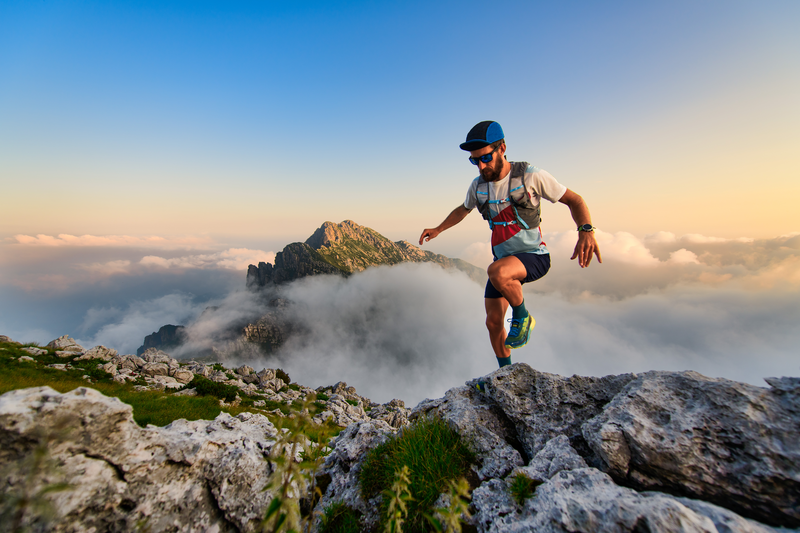
94% of researchers rate our articles as excellent or good
Learn more about the work of our research integrity team to safeguard the quality of each article we publish.
Find out more
MINI REVIEW article
Front. Med. , 27 June 2019
Sec. Pulmonary Medicine
Volume 6 - 2019 | https://doi.org/10.3389/fmed.2019.00145
Melanocortin agonists are ancient neuropeptides that have steroidogenesis and anti-inflammatory properties. They activate melanocortin receptors (MCR), a family of five seven-transmembrane G-protein coupled receptors. MC1R and MC3R are mainly involved in immunomodulatory effects. Adrenocorticotropin hormone (ACTH) and alpha-Melanocortin stimulating hormone (α-MSH) reduce pro-inflammatory cytokines in several pulmonary inflammatory disorders including asthma, sarcoidosis, and the acute respiratory distress syndrome. They have also been shown to reduce fibrogenesis in animal models with pulmonary fibrosis. By understanding the functions of MCR in macrophages, T-helper cell type 1, and T-helper cell type 17, we may uncover the mechanism of action of melanocortin agonists in sarcoidosis. Further translational and clinical research is needed to define the role of ACTH and α-MSH in pulmonary diseases.
Melanocortin systems are well-known for their regulation of skin pigmentation and steroidogenesis. Interest in melanocortin receptor agonists in pulmonary medicine stems from their role as potential modifiers of inflammatory disease. In this review, we provide insight into the structures and functionality of melanocortin receptors and their agonists in pulmonary inflammatory and fibrotic diseases.
Melanocortin signaling peptides (melanocortins), consisting of adrenocorticotropin hormone (ACTH), α-melanocyte stimulating hormone (α-MSH), beta-melanocyte-stimulating hormone (β-MSH), and gamma-melanocyte-stimulating hormone (γ-MSH), have been studied in numerous physiologic and diseased states. All melanocortins are derived from the pro-opiomelanocortin prohormone (POMC), which is post-translationally modified by pro-convertase 1 or 2 to generate each peptide as shown in Figure 1 (3). When POMC is produced, it is uniquely cleaved in each tissue allowing ACTH/MSH-derived peptides to act locally. The melanocortin peptides contain the core amino acid sequence His-Phe-Arg-Trp, which is integral to their ability to bind their receptors (4, 5).
Figure 1. Structure of POMC (Pre-Opiomelanocortin) gene and its post-translational processing and modification products in the anterior and intermediate pituitary gland. Lys, Lysine; Arg, Arginine; Cys, Cystine; α-, β- and λ-MSH, α-, β- and λ-Melanocyte Stimulating Hormone; ACTH, Adrenocorticotropic Hormone; β- and λ- LPH, β- and λ- Lipotrophin; N-POMC, N-Pro-Opiomelanocortin. The figure adapted from Bicknell (1) and Mulcahy and Nillni (2) works with modification.
ACTH is maybe best known for its actions on steroidogenesis in the adrenal cortex. The adrenal cortex is divided into three histologically distinct layers: the zona glomerulosa (ZG), zona fasciculata (ZF), and zona reticularis (ZR). The ZG is predominantly responsible for secretion of aldosterone which is primarily regulated by renin. The ZF and ZG secrete glucocorticoids and the androgen precursors, dehydroepiandrosterone (DHEA), respectively, both of which are controlled by ACTH secretion (6). While the bulk of research has been on the adrenal actions of ACTH, recent studies have begun to shed light on extra-adrenal functions. Those studies have focused on the influence of adipocyte differentiation and function as well as matrix synthesis in mesenchymal cells (7–9). It has been proposed that ACTH increases intracellular calcium in mesenchymal cells and initiate differentiations. (10) Other roles of ACTH that are beginning to be explored are thymic cell growth and differentiation, androgen production via direct action on Leydig cells, a reno-protective effect in chronic kidney disease, and influences on mood (6).
α-MSH is a 13 amino acid peptide most known for its cutaneous response to ultraviolet light leading to increased skin pigmentation (11). It has also been shown to possess anti-inflammatory and anti-microbial effects all via melanocortin receptor signaling (12–14). β-MSH and γ-MSH have been studied considerably less than α-MSH or ACTH. β-MSH does not exist in rodents due to lack of N-terminal cleavage site (15). Therefore, there is little information on its functionality. Recently, a mutation in β-MSH gene (Y5C-β-MSH) was associated with obesity in children and highlighted the essential role of β-MSH in the hypothalamic control of body weight in humans (16).
γ-MSH was named for homology to alpha and beta but without known melanotropic activity (17). γ-MSH has 2 cleavage products gamma-1 and gamma-3, both with a critical N-terminal lysine residue. The work that has been done on Lys-gamma3-MSH has shown that it functions both in vitro and in vivo to potentiate the steroidogenic effects of ACTH on the rat adrenal. Specifically, it was demonstrated that they act together to increase the activity of hormone sensitive lipase (18).
The melanocortin receptors are a family of five seven-transmembrane G-protein coupled receptors (GPCRs) and are the smallest GPCRs known. Their transmembrane domains are alpha-helical with the conserved motif of aspartic acid-arginine-tyrosine at the junction of the TM3 domain and contain cysteine at the C terminus (19). Human melanocortin receptor genes are located on single exons within autosomes. In humans, MC1R is found on chromosome 16, MC3R is on chromosome 20, while MC2R, MC4R, and MC5R are on chromosome 18 (20). Sequence homologies among the receptors range from 38 to 60%, with MC2R demonstrating the least homology with the other receptors (21). Each receptor is coupled to adenylate cyclase, triggering formation of cAMP and activating protein kinase C. This leads to influx of extracellular calcium resulting in IP3 activation. IP3 then activates the MAPK, and JAK-STAT pathways (as shown in Figures 2, 3) (23). The receptors are widespread throughout the body, exhibiting myriad ligand affinities, tissue and cell distribution, and downstream effects (24).
Figure 2. Downstream effects of Melanocortin-1 receptor stimulation in melanocytes: On stimulation of the receptor, Adenyl cyclase is activated and cAMP is generated activating multiple downstream mediators involved in melanogenesis and melanocyte proliferation. Adapted from Joshi et al. (22) work with modification.
Figure 3. Role of Melanocortin-1 receptor on macrophages in inhibiting inflammation. MC1R activates adenyl cyclase and generates cAMP, activating protein kinase-C. This leads to influx of extracellular calcium and activation IP3. IP3 then activates MAPK and JAK-STAT pathways which inhibit the degradation of IκB and activate CREB. CREB is involved in downstream anti-inflammatory effects. Adapted from Zhang et al. (23) work with modification.
MC1R was first cloned in 1992 and has been studied extensively for its role in regulation of human integumentary pigmentation. It contains 317 amino acids and was originally referred to as α-MSH receptor, the name of its major ligand (25). MC1R is formed from 7 trans-cytoplasmic membrane segments and an extramembrane domain contains 37 AA as shown in magnification circle in Figure 4 (27). Video 1 shows a 3D secondary molecular structure of MC1R. MC1R has equal affinity for α-MSH, ACTH, and β-MSH, but less to γ-MSH (24). MC1R on human melanocytes is stimulated by its ligands to enhance melanocyte proliferation and melanogenesis as shown in Figure 2. This receptor is highly polymorphic and its variants have been associated with increasing risk of melanoma and non-melanoma skin cancers (22). MC1R is expressed on endothelial cells, monocytes, macrophages, lymphocytes, neutrophils, mast cells, intestinal epithelia, as well as, in testicular, ovarian, placental, lung, and liver tissue (28). Mutations in the MC1R gene may modify the immune response in the setting of inflammation. Seaton and co-workers reviewed 1,246 subjects with blunt injury and found that those who carried a polymorphism at the rs885479 location of MC1R gene had a lower risk (OR = 0.45, P = 0.006) of complicated sepsis (29).
Figure 4. Model of the MC1R secondary structure with 7 core domains and a rich tail. Modeling was performed by using Swiss-Model (26).
The MC2R gene is located on chromosome 18 and contains 325 amino acids (30). MC2R is predominantly expressed in the adrenal cortex, where it mediates the effects of ACTH. It is believed to bind ACTH exclusively, which is crucial in regulating steroidogenesis (31). Mutations affecting the normal function of MC2R are implicated in familial glucocorticoid deficiency, including polymorphism on S74I, R146H/560delT, and 579-581delTGT (32).
MC3R, a 361 amino acids-long peptide coded on chromosome 20, is the least selective of the melanocortin receptors, binding the four melanocortins with equal affinity (33). MC3R expresses on macrophages and intestinal epithelial cells. It is also found in lymphocytes, brain, heart, and placental tissues, as well as skeletal muscles (28, 34).
Human MC4R was cloned by Gantz et al. (33), who traced it to chromosome 18 as a gene encoding a protein with 333 amino acids in length (35). MC4R is expressed throughout the central nervous system (36). It has similar affinity to ACTH, α-MSH, and β-MSH, and to a much lesser extent to γ-MSH. MC4R is involved with energy homeostasis, sexual behavior, nociception, inflammation, and neuroprotection (37, 38). Inhibition of MC4R in mice results in obesity secondary to a hyperphagic, hyperinsulinemic, and hyperglcyemic state (39).
MC5R was the last of the melanocortin gene family to be cloned by homology screening from human genomic DNA in 1993 and subsequently in the mouse genome the following year (40).
The MC5R has a similar ligand recognition affinity as MC1R and MC4R in which it binds most avidly to α-MSH and to a lesser extent ACTH. It does not bind to γ-MSH (41). MC5R expresses in numerous tissue types including the adrenal glands, fat cells, as well as kidney, thymus, skin, testicular, ovarian, uterine, esophageal, duodenal, liver, and lung tissue. It is also found in lymph nodes, bone marrow, skeletal muscle, and exocrine glands (42). The presence of MC5R on both B- and T- lymphocytes suggests it may play a role in regulation of the immune system and inflammatory response (42). MC5R has a role in exocrine gland function and thermoregulation. In the MC5R knockout mouse model, water repulsion and thermoregulation are impaired as a result of decreased production of sebaceous lipids (43). Recently, Trotta et al. proposed that stimulation of MC5R with α-MSH reduced risk of high glucose-induced cardiac hypertrophy (44).
MC1R and MC3R are the most studied members of the melanocortin system family with respect to inflammation. They may be potentially significant in the context of pulmonary diseases. Evidence of the anti-inflammatory properties of the melanocortin system are described in dermatologic, central nervous system, and pulmonary disorders.
Chen et al. investigated the immunoregulatory effects of MC1R. They found that MC1R inhibited the inflammatory response in Raw 264.7 cells and atopic dermatitis (AD) mice model (45). AD is a chronic allergic disease with severe irritation and inflammation of the skin (46). An AD model in mice was developed by using contact allergens. It was shown that α-MSH suppressed dermatitis in these AD mice (45). Interestingly, it has been shown that the agonists of MC1R and MC5R have inhibitory effects on IgE-mediated allergic inflammation (46).
Early into the investigation of α-MSH, it was shown that it acted as an antipyretic in the setting of experimental fever (47). Glyn and Lipton induced fever by injection of either endogenous or exogenous pyrogens. The pyogenic response was significantly reduced regardless of bacterial endotoxin or PGE2 injection (47). Their findings were later validated by others in rabbit and monkey models (48, 49).
Han et al. studied the effect of α-MSH on nerve repair. They developed a mice autoimmune encephalitis model and suggested that α-MSH promoted the restoration of injured nerves on the spinal cord. Furthermore, α-MSH has an inhibitory effect on the secretion of pro-inflammatory cytokines including IL-2 and IFN-γ (50).
The presence of MC1R and MC3R were detected on wild type mice alveolar macrophages (51). Getting et al. showed that melanocortin peptides inhibit leukocyte accumulation in a mice model of pulmonary allergic and non-allergic inflammation. They found that this protective effect is associated with the activation of MC3R on alveolar macrophages (51).
Allergic airway disease is characterized by exaggerated airway response to agents in the environment. Raap et al. developed a mice model for asthma by three intraperitoneal injections and inhalations of 10 μg of ovalbumin (52). To understand the role of melanocortin signaling in asthma, they injected 1 mg/kg of α-MSH into the tail of mice before sensitization. They found a significant reduction in eosinophil counts in bronchoalveolar lavage (BAL) (P < 0.001) and a significant decrease in serum levels of IgE (p < 0.001) IL-4 and IL-5 (both p < 0.001) among asthmatic mice in comparison to controls. Their study suggests melanocortin receptor agonists have anti-Th2 T-cells activity.
Colombo et al. recently conducted an animal study to discover the potential therapeutic effects of α-MSH on acute lung injury (ALI). They developed an ALI rat model by injecting a 1 mg bleomycin instillation into the trachea. They injected 100 μg of α-MSH immediately after bleomycin and harvested lungs 8, and 24 h later in case and control groups. They found α-MSH significantly prevented lung edema (lung weight in control vs. α-MSH group, 5.8 ± 0.5 vs. 3.9 ± 0.1, respectively, p <0.05 respectively), and reduced circulatory NO (nitrite) concentrations in the case group. Several genes associated with inflammation including Cdkn1a, Hmox1, and Hspa1a were upregulated in mice with ALI that had normalized in the mice injected with α-MSH. Their findings support potential therapeutic properties of α-MSH in ALI (53).
Jiangping et al. discovered the therapeutic effects of α-MSH in ALI by using a mouse model of ALI. They caused bilateral renal ischemia for 40 min in mice to develop ALI. The mice were then given 25 mg of α-MSH or saline in case and control groups before the clamps were removed. The treatment with α-MSH was repeated at 8 and 16 h post ischemia. The leukocyte numbers and injury score in lung specimens demonstrated significant reduction in the α-MSH group in comparison with saline control group. The α-MSH group had significantly lower expressions of TNF-α and intracellular adhesion moelcul-1 (ICAM-1) genes after renal ischemia (54).
Kristensen et al. investigated the role of α-MSH in the systemic inflammatory response syndrome (SIRS). This study employed the use of AP214 which is an analog of α-MSH with six lysine residues at the N-terminus of native α-MSH, resulting in a higher binding affinity to its receptors. They developed a porcine model for SIRS with intravascular infusion of lipopolysaccharide (LPS from Escherichia coli endotoxin). Animals were then randomized to receive either AP214 or saline injections. When the porcine is exposed to LPS, it shows a dose dependent increase in pulmonary vascular resistance, a well-known pathophysiologic change in acute respiratory distress syndrome (ARDS) which is also observed in sepsis. ARDS in the setting of sepsis is triggered in part by endothelial damage as a result of the inflammatory process. Remarkably, the peak pulmonary pressures and pulmonary vascular resistance were significantly (~33%) reduced in the AP214 group (55).
The mechanism of action of α-MSH to inhibit inflammation is not well-known. It is suggested that α-MSH exhibits its effect in attenuating the inflammatory response by down regulating nuclear factor kappa B (NFκB) (56). NFκB is a nuclear transcription factor that plays a key role in the cytokine production. In the absence of inflammatory signals, NFκB is inactive as a heterodimeric molecule, which is composed mainly of two subunits, p65 (RelA), and p50 (NF-κB4) (57). Furthermore, a regulatory protein of NFκB, IκB acts as a molecular off switch. When inflammatory signals are present, IκB undergoes phosphorylation, ubiquitination, and proteolytic degradation allowing for the activation of NFκB, in particular the p65 subunit (57). Authors believe that the cAMP response element binding protein (CREB) activation has a central role in reducing levels of a few pro-inflammatory cytokines such as IFN- γ and IL-7 as shown in Figure 2.
Fibrotic and sclerotic conditions consist of a heterogeneous group of disorders including hypertrophic scars, keloids, scleroderma, systemic sclerosis, cirrhosis of the liver, and interstitial lung fibrosis.
Extracellular matrix (ECM) remodeling is the common denominator in every fibrotic and sclerotic disease regardless of etiology (58). One of the most common models to study fibrosis is the bleomycin model (BLM). Kokot et al. developed a mice model of scleroderma by subcutaneously injecting bleomycin 10 μg per day for 21 days. Mice were treated with α-MSH (5 μg subcutaneously per day) for 3 weeks. Treatment with α-MSH suppressed BLM-induced expression of type I and type III collagen an effect which was cAMP-dependent (59). Luo et al. recently conducted a study asking whether melanocortin signaling is altered in keloid formation. Normal and keloid human fibroblasts were cultured to compare melanocortin receptor expression. In keloid-derived fibroblasts, MC1R gene expression was significantly reduced by less than half compared to normal fibroblasts using RT-PCR (60).
Liver fibrosis results from a progressive accumulation of fibrillar ECM in the liver as a consequence of repeated liver damage. Lee and co-workers developed a liver fibrosis model with injection of carbon tetrachloride (CCl4) 1 ml/kg body weight twice a week for 10 weeks (61). Mice were electrotransferred with the ACTH 1-17 gene after fibrosis development. Histopathology and measurement of collagen contents of the livers showed that transfected mice significantly reversed CCL4 liver fibrosis in comparison to controls (liver collagen content in α-MSH group was 23.7 ± 4.7 vs. 59.7 ± 5.0 μm/mg in control group, p < 0.01).
The effects of α-MSH on attenuating fibrosis is due to a reduction in pro-inflammatory cytokine production and attenuation of procollagen synthesis (62).
Xu et al. investigated effects of an α-MSH analog on a bleomycin pulmonary fibrosis mice model. They found that an α-MSH analog reduced the mRNA expression of type I and III procollagen and production of hydroxyproline in the bleomycin treated mice. They also demonstrated that lung production of TNF-α, IL-6, macrophage inflammatory protein 2, and TGF-β1 was significantly reduced in the α-MSH analog treated group in comparison to controls (63).
Sarcoidosis is a complex, multisystem disorder characterized by non-caseating granulomatous inflammation (64). The presentation of sarcoidosis ranges greatly from asymptomatic with incidental findings on imaging, to significant morbidity and potential mortality secondary to mainly pulmonary insufficiency and cardiac arrhythmia (65). Though there are myriad manifestations of the disease, the lungs and thoracic lymph nodes are almost always involved, causing cough, dyspnea, or a decline in lung function (66). Immunosuppression is the mainstay of treatment as the disease is believed to be mediated largely via immune system dysregulation (67).
Prednisone and ACTH with 39 amino acid (repository corticotropin, a long acting corticotropin) are the only two medications currently approved by The Food and Drug Administration (FDA) for the treatment of sarcoidosis (68, 69). ACTH binds with MCRs and attenuates pro-inflammatory cytokines that play a central role in sarcoidosis. Baughman et al. used high dose repository corticotropin (80 IU) twice per week for 47 subjects with advanced sarcoidosis (70). Twenty seven out of 29 subjects who finished 6 months of treatment showed improvement or stability of disease. Reduction of the oral corticosteroid dose was recorded in 27 subjects. Eighteen subjects were treated for <3 months due to side effects, death, or cost. The observed effect of repository corticotropin in this study could be beyond the effect of steroidogenesis in the adrenal glands. Repository corticotropin may also impart anti-inflammatory properties on immune cells via MCR agonist effects (71).
It has been shown that the granulomatous inflammation of sarcoidosis is regulated by T helper 1 (Th1) activating cytokines including IFN-γ, interleukin-12. There is also a decreased expression of the T helper 2 (Th2) cytokines IL-4 and IL-5 (72). Of note, IL-12 has been shown to be the key regulator of the Th1 immune response and is upregulated in lungs of sarcoidosis. It has been shown that α-MSH inhibits the release of pro-inflammatory Th1 cytokines from alveolar macrophages. When macrophages are treated with an inflammatory endotoxin, there is increased secretion of IL-12 and IFN-γ compared to controls. When these alveolar macrophages are exposed to α-MSH, the production of those Th1 cytokines is significantly decreased (73).
Although α-MSH has been studied for decades, the field of melanocortin agonists in pulmonary disease is in its infancy. The new studies, particularly those targeting the effects of melanocortin agonists in pulmonary diseases should be integrated with genomics and proteomics to gain a complete picture of biologic and cellular processes. The role of MC1R on the lung immune system should be studied more. The effect of MC1R agonists including a-MSH may improve inflammatory lung diseases including sarcoidosis. Our team developed a set of experiments to study the anti-inflammatory properties of α-MSH in sarcoidosis by using an in-vitro and mice model.
Validation via large multicentric studies should be done to confirm the therapeutic properties of melanocortin agonists in sarcoidosis, ILD, and airway diseases.
AM and LL conducted literature review, conducted exploratory analysis and helped to develop the first draft of the manuscript. HA helped to develop figures of the manuscript. AC conducted literature review, and helped in developing the first draft of the manuscript. CZ conducted literature review and helped in writing the paper. MM conducted literature review, conducted exploratory analysis, modeled the 2nd protein structure, and developed the final version of the manuscript.
This work was supported in part by Health Resources and Services Administration contract 234-2005-37011C, and Mallinckrodt Pharmaceuticals for research grant support. The content is the responsibility of the authors alone and does not necessarily reflect the views or policies of the Department of Health and Human Services, nor does mention of trade names, commercial products, or organizations imply endorsement by the U.S. Government.
The authors declare that the research was conducted in the absence of any commercial or financial relationships that could be construed as a potential conflict of interest.
The Supplementary Material for this article can be found online at: https://www.frontiersin.org/articles/10.3389/fmed.2019.00145/full#supplementary-material
Video 1. The 3rd structure of MC1R by using Swiss-Model (26).
1. Bicknell AB. The tissue-specific processing of pro-opiomelanocortin. J Neuroendocrinol. (2008) 20:692–9. doi: 10.1111/j.1365-2826.2008.01709.x
2. Mulcahy LR, Nillni EA. Discovery of new peptides from old prohormones: insights for energy balance and beyond. Front Biosci. (2007)12:3545–53. doi: 10.2741/2333
3. Benjannet S, Rondeau N, Day R, Chretien M, Seidah NG. PC1 and PC2 are proprotein convertases capable of cleaving proopiomelanocortin at distinct pairs of basic residues. Proc Natl Acad Sci USA. (1991) 88:3564–8. doi: 10.1073/pnas.88.9.3564
4. Li JY, Dubois MP, Dubois PM. Ultrastructural localization of immunoreactive corticotropin, beta-lipotropin, alpha- and beta-endorphin in cells of the human fetal anterior pituitary. Cell Tissue Res. (1979) 204:37–51. doi: 10.1007/BF00235163
5. Harris JI, Lerner AB. Amino-acid sequence of the alpha-melanocyte-stimulating hormone. Nature. (1957) 179:1346–7. doi: 10.1038/1791346a0
6. Gallo-Payet N. 60 YEARS OF POMC: adrenal and extra-adrenal functions of ACTH. J Mol Endocrinol. (2016) 56:T135–56. doi: 10.1530/JME-15-0257
7. Evans JF, Fernando A, Ragolia L. Functional melanocortin-2 receptors are expressed by mouse aorta-derived mesenchymal progenitor cells. Mol Cell Endocrinol. (2012) 355:60–70. doi: 10.1016/j.mce.2012.01.019
8. Noon LA, Clark AJ, King PJ. A peroxisome proliferator-response element in the murine mc2-r promoter regulates its transcriptional activation during differentiation of 3T3-L1 adipocytes. J Biol Chem. (2004) 279:22803–8. doi: 10.1074/jbc.M401861200
9. Zaidi M, Sun L, Robinson LJ, Tourkova IL, Liu L, Wang Y, et al. ACTH protects against glucocorticoid-induced osteonecrosis of bone. Proc Natl Acad Sci USA. (2010) 107:8782–7. doi: 10.1073/pnas.0912176107
10. Evans JF, Ragolia L. Systemic and local ACTH produced during inflammatory states promotes osteochondrogenic mesenchymal cell differentiation contributing to the pathologic progression of calcified atherosclerosis. Med Hypotheses. (2012) 79:823–6. doi: 10.1016/j.mehy.2012.09.001
11. Catania A, Cutuli M, Garofalo L, Carlin A, Airaghi L, Barcellini W, et al. The neuropeptide alpha-MSH in host defense. Ann N Y Acad Sci. (2000) 917:227–31. doi: 10.1111/j.1749-6632.2000.tb05387.x
12. Lipton JM, Ceriani G, Macaluso A, McCoy D, Carnes K, Biltz J, et al. Antiinflammatory effects of the neuropeptide alpha-MSH in acute, chronic, and systemic inflammation. Ann N Y Acad Sci. (1994) 741:137–48. doi: 10.1111/j.1749-6632.1994.tb23095.x
13. Lipton JM, Catania A. Anti-inflammatory actions of the neuroimmunomodulator alpha-MSH. Immunol Today. (1997) 18:140–5. doi: 10.1016/S0167-5699(97)01009-8
14. Cutuli M, Cristiani S, Lipton JM, Catania A. Antimicrobial effects of alpha-MSH peptides. J Leukoc Biol. (2000) 67:233–9. doi: 10.1002/jlb.67.2.233
15. Bennett HP. Biosynthetic fate of the amino-terminal fragment of pro-opiomelanocortin within the intermediate lobe of the mouse pituitary. Peptides. (1986) 7:615–22. doi: 10.1016/0196-9781(86)90036-7
16. Biebermann H, Castaneda TR, van Landeghem F, von Deimling A, Escher F, Brabant G, et al. A role for beta-melanocyte-stimulating hormone in human body-weight regulation. Cell Metab. (2006) 3:141–6. doi: 10.1016/j.cmet.2006.01.007
17. Roselli-Rehfuss L, Mountjoy KG, Robbins LS, Mortrud MT, Low MJ, Tatro JB, et al. Identification of a receptor for gamma melanotropin and other proopiomelanocortin peptides in the hypothalamus and limbic system. Proc Natl Acad Sci USA. (1993) 90:8856–60. doi: 10.1073/pnas.90.19.8856
18. Pedersen RC, Brownie AC. Lys-gamma 3-melanotropin binds with high affinity to the rat adrenal cortex. Endocrinology. (1983) 112:1279–87. doi: 10.1210/endo-112-4-1279
19. Yang Y. Structure, function and regulation of the melanocortin receptors. Eur J Pharmacol. (2011) 660:125–30. doi: 10.1016/j.ejphar.2010.12.020
20. Switonski M, Mankowska M, Salamon S. Family of melanocortin receptor (MCR) genes in mammals-mutations, polymorphisms and phenotypic effects. J Appl Genet. (2013) 54:461–72. doi: 10.1007/s13353-013-0163-z
21. Roy S, Roy SJ, Pinard S, Taillefer LD, Rached M, Parent JL, et al. Mechanisms of melanocortin-2 receptor (MC2R) internalization and recycling in human embryonic kidney (hek) cells: identification of Key Ser/Thr (S/T) amino acids. Mol Endocrinol. (2011) 25:1961–77. doi: 10.1210/me.2011-0018
22. Joshi AD, Li X, Kraft P, Han J. Hierarchical modeling of melanocortin 1 receptor variants with skin cancer risk. Genet Epidemiol. (2018) 42:571–86. doi: 10.1002/gepi.22137
23. Zhang Y, Wu X, He Y, Kastin AJ, Hsuchou H, Rosenblum CI, et al. Melanocortin potentiates leptin-induced STAT3 signaling via MAPK pathway. J Neurochem. (2009) 110:390–9. doi: 10.1111/j.1471-4159.2009.06144.x
24. Cone RD. Studies on the physiological functions of the melanocortin system. Endocr Rev. (2006) 27:736–49. doi: 10.1210/er.2006-0034
25. Chhajlani V, Wikberg JE. Molecular cloning and expression of the human melanocyte stimulating hormone receptor cDNA. FEBS Lett. (1992) 309:417–20. doi: 10.1016/0014-5793(92)80820-7
26. Guex N, Peitsch MC, Schwede T. Automated comparative protein structure modeling with SWISS-MODEL and Swiss-PdbViewer: a historical perspective. Electrophoresis. (2009) 30(Suppl. 1):S162–73. doi: 10.1002/elps.200900140
27. Chhajlani V, Wikberg JE. Molecular cloning and expression of the human melanocyte stimulating hormone receptor cDNA (FEBS 11553). FEBS Lett. (1996) 390:238. doi: 10.1016/0014-5793(96)81375-5
28. Brzoska T, Luger TA, Maaser C, Abels C, Bohm M. Alpha-melanocyte-stimulating hormone and related tripeptides: biochemistry, antiinflammatory and protective effects in vitro and in vivo, and future perspectives for the treatment of immune-mediated inflammatory diseases. Endocr Rev. (2008) 29:581–602. doi: 10.1210/er.2007-0027
29. Seaton ME, Parent BA, Sood RF, Wurfel MM, Muffley LA, O'Keefe GE, et al. Melanocortin-1 receptor polymorphisms and the risk of complicated sepsis after trauma: a candidate gene association study. Shock. (2017) 47:79–85. doi: 10.1097/SHK.0000000000000708
30. Vamvakopoulos NC, Rojas K, Overhauser J, Durkin AS, Nierman WC, Chrousos GP. Mapping the human melanocortin 2 receptor (adrenocorticotropic hormone receptor; ACTHR) gene (MC2R) to the small arm of chromosome 18 (18p11.21-pter). Genomics. (1993) 18:454–5. doi: 10.1006/geno.1993.1499
31. Ruggiero C, Lalli E. Impact of ACTH signaling on transcriptional regulation of steroidogenic genes. Front Endocrinol. (2016) 7:24. doi: 10.3389/fendo.2016.00024
32. Lin L, Hindmarsh PC, Metherell LA, Alzyoud M, Al-Ali M, Brain CE, et al. Severe loss-of-function mutations in the adrenocorticotropin receptor (ACTHR, MC2R) can be found in patients diagnosed with salt-losing adrenal hypoplasia. Clin Endocrinol. (2007) 66:205–10. doi: 10.1111/j.1365-2265.2006.02709.x
33. Gantz I, Konda Y, Tashiro T, Shimoto Y, Miwa H, Munzert G, et al. Molecular cloning of a novel melanocortin receptor. J Biol Chem. (1993) 268:8246–50.
34. Bertolini A, Tacchi R, Vergoni AV. Brain effects of melanocortins. Pharmacol Res. (2009) 59:13–47. doi: 10.1016/j.phrs.2008.10.005
35. Gantz I, Miwa H, Konda Y, Shimoto Y, Tashiro T, Watson SJ, et al. Molecular cloning, expression, and gene localization of a fourth melanocortin receptor. J Biol Chem. (1993) 268:15174–9.
36. Kishi T, Aschkenasi CJ, Lee CE, Mountjoy KG, Saper CB, Elmquist JK. Expression of melanocortin 4 receptor mRNA in the central nervous system of the rat. J Comp Neurol. (2003) 457:213–35. doi: 10.1002/cne.10454
37. Lasaga M, Debeljuk L, Durand D, Scimonelli TN, Caruso C. Role of alpha-melanocyte stimulating hormone and melanocortin 4 receptor in brain inflammation. Peptides. (2008) 29:1825–35. doi: 10.1016/j.peptides.2008.06.009
38. Clement K, Biebermann H, Farooqi IS, Van der Ploeg L, Wolters B, Poitou C, et al. MC4R agonism promotes durable weight loss in patients with leptin receptor deficiency. Nat Med. (2018) 24:551–5. doi: 10.1038/s41591-018-0015-9
39. Huszar D, Lynch CA, Fairchild-Huntress V, Dunmore JH, Fang Q, Berkemeier LR, et al. Targeted disruption of the melanocortin-4 receptor results in obesity in mice. Cell. (1997) 88:131–41. doi: 10.1016/S0092-8674(00)81865-6
40. Gantz I, Shimoto Y, Konda Y, Miwa H, Dickinson CJ, Yamada T. Molecular cloning, expression, and characterization of a fifth melanocortin receptor. Biochem Biophys Res Commun. (1994) 200:1214–20. doi: 10.1006/bbrc.1994.1580
41. Catania A, Gatti S, Colombo G, Lipton JM. Targeting melanocortin receptors as a novel strategy to control inflammation. Pharmacol Rev. (2004) 56:1–29. doi: 10.1124/pr.56.1.1
42. Chhajlani V. Distribution of cDNA for melanocortin receptor subtypes in human tissues. Biochem Mol Biol Int. (1996) 38:73–80.
43. Chen W, Kelly MA, Opitz-Araya X, Thomas RE, Low MJ, Cone RD. Exocrine gland dysfunction in MC5-R-deficient mice: evidence for coordinated regulation of exocrine gland function by melanocortin peptides. Cell. (1997) 91:789–98. doi: 10.1016/S0092-8674(00)80467-5
44. Trotta MC, Maisto R, Alessio N, Hermenean A, D'Amico M, Di Filippo C. The Melanocortin MC5R as a new target for treatment of high glucose-induced hypertrophy of the cardiac H9c2 cells. Front Physiol. (2018) 9:1475. doi: 10.3389/fphys.2018.01475
45. Chen W, Li J, Qu H, Song Z, Yang Z, Huo J, et al. The melanocortin 1 receptor (MC1R) inhibits the inflammatory response in Raw 264.7 cells and atopic dermatitis (AD) mouse model. Mol Biol Rep. (2013) 40:1987–96. doi: 10.1007/s11033-012-2256-x
46. Etori M, Yonekubo K, Sato E, Mizukami K, Hirahara K, Karasuyama H, et al. Melanocortin receptors 1 and 5 might mediate inhibitory effects of α-melanocyte-stimulating hormone on antigen-induced chronic allergic skin inflammation in IgE transgenic mice. J Invest Dermatol. (2012) 132:1925–7. doi: 10.1038/jid.2012.68
47. Glyn JR, Lipton JM. Hypothermic and antipyretic effects of centrally administered ACTH (1–24) and alpha-melanotropin. Peptides. (1981) 2:177–87. doi: 10.1016/S0196-9781(81)80032-0
48. Glyn-Ballinger JR, Bernardini GL, Lipton JM. alpha-MSH injected into the septal region reduces fever in rabbits. Peptides. (1983) 4:199–203. doi: 10.1016/0196-9781(83)90114-6
49. Shih ST, Lipton JM. Intravenous alpha-MSH reduces fever in the squirrel monkey. Peptides. (1985) 6:685–7. doi: 10.1016/0196-9781(85)90172-X
50. Han D, Tian Y, Zhang M, Zhou Z, Lu J. Prevention and treatment of experimental autoimmune encephalomyelitis with recombinant adeno-associated virus-mediated alpha-melanocyte-stimulating hormone-transduced PLP139–151-specific T cells. Gene Ther. (2007)14:383–95. doi: 10.1038/sj.gt.3302862
51. Getting SJ, Riffo-Vasquez Y, Pitchford S, Kaneva M, Grieco P, Page CP, et al. A role for MC3R in modulating lung inflammation. Pulm Pharmacol Ther. (2008) 21:866–73. doi: 10.1016/j.pupt.2008.09.004
52. Raap U, Brzoska T, Sohl S, Path G, Emmel J, Herz U, et al. Alpha-melanocyte-stimulating hormone inhibits allergic airway inflammation. J Immunol. (2003) 171:353–9. doi: 10.4049/jimmunol.171.1.353
53. Colombo G, Gatti S, Sordi A, Turcatti F, Carlin A, Rossi C, et al. Production and effects of alpha-melanocyte-stimulating hormone during acute lung injury. Shock. (2007) 27:326–33. doi: 10.1097/01.shk.0000239764.80033.7e
54. Deng J, Hu X, Yuen PS, Star RA. Alpha-melanocyte-stimulating hormone inhibits lung injury after renal ischemia/reperfusion. Am J Respir Crit Care Med. (2004) 169:749–56. doi: 10.1164/rccm.200303-372OC
55. Kristensen J, Jonassen TE, Rehling M, Tonnesen E, Sloth E, Nielsen S, et al. The α-MSH analogue AP214 attenuates rise in pulmonary pressure and fall in ejection fraction in lipopolysaccharide-induced systemic inflammatory response syndrome in pigs. Clin Physiol Funct Imaging. (2011) 31:54–60. doi: 10.1111/j.1475-097X.2010.00979.x
56. Manna SK, Aggarwal BB. Alpha-melanocyte-stimulating hormone inhibits the nuclear transcription factor NF-kappa B activation induced by various inflammatory agents. J Immunol. (1998) 161:2873–80.
57. Haddad JJ, Lauterbach R, Saade NE, Safieh-Garabedian B, Land SC. Alpha-melanocyte-related tripeptide, Lys-d-Pro-Val, ameliorates endotoxin-induced nuclear factor kappaB translocation and activation: evidence for involvement of an interleukin-1beta193–195 receptor antagonism in the alveolar epithelium. Biochem J. (2001) 355:29–38. doi: 10.1042/bj3550029
58. Karsdal MA, Nielsen MJ, Sand JM, Henriksen K, Genovese F, Bay-Jensen AC, et al. Extracellular matrix remodeling: the common denominator in connective tissue diseases. Possibilities for evaluation and current understanding of the matrix as more than a passive architecture, but a key player in tissue failure. Assay Drug Dev Technol. (2013) 11:70–92. doi: 10.1089/adt.2012.474
59. Kokot A, Sindrilaru A, Schiller M, Sunderkotter C, Kerkhoff C, Eckes B, et al. alpha-melanocyte-stimulating hormone suppresses bleomycin-induced collagen synthesis and reduces tissue fibrosis in a mouse model of scleroderma: melanocortin peptides as a novel treatment strategy for scleroderma? Arthrit Rheum. (2009) 60:592–603. doi: 10.1002/art.24228
60. Luo LF, Shi Y, Zhou Q, Xu SZ, Lei TC. Insufficient expression of the melanocortin-1 receptor by human dermal fibroblasts contributes to excess collagen synthesis in keloid scars. Exp Dermatol. (2013) 22:764–6. doi: 10.1111/exd.12250
61. Lee TH, Jawan B, Chou WY, Lu CN, Wu CL, Kuo HM, et al. Alpha-melanocyte-stimulating hormone gene therapy reverses carbon tetrachloride induced liver fibrosis in mice. J Gene Med. (2006) 8:764–72. doi: 10.1002/jgm.899
62. Bohm M, Raghunath M, Sunderkotter C, Schiller M, Stander S, Brzoska T, et al. Collagen metabolism is a novel target of the neuropeptide α-melanocyte-stimulating hormone. J Biol Chem. (2004) 279:6959–66. doi: 10.1074/jbc.M312549200
63. Xu PB, Mao YF, Meng HB, Tian YP, Deng XM. STY39, a novel alpha-melanocyte-stimulating hormone analogue, attenuates bleomycin-induced pulmonary inflammation and fibrosis in mice. Shock. (2011) 35:308–14. doi: 10.1097/SHK.0b013e3181f8f15e
64. Mirsaeidi M, Machado RF, Schraufnagel D, Sweiss NJ, Baughman RP. Racial difference in sarcoidosis mortality in the United States. Chest. (2015) 147:438–49. doi: 10.1378/chest.14-1120
65. Al-Kofahi K, Korsten P, Ascoli C, Virupannavar S, Mirsaeidi M, Chang I, et al. Management of extrapulmonary sarcoidosis: challenges and solutions. Ther Clin Risk Manag. (2016) 12:1623–34. doi: 10.2147/TCRM.S74476
66. Newman LS, Rose CS, Maier LA. Sarcoidosis. N Engl J Med. (1997) 336:1224–34. doi: 10.1056/NEJM199704243361706
67. Enzenauer RJ, West SG. Sarcoidosis in autoimmune disease. Semin Arthritis Rheum. (1992) 22:1–17. doi: 10.1016/0049-0172(92)90043-D
68. Michael M Jr, Cole R, Beeson PB, Olson B. Sarcoidosis: preliminary observations from an analysis of 350 cases. Trans Annu Meet Natl Tuberc Assoc. (1950)46:208–12.
69. Miller MA, Bass HE. Effect of acthar-c (ACTH) in sarcoidosis. Ann Intern Med. (1952) 37:776–84. doi: 10.7326/0003-4819-37-4-776
70. Baughman RP, Barney JB, O'Hare L, Lower EE. A retrospective pilot study examining the use of Acthar gel in sarcoidosis patients. Respir Med. (2016) 110:66–72. doi: 10.1016/j.rmed.2015.11.007
71. Ross AP, Ben-Zacharia A, Harris C, Smrtka J. Multiple sclerosis, relapses, and the mechanism of action of adrenocorticotropic hormone. Front Neurol. (2013) 4:21. doi: 10.3389/fneur.2013.00021
72. Moller DR. Cells and cytokines involved in the pathogenesis of sarcoidosis. Sarcoidosis Vasc Diffuse Lung Dis. (1999) 16:24–31.
Keywords: MCR, ACTH, α-MSH, sarcoidosis, melanocortin
Citation: Moscowitz AE, Asif H, Lindenmaier LB, Calzadilla A, Zhang C and Mirsaeidi M (2019) The Importance of Melanocortin Receptors and Their Agonists in Pulmonary Disease. Front. Med. 6:145. doi: 10.3389/fmed.2019.00145
Received: 14 March 2019; Accepted: 10 June 2019;
Published: 27 June 2019.
Edited by:
Argyrios Tzouvelekis, Alexander Fleming Biomedical Sciences Research Center, GreeceReviewed by:
Esmaeil Mortaz, National Research Institute Tuberculosis and Lung Diseases, IranCopyright © 2019 Moscowitz, Asif, Lindenmaier, Calzadilla, Zhang and Mirsaeidi. This is an open-access article distributed under the terms of the Creative Commons Attribution License (CC BY). The use, distribution or reproduction in other forums is permitted, provided the original author(s) and the copyright owner(s) are credited and that the original publication in this journal is cited, in accordance with accepted academic practice. No use, distribution or reproduction is permitted which does not comply with these terms.
*Correspondence: Mehdi Mirsaeidi, bXNtMjQ5QG1lZC5taWFtaS5lZHU=
Disclaimer: All claims expressed in this article are solely those of the authors and do not necessarily represent those of their affiliated organizations, or those of the publisher, the editors and the reviewers. Any product that may be evaluated in this article or claim that may be made by its manufacturer is not guaranteed or endorsed by the publisher.
Research integrity at Frontiers
Learn more about the work of our research integrity team to safeguard the quality of each article we publish.