- 1Department of Pharmacy, Pharmaceutical Biology, Saarland University, Saarbrücken, Germany
- 2Center for Bioinformatics, Saarland University, Saarbrücken, Germany
- 3Department of Computer Science, German Jordanian University, Amman, Jordan
- 4Genetics/Epigenetics, Saarland University, Saarbrücken, Germany
- 5Department of Pathology, Medical Faculty, Otto von Guericke University Magdeburg, Magdeburg, Germany
- 6Institute of Clinical-Experimental Surgery, Saarland University Hospital, Homburg, Germany
- 7Systems Toxicology, Leibniz Research Centre for Working Environment and Human Factors (IfADo) at the TU Dortmund, Dortmund, Germany
- 8Laboratory of Hepato-Gastroenterology, Institut de Recherche Expérimentale et Clinique, Université Catholique de Louvain, Brussels, Belgium
- 9Department of Biosciences and Nutrition, Karolinska Institutet, Huddinge, Sweden
- 10Center for Molecular Medicine Cologne (CMMC), Institute of Neurophysiology, University of Cologne, Cologne, Germany
- 11Institute of Pathology, Medical University of Graz, Graz, Austria
- 12Department of Pathology, Medical University Innsbruck, Innsbruck, Austria
The insulin-like growth factor 2 (IGF2) mRNA binding proteins (IMPs/IGF2BPs) IMP1 and 3 are regarded as oncofetal proteins, whereas the hepatic IMP2 expression in adults is controversially discussed. The splice variant IMP2-2/p62 promotes steatohepatitis and hepatocellular carcinoma. Aim of this study was to clarify whether IMP2 is expressed in the adult liver and influences progression toward cirrhosis. IMP2 was expressed at higher levels in embryonic compared to adult tissues as quantified in embryonic, newborn, and adult C57BL/6J mouse livers and suggested by analysis of publicly available human data. In an IMP2-2 transgenic mouse model microarray and qPCR analyses revealed increased expression of liver progenitor cell (LPC) markers Bex1, Prom1, Spp1, and Cdh1 indicating a de-differentiated liver cell phenotype. Induction of these LPC markers was confirmed in human cirrhotic tissue datasets. The LPC marker SPP1 has been described to play a major role in fibrogenesis. Thus, DNA methylation was investigated in order to decipher the regulatory mechanism of Spp1 induction. In IMP2-2 transgenic mouse livers single CpG sites were differentially methylated, as quantified by amplicon sequencing, whereas human HCC samples of a human publicly available dataset showed promoter hypomethylation. In order to study the impact of IMP2 on fibrogenesis in the context of steatohepatitis wild-type or IMP2-2 transgenic mice were fed either a methionine-choline deficient (MCD) or a control diet for 2–12 weeks. MCD-fed IMP2-2 transgenic mice showed a higher incidence of ductular reaction (DR), accompanied by hepatic stellate cell activation, extracellular matrix (ECM) deposition, and induction of the LPC markers Spp1, Cdh1, and Afp suggesting the occurrence of de-differentiated cells in transgenic livers. In human cirrhotic samples IMP2 overexpression correlated with LPC marker and ECM component expression. Progression of liver disease was induced by combined MCD and diethylnitrosamine (DEN) treatment. Combined MCD-DEN treatment resulted in shorter survival of IMP2-2 transgenic compared to wild-type mice. Only IMP2-2 transgenic livers progressed to cirrhosis, which was accompanied by strong DR. In conclusion, IMP2 is an oncofetal protein in the liver that promotes DR characterized by de-differentiated cells toward steatohepatitis-associated cirrhosis development with poor survival.
Introduction
The family of insulin-like growth factor (IGF) 2 mRNA binding proteins (IMPs/IGF2BPs), namely IMP1 and IMP3, have been described as oncofetal proteins. However, whether IMP2 is an oncofetal protein or not remains a matter of debate (1–3). IMP2 has been described to be essential for preserving glioblastoma cancer stem cells (4). It is known that the occurrence of hepatic stem cells, i.e., liver progenitor cells (LPCs) worsens prognosis in liver disease. Noteworthy, more than half of the earliest hepatic premalignant lesions consist of LPCs and intermediate hepatocytes (5). Human and murine HCCs show LPC gene signatures and contain LPC fractions (6). Concordantly, p53-deficient or H-RAS/SV40LT-transduced isolated LPCs can generate HCC in xenotransplant models (7, 8). In cholangiocarcinoma aberrant expression of stem cell markers suggest LPCs to be the cell of origin in tumors (9). Interestingly, mixed types of cholangiocarcinoma and HCC have been described in the literature, which are thought to derive from transformed progenitor cells (10). Thus, it has been suggested that LPCs may be precursor cells for malignant transformation (11). However, recent evidence using lineage tracing in different mouse models demonstrates that HCC rather seem to develop from dedifferentiated hepatocytes than from LPCs (12, 13).
Still, the majority of non-malignant chronic liver diseases are characterized by LPC activation (5). In ductular reaction (DR), activated LPCs organize in strings (14) and finally lead to increased numbers of bile ducts that form a denser mesh around portal vein branches (15) or even infiltrate into the lobular parenchyme (16). Interestingly, the degree of DR correlates with disease progression (17). DR comparable to the one found in human non-alcoholic steatohepatitis (NASH) can be induced by a methionine-choline deficient diet (MCD) showing high similarity with NASH samples with advanced fibrosis (18).
In this study, we confirm the oncofetal appearance of IMP2 in murine and human liver. Employing transgenic mice we show that IMP2-2 is able to promote DR resulting in cirrhosis and short survival in the steatohepatitis model. Human gene expression data further support the association of IMP2 with marker genes of undifferentiated or dedifferentiating cells in liver disease.
Methods
Animals
Liver tissue from C57BL/6J mice at embryonic day E12.5 (n = 5), newborns (P0, n = 8), and at the age of 2, 10, and 85 weeks (each n = 6) were obtained. Publicly available RNA sequencing data sets from murine (PRJNA66167) and human (PRJNA280600) fetal and adult liver (19) were analyzed.
Wildtype (wt) and IMP2-2 transgenic (tg) mice were generated as previously described (20). At the age of 5 weeks, livers were examined by qPCR (wt: n = 13, tg: n = 13) and microarray analysis (wt: n = 10, tg: n = 10). In order to induce NASH, wt and IMP2-2 tg mice were randomly divided into experimental groups at the age of 3 weeks and were fed either a methionine choline deficient diet (MCD, #960439, MP Biomedicals, Germany) or a MCD diet supplemented with choline bitartrate (2 g/kg) and DL-methionine (3 g/kg) (MCS, #960441, MP Biomedicals, Germany) for 2, 4, or 12 weeks (21) (2 weeks: MCS: wt: 5 females and 5 males; tg: 6 females and 4 males; MCD: wt: 5 females and 7 males; tg: 7 females and 5 males; 4 weeks: MCS: wt: 5 females and 5 males; tg: 5 females and 5 males; MCD: wt: 6 females and 6 males; tg: 6 females and 6 males; 12 weeks: MCS: wt: 5 females and 4 males; tg: 6 females and 5 males; MCD: wt: 5 females and 5 males; tg: 5 females and 4 males). For the combined treatment with MCD and the genotoxic carcinogen diethylnitrosamine (DEN) (22) two different treatment schemes were used: one set of 3 week old wt (n = 28; females: n = 14, males: n = 14) and IMP2-2 tg (n = 24; females: n = 9, males: n = 15) mice received an MCD diet and 4 weeks after the onset of MCD feeding one injection of DEN (5 mg/kg BW i.p.). Mice were sacrificed at the age of 15 weeks or before when at least one of the stopping criterions (behavioral disorder, loss of weight >50%, abnormal water or feed uptake, matt coat, or an impairment of the locomotor system) was met. The other set of 2 week old wt (n = 10) and IMP2-2 tg (n = 13) mice received a single injection of diethylnitrosamine (DEN, 5 mg/kg BW i.p.) and were fed a MCD diet at the age of 8 weeks (wt: n = 10, females: n = 5, males: n = 5; tg: n = 13, females: n = 7, males: n = 6). Mice were sacrificed at the age of 29 weeks. Mice were housed in a 12/12 h light/dark cycle under constant conditions (temperature: 22°C ± 2°C; relative humidity: 55% ± 10%) with an enriched environment and food and water ad libitum. Mice were sacrifized by cervical dislocation or decapitation (embros, newborns) and whole blood samples were taken and liver was excised. Two thirds of the liver was flash-frozen in liquid nitrogen and stored at −80°C for further analysis. One third of the liver containing half of the left lateral lobe and half of the medial lobe in the same cassette was fixed in 4% PBS-buffered formalin for 24 h. After fixation samples were dehydrated and embedded in paraffin.
Whole blood samples were incubated for 1 h at room temperature to allow agglutination of red blood cells and subsequently centrifuged for 10 min at 13,500 × g at 4°C. The supernatant was transferred into a fresh tube, diluted 1:3 with 0.9% NaCl, and stored at −80°C until measurement. Serum alanine aminotransferase (ALT), aspartate aminotransferase (AST), glucose, triglycerides (TG), cholesterol, and high density lipoprotein (HDL) levels were determined by a PPE Modular analyzer using Roche® reagents at a constant temperature of 37°C (Roche Diagnostics, Mannheim, Germany). Measurements were performed at the “Zentrallabor des Universitätsklinikums des Saarlandes” (Homburg, Germany).
RNA Isolation, Reverse Transcription, and qPCR
In general, RNA isolation and qPCR were performed as previously described (23–25). Total RNA was extracted using Qiazol lysis reagent (Qiagen, Germany) according to the manufacturer's instructions and treated with DNase I (Ambion, Germany) to remove residual DNA. cDNA was synthesized using the high-capacity cDNA reverse transcription kit (Applied Biosystems) according to the manufacturer's protocol using random primers. qPCR was performed using 5x HOT FIREPol® EvaGreen® qPCR Mix Plus (Solis BioDyne, Estonia) according to the manufacturer's instructions and the CFX96 Touch™ Real-Time PCR Detection System (Bio-Rad, Germany). For absolute quantification PCR products were cloned into pGEMTeasy (Promega, Germany) and respective dilutions were run alongside the samples to generate a standard curve. All samples and standards were analyzed in triplicate using the following PCR conditions: 95°C for 15 min, followed by 39 cycles of 20 s at 94°C, 20 s at the primer-specific annealing temperature, and 20 s at 72°C. The relative expression was normalized to 18S or Ppia mRNA values depending on their stability. In sum, three different housekeeping genes (18s, Ppia/Cyclophilin, and Csnk2a2) were tested using the GeNorm tool and Normfinder (26, 27). Real-time quantitative PCR (qRT-PCR) was performed using the following primer pairs: Rn18S 5′-AGGTCTGTGATGCCCTTA GA-3′ and 5′-GAATGGGGTTCAACGGGT TA-3′; Ppia 5′-GGCCGATGACGAGCCC-3′ and 5′-TGTCTTTGGAACTTTGTCTGC-3′; Afp 5′-CCAGGAAGTCTGTTTCACAGAAG-3′ and 5′-CAAAAGGCTCACACCAAAGAG-3′; Krt19 5′-AGCGTGATCAGCGGTTTTG-3′ and 5′-CCTGGTTCTGGCGCTCTATG-3′; Sox9 5′-CCAGCAAGAACAAGCCACAC-3′ and 5′-CTTGCCCAGAGTCTTGCTGA-3′; Spp1 5′-CCGAGGTGATAGCTTGGCTTAT-3′ and 5′-GACTCCTTAGACTCACCGCTC-3′; Cdh1 5′-CTTTTCGGAAGACTCCCGATT-3′ and 5′-GCTTTAGATGCCGCTTCACTGT-3′; Imp2 5′-CTGATCCCAGGGCTAAACCTC-3′ and 5′-AAGGGGTGATAGGGAGGACTG-3′; Dlk1 5′-ACTTGCGTGGACCTGGAGAA-3′ and 5′-CTGTTGGTTGCGGCTACGAT-3′.
Western Blot Analysis
Western blots were performed as previously described (20).
Histology
Histochemistry and immunohistochemistry were performed as previously described (21, 25, 28). Paraffin-embedded liver sections were stained with hematoxylin-eosin (HE) and Sirius Red. Immunohistochemical (IHC) KRT19 and AFP detection was achieved using Dako EnVision+ System-HRP Labeled Polymer Anti Rabbit (#K4003, DAKO, USA) with anti-KRT19 antibody (1:1,000, #52625, abcam, UK), anti-DLK1 (1:1,000, #21682, abcam, UK) or anti-AFP antibody (1:1,000, #46799, abcam, UK). Epitopes were demasked with citrate buffer (10 mM, pH 6.0) in a waterbath at 95°C for 45 min. For Laminin detection epitopes were demasked by proteinase K (20 μg/ml in TE buffer) incubation for 15 min at 37°C in a waterbath. Anti-laminin antibody (1:200, #L9393, Sigma) was incubated for 1 h at room temperature followed by incubation with anti-rabbit Envision (Dako, Germany) for 20 min at room temperature and DAB staining. Hyaluronic acid was detected by staining with a biotin-labeled hyaluronic acid binding protein (1:100, #AMS.HKD-BC41, Amsbio) for 1 h at room temperature and detection by Streptavidin-HRP (#K3954, Dako, Germany) for 20 min at room temperature and DAB staining. Stainings were evaluated for steatosis, inflammation, DR, and fibrosis by two independent, blinded investigators with the scoring system shown in Table 1. Fibrosis scoring was assessed according to Kleiner et al. (29). Steatosis scoring was performed according to Simon et al. (21). Scoring system for ductular reaction was based on Chen et al. (30). We adapted the scoring of the latter study by including two different cirrhosis scores: 3 = cirrhosis with few and 4 = cirrhosis with many DR (see Table 1). In order to avoid high complexity we additionally combined score 1 and 2 from Chen et al. into score 1 in the current study.
Cell Culture
Huh7 cells were cultured in RPMI-1640 medium supplemented with fetal calf serum, penicillin/streptomycin and glutamine (Sigma-Aldrich, Taufkirchen, Germany) at 37°C and 5% CO2. Cells were treated with decitabine/5-aza-2′-deoxycytidine (#A3656, Sigma-Aldrich) over 3 days. The DNA-methyltransferase inhibitor was freshly added daily. Authentication of cell lines was confirmed by DSMZ.
Amplicon Sequencing Analysis
Ten percent of each bisulfite-treated sample were used as template in a 30 μL PCR mix: buffer BD (80 mM Tris-HCL, 20 mM (NH4)2SO4), 2.5 mM MgCl2, 0.2 mM of each dNTP, 200 nm each of the forward and reverse primers (sequences see Table 2) with universal Illumina adapters attached at the 5′ end, and 3U HotFirePol (Solis BioDyne). For each DNA lot, PCR reactions were performed in triplicates starting with 15 min denaturation at 95°C followed by 42 cycles of 95°C for 60 s, annealing for 120 s (see Table 2), 72°C for 120 s, and a final 5-min extension step at 72°C. About 5 μL PCR reaction were loaded on 1.2% agarose gels. Remaining 25 μL of the PCR reaction were purified with Agencourt Ampure XP beads (Beckman Coulter, Krefeld, Germany), diluted, pooled, and sequenced (Illumina v3 chemistry, 2 × 300 bp paired end) on Illumina MiSeq following the manufacturer's instructions aiming at 10,000 reads per amplicon and sample. Alignments and evaluation were done with the BiQ Analyzer HT program filtering all reads with more than 10% unrecognized CpG sites (31). Primers used for PCR for amplicon sequencing of murine Spp1 can be found in Table 2.
Microarray Analysis
For Affymetrix gene expression microarray analysis RNA was extracted from snap frozen liver tissue of 5 week-old IMP2-2 tg mice (n = 10) and corresponding controls (n = 10), as described in Godoy et al. (32). The Gene Chip IVT Labeling kit (Affymetrix, High Wycombe, UK) was used to generate biotinylated cRNA. Purification and fragmentation was performed according to Affymetrix's protocol. Hybridization to Mouse Genome 430 2.0 Affymetrix GeneChips (Santa Clara, CA, USA) was conducted for 16 h at 45°C according to the manufacturer's instructions. Microarrays were scanned with an Affymetrix scanner controlled by Affymetrix Microarray Suite software. Robust Multi-array Average (RMA) algorithm (33) was used for normalization of the gene expression array data and the R package limma (34) was used for calculation of differential gene expression. Adjustment for multiple testing was conducted with the method of Benjamini and Hochberg (FDR: false discovery rate) (35). Data are available on Gene Omnibus (GEO-ID: GSE130999).
Public Gene Omnibus (GEO) and TCGA Datasets
GEO dataset GSE14323 and the liver hepatocellular carcinoma dataset from TCGA were analyzed as described previously (21, 25, 28, 36). The GEO dataset GSE14323 was analyzed for differential gene expression in human tissue samples of normal liver (n = 19), pre-malignant cirrhosis (n = 41), cirrhosis of HCC-livers (n = 17), and HCC (n = 48) as described previously (21, 25, 28, 36). TCGA liver cancer samples (n = 159) were analyzed for promoter methylation of SPP1. Statistical significance was analyzed in the R-cran statistical environment by the Kolmogorov–Smirnov test followed by FDR-correction with the Benjamini-Hochberg method Q = 0.05, and up to m = 10 (35). Pearson correlation was applied to detect correlations between genes of interest.
High-Resolution Ultrasound
Mice were anesthesized with isoflurane and put on a heated stage in supine position. Chemical depilation (Nair hair removal cream) was done to prevent air trapped in the fur from interfering with ultrasound coupling into the animal. After removing the liver, ultrasound coupling gel (Aquasonic 100, Parker, Fairfield, NJ, USA) was circumferentially applied to the ex situ liver, to distinguish between the stage and the ex situ liver. Imaging was performed by means of a Vevo 770 high-resolution ultrasound system (VisualSonics, Toronto, ON, Canada) and a real-time microvisualization 704 Scanhead (VisualSonics) with a center frequency of 40 MHz and a focal depth of 6 mm. For 3D imaging the scanhead, driven by a linear motor, scanned first the in situ and then the ex situ liver. The technique yielded two-dimensional (2D) images at parallel and uniformly spaced 100 μm steps. The 2D image planes enabled rapid 3D image reconstruction, displaying a dynamic cube view format, as previously described (37).
Statistical Analysis
OriginPro 2018G software (OriginLab Corporation) was used for illustration and statistical analyses. Results are shown as means ± SEM or box plots with 25th/75th percentile boxes, geometric medians (line), means (square). Statistical significance was determined by Mann-Whitney U-test or t-test where applicable. Chi2 test was used for categorical data. Statistics of Kaplan-Meier survival analysis was performed by log rank test.
Results
Resource Identification Initiative
Analysis of publicly available gene expression data showed high IMP2 expression in fetal mouse and human livers compared to the respective adult tissue (Figure 1A). Embryonic markers, such as IGF2 and H19, were highly expressed in fetal livers (Figure 1A). The low expression of Imp2 in adult and high expression in fetal liver was confirmed by qPCR analysis of a time course of fetal, neonatal, young, and old mice (Figure 1B). Expression of IMP2 protein matched that of its transcript (Figures 1C,D). Further, the high levels of H19 and Igf2 in embryonic livers and low levels in adult livers were confirmed in the same time course (Figures 1E,F). Interestingly, H19 expression was highest in livers of newborn and young animals (Figure 1F).
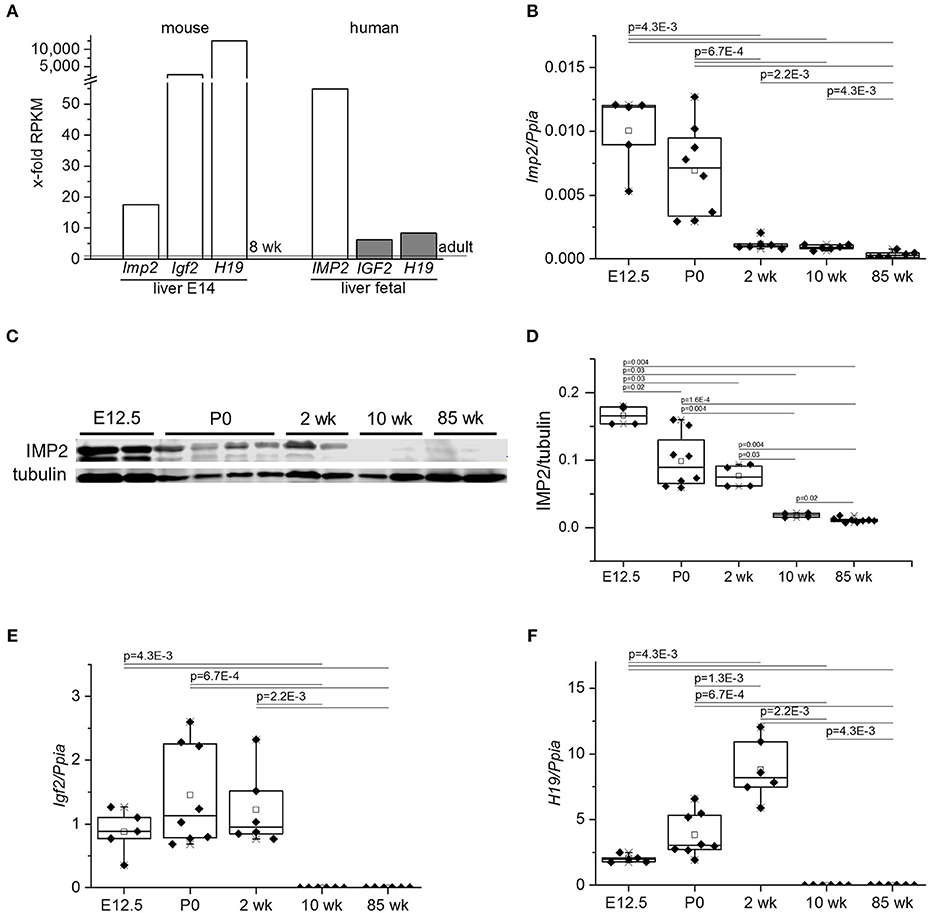
Figure 1. Expression of IMP2, IGF2, and H19 in embryonic and adult livers. (A) IMP2, IGF2, and H19 gene expression from publicly available RNA sequencing data sets of murine (PRJNA66167) and human (PRJNA280600) fetal liver compared to adults. Data are expressed as x-fold from 8 week-old murine or adult human tissues indicated by dashed lines, respectively. RPKM: Reads per kilobase transcript per million reads. (B,E,F) qPCR analysis of Imp2 (B), Igf2 (E), and H19 (F) in livers of fetal (E12.5; n = 5), newborns (P0, n = 8), 2, 10, and 85 week-old (each n = 6) mice. Expression levels were normalized to Ppia/Cyclophilin as housekeeping gene. (C,D) Western blot analysis of IMP2 protein expression in fetal, newborn, young, and adult mouse livers. Representative image of Western blot (C) and densitometric quantification of IMP2 levels normalized to tubulin (D) are shown (E12.5: n = 4; P0: n = 8; 2 and 10 week: n = 4; 85 week: n = 6). P-values were calculated by Mann-Whitney U-test.
These data showed the highest IMP2 expression in embryonic liver. We therefore hypothesized that IMP2 in adult liver may represent a marker of immaturity or dedifferentiation and aimed at investigating the impact of IMP2 expression on hepatic progenitor cell features. Since peritumoral DR correlates with fibrosis stage (38) we first analyzed the expression of IMP2 and common progenitor cell marker genes in gene expression data sets from human cirrhotic tissue (Figure 2A). Human cirrhotic and cirrhosis-associated HCC tissues showed an increase of IMP2 expression and of the progenitor cell markers SOX9, SPP1/osteopontin, CDH1/E-cadherin, AFP, PROM1/CD133, BEX1, EPCAM (Figure 2A). We next investigated the consequences of overexpressing IMP2-2 in the liver. Array analysis of IMP2-2 tg mice revealed an upregulation of the murine homologs LPC marker genes except for Sox9 (Figure 2B). Upregulation was confirmed by qPCR for some of the genes, i.e., Spp1, Afp, and Cdh1 (Figure 2C). Krt19, which was not on the array platform and Sox9, both markers of DR, were not upregulated (Figure 2C).
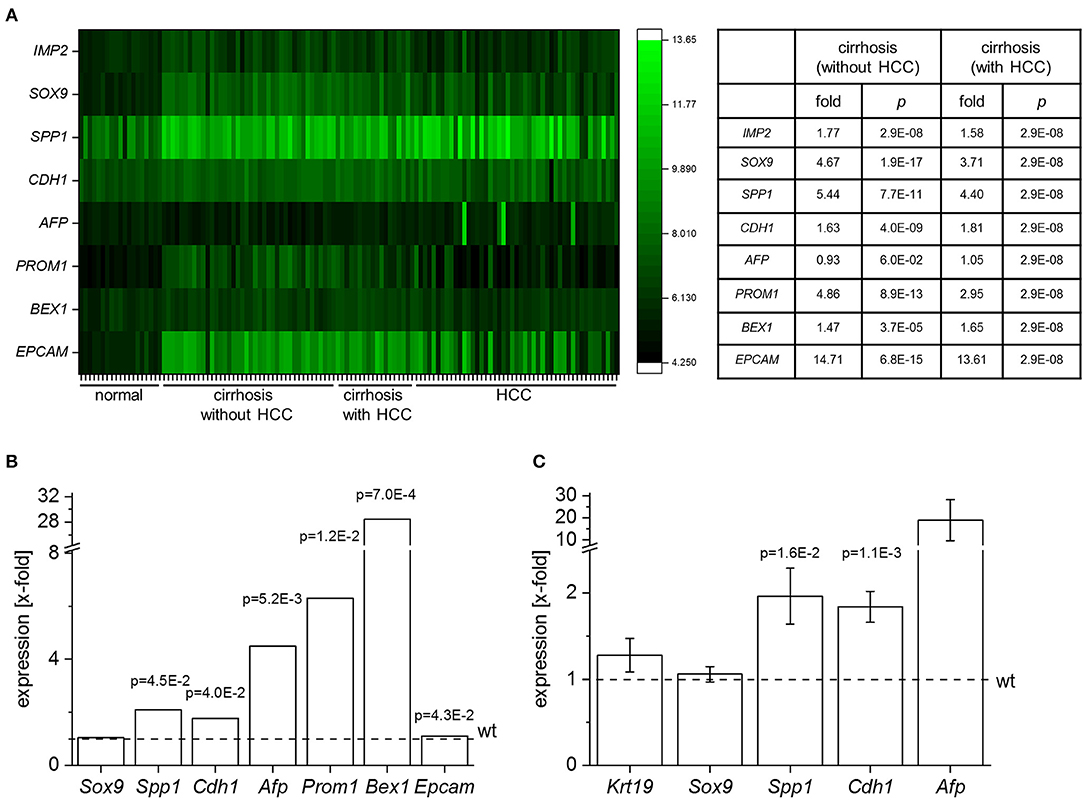
Figure 2. IMP2-2 induces stem cell marker expression. (A) Expression analysis of IMP2, SOX9, SPP1, CDH1, AFP, PROM1, BEX1, EPCAM in human samples of normal liver tissue (n = 19), cirrhotic tissue (without HCC; n = 41), cirrhotic tissue of HCC-livers (with HCC; n = 17), and HCC tissue (n = 48) (GSE14323). The table shows expression values as x-fold of healthy (n = 19) liver samples. P-values were generated by comparison to healthy tissue (Mann Whitney U-test or Welch's t-test, FDR-corrected by Benjamini-Hochberg method). (B) Gene expression of Sox9, Spp1, Cdh1, Afp, Prom1, Bex1, and Epcam in 5 week-old IMP2-2 transgenic animals (tg) determined by micro array analysis (n = 10 per genotype). Data are expressed as x-fold of wildtype (wt) animals (indicated as dashed line). FDR adjusted p-values are shown. For Afp median value of five different probes is shown (probe 1: x-fold = 17.62, adj. p = 0.002; probe 2: x-fold = 5.35, adj. p = 0.008; probe 3: x-fold = 4.52, adj. p = 0.005; probe 4: x-fold = 1.38, adj. p = 0.009; probe 5: x-fold = 0.95, adj. p = 0.35). (C) Krt19, Sox9, Spp1, Cdh1, and Afp mRNA expression of IMP2-2 tg mice (n = 13) normalized to the values of wt mice (n = 13). P-values were calculated in comparison with wt animals (Welch's t-test, FDR-corrected by Benjamini-Hochberg method).
IMP2-2 tg mice have been shown to develop steatosis without any sign of liver damage (20). Due to the increased levels of markers of de-differentiation, IMP2-2 tg mice were challenged with an MCD diet, which is known to induce DR within 8 weeks in the setting of steatohepatitis (18). In IMP2-2 tg mice DR was observed in 25% of the IMP2-2 tg animals fed the MCD diet already after 2 weeks (Figure 3A). On the control diet (MCS) none of the IMP2-2 tg mice of the 2 week time point and only 10% of the IMP2-2 tg mice of the 4 week timepoint developed DR (Figure 3A). More pronounced DR was observed in the IMP2-2 tg livers after 4 weeks of MCD feeding as shown by immunohistochemistry of KRT19 (Figures 3A,B). After 12 weeks of MCD diet more severe DR was observed in wt as well as in IMP2-2 tg mice with no statistically significant difference between both genotypes (data not shown), but fibrosis was still increased in the transgenics (Figure 3C). Interestingly, the IMP2-2 transgene was not only expressed in hepatocytes but also DR cells under MCD feeding (Figure 4A). After 4 weeks DR in IMP2-2 tg mice was characterized by increased expression of the cholangiocyte and progenitor cell markers Krt19 and Sox9 (Figure 4B). The progenitor cell marker genes Spp1 and Afp were induced to a higher extent in MCD-fed IMP2-2 tg mice compared to wt livers (Figure 4B). Cdh1 tended to be increased in IMP2-2 tg livers on MCD diet (Figure 4B). Igf2 levels were especially increased in female mice (data not shown), while the increase of the other progenitor cell markers in tg mice were sex independent. Since SPP1 was shown to activate hepatic stellate cells (HSCs), which can then produce extracellular matrix (ECM) components, we investigated α-smooth muscle actin (α-SMA) and Col1a1 levels as a measure of differentiation to myofibroblasts in MCD-fed mice and observed increased Col1a1 mRNA levels and α-SMA positive cells in IMP2-2 tg animals (Figures 4B,C). Myofibroblasts are known to produce ECM components. Thus, immunohistochemistry was performed to investigate the deposition of ECM components in the areas of DR of IMP2-2 tg livers. DR cells were surrounded by the ECM components laminin and hyaluronic acid (Figure 4D). In human cirrhotic tissue SPP1 was upregulated (Figure 2A) and strongly correlated with IMP2 expression (R2 = 0.42 p = 0.006 in cirrhosis). Further correlation analysis revealed a strong association of IMP2 and SPP1 with ECM components, i.e., laminin b (LAMB), laminin c (LAMC), hyaluronic acid receptor CD44, and fibrillin 1 (FBN1) (Figure 4E).
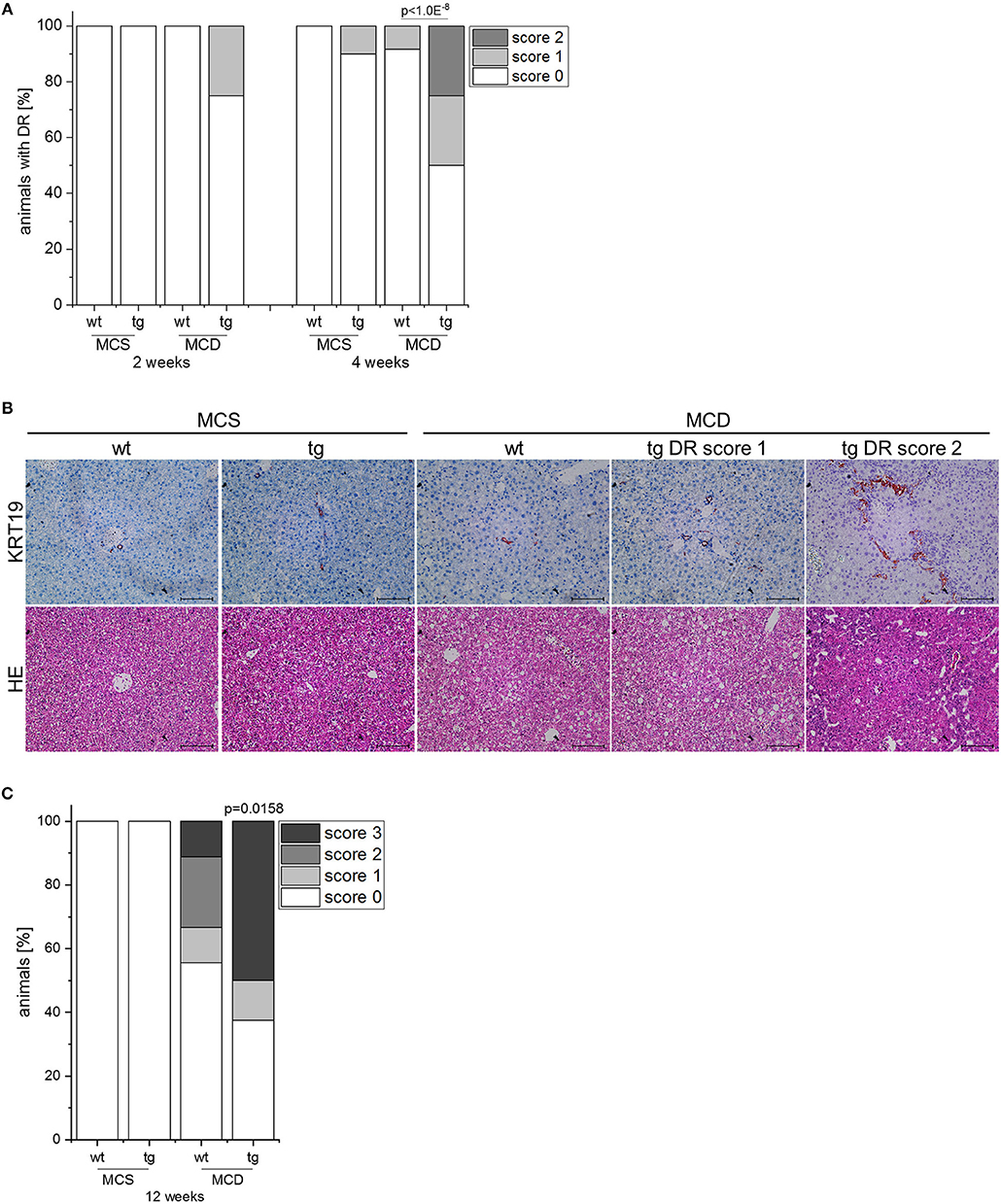
Figure 3. IMP2-2 expression accelerates DR in the MCD model. (A) Histological scoring of DR in IMP2-2 transgenic (tg) and wildtype (wt) mice, which were fed either a methionine-choline deficient (MCD) diet or a methionine-choline supplemented (MCS) control diet for 2 and 4 weeks (2 weeks: MCS: n = 10 per genotype, MCD: n = 12 per genotype; 4 weeks: n = 10 per genotype, MCD: n = 12 per genotype). The p-value was calculated by chi-square test. (B) Representative images of HE and KRT19 stainings from wt and tg mice on MCS or MCD diet for 4 weeks. For tg mice representative images of DR score 1 and DR score 2 are shown (scale bars: 100 μm). (C) Histological scoring of Sirius red stained sections for fibrosis of wt and tg mice, which were fed either a methionine-choline deficient (MCD) diet or a methionine-choline supplemented (MCS) control diet for 12 weeks (MCS: n = 9–11 per genotype, MCD: n = 9–10 per genotype).
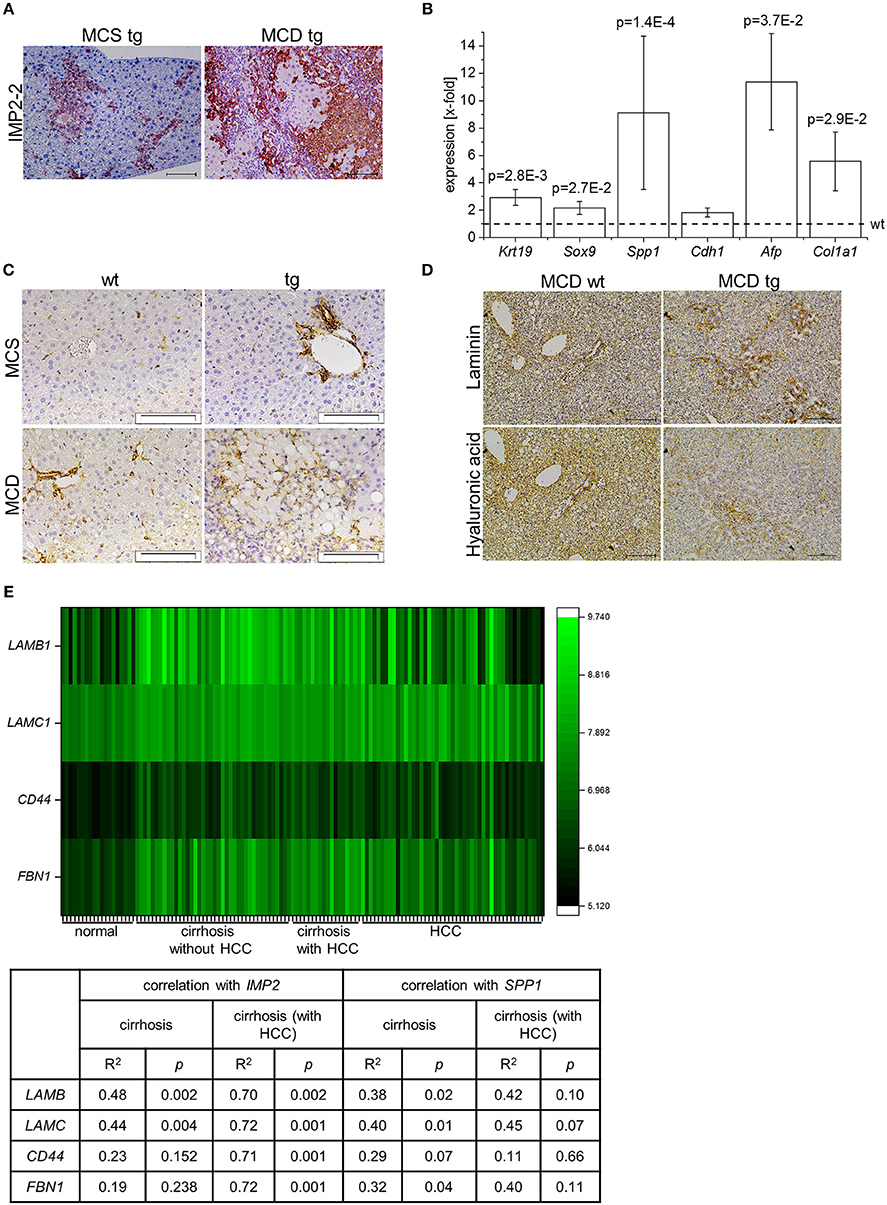
Figure 4. IMP2-2 expression correlates with the expression of extracellular matrix components. (A) Representative images of IMP2 staining in tg mice on MCS or MCD diet for 4 weeks (scale bars: 100 μm). (B) Krt19, Sox9, Spp1, Cdh1, Afp, Col1a1 mRNA expression levels of 4 weeks MCD-fed IMP2-2 tg mice (n = 12), shown as x-fold of wild-types (n = 12; indicated by dashed line). 18S was used as a housekeeping gene. Statistical significance compared to wt was determined by Mann-Whitney U-test or Welch's t-test. (C) Representative immunohistochemical staining for α-SMA in wt and tg mice fed a MCD or a MCS control diet for 4 weeks (MCS: n = 10 per genotype, MCD: n = 12 per genotype; scale bars: 50 μm). (D) Representative image of an area of DR stained for laminin (upper panel) and hyaluronic acid (lower panel) in wt and tg mice fed a MCD diet for 4 weeks (n = 12 per genotype; scale bars: 100 μm). (E) Heatmap shows expression of LAMB1, LAMC1, CD44 and FBN1 in human samples of normal liver tissue (n = 19), cirrhotic tissue (n = 41), cirrhotic tissue of HCC-livers (n = 17), and HCC tissue (n = 48) (GSE14323). The table shows a correlation analysis of IMP2 and SPP1 with LAMB1, LAMC1, CD44, and FBN1 in human samples of cirrhotic tissue (n = 41) and cirrhotic tissue of HCC-livers (n = 17) (GSE14323). The correlation coefficient was calculated by Pearson correlation.
SPP1 is known to promote fibrogenesis (39) thereby linking liver progenitor cell activation to fibrotic changes (40). Since Spp1 expression was reported to be regulated by altered DNA methylation in murine CCl4-induced fibrosis (41), we hypothesized that SPP1 hypomethylation might cause SPP1 induction in HCC. Therefore, we measured SPP1 expression after incubation of HCC cells with the demethylating agent decitabine as well as SPP1 DNA methylation in human samples. In fact, decitabine treatment in in vitro cultivated HCC cells strongly induced SPP1 expression (Figure 5A). In silico analysis of the human TCGA HCC dataset revealed SPP1 promoter hypomethylation in HCC patient tissues compared to normal liver tissues (Figure 5B). In order to test whether elevated Spp1 expression in untreated IMP2-2 tg mice is due to epigenetic changes we performed amplicon-based local deep sequencing covering the whole Spp1 gene with 60 single CpG positions in IMP2-2 tg and wt mice (42). Although Spp1 expression was significantly elevated in untreated IMP2-2 tg mice (Figure 5C), only minor methylation changes in single CpG sites of Spp1 were observed (Figure 5D). CpG#1 was significantly hypomethylated in IMP2-2 tg compared to wt mice (Figure 5D).
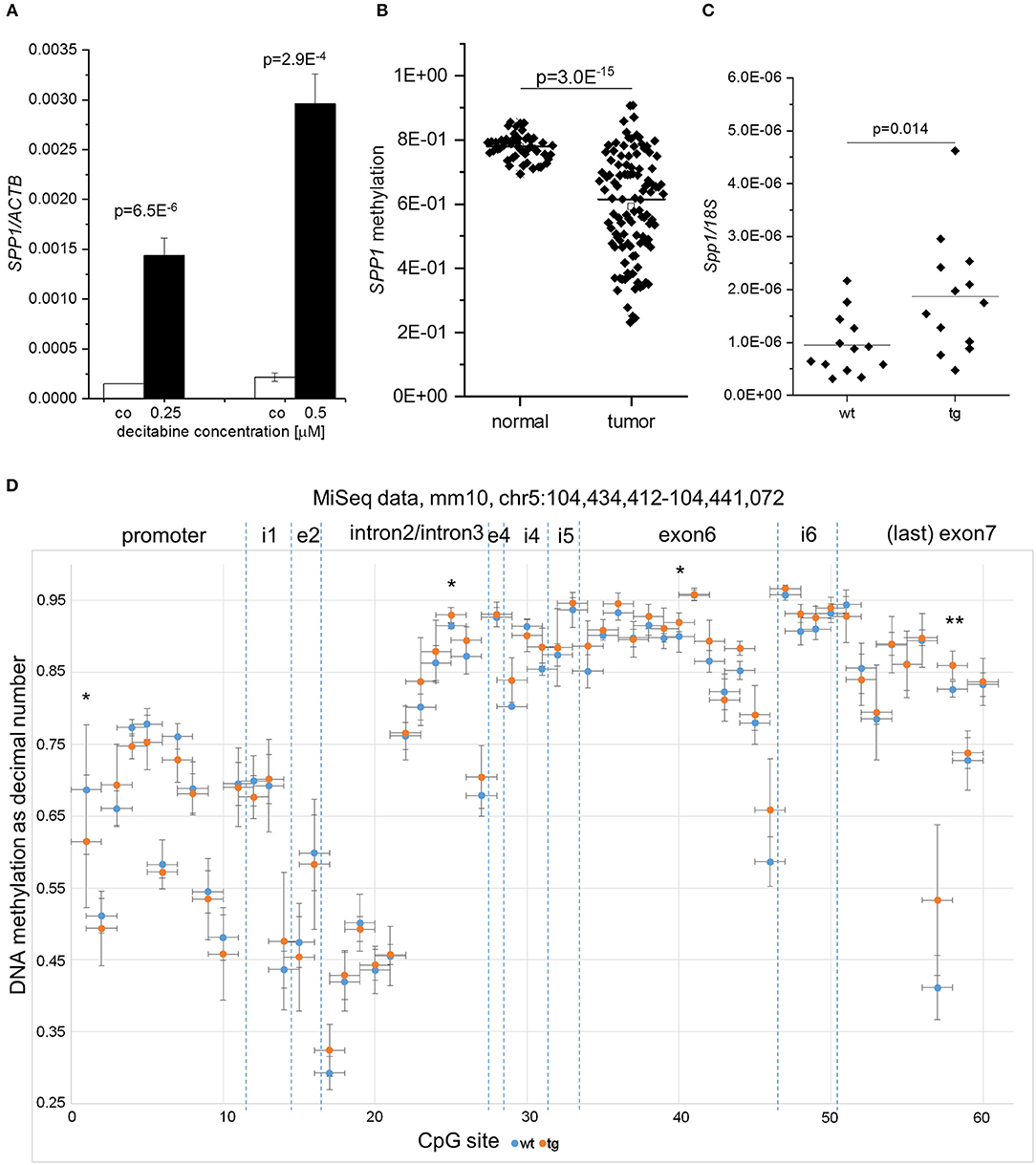
Figure 5. Differential SPP1 methylation in human HCC and IMP2-2 tg mice. (A) SPP1 expression in Huh7 cells treated with either 0.25 or 0.5 μM decitabine for 72 h quantified by qPCR. Decitabine-containing medium was changed every 24 h. (B) SPP1 promoter methylation in the TCGA liver cancer set in tumor vs. non-tumorous tissues. (C) Spp1 expression in IMP2-2 tg and wt mice on normal chow quantified by qPCR. 18S was used as housekeeping gene. (D) Methylation of CpG sites within the Spp1 gene in IMP2-2 tg (orange) vs. wt (blue) mice analyzed by amplicon sequencing. CpG sites are plotted along the x-axis according to their location in the gene, i.e., in the promoter region, introns [intron1 (i1), intron2/intron3, intron4 (i4), intron5 (i5), and intron6 (i6)], and exons (exon2 (e2), exon4 (e4), exon6, exon7). *p < 0.05; **p < 0.01.
Since DR associates with fibrosis, it is associated with poor prognosis in liver disease (43). Therefore, we analyzed whether additional administration of the genotoxic carcinogen DEN caused more severe fibrosis in IMP2-2 tg livers. Two different treatment schemes were used with DEN injection before or after the onset of MCD feeding (Figures 6A, 7A).
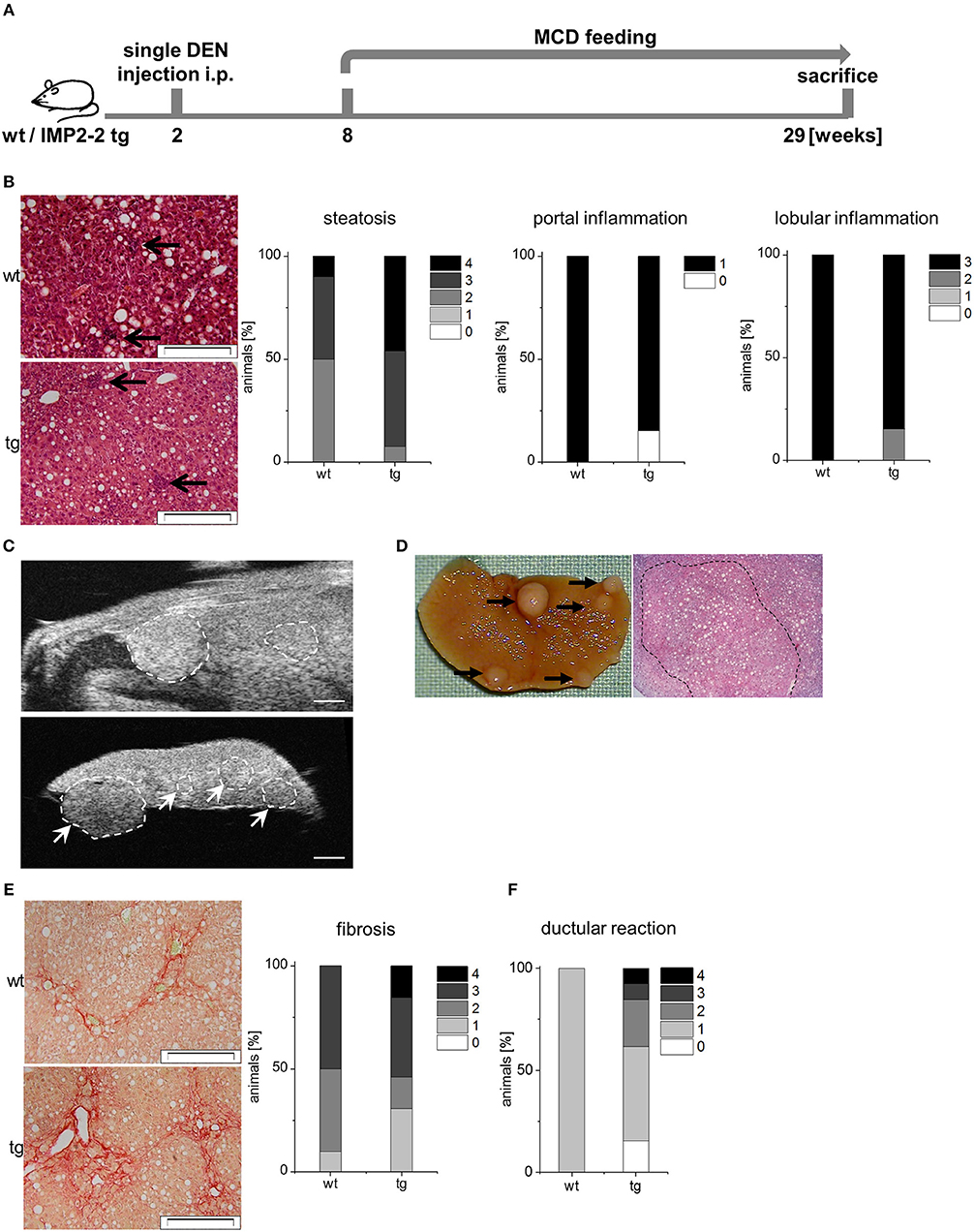
Figure 6. IMP2-2 triggers cirrhotic changes in the DEN-MCD model. (A) Treatment scheme of the DEN/MCD model. Administration of diethylnitrosamine (DEN) 6 weeks before the onset of MCD feeding (DEN-MCD). (B) Representative images of HE (left) and histological scorings for steatosis, lobular and portal inflammation of wt and tg mice treated with DEN-MCD. Arrows indicate inflammatory foci (scale bars: 50 μm). (C,D) Representative images of high-resolution ultrasound (C) and macroscopic view (D) of a liver of DEN-MCD mice. (C) High-resolution ultrasound imaging of an in situ (upper image) and ex situ (lower image) liver. Scale bars 0.9 mm (upper image), 1.2 mm (lower image). (E) Representative images of Sirius Red staining (left) and histological scoring for fibrosis (right) of wt and tg mice treated with DEN-MCD (wt: n = 10, tg: n = 13) (wt: score 2; tg: score 4; scale bars: 50 μm). (F) Histological scoring of KRT19 staining for DR (right) of wt and tg mice treated with DEN-MCD (wt: n = 10, tg: n = 13).
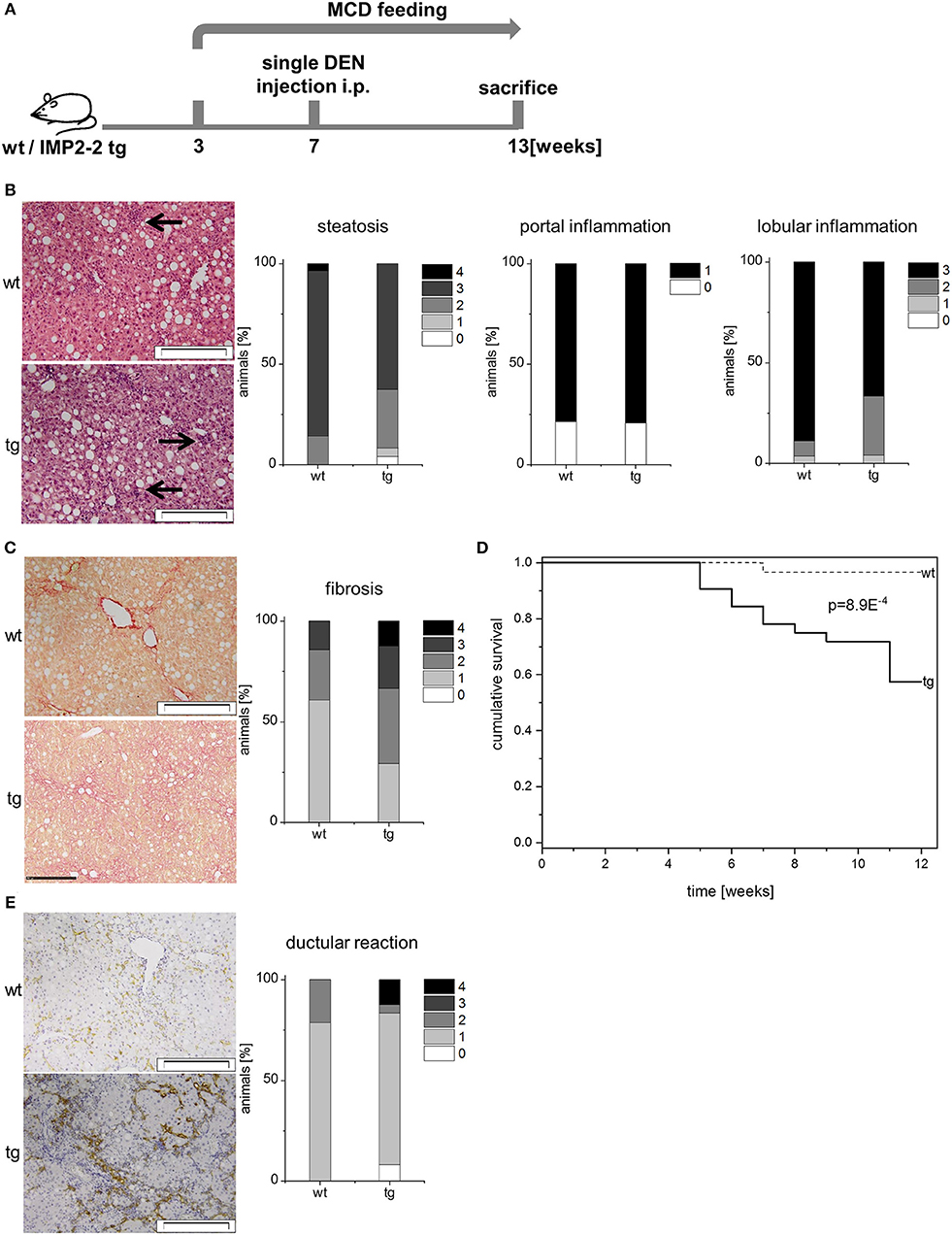
Figure 7. Transgenic expression of IMP2-2 leads to shorter survival in the MCD-DEN model. (A) Treatment scheme of the MCD/DEN model. Administration of DEN 5 weeks after the onset of MCD feeding (MCD-DEN). (B) Representative images of HE (left) and histological scorings for steatosis, lobular and portal inflammation of wt and tg mice treated with MCD-DEN (wt: n = 28, tg: n = 24). Arrows indicate inflammatory foci (scale bars: 50 μm). (C) Representative images of Sirius Red staining (left) and histological scoring for fibrosis (right) of wt and tg mice treated with MCD-DEN (wt: n = 28, tg: n = 24) (wt: score 1; tg: score 3; scale bars: 50 μm). (D) Kaplan-Meier survival plot referring to wt and tg animals treated with MCD-DEN (wt: n = 28, tg: n = 24). (E) Representative images of KRT19 staining (left) and histological scoring for DR (right) of wt and tg mice treated with MCD-DEN (wt: n = 28, tg: n = 24) (scale bars: 200 μm).
In the DEN-MCD treated animals (Figure 6A) high ALT and AST levels were found (Table 3). No difference between the genotypes regarding AST, ALT, triglycerides, cholesterol, HDL, and glucose were observed (Table 3). Steatosis ranged from score 2–4 in both genotypes and also inflammation was rather advanced in both genotypes (Figure 6B). Ultrasound analysis followed by macroscopic analysis exhibited the presence of cirrhotic nodules in IMP2-2 tg livers (nodule size >0.5 mm: wt: n = 2 with and n = 8 without nodules, tg: n = 10 with and n = 1 without nodules, p = 0.0019 tg compared to wt; Figures 6C,D). Sirius red staining confirmed fibrotic changes leading to cirrhosis only in the IMP2-2 tg livers (Figure 6E) with a varying extent of DR in the transgenics, while wt livers only showed weak DR (Figure 6F).
The MCD-DEN model (Figure 7A) revealed no difference in serum parameters (AST, ALT, triglycerides, cholesterin, HDL, and glucose), steatosis, and inflammation between the genotypes (Figure 7A; Table 3). In line with the other treatment scheme no difference between the genotypes was observed regarding steatosis and inflammation (Figure 7B), but cirrhosis was only present in the IMP2-2 tg livers (Figure 7C) with only few macroscopically visible nodules with no significant difference between wt and tg regarding occurrence of nodules. In the MCD-DEN set cirrhosis was linked to a significantly shorter survival time of the transgenic animals compared to wt controls (p = 0.0056, Figure 7D). The MCD-DEN treatment group contained livers showing cirrhosis accompanied by strong DR (Figure 7E). Investigation of two progenitor cell markers, DLK1 and AFP, revealed a significant upregulation of Afp mRNA and protein levels in the IMP2-2 tg animals in the MCD-DEN model (Supplementary Figures 1, 2).
Discussion
IMPs were originally identified and characterized as fetal proteins (44). Although IMP2 was first described as an oncofetal protein (3), IMP2 mRNA was suggested to be ubiquitously expressed in adult tissue (1). However, our data clearly show that IMP2 RNA and protein is expressed in fetal but not in adult human and mouse liver. IMP2 has been described to be re-expressed in liver cancer (45) and to preserve glioblastoma stem cells (4) underlining its oncofetal character. Moreover, IMP2 is more abundant than IMP1 and IMP3 in most cancer types and promotes an undifferentiated character of HCC (28).
Due to this oncofetal expression in liver we hypothesized that IMP2-2 might affect liver pathogenesis related to a de-differentiated cell phenotype. We show that IMP2-2 tg livers were more susceptible to DR provoked by an MCD diet. In untreated livers expression of the IMP2-2 transgene did not result in DR but caused elevated expression of progenitor cell markers.
In untreated livers of IMP2-2 tg mice a complex picture was obtained, characterized by the upregulated biliary/progenitor cell markers (Spp1), fetal hepatocytic marker (Afp), and classical stem/progenitor cell markers (Cdh1, Prom1, Bex1, and Epcam). Challenging these mice with the MCD diet resulted in DR and additional upregulation of biliary/progenitor cell markers (Krt19 and Sox9). In general, DR, which mostly constitutes of cells of immature biliary or hepatobiliary phenotype, are diverse in their cellular components (46). Although SPP1 and SOX9 usually represent cholangiocyte-like cell markers (47, 48), they have been shown to be also expressed in de-differentiated mature hepatocytes upon on injury by 3,5-diethoxycarbonyl-1,4-dihydrocollidine (DDC) (49). Furthermore, a de-differentiated SPP1/SOX9-positive hepatocyte population has been described in DDC-injured livers, which can be found near Epcam-positive expanding DR cells (50). Upregulation of Afp in the IMP2-2 livers (51) further suggests a de-differentiation process (52). Moreover, AFP expression was associated with EPCAM and PROM1 expression in BEX1-acclerated liver injury in the choline-deficient, ethionine-supplemented (CDE) diet model (53).
Interestingly, IMP2-2 tg mice showed significantly elevated levels of Cdh1, which is an epithelial marker overexpressed in LPCs. CDH1 expression can enhance maintenance of embryonic stem cell pluripotency [reviewed in (54)] and progressively increases with elevated numbers of progenitor cells (55). CDH1 expressing cells probably overlap with cells positive for DLK1 (56), which is also induced in IMP2-2 tg livers (28). In the fetal liver, DLK1 is specific for AFP-positive hepatoblasts (57). As the biliary markers Krt19 and Sox9 were not altered in IMP2-2 tg mice on normal chow, IMP2-2 tg mice seem to have an increased amount of de-differentiated hepatocyte-like cells (58). Still, as DLK1-positive progenitor cells are bipotential progenitor cells (59), they might also be the source of the accelerated occurrence of KRT19-positive DR cells observed on MCD feeding in IMP2-2 tg animals with significantly increased levels of both biliary markers Sox9 and Krt19. After partial hepatectomy proliferation of a specific subpopulation of cells positive for KRT19, AFP, and DLK1 has been described (58). Taken together, the MCD diet seems to provoke DR in IMP2-2 tg mice, which is characterized by a mixed cell population of a de-differentiated or undifferentiated phenotype.
Expression of SPP1 is associated with severe NASH in humans (60). SPP1 expression has been shown to be regulated by promoter methylation in liver fibrosis (41). Methylation changes of the SPP1 gene differed between human HCC and our IMP2-2 mouse model. Only single CpG sites were altered regarding methylation. CpG#1, which was hypomethylated in the IMP2-2 tg mice, is located within the binding site of runt-related transcription factor 2 (Runx2) in the Spp1 promoter. Interestingly, reduced methylation of CpG#1 of the human SPP1 promoter was reported to influence transcription factor binding in SH-SY5Y bone marrow cells, thereby leading to increased SPP1 expression (61) suggesting a similar mechanism for IMP2-2 induced Spp1 expression. SPP1 is able to activate HSCs leading to ECM production (62, 63) thereby promoting fibrosis. Immunohistochemical stainings of IMP2-2 tg livers of MCD-fed mice revealed a morphologically mixed type of DR cells in small nests and in bile duct-like formations. More precisely, ECM deposition and activation of matrix-producing cells occurred in front of proliferating DR cells as it has been described for the CDE model (55). Also progenitor cells positive for DLK1 were reported to be surrounded by a laminin layer in the rat liver (58). The laminin and hyaluronic acid staining pattern in IMP2-2 tg livers supports the hypothesis that the DR cell compartments are surrounded and supported by an ECM layer (55).
The shortened survival in IMP2-2 tg mice in MCD-DEN regime shows that IMP2-2 increases liver damage in chronic liver disease. The IMP2-2 transgenic mice were more susceptible toward DR and fibrosis caused by the MCD diet alone, since DR was already observed after 2 weeks of feeding. The difference between the genotypes was even more pronounced after 4 weeks, but became similar between IMP2-2 transgenic and wt mice after 12 weeks. This is explained by the fact that 12 weeks of MCD diet are generally accepted to be sufficient for induction of DR and fibrosis in wild-type mice (18). Therefore, the increased susceptibility of the IMP2-2 tg mice is probably masked by the advanced diet-induced liver disease in the latest analyzed time point of 12 weeks. With an additional challenge, such as DEN, IMP2-2 tg livers were again more susceptible. In fact, cirrhosis only occurred in IMP2-2 tg livers, which is probably due to the triggered progression of fibrosis by IMP2-2 (21).
Taken together, hepatic IMP2-2 expression leads to a de-differentiated cell population and enhanced DR, which promotes the development of cirrhosis in the MCD mouse model. Concordantly, also human cirrhotic samples expressed high IMP2 levels suggesting a similar role for IMP2 in human cirrhosis.
Ethics Statement
This study was carried out in accordance with the recommendations of the German guideline TierSchG and the European guideline 2010/63/EU. The protocol was approved by the animal welfare committee of Saarland University (permission number: 34/2010, 11/2013).
Author Contributions
BC, AA, AB, ST, NN, YS, CK, CC, NH, and SK conceived and carried out experiments and analyzed the data. AS, JGH, VH, ML, JW, JH, IL, and AK analyzed the data. SK concepted and supervised the study. All authors were involved in writing the paper and had final approval of the submitted and published versions.
Funding
We acknowledge support by the Deutsche Forschungsgemeinschaft (DFG, German Research Foundation) and Saarland University within the funding programme Open Access Publishing. This work was funded, in part, by an EASL Dame Sheila Sherlock Fellowship (to SK), a Bank Austria Visiting Scientists Programme Fellowship (to SK), and a Boehringer Ingelheim Travel grant (to SK). The results upon which this publication is based were partly funded by the Federal Ministry of Education and Research under the Project Number (O1KU1216F) (to AK). The responsibility for the content of this publication lies with the authors.
Conflict of Interest Statement
The authors declare that the research was conducted in the absence of any commercial or financial relationships that could be construed as a potential conflict of interest.
Acknowledgments
We thank Katharina Engel, Kevan Hosseini, and Tabea Trampert for excellent technical assistance in the analyses of serum parameters, histological stainings, cell culture, and local deep sequencing.
Supplementary Material
The Supplementary Material for this article can be found online at: https://www.frontiersin.org/articles/10.3389/fmed.2019.00179/full#supplementary-material
References
1. Bell JL, Wächter K, Mühleck B, Pazaitis N, Köhn M, Lederer M, et al. Insulin-like growth factor 2 mRNA-binding proteins (IGF2BPs): post-transcriptional drivers of cancer progression? Cell Mol Life Sci. (2013) 70:2657–75. doi: 10.1007/s00018-012-1186-z
2. Dai N, Rapley J, Ange M, Yanik FM, Blower MD, Avruch J. mTOR phosphorylates IMP2 to promote IGF2 mRNA translation by internal ribosomal entry. Genes Dev. (2011) 25:1159–72. doi: 10.1101/gad.2042311
3. Zhang JY, Chan EKL, Peng XX, Tan EM. A novel cytoplasmic protein with RNA-binding motifs is an autoantigen in human hepatocellular carcinoma. J Exp Med. (1999) 189:1101–10. doi: 10.1084/jem.189.7.1101
4. Janiszewska M, Suvà ML, Riggi N, Houtkooper RH, Auwerx J, Clément-Schatlo V, et al. Imp2 controls oxidative phosphorylation and is crucial for preservin glioblastoma cancer stem cells. Genes Dev. (2012) 26:1926–44. doi: 10.1101/gad.188292.112
5. Roskams T. Liver stem cells and their implication in hepatocellular and cholangiocarcinoma. Oncogene. (2006) 25:3818–22. doi: 10.1038/sj.onc.1209558
6. Lee JS, Heo J, Libbrecht L, Chu IS, Kaposi-Novak P, Calvisi DF, et al. A novel prognostic subtype of human hepatocellular carcinoma derived from hepatic progenitor cells. Nat Med. (2006) 12:410–6. doi: 10.1038/nm1377
7. Dumble ML, Croager EJ, Yeoh GCT, Quail EA. Generation and characterization of p53 null transformed hepatic progenitor cells: oval cells give rise to hepatocellular carcinoma. Carcinogenesis. (2002) 23:435–45. doi: 10.1093/carcin/23.3.435
8. Holczbauer Á, Factor VM, Andersen JB, Marquardt JU, Kleiner DE, Raggi C, et al. Modeling pathogenesis of primary liver cancer in lineage-specific mouse cell types. Gastroenterology. (2013) 145:221–31. doi: 10.1053/j.gastro.2013.03.013
9. Nomoto K, Tsuneyama K, Cheng C, Takahashi H, Hori R, Murai Y, et al. Intrahepatic cholangiocarcinoma arising in cirrhotic liver frequently expressed p63-positive basal/stem-cell phenotype. Pathol Res Pract. (2006) 202:71–6. doi: 10.1016/j.prp.2005.10.011
10. Kim H, Park C, Han KH, Choi J, Kim YB, Kim JK, et al. Primary liver carcinoma of intermediate (hepatocyte-cholangiocyte) phenotype. J Hepatol. (2004) 40:298–304. doi: 10.1016/j.jhep.2003.10.023
11. Yamashita T, Wang XW. Cancer stem cells in the development of liver cancer. J Clin Invest. (2013) 123:1911–8. doi: 10.1172/JCI66024
12. Jörs S, Jeliazkova P, Ringelhan M, Thalhammer J, Dürl S, Ferrer J, et al. Lineage fate of ductular reactions in liver injury and carcinogenesis. J Clin Invest. (2015) 125:2445–57. doi: 10.1172/JCI78585
13. Mu X, Español-Suñer R, Mederacke I, Affò S, Manco R, Sempoux C, et al. Hepatocellular carcinoma originates from hepatocytes and not from the progenitor/biliary compartment. J Clin Invest. (2015) 125:3891–903. doi: 10.1172/JCI77995
14. Roskams T, Desmet V. Ductular reaction and its diagnostic significance. Semin Diagn Pathol. (1998) 15:259–69.
15. Vartak N, Damle-Vartak A, Richter B, Dirsch O, Dahmen U, Hammad S, et al. Cholestasis-induced adaptive remodeling of interlobular bile ducts. Hepatology. (2016) 63:951–64. doi: 10.1002/hep.28373
16. Jansen PL, Ghallab A, Vartak N, Reif R, Schaap FG, Hampe J, et al. The ascending pathophysiology of cholestatic liver disease. Hepatology. (2017) 65:722–38. doi: 10.1002/hep.28965
17. Lowes KN, Brennan BA, Yeoh GC, Olynyk JK. Oval cell numbers in human chronic liver diseases are directly related to disease severity. Am J Pathol. (1999) 154:537–41. doi: 10.1016/S0002-9440(10)65299-6
18. Machado MV, Michelotti GA, Xie G, Almeida Pereira T, Boursier J, Bohnic B, et al. Mouse models of diet-induced nonalcoholic steatohepatitis reproduce the heterogeneity of the human disease. PLoS ONE. (2015) 10:e0127991. doi: 10.1371/journal.pone.0127991
19. Yue F, Cheng Y, Breschi A, Vierstra J, Wu W, Ryba T, et al. A comparative encyclopedia of DNA elements in the mouse genome. Nature. (2014) 515:355–64. doi: 10.1038/nature13992
20. Tybl E, Shi FD, Kessler SM, Tierling S, Walter J, Bohle RM, et al. Overexpression of the IGF2−mRNA binding protein p62 in transgenic mice induces a steatotic phenotype. J Hepatol. (2011) 54:994–1001. doi: 10.1016/j.jhep.2010.08.034
21. Simon Y, Kessler SM, Bohle RM, Haybaeck J, Kiemer AK. The insulin-like growth factor 2 (IGF2) mRNA-binding protein p62/IGF2BP2-2 as a promoter of NAFLD and HCC? Gut. (2014) 63:861–3. doi: 10.1136/gutjnl-2013-305736
22. Brendler SY, Tompa A, Hutter KF, Preussmann R, Pool-Zobel BL. In vivo and in vitro genotoxicity of several N-nitrosamines in extrahepatic tissues of the rat+. Carcinogenesis. (1992) 13:2435–41. doi: 10.1093/carcin/13.12.2435
23. Kessler SM, Laggai S, van Wonterghem E, Gemperlein K, Müller R, Haybaeck J, et al. Transient hepatic overexpression of insulin-like growth factor 2 induces free cholesterol and lipid droplet formation. Front Physiol. (2016) 7:147. doi: 10.3389/fphys.2016.00328
24. Laggai S, Kessler SM, Boettcher S, Lebrun V, Gemperlein K, Lederer E, et al. The IGF2 mRNA binding protein p62/IGF2BP2-2 induces fatty acid elongation as a critical feature of steatosis. J Lipid Res. (2014) 55:1087–97. doi: 10.1194/jlr.M045500
25. Simon Y, Kessler SM, Gemperlein K, Bohle RM, Müller R, Haybaeck J, et al. Elevated free cholesterol as a hallmark of non-alcoholic steatohepatitis in p62/insulin-like growth factor 2 mRNA binding protein 2-2 transgenic animals. World J Gastroenterol. (2014) 20:17839–50. doi: 10.3748/wjg.v20.i47.17839
26. Andersen CL, Jensen JL, Ørntoft TF. Normalization of real-time quantitative reverse transcription-PCR data: a model-based variance estimation approach to identify genes suited for normalization, applied to bladder and colon cancer data sets. Cancer Res. (2004) 64:5245–50. doi: 10.1158/0008-5472.CAN-04-0496
27. Vandesompele J, De Preter K, Pattyn F, Poppe B, Van Roy N, De Paepe A, et al. Accurate normalization of real-time quantitative RT-PCR data by geometric averaging of multiple internal control genes. Genome Biol. (2002) 3:research0034.1. doi: 10.1186/gb-2002-3-7-research0034
28. Kessler SM, Laggai S, Barghash A, Schultheiss CS, Lederer E, Artl M, et al. IMP2/p62 induces genomic instability and an aggressive hepatocellular carcinoma phenotype. Cell Death Dis. (2015) 6:e1894. doi: 10.1038/cddis.2015.241
29. Kleiner DE, Brunt EM, Van Natta M, Behling C, Contos MJ, Cummings OW, et al. Design and validation of a histological scoring system for nonalcoholic fatty liver disease. Hepatology. (2005) 41:1313–21. doi: 10.1002/hep.20701
30. Chen Y, Guldiken N, Spurny M, Mohammed HHA, Haybaeck J, Pollheimer MJ, et al. Loss of keratin 19 favours the development of cholestatic liver disease through decreased ductular reaction. J Pathol. (2015) 237:343–54. doi: 10.1002/path.4580
31. Lutsik P, Feuerbach L, Arand J, Lengauer T, Walter J, Bock C. BiQ analyzer HT: locus-specific analysis of DNA methylation by high-throughput bisulfite sequencing. Nucleic Acids Res. (2011) 39:W551–6. doi: 10.1093/nar/gkr312
32. Godoy P, Widera A, Schmidt-Heck W, Campos G, Meyer C, Cadenas C, et al. Gene network activity in cultivated primary hepatocytes is highly similar to diseased mammalian liver tissue. Arch Toxicol. (2016) 90:2513–29. doi: 10.1007/s00204-016-1761-4
33. Irizarry RA, Hobbs B, Collin F, Beazer-Barclay YD, Antonellis KJ, Scherf U, et al. Exploration, normalization, and summaries of high density oligonucleotide array probe level data. Biostatistics. (2003) 4:249–64. doi: 10.1093/biostatistics/4.2.249
34. Smyth GK, Michaud J, Scott HS. Use of within-array replicate spots for assessing differential expression in microarray experiments. Bioinformatics. (2005) 21:2067–75. doi: 10.1093/bioinformatics/bti270
35. Benjamini Y, Hochberg Y. Controlling the false discovery rate: a practical and powerful approach to multiple testing. J R Stat Soc Series B Stat Methodol. (1995) 57:289–300. doi: 10.1111/j.2517-6161.1995.tb02031.x
36. Kessler SM, Barghash A, Laggai S, Helms V, Kiemer AK. Hepatic hepcidin expression is decreased in cirrhosis and HCC. J Hepatol. (2015) 62:977–9. doi: 10.1016/j.jhep.2014.10.046
37. Frueh FS, Körbel C, Gassert L, Müller A, Gousopoulos E, Lindenblatt N, et al. High-resolution 3D volumetry versus conventional measuring techniques for the assessment of experimental lymphedema in the mouse hindlimb. Sci Rep. (2016) 6:34673. doi: 10.1038/srep34673
38. Ye F, Jing YY, Guo SW, Yu GF, Fan QM, Qu FF, et al. Proliferative ductular reactions correlate with hepatic progenitor cell and predict recurrence in HCC patients after curative resection. Cell Biosci. (2014) 4:50. doi: 10.1186/2045-3701-4-50
39. Leung TM, Wang X, Kitamura N, Fiel MI, Nieto N. Osteopontin delays resolution of liver fibrosis. Lab Invest. (2013) 93:1082–9. doi: 10.1038/labinvest.2013.104
40. Wang X, Lopategi A, Ge X, Lu Y, Kitamura N, Urtasun R, et al. Osteopontin induces ductular reaction contributing to liver fibrosis. Gut. (2014) 63:1805–18. doi: 10.1136/gutjnl-2013-306373
41. Komatsu Y, Waku T, Iwasaki N, Ono W, Yamaguchi C, Yanagisawa J. Global analysis of DNA methylation in early-stage liver fibrosis. BMC Med Genomics. (2012) 5:5. doi: 10.1186/1755-8794-5-5
42. Gries J, Schumacher D, Arand J, Lutsik P, Markelova MR, Fichtner I, et al. Bi-PROF: bisulfite profiling of target regions using 454 GS FLX Titanium technology. Epigenetics. (2013) 8:765–71. doi: 10.4161/epi.25242
43. Richardson MM, Jonsson JR, Powell EE, Brunt EM, Neuschwander–Tetri BA, Bhathal PS, et al. Progressive fibrosis in nonalcoholic steatohepatitis: association with altered regeneration and a ductular reaction. Gastroenterology. (2007) 133:80–90. doi: 10.1053/j.gastro.2007.05.012
44. Nielsen J, Christiansen J, Lykke-Andersen J, Johnsen AH, Wewer UM, Nielsen FC. A family of insulin-like growth factor II mRNA-binding proteins represses translation in late development. Mol Cell Biol. (1999) 19:1262–70. doi: 10.1128/MCB.19.2.1262
45. Kessler SM, Pokorny J, Zimmer V, Laggai S, Lammert F, Bohle RM, et al. IGF2 mRNA binding protein p62/IMP2-2 in hepatocellular carcinoma: antiapoptotic action is independent of IGF2/PI3K signaling. Am J Physiol Gastrointest Liver Physiol. (2013) 304:G328–36. doi: 10.1152/ajpgi.00005.2012
46. Gouw ASH, Clouston AD, Theise ND. Ductular reactions in human liver: diversity at the interface. Hepatology. (2011) 54:1853–63. doi: 10.1002/hep.24613
47. Pritchett J, Harvey E, Athwal V, Berry A, Rowe C, Oakley F, et al. Osteopontin is a novel downstream target of SOX9 with diagnostic implications for progression of liver fibrosis in humans. Hepatology. (2012) 56:1108–16. doi: 10.1002/hep.25758
48. Syn WK, Choi SS, Liaskou E, Karaca GF, Agboola KM, Oo YH, et al. Osteopontin is induced by hedgehog pathway activation and promotes fibrosis progression in nonalcoholic steatohepatitis. Hepatology. (2011) 53:106–15. doi: 10.1002/hep.23998
49. Tarlow BD, Pelz C, Naugler WE, Wakefield L, Wilson EM, Finegold MJ, et al. Bipotential adult liver progenitors are derived from chronically injured mature hepatocytes. Cell Stem Cell. (2014) 15:605–18. doi: 10.1016/j.stem.2014.09.008
50. Tanimizu N, Ichinohe N, Yamamoto M, Akiyama H, Nishikawa Y, Mitaka T. Progressive induction of hepatocyte progenitor cells in chronically injured liver. Sci Rep. (2017) 7:39990. doi: 10.1038/srep39990
51. Amirabad AD, Ramasamy P, Wierz M, Nordström K, Kessler SM, Schulz MH, et al. Transgenic expression of the RNA binding protein IMP2 stabilizes miRNA targets in murine microsteatosis. Biochim Biophys Acta Mol Basis Dis. (2018) 1864:3099–108. doi: 10.1016/j.bbadis.2018.05.024
52. Yanger K, Zong Y, Maggs LR, Shapira SN, Maddipati R, Aiello NM, et al. Robust cellular reprogramming occurs spontaneously during liver regeneration. Genes Dev. (2013) 27:719–24. doi: 10.1101/gad.207803.112
53. Gu Y, Wei W, Cheng Y, Wan B, Ding X, Wang H, et al. A pivotal role of BEX1 in liver progenitor cell expansion in mice. Stem Cell Res Ther. (2018) 9:164. doi: 10.1186/s13287-018-0905-2
54. Soncin F, Ward CM. The function of e-cadherin in stem cell pluripotency and self-renewal. Genes. (2011) 2:229–59. doi: 10.3390/genes2010229
55. Van Hul NKM, Abarca-Quinones J, Sempoux C, Horsmans Y, Leclercq IA. Relation between liver progenitor cell expansion and extracellular matrix deposition in a CDE-induced murine model of chronic liver injury. Hepatology. (2009) 49:1625–35. doi: 10.1002/hep.22820
56. Schievenbusch S, Sauer E, Curth HM, Schulte S, Demir M, Toex U, et al. Neighbor of punc e 11: Expression pattern of the new hepatic stem/progenitor cell marker during murine liver development. Stem Cells Dev. (2012) 21:2656–66. doi: 10.1089/scd.2011.0579
57. Nierhoff D, Ogawa A, Oertel M, Chen YQ, Shafritz DA. Purification and characterization of mouse fetal liver epithelial cells with high in vivo repopulation capacity. Hepatology. (2005) 42:130–9. doi: 10.1002/hep.20735
58. Tanimizu N, Tsujimura T, Takahide K, Kodama T, Nakamura K, Miyajima A. Expression of Dlk/Pref-1 defines a subpopulation in the oval cell compartment of rat liver. Gene Expr Patterns. (2004) 5:209–18. doi: 10.1016/j.modgep.2004.08.003
59. Seth A, Ye J, Yu N, Guez F, Bedford DC, Neale GA, et al. Prox1 ablation in hepatic progenitors causes defective hepatocyte specification and increases biliary cell commitment. Development. (2014) 141:538–47. doi: 10.1242/dev.099481
60. Moylan CA, Pang H, Dellinger A, Suzuki A, Garrett ME, Guy CD, et al. Hepatic gene expression profiles differentiate presymptomatic patients with mild versus severe nonalcoholic fatty liver disease. Hepatology. (2014) 59:471–82. doi: 10.1002/hep.26661
61. Shen CJ, Tsou YA, Chen HL, Huang HJ, Wu SC, Cheng WTK, et al. Osteoponin promoter controlled by DNA methylation: aberrant methylation in cloned porcine genome. BioMed Res Int. (2014) 2014:327538. doi: 10.1155/2014/327538
62. Arriazu E, Ge X, Leung TM, Magdaleno F, Lopategi A, Lu Y, et al. Signalling via the osteopontin and high mobility group box-1 axis drives the fibrogenic response to liver injury. Gut. (2016) 66:1123–37. doi: 10.1136/gutjnl-2015-310752
Keywords: liver cancer, stem cell, de-differentiation, oval cell, HCC, fibrosis
Citation: Czepukojc B, Abuhaliema A, Barghash A, Tierling S, Naß N, Simon Y, Körbel C, Cadenas C, van Hul N, Sachinidis A, Hengstler JG, Helms V, Laschke MW, Walter J, Haybaeck J, Leclercq I, Kiemer AK and Kessler SM (2019) IGF2 mRNA Binding Protein 2 Transgenic Mice Are More Prone to Develop a Ductular Reaction and to Progress Toward Cirrhosis. Front. Med. 6:179. doi: 10.3389/fmed.2019.00179
Received: 17 January 2019; Accepted: 23 July 2019;
Published: 04 September 2019.
Edited by:
Salvatore Piscuoglio, University Hospital of Basel, SwitzerlandReviewed by:
Laurent Georges Dollé, Biothèque Wallonie Bruxelles, BelgiumCaner Ercan, Gazi University, Turkey
Copyright © 2019 Czepukojc, Abuhaliema, Barghash, Tierling, Naß, Simon, Körbel, Cadenas, van Hul, Sachinidis, Hengstler, Helms, Laschke, Walter, Haybaeck, Leclercq, Kiemer and Kessler. This is an open-access article distributed under the terms of the Creative Commons Attribution License (CC BY). The use, distribution or reproduction in other forums is permitted, provided the original author(s) and the copyright owner(s) are credited and that the original publication in this journal is cited, in accordance with accepted academic practice. No use, distribution or reproduction is permitted which does not comply with these terms.
*Correspondence: Sonja M. Kessler, s.kessler@mx.uni-saarland.de