- 1Division of Infectious Diseases, Los Angeles Biomedical Research Institute at UCLA Medical Center, Torrance, CA, United States
- 2David Geffen School of Medicine at UCLA, Los Angeles, CA, United States
Tedizolid (TZD) is an oxazolidinone derivative which demonstrates bacteriostatic activity through inhibition of protein synthesis. We compared the efficacies of TZD and an earlier-generation oxazolidinone, linezolid (LZD), in an experimental murine model of bacteremia caused by two VRE strains (one each E. faecium and E. faecalis). LZD exhibited significantly better efficacy in terms of reduced VRE blood and target tissue densities than TZD in this model.
Text
Infections caused by enterococci (especially VRE strains) are major problems worldwide (1–4). VRE strains, particularly in immunocompromised patients, are a major and steadily increasing cause of bacteremia (5). VRE bloodstream infections (VRE-BSI) rank as one of the top four major infections among hospitalized patients (5). Commonly used antimicrobial agents used to treat VRE-BSI infections, such as daptomycin, oritavancin, dalbavancin, quinupristin-dalfopristin, and tigecycline, each have a number of important side effects, both acutely and more long-term (2). Currently, optimal treatment for VRE-BSI remains problematic and undefined. Thus, plausible alternative approaches for the treatment of such infections are urgently required.
We have recently employed a discriminative murine model of acute VRE-BSI to measure the host's ability to control infection and the impact of selected therapeutic interventions (1, 2). TZD is an oxazolidinone-class antibiotic, approved by the FDA in 2014, for the treatment of acute bacterial skin and skin structure infections (ABSSSI) caused by certain bacteria, including MRSA,VRE and various Streptococcus species (3). Recently Sahm et al. evaluated TZD's overall activity and emergence of resistance in gram-positive bacteria (3). In addition, the comparative efficacy of LZD and daptomycin in the same experimental murine model of VRE bacteremia were reported before (1, 2). However, to our knowledge, there have been no studies that have examined a head-to-head comparative efficacy analysis of TZD vs. LZD in this experimental VRE-BSI model.
In parallel to in vivo studies, we also tested TZD vs. LZD to assess their comparative capacities to exert in vitro bactericidal impacts on both VRE study strains. The LZD and TZD MICs (minimum inhibitory concentration; lowest concentration of antibiotics which kills or prevents the minimum growth of the bacteria) of the 613 and 447 VRE strains were carried out by microbroth dilution assay (6). We then employed a range of LZD and TDZ concentrations in time-kill curves, ranging from sub-MIC to 5 × MIC, utilizing an initial inoculum of ~1 × 105 CFU/mL (7). We selected the following incubation time-points, for analyses (0, 2, 4, 6, 24 h of incubation at 37°C). Thus, an aliquot of all reaction tubes was quantitatively cultured at each time-point (7). Summary data were expressed as mean log10 CFU/mL (±SD) of surviving counts. A minimum of two experimental runs were performed on separate days. A “bactericidal effect” was defined as at least a 3 log10 CFU/mL reduction in counts as compared to the initial inoculum (7).
In this investigation, we studied the comparative efficacy of TZD vs. LZD in an acute murine VRE-BSI model, using two well-characterized VRE strains (E. faecalis 613 and E. faecium 447) (4, 6); For inducing this experimental infection, a tail vein challenge of BALB/c mice with our two VRE study strains above was done. The final inoculum utilized for each strain (1 × 109 CFU/mL) was determined by a number of pilot studies that showed significant infection levels in the blood and three target organs (lung, kidney, spleen) in each animal, without excessive mortality (see below). The mice were purchased from Jackson Lab Laboratory, Bar Harbor ME. All studies were approved by the Los Angeles Biomedical Research Institute IACUC Committee.
In brief, our initial pilot studies were focused on determining the inoculum of the two VRE strains that resulted in a at least a 95% infectious dose (ID95) for this murine model. The ID95 for this study represented the optimal inoculum resulting in a non-lethal, reliable and durable infection for 95% of animals. For these inoculum-ranging studies, infection was given by tail vein challenge of BALB/c mice at a 106, 107, 108, or 109 CFU/mL inocula. These inocula represent a standard inoculum range for this murine model of VRE-BSI. At 24 h post-challenge, animals were sacrificed, and blood, kidney spleen and lung were sterilely removed and quantitatively cultured in BHI media (1, 2). Individual target organ summary data were expressed as either log10 CFU/mL blood or log10 CFU/g tissue.
On the basis of the ID95 data, (see Supplementary Table 1), BALB/c mice were given a tail vein challenge of the 1 × 109 CFU/mL inoculum, then randomized at 24 h post-infection to receive either: (i) no therapy (controls); (ii) LZD for 3 d; or (iii) TZD for 3 d. The dose-regimen of LZD and TZD were: 120 mg/kg SC bid, or 10 mg/kg/day ip, respectively. These doses were selected for the in vivo studies based on their simulated targeted human PK-PD profiles (8, 9). At least 24 h after the last TZD or LZD dose (day 4), one-half of surviving mice were euthanized for an end-of-therapy evaluation (EOT), with blood, kidney, spleen and lung removed and quantitatively cultured as above. For the remaining one-half of surviving animals, an evaluation of “relapse” was carried out on day 8 (i.e., after 4 antibiotic treatment-free days); at sacrifice, the blood and target tissues were again removed and quantitatively cultured as above (1, 2).
The two-tailed Student t-test was used for statistical analyses of quantitative data. P ≤ 0.05 were considered significant.
The mean TZD and LZD MICs were (0.25 and 0.5 μg/mL) and (1.0 and 2.0 μg/mL) for 447 and 613 VRE strains, respectively. As shown in Figure 1, (Supplementary Table 2), neither LZD nor TZD was bactericidal for either VRE strain. Thus, both agents were essentially bacteriostatic against the VRE study strains in vitro.
In the VR-E. faecium 447 strain, in comparing the treatment and relapse outcomes of LZD vs. TZD, LZD resulted in a significantly lower kidney bacterial burden (P < 0.05); this same difference was not found in other target tissues (Table 1). Similarly, LZD significantly prevented the relapse of infection with this strain in the kidney. Further, the treatment outcome of LZD vs. TZD in VR-E. faecalis 613 strain, LZD treatment yielded significantly lower CFU counts in all target organs (P < 0.05) (Table 1). However, the relapse group microbiologic outcomes comparing LZD vs. TZD for this strain were similar, and the data were not statistically significant (Table 1).
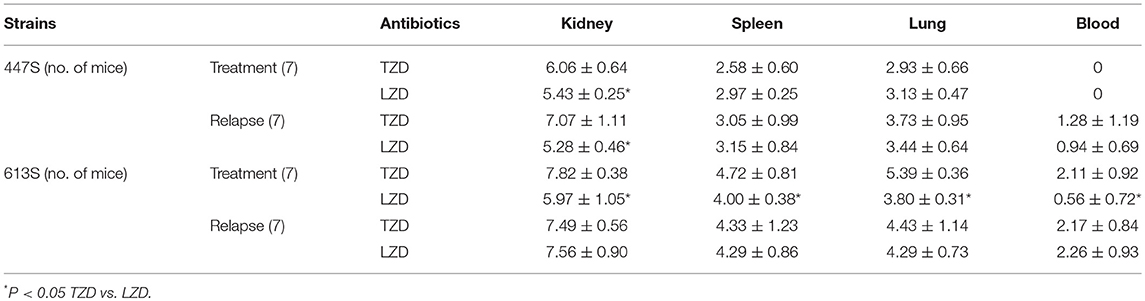
Table 1. VRE-BSI using 447 E. faecium and 613 E. faecalis comparing LZD and TZD in terms of tissue burden (CFUcount) [log CFU/g ±SD] at EOT on day 4.
In our study, neither LZD nor TZD was highly efficacious in this murine VRE-BSI model, although, overall, LZD seemed to yield better treatment outcomes than TZD. Paradoxically, neither drug was able to exert a bactericidal effect in vitro against either VRE strain. This suggested that the modestly enhanced in vivo impact of LZD in this model may relate to an in vivo “synergy” between this agent and innate host defense cells (e.g., PMNs) and/or molecules (e.g., host defense peptides; antibody, complement, etc.) (10, 11). In contrast to our data, there have been several studies carried out by various groups, including us, regarding the comparative activity of TZD vs. LZD in Staphylococcus aureus, enterococci and Streptococcus pneumonia, employing distinct in vitro and in vivo scenarios, suggesting that TZD had significantly better activity than LZD (12–16).
We recognize that our current study have methodologic challenges which somewhat limit interpretation. For example, we studied only one E.faecium and E. faecalis strain in single in vivo model. Thus, further studies (including murine BSI model) in the distinct in vivo infection model such as endovascular and skin and soft tissue infection model etc., comparing TZD and LZD, and utilizing additional strains of VR-E. faecium and VR-E. faecalis, will be required to adjudicate our observations.
Ethics Statement
Animals were maintained in accordance with the American Association for Accreditation of Laboratory Animal Care criteria and were cared for in accordance with national guidance. Animal studies were approved by the LABioMed at Harbor-UCLA Medical Center IACUC Committee.
Author Contributions
All authors listed have made a substantial, direct and intellectual contribution to the work, and approved it for publication.
Funding
This research was supported by grants from Merck & Co. Inc. (grant #55187 and #58305) to NM. Merck would support the publication cost after acceptance of manuscript to publish in Frontiers in Medicine.
Conflict of Interest Statement
The authors declare that the research was conducted in the absence of any commercial or financial relationships that could be construed as a potential conflict of interest.
Acknowledgments
We thank Dr. Cesar Arias (School of Medicine, University of Texas, Houston) for providing the VRE strains. We are thankful to Prof. Arnold Bayer, David Geffen School of Medicine at UCLA and LA Biomed at Harbor-UCLA Med. Ctr. for critically reviewing the manuscript and making valuable suggestions. This work was presented in part at the 27th European Congress of Clinical Microbiology and Infectious Diseases (ECCMID) Meeting, Vienna, Austria, April 22-25, 2017, Abstract number 4200.
Supplementary Material
The Supplementary Material for this article can be found online at: https://www.frontiersin.org/articles/10.3389/fmed.2019.00031/full#supplementary-material
References
1. Xiong YQ, Abdelhady W, Elles SJ, Bayer AS, Mckinnell JA. Comparison of linezolid and daptomycin in an experimental murine model of VRE faecium bacteremia; emergence of daptomycin – nonsusceptibility during daptomycin treatment. In: ICAAC (Interscience conference on Antimicrobial Agents and Chemotherapy) Meeting, Poster # 1365 Torrance, CA.
2. Xiong YQ, Abdelhady W, Elles SJ, Bayer AS, Mckinnell JA. Murine model of acute vancomycin-resistant enterococcal (VRE) bacteremia. In: ICAAC meeting, Poster # 1351 Torrance, CA.
3. Sahm Deane J, Bien PA, Locke JB, Zuill DE, Shaw KJ, Bartizal KF. Results of the surveillance of tedizolid activity and resistance program: in vitro susceptibility of gram-positive pathogens collected in 2011 and 2012 from the United States and Europe. Diagn. Microbiol Infect Dis. (2015) 81:112–8. doi: 10.1016/j.diagmicrobio.2014.08.011
4. Mishra NN, Bayer AS, Tran TT, Shamoo Y, Mileykovskaya E, Dowhan W, et al. Daptomycin resistance in enterococci is associated with distinct alterations of cell membrane phospholipid content. PLoS ONE (2012) 7:e43958. doi: 10.1371/journal.pone.0043958
5. Benamu E, Deresinski S. Vancomycin-resistant enterococcus infection in the hematopoietic stem cell transplant recipient: an overview of epidemiology, management, and prevention. F1000 Res. (2018) 7:3. doi: 10.12688/f1000research.11831.1
6. Arias CA, Panesso D, McGrath DM, Qin X, Mojica MF, Miller C, et al. Genetic basis for in vivo daptomycin resistance in enterococci. N Engl J Med. (2011) 365:892–900. doi: 10.1056/NEJMoa1011138
7. Zapata B, Alvarez DN, Farah S, Garcia-de-la-Maria C, Miro JM, Sakoulas G, et al. Prevention of high-level daptomycin-resistance emergence in vitro in Streptococcus mitis-oralis by using combination antimicrobial strategies. Curr Microbiol. (2018) 75:1062–7. doi: 10.1007/s00284-018-1491-3
8. Lodise TP, Drusano GL. Use of pharmacokinetic/pharmacodynamic systems analyses to inform dose selection of tedizolid phosphate. Clin Infect Dis. (2014) 58 (Suppl. 1):S28–34. doi: 10.1093/cid/cit615
9. Slatter JG, Adams LA, Bush EC, Chiba K, Daley-Yates PT, Feenstra KL, et al. Pharmacokinetics, toxicokinetics, distribution, metabolism and excretion of linezolid in mouse, rat and dog. Xenobiotica (2002) 32:907–24. doi: 10.1080/00498250210158249
10. Xiong YQ, Bayer AS, Yeaman MR. Inhibition of intracellular macromolecular synthesis in Staphylococcus aureus by thrombin-induced platelet microbicidal proteins. J Infect Dis. (2002) 185:348–56. doi: 10.1086/338514
11. Mishra NN, Yang SJ, Chen L, Muller C, Saleh-Mghir A, Kuhn S, et al. Emergence of daptomycin resistance in daptomycin-naïve rabbits with methicillin-resistant Staphylococcus aureus prosthetic joint infection is associated with resistance to host defense cationic peptides and mprF polymorphisms. PLoS ONE (2013) 8:e71151. doi: 10.1371/journal.pone.0071151
12. Choi S, Im W, Bartizal K. Activity of tedizolid phosphate (TR-701) in murine models of infection with penicillin-resistant and penicillin-sensitive Streptococcus pneumoniae. Antimicrob Agents Chemother. (2012) 56:4713–7. doi: 10.1128/AAC.00346-12
13. Tessier PR, Keel RA, Hagihara M, Crandon JL, Nicolau DP. Comparative in vivo efficacies of epithelial lining fluid exposures of tedizolid, linezolid, and vancomycin for methicillin-resistant Staphylococcus aureus in a mouse pneumonia model. Antimicrob Agents Chemother. (2012) 56:2342–6. doi: 10.1128/AAC.06427-11
14. Housman ST, Pope JS, Russomanno J, Salerno E, Shore E, Kuti JL, Nicolau DP. Pulmonary disposition of tedizolid following administration of once-daily oral 200-milligram tedizolid phosphate in healthy adult volunteers. Antimicrob Agents Chemother. (2012) 56:2627–234. doi: 10.1128/AAC.05354-11
15. Bayer AS, Abdelhady W, Li L, Gonzales R, Xiong YQ. Comparative efficacies of tedizolid phosphate, linezolid, and vancomycin in a murine model of subcutaneous catheter-related biofilm infection due to methicillin-susceptible and -resistant Staphylococcus aureus. Antimicrob Agents Chemother. (2016) 60:5092–6. doi: 10.1128/AAC.00880-16
Keywords: TZD, LZD, murine model, VRE, bacteremia
Citation: Abdelhady W and Mishra NN (2019) Comparative Efficacies of Linezolid vs. Tedizolid in an Experimental Murine Model of Vancomycin-Resistant Enterococcal (VRE) Bacteremia. Front. Med. 6:31. doi: 10.3389/fmed.2019.00031
Received: 12 December 2018; Accepted: 04 February 2019;
Published: 20 February 2019.
Edited by:
George Dimopoulos, University General Hospital Attikon, GreeceReviewed by:
Gennaro De Pascale, Università Cattolica del Sacro Cuore, ItalyTianyu Zhang, Guangzhou Institutes of Biomedicine and Health (CAS), China
Copyright © 2019 Abdelhady and Mishra. This is an open-access article distributed under the terms of the Creative Commons Attribution License (CC BY). The use, distribution or reproduction in other forums is permitted, provided the original author(s) and the copyright owner(s) are credited and that the original publication in this journal is cited, in accordance with accepted academic practice. No use, distribution or reproduction is permitted which does not comply with these terms.
*Correspondence: Nagendra N. Mishra, nnmishra@ucla.edu; nmishra@labiomed.org