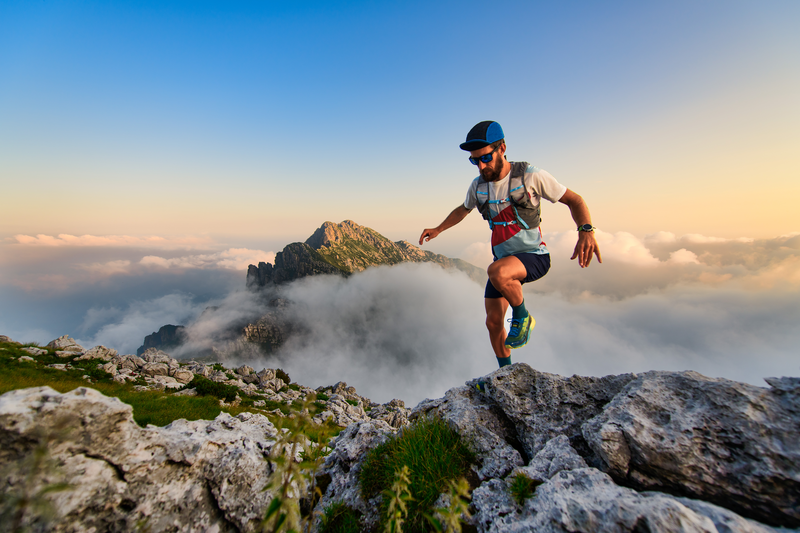
94% of researchers rate our articles as excellent or good
Learn more about the work of our research integrity team to safeguard the quality of each article we publish.
Find out more
ORIGINAL RESEARCH article
Front. Med. , 14 August 2018
Sec. Infectious Diseases: Pathogenesis and Therapy
Volume 5 - 2018 | https://doi.org/10.3389/fmed.2018.00225
Introduction: Klebsiella pneumoniae is one of the most important infectious agents in neonates. There are “classic” and hypervirulent strains of K. pneumoniae. The “classic” non-virulent strain of K. pneumoniae, producing extended-spectrum beta-lactamases (ESBLs), is associated with nosocomial infections. Hypervirulent K. pneumoniae strains are associated with invasive infections in previously healthy adult people, and most of them exhibit antimicrobial susceptibility. The role of virulent strains of K. pneumoniae (including hv-KP) in neonatal infections is unknown. The aim of the study was the assessment of the impact of virulence factors and antibiotic resistance of K. pneumoniae strains on clinical features and outcomes of neonatal infection.
Materials and Methods: Two groups of infants were enrolled. The first group consisted of 10 neonates with sepsis caused by K. pneumoniae. The second group consisted of 10 neonates with urinary tract infection (UTI) caused by K. pneumoniae. We investigated the susceptibility of K. pneumoniae isolates to antibiotics, the ability of the microorganism to produce ESBL, and virulence factors, including the rmpA gene, aerobactin, and colibactin genes. In neonates with sepsis, we investigated K. pneumoniae isolates, which was taken from the blood, in neonates with UTI—from the urine.
Results: In neonates with sepsis testing of K. pneumoniae isolates for ESBL production was positive in 60% of cases, in neonates with UTI—in 40% of cases. All blood and urine ESBL producing K. pneumoniae isolates were resistant to ampicillins, including protected ones, and third-generation cephalosporins. At the same time, these isolates were sensitive to meropenem, amikacin, and ciprofloxacin. The rmpA gene was detected in four blood, and three urine K. pneumoniae isolates. In neonates with sepsis rmpA gene in two cases was detected in ESBL-producing K. pneumoniae isolates. They were infants with meningitis, and both cases were fatal. In the group of infants with UTI, the rmpA gene was detected only in K. pneumoniae isolates not producing ESBL. Aerobactin and colibactin genes were detected in two neonates with sepsis and in three neonates with UTI. In all cases, aerobactin and colibactin genes were detected only in rmpA-positive K. pneumoniae isolates. Out of three fatal outcomes, two cases were caused by hv-KP producing ESBL.
Conclusion: The prevalence of virulent strains of K. pneumoniae among neonates with sepsis and other neonatal infection is higher than we think. The most severe forms of neonatal sepsis with an unfavorable outcome in our study were due to virulent strains of K. pneumoniae.
Klebsiella pneumoniae is one of the leading causes of neonatal sepsis (NS) (1, 2). This microorganism accounts for NS in 4–9% of cases in developed countries, and 16–28% in developing countries (3–6). There are “classic” and hypervirulent strains of K. pneumoniae (7). “Classic” non-virulent K. pneumoniae (c-KP) strains are usually associated with pneumonia, urinary tract infection, nosocomial infections, and neonatal sepsis in immunocompromised patients (8). C-KP can cause NS outbreaks in hospitals and ICU (9, 10). C-KP strains have recently gained notoriety due to their propensity to acquire antimicrobial resistance. Within the last few decades, extended-spectrum β-lactamase (ESBL)-positive K. pneumoniae isolates have been recovered worldwide, especially in intensive care units (ICU) (11). The prevalence of ESBL-producing strains of K. pneumoniae is 23% in the USA, and up to 85–100% in some European countries (11). Hypervirulent strains of K. pneumoniae (hv-KP) were first recognized in Taiwan in the last twentieth century and caused liver abscesses, meningitis, and endophthalmitis in previously healthy adult patients (12–14). Currently, hv-KP strains are being spread in different parts of the world (15, 16). High virulence of hv-KP is associated mainly with enhanced capsule production, that can be triggered by a regulator of the mucoid phenotype (rmpA) gene and mucoviscosity-associated gene A (magA) (7, 17). In addition to c-KP and hv-KP, in recent years the third type of K. pneumoniae was detected, which characterized by a combination of antibiotic resistance and hypervirulence (16, 18, 19). The prevalence of antibiotic resistance in hv-KP isolates is rare compared with the high prevalence of antibiotic resistant c-KP isolates (20). A report in 2016 showed that in China 12.6% of hv-KP isolates from several invasive infections produced ESBL (21). The role of virulent strains of K. pneumoniae (including hv-KP) in neonatal infections is unknown. We hypothesized that virulent determinants of K. pneumoniae might affect clinical features and outcomes on neonatal infection. We assume that virulent strains of K. pneumoniae in neonates can lead to the development of the most severe forms of neonatal infection (purulent meningitis and sepsis with one or more foci of infection). C-KP in neonates is associated mainly with prematurity, the immaturity of the immune system, prolonged staying in the intensive care unit (ICU), mechanical ventilation, using intravenous or urinary catheters (1). Therefore, the main clinical manifestations of neonatal infection caused by c-KP can be pneumonia, urinary tract infection, and septicemia.
The aim of the study was the assessment of the impact of virulence factors and antibiotic resistance of K. pneumoniae strains on clinical features and outcomes of neonatal infection.
This study was conducted over a period of 18 months between May 2015 and October 2016. Two groups of neonates were enrolled. The first group consisted of 10 neonates with culture-proven sepsis, caused by K. pneumoniae. Neonates with sepsis were admitted to the Neonatal Intensive Care Unit (NICU) of the City Children's Clinical Hospital N1 in Kazan. During this period, the diagnosis of culture proven sepsis was established for 28 neonates admitted in NICU of this hospital. In 14 cases (50%), neonatal sepsis was caused by Staphylococcus spp., in 13 cases (46%)—by K. pneumoniae, and one case was due to Candida spp. Seven neonates have died. Most of them (six neonates, 86%) were neonates with sepsis caused by K. pneumoniae. In accordance with the Report of the Expert Meeting on Neonatal and Pediatric Sepsis (8 June 2010, EMA, London) (22), sepsis was defined as the presence of at least two clinical symptoms and at least two laboratory signs in presence of or as a result of a suspected or proven infection (positive blood culture). The clinical symptoms are (1) body temperature instability; (2) cardiovascular instability; (3) skin and subcutaneous lesions such as petechial rash or sclerema; (4) apnoea or increased oxygen requirement, or requirement for ventilation support; (5) feeding intolerance or abdominal distension; and (6) irritability, lethargy, or hypotonia. Its laboratory signs are (1) a white blood cell (WBC) count of <4 or >20 × 109 cells/L; (2) an immature to total neutrophil ratio (I/T) of >0.2; (3) a platelet count of <100 × 109/L; (4) C-reactive protein (CRP) levels of >15 mg/dL; (5) blood glucose values of >180 mg/dL or hypoglycemia (<40 mg/dL) confirmed at least twice; and (6) metabolic acidosis as characterized by a base excess (BE) of ≤10 mmol/L. Blood cultures of all 10 neonates included in the study were positive for K. pneumoniae. The second group (comparison group) consisted of 10 neonates with urinary tract infection (UTI). The criteria for inclusion of neonates in this group were leukocyturia (>10 leukocytes/mL) and bacteriuria (>105 colony-forming units/mL). Four out of ten infants had a fever and were admitted to the hospital, another six were outpatient infants and had no any clinical symptoms. From the urine of all infants was isolated K. pneumoniae.
We studied the antibiotic susceptibility of K. pneumoniae, its ability to produce ESBL, and its virulence factors including rmpA, aerobactin, and colibactin genes. A distinctive feature of hv-KP is its hypermucoviscous phenotype.
The Ethics Committee of the City children's hospital approved this study, the written informed consent obtained from the parents following the principles authorized under this protocol (the Federal Law N323-FL dated on 21.11. 2011 “On Protection of Health of the Citizens in the Russian Federation”).
Serum CRP levels were evaluated with the use of the Randox Full Range CRP immunoturbidimetry assay (Randox Laboratories, Crumlin, Northern Ireland, UK), according to the manufacturer's recommendations.
Blood samples: 1 ml of blood was collected with a sterile syringe and mixed with 20 ml of a Brain-Heart Infusion broth (Conda Pronadisa, Spain). The mixture was incubated at 37°C for 7 days, streaked onto the surface of blood agar and MacConkey agar (Oxoid, UK) and incubated at 37°C for 24 h (23). Urine samples were collected in sterile containers. A loopful of the urine samples were streaked onto the surface of blood agar and MacConkey agar (Oxoid, UK) and incubated at 37°C for 24 h. All isolates were identified according to morphological and biochemical tests (Gram stain, capsule stain, motility test, indole production test, urease production test, Methyl Red test, Voges-Proskauer test) (24) and confirmed by matrix-assisted laser desorption-ionization time-of-flight mass spectrometry (Microflex, Bruker Daltonics, Bremen, Germany).
The antibiotic susceptibility of K. pneumoniae isolates was determined by the Kirby–Bauer disk diffusion method according to Clinical and Laboratory Standards Institute guidelines (CLSI) (25). Suspension of each K. pneumoniae isolate was spread by sterile glass rods on the surface of Mueller Hinton agar (Oxoid, UK). Then antibiotic discs (BioRad, France) were placed onto the surface of the inoculated Mueller Hinton agar plate. The plate was then incubated at 35°C for 18 h. Antimicrobial susceptibility was determined by measuring the diameter of the inhibition zone according to CLSI (25).
The K. pneumoniae isolates were evaluated for ESBLs by using a double-disk method according to CLSI (25). Amoxicillin/clavulanate discs were placed in the center of Mueller Hinton agar plate (Oxoid, UK). The disc of ceftazidime and cefotaxime were placed at the distance of 20 mm from the amoxicillin/clavulanic acid disc. The plates were incubated aerobically at 35°C for 18 h before the zone size recorded. A positive result was indicated when the inhibition zones around any of the cephalosporin discs were augmented in the direction of the disc containing clavulanic acid.
Single colonies, cultured on blood agar and MacConkey agar plates (Oxoid, UK), were obtained and studied for their ability to form viscous strings. The hypermucoviscousity was determined by the formation of viscous strings of >5 mm length (14). Isolation of K. pneumoniae strains and microbiological studies of obtained isolates were performed in the bacteriological laboratory of the Republican Clinical Infectious Diseases Hospital.
Bacterial colonies were grown on the surface of blood agar and MacConkey agar, collected and suspended in 50–100 μl of sterile distilled water. Total DNA was extracted from suspended cells with the use of an extraction kit (“Litech,” Russia) according to the manufacturer's recommendations.
DNA samples were analyzed by using polymerase chain reaction (PCR). Primer sequences used to amplify fragments of the target genes are listed in Table 1. The reaction mix composition and PCR programs for amplification can be provided upon request. The amplicones were separated in 1.5% agarose gel and purified with the use of the GeneJET Gel Extraction Kit (Thermo Scientific, USA) according to the manufacturer's recommendations. The PCR-products were sequenced on a 3730 DNA Analyzer (Life technologies, USA) and compared with K. pneumoniae str. Kp52.145 plasmid II and chromosomal genome sequences from GenBank (Accession numbers FO834905.1 and FO834906.1, respectively) to confirm the target genes.
Out of 10 neonates with NS 5 were the term and 5 were preterm. Three preterm neonates were born earlier than 32 weeks of gestation, and another two ones—in the period from 32 to 36 weeks of gestation (Table 2). Two neonates were born with extremely low body weight (ELBW). Sepsis developed in five neonates on the first week of life, in three ones on the 2nd and 3rd weeks, respectively, and in two neonates on the 4th week. Sepsis was diagnosed in 5 neonates during their staying in a maternity ward, and in three ones (neonates with the ELBW)—in the NICU of the Children's Clinical Hospital. Two neonates fell ill at home.
NS clinically manifested in purulent meningitis (n = 3), enterocolitis (n = 3), and pneumonia plus enterocolitis (n = 2). In two cases the disease was associated with septicemia. Three mothers of neonates with sepsis had colpitis during pregnancy. The patients received a comprehensive treatment, including antibiotics, infusion therapy, mechanical ventilation, and intravenous immunoglobulins. Three cases of neonatal sepsis were fatal; two neonates had purulent meningitis and one necrotizing enterocolitis. Other seven neonates with sepsis successfully recovered.
Out of 10 neonates with UTI eight babies were born term and two—preterm. Both preterm neonates were born at 35 weeks of gestation. UTI developed in four neonates on the 3rd week of life, in six neonates on the 4th week. Only four out of ten infants had clinical manifestations, the main of which were fever and irritability. In the remaining six infants, UTI was asymptomatic. Two mothers of neonates with UTI had colpitis during pregnancy, in one case—pyelonephritis. All neonates received antibiotic therapy based on the findings of microbiological sensitivity tests.
In neonates with NS, all K. pneumoniae isolates were resistant to ampicillin, and six of them being resistant to all aminopenicillins (including protected ones), gentamycin and third-generation cephalosporins. At the same time, all K. pneumoniae isolates were sensitive to meropenem, amikacin, and ciprofloxacin (Table 3). Six isolates of K. pneumoniae produced ESBLs. In neonates with UTI, all K. pneumoniae isolates also were resistant to ampicillins. Six of them were resistant to gentamycin, four isolates—to protected aminopenicillins and third generation cephalosporins. All K. pneumoniae isolates were sensitive to meropenem, amikacin, and ciprofloxacin. Test for ESBL production was positive for 4 isolates of K. pneumoniae.
In the neonatal sepsis group, the rmpA gene (the main virulence factor of K. pneumoniae) was detected in 4 cases (40%). The presence of the rmpA gene in K. pneumoniae was associated with the development of purulent meningitis (3 cases) and enterocolitis (1 case). Only full-term infants had meningitis. Two of four children with the rmpA gene in K. pneumoniae isolates died. The “string test” was positive if the colony of K. pneumoniae showed the presence of the rmpA gene only. Aerobactin and colibactin genes were detected in two K. pneumoniae isolates. Both aerobactin-producing isolates were positive for iro D and iro N. One of two colibactin-producing isolates was positive for clb A and clb B, another was positive for clb B only. Aerobactin and colibactin genes were detected only in K. pneumoniae isolates, taken from the neonates with purulent meningitis who had an unfavorable outcome (Table 4). The production of aerobactin and colibactin directly correlated with the presence of the rmpA gene. The aerobactin and colibactin genes were not detected in two rmpA-positive K.pneumoniae isolates. In one case it was an infant with meningitis, who had a favorable outcome, in the other case—an infant with enterocolitis.
In neonates with UTI the rmpA gene, aerobactin, and colibactin genes were detected in 3 (30%) K. pneumoniae isolates. All aerobactin-producing isolates were positive for iro D and iro N. All colibactin-producing isolates were positive for clb A and clb B. The aerobactin and colibactin genes were not detected in rmpA-negaitive K. pneumoniae isolates.
The study of the impact of virulence factors of K. pneumoniae isolates on the clinical manifestations of neonatal infections revealed a high probability of the development of purulent meningitis (OR 9; CI 0.7–113) with an unfavorable outcome (OR 4.8; CI 0.3–65.7), but these differences were not statistically significant (Table 5).
Table 5. Comparison of clinical and laboratory data from neonates with and without virulent determinants.
Numerous studies have shown that antibiotic resistance and virulence of microorganisms are significant risk factors for NS (30, 31). Antibiotic resistance of bacteria is the main cause of non-effective therapy of nosocomial infections and sepsis. The antibiotic resistance of K. pneumoniae strains is associated mainly with the production of ESBL. In 2017 the World Health Organization included ESBL-producing K. pneumoniae in the list of the most dangerous superbugs along with Acinetobacter baumani and Pseudomonas aeruguinosa (32). Neonates are most vulnerable to infection. The predisposing factors to Gram-negative sepsis in newborns are associated with prematurity and low birth weight, as well as a prolonged stay in ICU (33, 34). In our study, in the neonatal sepsis group, five neonates were preterm, and two of them were born with extremely low body weight (ELBW). Six blood isolates of K. pneumoniae strains (60%) produced ESBLs. In neonates with UTI, K. pneumoniae isolates produced ESBL in 40% of cases. Contamination of neonates with ESBL producing K. pneumoniae strains could occur in a maternity ward, or during prolonged staying in ICU. Testing for antibiotic resistance showed that all ESBL producing K. pneumoniae isolates (from blood and urine) were resistant to all aminopicillins and third-generation cephalosporins. At the same time, all ESBL producing K. pneumoniae isolates were sensitive to meropenem, amikacin, and ciprofloxacin. Obviously, that meropenem should be considered as priority antibiotic therapy in cases of neonatal infections caused by ESBL-producing strains of K. pneumoniae. All blood and urine K. pneumoniae isolate, not producing ESBL, were sensitive to protected aminopenicillins, third-generation cephalosporins, meropenem, amikacin, and ciprofloxacin. Therefore, in cases of neonatal infections caused by ESBL-not producing strains of K. pneumoniae, protected aminopenicillins and cephalosporins III should be used as the first line of antibiotic therapy. The early onset of antibiotic therapy is known to play a crucial role in the efficacy of sepsis therapy since its delay increases the risk of death (35). However, clinical symptoms and laboratory signs of NS are not specific, and its early identification is a rather difficult task. The involvement of gastrointestinal tract may indirectly indicate the development of infection caused by Gram-negative bacteria, as the intestine is the main site of their colonization. Among laboratory signs of sepsis associated with gram-negative bacteria, a number of researchers designate common development of thrombocytopenia (36). In our study, all 10 neonates with sepsis had thrombocytopenia, which was severe (<50 × 109/L) in 8 ones. Five infants had enterocolitis. The most severe forms of sepsis in the infants studied, as well as its adverse outcomes, were associated with meningitis. It is worthy to note that neonatal meningitis occurred only in full-term infants, two of them were discharged home from maternity wards and fell ill on the second week of life. When analyzing this situation, we tend to associate the development of sepsis in neonates with their staying in maternity wards, because most K. pneumoniae isolates were ESBL-producers. This also can be supported by the fact that sepsis caused by K. pneumoniae occurred in six neonates who were in the same hospital during the same period. Unfortunately, neither medical staff of the maternity houses nor the mothers of these neonates were examined for K. pneumoniae carriage, so the above assumption cannot be confirmed. Despite the well-known role of ESBL-producing K. pneumoniae strains in the development of late onset of NS, it is also evident that the virulence factors of K. pneumoniae play an essential role in the development of the most severe forms of NS. The factors that are implicated in the virulence of K. pneumoniae strains include capsular polysaccharides, lipopolysaccharides, fimbrial adhesins, and siderophores (37, 38). The hypervirulent phenotype of K. pneumoniae is associated with the presence of the rmpA gene (14). Hv-KP causes the most severe, invasive forms of infection in previously healthy adults (liver abscesses, meningitis, and endophthalmitis) (12–14). Unlike cv-KP, hv-KP strains are more resistant to the complement and phagocytosis (38, 39). The mechanisms providing such stability are diverse and include protection against opsonization and phagocytosis by macrophages and neutrophils due to the presence of a thick capsule in K. pneumoniae and the blockade of TLR, in particular, TLR4, which inhibits the expression of interleukin-8 (38). Commonly, high virulence is typical for K. pneumoniae strains that cause community-acquired infections, and these microorganisms are usually sensitive to the majority of external antimicrobial factors. At the same time, recent studies demonstrate a new trend: hv-KP have acquired the main property of nosocomial microorganisms—antibacterial resistance. The impact of the hv-KP strain on the clinical features of neonatal infection is unknown. In our study, the rmpA gene was found in K. pneumoniae isolates in both groups of neonates, with NS and UTI. The detection of the rmpA gene in K. pneumoniae strains isolated from neonates with sepsis in three of four cases was associated with purulent meningitis. The detection of the rmpA gene in neonates with UTI was unexpected for us. Obviously, that other virulence factors, in particular, lipopolysaccharide (LPS) and siderophores, are necessary to ensure the virulence of K. pneumoniae. LPS protects against humoral defenses and activate immune system (20). Lipid A of LPS is a ligand for TLR4 (20). O-antigen of LPS protects against complement (20). Strains of K. pneumoniae that contain a full-length O-antigen (“smooth LPS”) are resistant to complement-mediating killing, while those with truncated or absent of O chains (“rough LPS”) are susceptible to complement-mediating killing, even in the presence of capsule (40). The lack of O-antigen K. pneumoniae less virulent. Detection of the rmpA gene in K. pneumoniae isolates in neonates with UTI indicates that the prevalence of virulent K. pneumoniae strains may be higher than we think. The prevalence of hv-KP in Russian Federation is unknown. The prevalence of hv-KP is very high in Asia (up to 90%) (41), in other regions of the world, it is less. In Spain and Canada, the prevalence of hv-KP was estimated at 5.4 and 8.2%, respectively (42, 43). In our study, two cases were caused by ESBL-producing K. pneumoniae isolates which were containing the rmpA gene. They were infants with meningitis, and both cases were fatal. In the group of infants with UTI, the rmpA gene was detected only in K. pneumoniae isolates not producing ESBL. Despite the small number of neonates included in this study, it is obvious that the acquisition of multidrug resistance by virulent strains of K. pneumoniae can worsen the course and prognosis of the disease. Siderophores—aerobactin, enterobactin, yersiniabactin, and colibactin are most important virulent factors that promote K. pneumoniae growth and survival in an infected host (7, 38). Enterobactin expression is ubiquitous among c-KP and hv-KP (44, 45). But aerobactin is expressed by 6% of c-KP and 93–100% of hv-KP isolates (44, 45). Siderophores are small, high-affinity iron chelating molecules secreted by a wide variety of microorganisms that are critical for virulence in many Gram-negative bacteria (7, 20). Iron is known to be essential for bacterial growth and replication (46). Access to iron molecules is an important factor that provides invasive properties of K. pneumoniae in an infection in immunocompetent people (46). Hv-KP strains produce siderophore molecules in a larger number and in their more active form than c-KP do (46). It has been shown that the development of invasive forms of the disease is associated with the production of several siderophores by K. pneumoniae strains (47). In our study, K. pneumoniae strains producing siderophores were detected in two neonates with sepsis and in three neonates with UTI. In all cases, aerobactin and colibactin genes were detected only in rmpA-positive K. pneumoniae isolates. However, fatal outcomes were noted only in cases caused by virulent strains of KP. In spite of the association between development of purulent meningitis and an unfavorable outcome in neonates infected mainly by virulent KP strains, we were not able to confirm this situation by statistical methods. Probably, this is due to a small number of newborns with purulent meningitis, which rarely occurs in this age group. We could not confirm the initial hypothesis that the development only the most severe forms of neonatal infection (purulent meningitis) is associated with virulent KP strains. UTI also can be caused by virulent strains of K. pneumoniae. Obviously, the role of virulent strains of K. pneumoniae will be increased in the future.
The prevalence of virulent strains of K. pneumoniae among neonates with sepsis and other neonatal infection is higher than we think. The risk of development of severe forms of neonatal infection can be associated with virulence factors. The most severe forms of neonatal sepsis with an unfavorable outcome in our study were due to virulent strains of K. pneumoniae.
KK, VA, and AR: design of the study, writing the manuscript. YD: DNA extraction of K. pneumoniae strains with subsequent genotyping by PCR method to determine virulence factors. DS: collection of clinical data. SL: collection and interpretation of clinical data. NS: isolation of K. pneumoniae colonies, determination of their antibiotic resistance and ability to produce of extended spectrum β-lactamase.
This study was supported by the Russian Government Program of Competitive Growth of Kazan Federal University. AR was supported by state assignment 20.5175.2017/6.7 of the Ministry of Education and Science of Russian Federation.
The authors declare that the research was conducted in the absence of any commercial or financial relationships that could be construed as a potential conflict of interest.
This study was supported by the Russian Government Program of Competitive Growth of Kazan Federal University. AR was supported by state assignment 20.5175.2017/6.7 of the Ministry of Education and Science of Russian Federation.
1. Camacho-Gonzales A, Spearman PW, Stoll BJ. Neonatal infectious diseases: evaluation of neonatal sepsis. Pediatr Clin North A (2013) 60:367–89. doi: 10.1016/j.pcl.2012.12.003
2. Verma P, Berwal PK, Nagaraj N, Swami S, Jivaji P, Narayan S. Neonatal sepsis: epidemiology, clinical spectrum, recent antimicrobial agents and their antibiotic susceptibility pattern. Int J Contemp Pediatr. (2015) 2:176–80. doi: 10.18203/2349-3291.ijcp20150523
3. Stoll BJ, Hansen N, Fanaroff AA, Wright LL, Carlo WA, Ehrenkranz RA, et al. Late-onset sepsis in very low birth weight neonates: the experience of the NICHD Neonatal Research Network. Pediatrics (2002) 110:285–91. doi: 10.1542/peds.110.2.285
4. Zaidi AK, Thaver D, Ali SA, Khan TA. Pathogens associated with sepsis in newborns and young infants in developing countries. Pediatr Infect Dis J. (2009) 28(Suppl. 1):S10–8. doi: 10.1097/INF.0b013e3181958769
5. Vergnano S, Menson E, Kennea N, Embleton N, Russell AB, Watts T, et al. Neonatal infections in England: the NeonIN surveillance network. Arch Dis Child Fetal Neonatal Ed. (2011) 96:F9–14. doi: 10.1136/adc.2009.178798
6. Zea-Vera A, Ochoa TJ. Challenges in the diagnosis and management of neonatal sepsis. J Trop Pediatr. (2015) 61:1–13. doi: 10.1093/tropej/fmu079
7. Shon AS, Bajwa RP, Russo TA. Hypervirulent (hypermucoviscous) Klebsiella pneumoniae: a new and dangerous breed. Virulence (2013) 4:107–18. doi: 10.4161/viru.22718
8. Podschun R, Ullmann U. Klebsiella spp. as nosocomial pathogens: epidemiology, taxonomy, typing methods, and pathogenicity factors. Clin Microbiol Rev. (1998) 4:589–603.
9. Haller S, Eller C, Hermes J, Kaase M, Steglich M, Radonic A, et al. What caused the outbreak of ESBL-producing Klebsiella pneumoniae in a neonatal intensive care unit, Germany 2009 to 2012? Reconstucting transmission with epidemiological analysis and whole-genome sequencing. BMJ (2015) 5:e007397. doi: 10.1136/bmjopen-2014-007397
10. Khaertynov KhS, Anohin VA, Nikolaeva IV, Semenova DR, Lyubin SA, Agapova IV. Neonatal sepsis caused by Klebsiella. Med News North Caucasus (2016) 11:82–6. doi: 10.14300/mnnc.2016.11004
11. Gelband H, Miller-Petrie M, Pant S, Gandra S, Levinson J, Barter D, et al. The State of the World's Antibiotics. Washington, DC: Center for Disease Dynamics, Economics, Policy (2015).
12. Liu YC, Cheng DL, Lin CL. Klebsiella pneumoniae liver abscess associated with septic endophthalmitis. Arch Intern Med. (1986) 146:1913–6.
13. Cheng DL, Liu YC, Yen MY, Liu CY, Wang RS. Septic metastatic lesions of the pyogenic liver abscess. Their association with Klebsiella pneumoniae bacteremia in diabetic patients. Arch Intern Med. (1991) 151:1557–9. doi: 10.1001/archinte.1991.00400080059010
14. Wang JH, Liu YC, Lee SS, Yen MY, Chen YS, Wang JH, et al. Primary liver abscess due to Klebsiella pneumoniae in Taiwan. Clin Infect Dis. (1998) 26:1434–8.
15. Decré D, Verdet C, Emirian A, Le Gourrierec T, Petit JC, Offenstadt G, et al. Emerging severe and fatal infections due to Klebsiella pneumoniae in two university hospitals in France. J Clin Microbiol. (2011) 49:3012–304. doi: 10.1128/JCM.00676-11
16. Bialek-Davenet S, Criscuolo A, Ailloud F, Passet V, Jones L, Delannoy-Vieillard AS, et al. Genomic definition of hypervirulent and multidrug-resistant Klebsiella pneumoniae clonal groups. Emerg Infect Dis. (2014) 20:1812–20. doi: 10.3201/eid2011.14020622
17. Yu WL, Ko WC, Cheng KC, Lee HC, Ke DS, Lee CC, et al. Association between rmpA and magA genes and clinical syndromes caused by Klebsiella pneumoniae in Taiwan. Clin Inect Dis. (2006) 42:1351–8. doi: 10.1086/503420
18. Zhang R, Lin D, Chan EW, Gu D, Chen GX, Chen S. Emergence of carbapenem-resistant serotype K1 hypervirulent Klebsiella pneumoniae strains in China. Antimicrob Agents Chemother. (2015) 60:709–11. doi: 10.1128/AAC.02173-15
19. Surgers L, Boyd A, Girard PM, Arlet G, Decré D. ESBL-producing strain of hypervirulent Klebsiella pneumoniae K2, France. Emerg Infect Dis. (2016) 22:1687–8. doi: 10.3201/eid2209.160681
20. Paczosa MK, Mecsas J. Klebsiella pneumoniae: going on the offense with a strong defense. Microbiol Mol Biol Rev. (2016) 80:629–61. doi: 10.1128/MMBR.00078-15
21. Zhang Y, Zhao C, Wang Q, Wang X, Chen H, Li H, et al. High prevalence of hypervirulent Klebsiella pneumoniae infection in china: geographic distribution, clinical characteristics, and antimicrobial resistance. Antimicrob Agents Chemother. (2016) 60:6115–20. doi: 10.1128/AAC.01127-16
22. Rossi P, Botgros R, Shane Tibby (UK), Nadel S, Manzoni P, Arenas-Lopez S. Report on the Expert Meeting on Neonatal and Paediatric Sepsis. London: European Medicines Agency (2010). Available online at: http://www.ema.europa.eu/docs/en_GB/document_library/Report/2010/12/WC500100199.pdf
23. Collee JG, Mackie TJ, McCartney JE. Practical Medical Microbiology. New York, NY: Churchill Livingstone (1996).
24. MacFaddin JF. Biochemical Tests for Identification of Medical Bacteria. 3rd Edn. Baltimore, MD: The Williams and Wilkins (2000).
25. Patel JB, Cockerill FR, Bradford PA, Eliopoulos GM, Hindler JA, Jenkins SG, et al. Performance Standards for Antimicrobial Susceptibility Testing. Wayne: Clinical and Laboratory Standards Institute (2015).
26. Luo Y, Wang Y, Ye L, Yang J. Molecular epidemiology and virulence factors of pyogenic liver abscess causing Klebsiella pneumoniae in China. Clin Microbiol Infect. (2014) 20:818–24. doi: 10.1111/1469-0691.12664
27. Compain F, Babosan A, Brisse S, Genel N, Audo J, Ailloud F, et al. Multiplex PCR for the detection of seven virulence factors and K1/K2 capsular serotypes of Klebsiella pneumoniae. J Clin Microbiol. (2014) 52:4377–80. doi: 10.1128/JCM.02316-14
28. Yu WL, Ko WC, Cheng KC, Lee CC, Lai CC, Chuang YC. Comparison of prevalence of virulence factors for Klebsiella pneumoniae liver abscesses between isolates with capsular K1/K2 and non-K1/K2 serotypes. Diagn Microbiol Infect Dis. (2008) 62:1–6. doi: 10.1016/j.diagmicrobio.2008.04.007
29. Lai YC, Lin AC, Chiang MK, Dai YH, Hsu CC, Lu MC, et al. Genotoxic Klebsiella pneumoniae in Taiwan. PLoS ONE. (2014) 9:e96292. doi: 10.1371/journal.pone.0096292
30. Centers for Disease Control and Prevention. Vital signs: carbapenem resistant Enterobacteriaceae. Morb Mortal Wkly Rep. (2013) 62:165–70.
31. Al-Hasan MN, Huskins WC, Lahr BD, Eckel-Passow JE, Baddour LM. Epidemiology and outcome of Gram-negative bloodstream infection in children: a population-based study. Epidemiol Infect. (2011) 139:791–6. doi: 10.1017/S0950268810001640
33. Samuelsson A, Isaksson B, Hanberger H, Olhager E. Late-onset neonatal sepsis, risk factors, and interventions: an analysis of recurrent outbreaks of Serratia marcescens, 2006-2011. J Hosp Infect. (2014) 86:57–63. doi: 10.1016/j.jhin.2013.09.017
34. Cortese F, Scicchitano P, Gesualdo M, Filaninno A, De Giorgi E, Schettini F, et al. Early and late infections in newborns: where do we stand? A review. Pediatr Neonatol. (2016) 57:265–73. doi: 10.1016/j.pedneo.2015.09.007
35. Rhodes A, Evans LE, Alhazzani W, Levy MM, Antonelli M, Ferrer R, et al. Surviving sepsis campaign: international guidelines for management of sepsis and septic shock: 2016. Intens Care Med. (2017) 43:304–77. doi: 10.1007/s00134-017-4683-6
36. Arif SH, Ahmad I, Ali SM, Khan HM. Thrombocytopenia and bacterial sepsis in neonates. Indian J Hematol Blood Transfus. (2012) 28:147–51. doi: 10.1007/s12288-011-0118-7
37. Broberg CA, Palacios M, Miller VL. Klebsiella: a long way to go towards understanding this enigmatic jet-setter. F1000Prime Rep. (2014) 6:64: doi: 10.12703/P6–64
38. Li B, Zhao Y, Liu C, Zhou D. Molecular pathogenesis of Klebsiella pneumonia. Future Microbiol. (2014) 9:1071–81. doi: 10.2217/fmb.14.48
39. Doorduijn DJ, Rooijakkers SH, van Schaik W, Bardoel BW. Complement resistance mechanisms of Klebsiella pneumoniae. Immunobiology. (2016) 221:1102–9. doi: 10.1016/j.imbio.2016.06.014
40. Merino S, Camprubi S, Alberti S, Benedi VJ, Thomas JM. Mechanisms of Klebsiella pneumoniae resistance to complement-mediating killing. Infect Immunol. (1992) 60:2529–35.
41. Ye M, Tu J, Jiang J, Bi Y, You W, Zhang Y, et al. Clinical and genomic analysis of liver abscess-causing Klebsiella pneumoniae identifies new liver abscess-associated virulence genes. Front Cell Infect Microbiol. (2016) 6:165. doi: 10.3389/fcimb.2016.00165
42. Cubero M, Grau I, Tubau F, Pallarés R, Dominguez MA, Linares J, et al. Hypervirulent Klebsiella pneumoniae clones causing bacteraemia in adults in a teaching hospital in Barcelona, Spain (2007-2013). Clin Microbiol Infect. (2016) 22:154–60. doi: 10.1016/j.cmi.2015.09.025
43. Peirano G, Pitout JD, Laupland KB, Meatherall B, Gregson DB. Population-based surveillance for hypermucoviscosity Klebsiella pneumoniae causing community-acquired bacteremia in Calgary, Alberta. Can J Infect Dis Med Microbiol. (2013) 24:e61–4. doi: 10.1155/2013/828741
44. Podschun R, Sievers D, Fischer A, Ullmann U. Serotypes, hemagglutinins, siderophore synthesis, and serum resistance of Klebsiella isolates causing human urinary tract infections. J Infect Dis. (1993) 168:1415–21. doi: 10.1093/infdis/168.6.1415
45. El Fertas-Aissani R, Messai Y, Alouache S, Bakour R. Virulence profiles and antibiotic susceptibility patterns of Klebsiella pneumoniae strains isolated from different clinical specimens. Pathol Biol. (2013) 61:209–16. doi: 10.1016/j.patbio.2012.10.004
46. Holden VI, Breen P, Houle S, Dozois CM, Bachman MA. Klebsiella pneumoniae siderophores induce inflammation, bacterial dissemination, and HIF-1 stabilization during pneumonia. mBio (2016) 7:1397–16. doi: 10.1128/mBio.01397-16
Keywords: neonatal sepsis, Klebsiella pneumoniae, virulence, antibiotic resistance, extended-spectrum beta-lactamases
Citation: Khaertynov KS, Anokhin VA, Rizvanov AA, Davidyuk YN, Semyenova DR, Lubin SA and Skvortsova NN (2018) Virulence Factors and Antibiotic Resistance of Klebsiella pneumoniae Strains Isolated From Neonates With Sepsis. Front. Med. 5:225. doi: 10.3389/fmed.2018.00225
Received: 31 January 2018; Accepted: 24 July 2018;
Published: 14 August 2018.
Edited by:
Olivier Vandenberg, Free University of Brussels, BelgiumReviewed by:
Pedro Xavier-Elsas, Universidade Federal do Rio de Janeiro, BrazilCopyright © 2018 Khaertynov, Anokhin, Rizvanov, Davidyuk, Semyenova, Lubin and Skvortsova. This is an open-access article distributed under the terms of the Creative Commons Attribution License (CC BY). The use, distribution or reproduction in other forums is permitted, provided the original author(s) and the copyright owner(s) are credited and that the original publication in this journal is cited, in accordance with accepted academic practice. No use, distribution or reproduction is permitted which does not comply with these terms.
*Correspondence: Khalit S. Khaertynov, a2hhbGl0NjVAcmFtYmxlci5ydQ==
Disclaimer: All claims expressed in this article are solely those of the authors and do not necessarily represent those of their affiliated organizations, or those of the publisher, the editors and the reviewers. Any product that may be evaluated in this article or claim that may be made by its manufacturer is not guaranteed or endorsed by the publisher.
Research integrity at Frontiers
Learn more about the work of our research integrity team to safeguard the quality of each article we publish.