeliger S, Nowak P, Aubert J, Mess C, et al. Neurovascular and neuroimmune aspects in the pathophysiology of rosacea. J Investig Dermatol Symp Proc. (2011) 15:53–62. doi: 10.1038/jidsymp.2011.6
41. Helfrich YR, Maier LE, Cui Y, Fisher GJ, Chubb H, Fligiel S, et al. Clinical, histologic, and molecular analysis of differences between erythematotelangiectatic rosacea and telangiectatic photoaging. JAMA Dermatol. (2015) 151:825–36. doi: 10.1001/jamadermatol.2014.4728
42. Muto Y, Wang Z, Vanderberghe M, Two A, Gallo RL, Di Nardo A. Mast cells are key mediators of cathelicidin-initiated skin inflammation in rosacea. J Invest Dermatol. (2014) 134:2728–36. doi: 10.1038/jid.2014.222
43. Kim M, Kim J, Jeong SW, Jo H, Woo YR, Park HJ. Inhibition of mast cell infiltration in an LL-37-induced rosacea mouse model using topical brimonidine tartrate 0.33% gel. Exp Dermatol. (2017) 26:1143–5. doi: 10.1111/exd.13381
44. Akiyama T, Ivanov M, Nagamine M, Davoodi A, Carstens MI, Ikoma A, et al. Involvement of TRPV4 in serotonin-evoked scratching. J Invest Dermatol. (2016) 136:154–60. doi: 10.1038/jid.2015.388
45. Mascarenhas NL, Wang Z, Chang YL, Di Nardo A. TRPV4 mediates mast cell activation in cathelicidin-induced rosacea inflammation. J Invest Dermatol. (2017) 137:972–5. doi: 10.1016/j.jid.2016.10.046
46. Chen Y, Moore CD, Zhang JY, Hall RP III, MacLeod AS, Liedtke W. TRPV4 moves toward center-fold in rosacea pathogenesis. J Invest Dermatol. (2017) 137:801–4. doi: 10.1016/j.jid.2016.12.013
48. Ashina K, Tsubosaka Y, Nakamura T, Omori K, Kobayashi K, Hori M, et al. Histamine induces vascular hyperpermeability by increasing blood flow and endothelial barrier disruption in vivo. PLoS ONE. (2015) 10:e0132367. doi: 10.1371/journal.pone.0132367
49. Tellechea A, Leal EC, Kafanas A, Auster ME, Kuchibhotla S, Ostrovsky Y, et al. Mast cells regulate wound healing in diabetes. Diabetes. (2016) 65:2006–19. doi: 10.2337/db15-0340
50. Yamamoto T, Hartmann K, Eckes B, Krieg T. Role of stem cell factor and monocyte chemoattractant protein-1 in the interaction between fibroblasts and mast cells in fibrosis. J Dermatol Sci. (2001) 26:106–11. doi: 10.1016/s0923-1811(00)00164-x
51. Robert S, Gicquel T, Victoni T, Valenca S, Barreto E, Bailly-Maitre B, et al. Involvement of matrix metalloproteinases (MMPs) and inflammasome pathway in molecular mechanisms of fibrosis. Biosci Rep. (2016) 36:e00360. doi: 10.1042/bsr20160107
52. Buhl T, Sulk M, Nowak P, Buddenkotte J, McDonald I, Aubert J, et al. Molecular and morphological characterization of inflammatory infiltrate in rosacea reveals activation of Th1/Th17 pathways. J Invest Dermatol. (2015) 135:2198–208. doi: 10.1038/jid.2015.141
53. Stassen M, Hartmann AK, Delgado SJ, Dehmel S, Braun A. Mast cells within cellular networks. J Allergy Clin Immunol. (2019) 144:S46–54. doi: 10.1016/j.jaci.2019.01.031
54. Kolaczkowska E, Kubes P. Neutrophil recruitment and function in health and inflammation. Nat Rev Immunol. (2013) 13:159–75. doi: 10.1038/nri3399
55. Jachetti E, D'Inca F, Danelli L, Magris R, Dal Secco C, Vit F, et al. Frontline science: mast cells regulate neutrophil homeostasis by influencing macrophage clearance activity. J Leukoc Biol. (2019) 105:633–44. doi: 10.1002/jlb.4hi1018-390r
56. Brown TT, Choi EY, Thomas DG, Hristov AC, Chan MP. Comparative analysis of rosacea and cutaneous lupus erythematosus: histopathologic features, T-cell subsets, and plasmacytoid dendritic cells. J Am Acad Dermatol. (2014) 71:100–7. doi: 10.1016/j.jaad.2014.01.892
57. Amir Ali A, Vender R, Vender R. The role of IL-17 in papulopustular rosacea and future directions. J Cutan Med Surg. (2019) 23:635–41. doi: 10.1177/1203475419867611
58. Bulfone-Paus S, Bahri R. Mast cells as regulators of T cell responses. Front Immunol. (2015) 6:394. doi: 10.3389/fimmu.2015.00394
59. Zhang T, Finn DF, Barlow JW, Walsh JJ. Mast cell stabilisers. Eur J Pharmacol. (2016) 778:158–68. doi: 10.1016/j.ejphar.2015.05.071
60. Fathy ME, Abo El Abass Mohamed S, Elmansi H, Belal F. Simultaneous determination of cromolyn sodium combined dosage forms using isocratic HPLC method. J Chromatogr Sci. (2017) 55:14–22. doi: 10.1093/chromsci/bmw142
61. Piwnica D, Rosignoli C, de Menonville ST, Alvarez T, Schuppli Nollet M, Roye O, et al. Vasoconstriction and anti-inflammatory properties of the selective alpha-adrenergic receptor agonist brimonidine. J Dermatol Sci. (2014) 75:49–54. doi: 10.1016/j.jdermsci.2014.04.002
62. Del Rosso JQ. Advances in understanding and managing rosacea: part 2: the central role, evaluation, and medical management of diffuse and persistent facial erythema of rosacea. J Clin Aesthet Dermatol. (2012) 5:26–36.
63. Park J, Park HJ. Botulinum toxin for the treatment of neuropathic pain. Toxins (Basel). (2017) 9:E260. doi: 10.3390/toxins9090260
64. Bloom BS, Payongayong L, Mourin A, Goldberg DJ. Impact of intradermal abobotulinumtoxinA on facial erythema of rosacea. Dermatol Surg. (2015) 41:S9–16. doi: 10.1097/dss.0000000000000277
65. Bharti J, Sonthalia S, Jakhar D. Mesotherapy with botulinum toxin for the treatment of refractory vascular and papulopustular rosacea. J Am Acad Dermatol. (2018). doi: 10.1016/j.jaad.2018.05.014
Keywords: mast cell, rosacea, pathophysiology, treatment, dermatology
Citation: Wang L, Wang Y-J, Hao D, Wen X, Du D, He G and Jiang X (2020) The Theranostics Role of Mast Cells in the Pathophysiology of Rosacea. Front. Med. 6:324. doi: 10.3389/fmed.2019.00324
Received: 22 October 2019; Accepted: 18 December 2019;
Published: 28 January 2020.
Edited by:
Ivan V. Litvinov, McGill University, CanadaReviewed by:
Alejandro Pablo Adam, Albany Medical College, United StatesKevin Pehr, McGill University, Canada
Copyright © 2020 Wang, Wang, Hao, Wen, Du, He and Jiang. This is an open-access article distributed under the terms of the Creative Commons Attribution License (CC BY). The use, distribution or reproduction in other forums is permitted, provided the original author(s) and the copyright owner(s) are credited and that the original publication in this journal is cited, in accordance with accepted academic practice. No use, distribution or reproduction is permitted which does not comply with these terms.
*Correspondence: Gu He, aGVndSYjeDAwMDQwO3NjdS5lZHUuY24=; Xian Jiang, amVubnl4aWFuaiYjeDAwMDQwOzE2My5jb20=