- Academic Unit of Reproductive and Developmental Medicine, University of Sheffield, Sheffield, United Kingdom
In addition to being a passage for sperm, menstruum, and the baby, the human vagina and its microbiota can influence conception, pregnancy, the mode and timing of delivery, and the risk of acquiring sexually transmitted infections. The physiological status of the vaginal milieu is important for the wellbeing of the host as well as for successful reproduction. High estrogen states, as seen during puberty and pregnancy, promote the preservation of a homeostatic (eubiotic) vaginal microenvironment by stimulating the maturation and proliferation of vaginal epithelial cells and the accumulation of glycogen. A glycogen-rich vaginal milieu is a haven for the proliferation of Lactobacilli facilitated by the production of lactic acid and decreased pH. Lactobacilli and their antimicrobial and anti-inflammatory products along with components of the epithelial mucosal barrier provide an effective first line defense against invading pathogens including bacterial vaginosis, aerobic vaginitis-associated bacteria, viruses, fungi and protozoa. An optimal host-microbial interaction is required for the maintenance of eubiosis and vaginal health. This review explores the composition, function and adaptive mechanisms of the vaginal microbiome in health and those disease states in which there is a breach in the host-microbial relationship. The potential impact of vaginal dysbiosis on reproduction is also outlined.
Introduction
The vaginal mucosal ecosystem is comprised of a stratified squamous non-keratinized epithelium overlaid by a mucosal layer continuously lubricated by cervicovaginal fluid (CVF). Together, these form a daunting physical and biochemical barrier against extraneous invading organisms. Apart from being an acidic medium containing an assortment of antimicrobial molecules including antibodies (IgA and IgG), mucins, β-defensins, secretory leucocyte protease inhibitor (SLPI), neutrophil gelatinase-associated lipocalin (NGAL), surfactant protein etc., CVF also facilitates the confinement of exogenous organisms (summarized in Figure 1) (1–3).
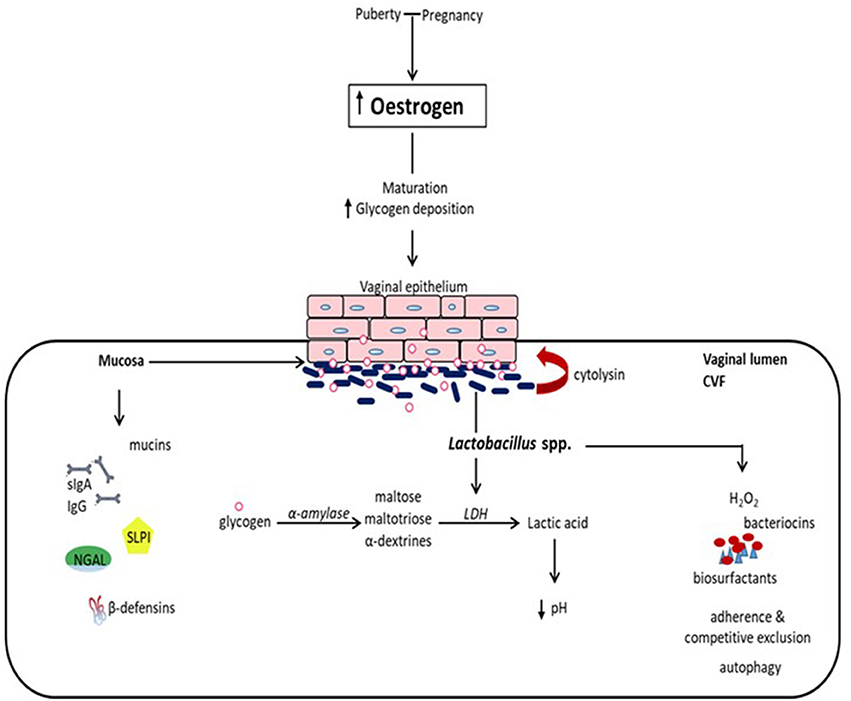
Figure 1. Eubiotic effect of estrogen and Lactobacillus species in the vaginal milieu. At puberty and during pregnancy, elevated levels of estrogen promote the maturation of and deposition of glycogen in vaginal epithelial cells. Glycogen from exfoliated and lysed epithelial cells is catabolized by α-amylase in the vaginal lumen to smaller polymers that are subsequently metabolized to lactic acid by Lactobacillus spp. Lactic acid and cytolysin produced by Lactobacilli stimulate the dissolution of epithelial cells by lysis and enhance the availability of glycogen. Lactic acid acidifies the vaginal milieu favoring the proliferation of Lactobacilli and inhibiting the growth of infection-associated organisms. This is reinforced by Lactobacilli through the production of hydrogen peroxide (H2O2), bacteriocins and biosurfactants, as well as the inhibition of the physical attachment of pathogens to the epithelium by competitive exclusion and the promotion of the engulfment and degradation of infected epithelial cells (autophagy). Additionally, there is concomitant production of mucins, immunoglobulins (secretory IgA and IgG), secretory leucocyte protease inhibitor (SLPI), neutrophil gelatinase-associated lipocalin (NGAL), and β-defensins, and other antimicrobial proteins, which all together provide a formidable first line of defense against infection. (CVF, cervicovaginal fluid; LDH, lactate dehydrogenase).
The vagina also harbors numerous microorganisms (the “microbiota”), that exist (in conjunction with their genes and products) in a regulated mutualistic relationship with the host (the “microbiome”) (4). Some of these microorganisms such as Lactobacillus species reinforce the defense against invasion and colonization by opportunistic pathogens. The composition of the vaginal microbiota/microbiome is dynamic and undergoes changes corresponding with hormonal fluctuations throughout the woman's reproductive life, i.e., from puberty to menopause, and during pregnancy (5).
The normal physiological vaginal microbiota was initially described in 1892 by Albert Döderlein as homogenous, consisting of only Gram-positive bacilli (Doderlein's bacilli) (6), believed to originate from the gut and currently known to be a part of the genus Lactobacillus (7). The evolution of this unique vaginal microbiome is supported by two evolutionary hypotheses: the “disease risk hypothesis” (8, 9), and the “obstetric protection hypothesis” (9), which suggest that the human vagina is selectively dominated by protective Lactobacillus species because humans are more susceptible to sexually transmitted diseases; and also at higher risk of pregnancy and parturition-associated microbial complications (8–10).
A number of protective Lactobacillus species dominates the healthy vaginal microbiota in most reproductive-age women. Recent advances in DNA sequencing techniques have revealed that the dominant Lactobacillus species in the vaginal microbiota include L. crispatus, L. gasseri, L. iners, and L. jensenii, while other anaerobes including Gardnerella, Atopobium, Mobiluncus, Prevotella, Streptococcus, Ureaplasma, Megasphaera etc. able to cause infections such as bacterial vaginosis (BV) are kept dormant by the protective action of lactobacilli. These high-resolution techniques have enabled the classification of the vaginal microbiota into five community state types (CSTs) with CSTI, II, III and V dominated by L. crispatus, L. gasseri, L. iners, and L. jensenii respectively, while CSTIV is dominated by mixed anaerobes similar to those found in BV (5, 11).
The prevalence of these organisms in the vaginal microbiota vary in different race/ethnic groups and biogeographical locations, with Blacks and Hispanics harboring more anaerobic bacterial species (CSTIV) and showing higher vaginal pH in the presence or absence of clinical infection (11, 12). Differences in prevalence are also related to lifestyle differences (11) and gene-environment interactions (12). Unlike other body viscera such as the gut, increased diversity of the vaginal microbiota is linked to increased susceptibility to disease and negative reproductive outcomes (5, 13).
In addition to epithelial cells and microbiota, the vagina also contains immune-related cells (such as neutrophils, macrophages, T and B cells, natural killer (NK) cells, etc.) (14) and specialized receptors, e.g., toll-like receptors (TLRs) and nod-like receptors (NLRs), with which it recognizes the presence of pathogenic microbial species (15). Microbial stimulation through ligands such as lipopolysaccharide (LPS) that stimulates TLR-4 triggers the release of cytokines and chemokines such IL-1β, IL-6, IL-8, and tumor necrosis factor-α (TNF-α). This release is activated and regulated via a nuclear factor-κB (NF-κB)-mitogen-activated protein kinase (MAPK) signaling pathway (15). Other immune factors including macrophages, NK cells, helper and cytotoxic T cells as wells as B-lymphocytes are subsequently recruited to mount appropriate immune responses. Such pathogen-stimulated inflammatory responses normally control infection but can in some instances breach the mucosal surface and facilitate transmission of some other infections such as HIV (1). Therefore, vaginal communities dominated by anaerobes are potentially associated with greater pro-inflammatory response than L. crispatus, L. gasseri or L. jensenii (3, 5). However, Lactobacilli and lactic acid via multiple mechanisms as discussed below promote antimicrobial defense without inducing immune-mediated inflammation unlike the pathogenic anaerobes (3).
The Effect of Estrogen on the Vaginal Ecosystem
The prepubertal vaginal microbiome is dominated by anaerobes, E. coli, diptheroids and coagulase-negative Staphylococci and significantly lesser glycogen (5). At puberty, the rising levels of estrogen promote the maturation, proliferation and accumulation of glycogen in the vaginal epithelial cells. Glycogen is catabolized by human α-amylase to maltose, maltotriose and α-dextrines, which are then metabolized to lactic acid by Lactobacillus species (Figure 1). This creates an acidic environment (pH, 3.5–4.5) conducive for the growth of Lactobacilli at the expense of other anaerobic bacterial species (1, 5). Lactobacilli dominance decreases as estrogen levels decline following menopause (16), and increases with vaginal estrogen replacement therapy.
The vaginal microbiota in normal pregnancy is predominated by Lactobacilli and is more stable than that in the non-pregnant state (12, 17, 18). This can be explained by the high level of estrogen during pregnancy resulting in increased vaginal glycogen deposition which enhances the proliferation of Lactobacilli-dominated vaginal microbiota (2). Also, studies have shown that menstruation significantly reversibly alters the vaginal microbial diversity, with about a 100-fold decrease in L. crispatus and increase in L. iners, G. vaginalis, P. bivia, and A. vaginae (19, 20). In essence, the normal acidic vaginal pH in reproductive-age women is driven by estrogen, glycogen, and Lactobacilli (1, 21–23).
An intriguing direct relationship between vaginal ostrogenization and candidiasis in postmenopausal women has also been reported (24). After menopause, estrogen-induced vaginal epithelial glycogen accumulation is associated with increased infection by Candida albicans that has glycogen as a major substrate. Contrastingly, in premenopausal women the activity of α-amylase that correlates with D- (but not L-) lactic acid and production of SLPI, NGAL, hyaluronidase-1 and matrix metalloproteinase (MMP)-8, was decreased in women infected with C. albicans (25). Increased glycogen availability secondary to exfoliation and lysis of glycogen-rich epithelial cells into the vaginal lumen by extracellular matrix degrading enzymes - hyaluronidase-1 and MMP-8, lactic acid and cytolysin could enhance α-amylase activity (25). This effect of vaginal ostrogenization, glycogen level and candidiasis in relation to menopausal status is likely to be of physiologic importance and necessitates further investigation.
Lactobacilli, sine qua non of Vaginal Homeostasis
Vaginal lactic acid is predominantly of bacterial origin (26). Under the influence of estrogen, the vaginal epithelium produces < 15% of L-lactic acid (26), while lactobacilli are the major source of both L- and D-lactic acid (27). Of the four most common vaginal Lactobacillus species, only L. iners lacks the ability to synthesize D-lactic acid and instead produces the L-isomer (3). Vaginal levels of α-amylase (produced by endocervical and fallopian tube cells) directly correlates with levels of D-lactic acid and other vaginal epithelial antimicrobial peptides such as SLPI and NGAL, but not with L-lactic acid (25). D-lactic acid is more protective against vaginal dysbiosis than L-lactic acid (27). Its levels are highest when L. crispatus is the dominant specie and lowest when L. iners, Gardnerella or Streptococcus predominate (27); and this partly accounts for the higher protection against urogenital infections (28, 29) and adverse reproductive outcomes (30) conferred by L. crispatus compared to L. iners. Lactic acid at physiological concentrations (e.g., 110 mM) acidifies vaginal secretions (to pH levels < 4), enhances the protective activities of H2O2 and bacteriocins (31, 32), and inhibits opportunistic infections such as G. vaginalis, Trichomonas vaginalis, Neisseria gonorrhoeae, Chlamydia trachomatis, herpes simplex virus (HSV), human papillomavirus (HPV), HIV etc. (33). Specifically, distinct from its bactericidal activity D-lactic acid inhibits Chlamydia infection through a pH-dependent effect on the vaginal epithelial cells and microenvironment (34). This conclusion arose from the significantly greater protection against chlamydia provided by L. crispatus, L. gasseri, and L. jensenii (34) that produce more D-lactic acid than L. iners that produces predominantly L-lactic acid (35). Also, D-lactic acid prevents upper genital tract infection by modulating the L-lactic acid-induced production of extracellular matrix metalloproteinase inducer (EMMPRIN) from vaginal epithelial cells, and inhibiting the production of MMP-8 (27).
The precise mechanism of the bactericidal activity of Lactobacillus is unclear but there is evidence that it is mediated through the protonated forms of both D- and L-lactic acid and not the lactate anion (36). At a pH value < pKa 3.9, the protonated or uncharged form of lactic acid predominates. Because it is a weak acid, 50% of lactic acid dissociates into lactate anion and H+ at a pH of 3.86. Lactic acid in its protonated form is membrane-permeant and unlike the lactate anion, does not require the proton-linked monocarboxylate transporters or the lactate-binding GPR81 receptors to enter cells (37, 38). Lactic acid preferentially lyses bacteria other than Lactobacillus species (23, 36); and causes bacterial cell death by acidifying the cytosol, disrupting intracellular function (39), increasing the permeability of the cell membrane to H2O2, diacetyl etc., thereby potentiating the antimicrobial effect of other substances (40). The reduced antimicrobial activity of lactic acid and increased risk of infection associated with unprotected sexual intercourse and menstruation could be attributed to the increase in vaginal pH after deposition of seminal fluid and flow of menstruum, which leads to formation of more lactate anion that has less antimicrobial and immunomodulatory activities (33, 36).
Lactic acid also performs some immunomodulatory actions on the genital tract mucosa and other cell types (41, 42). Lactic acid in its protonated form creates an anti-inflammatory state by stimulating the production of large amounts of the anti-inflammatory cytokine IL-1RA, and inhibiting the production of inflammatory cytokines (IL-6 and TNFα) and chemokines (IL-8, RANTES and macrophage inflammatory protein-3α, MIP-3α) in the presence of TLR-2,−3,−4 agonists (41). Lactic acid also inhibits nuclear translocation and activation of the transcription factor NF-κB in peripheral blood mononuclear cells and monocytes/macrophages (43, 44). It is worth noting that both D- and L-lactic acid exercise these anti-inflammatory effects that are enhanced by low pH < 3.86 by directly acting on cervicovaginal epithelial cells (41, 42).
Also, both D- and L-lactic acid can enhance vaginal epithelial cell survival by facilitating the repair of damaged DNA through the inhibition of histone deacetylase activity leading to increased acetylation of histones on the surface of DNA (45, 46). This epigenetic regulation of gene expression (45) permits the transcription of genes that were previously blocked and possibly promotes the secretion of components of the antimicrobial innate immune system, such as NGAL from vaginal epithelial cells, that selectively prevent the growth of bacteria other than lactobacilli (3, 47). These observations show great promise for the use of lactic acid-containing microbicides for therapeutic restoration of vaginal homeostasis and prevention of STIs including HIV.
Lactobacilli (apart from L. iners) produce hydrogen peroxide (H2O2), which inhibits the growth of catalase-negative anaerobic organisms by production of hydroxyl free radicals (48, 49). They can also bind to the surface of vaginal epithelium and competitively prevent other microbes from attaching to and infecting the cells. Lactobacilli can produce other antimicrobial peptides such as bacteriocins, bacteriocin-like substances and biosurfactants; and promote autophagy (engulfment and degradation) of intracellular bacteria, viruses and protozoa (Figure 1) (27). Hence, through these mechanisms, lactobacilli inhibit the growth of other potentially pathogenic endogenous vaginal bacteria and prevent the acquisition of exogenous bacteria. For these reasons a lactobacilli-dominated vaginal microbiota has been described as healthy and necessary for the overall wellbeing of the woman. However, it is important to note that about 25% of women ostensibly maintain healthy vaginal microbiota without lactobacilli dominance. These women have been found to harbor other lactic acid producers such as Atopobium, Megasphaera, Leptotrichia, Streptococcus, and Staphylococcus (50, 51).
In addition, the degree of protection conferred on the vaginal ecosystem is dependent on the predominant Lactobacillus specie. For example, an L. iners-dominated vaginal microbiota is usually associated with dysbiosis and appears less stable and more prone to transition. In contrast L. crispatus that produces both D- and L-lactic acid is associated with increased vaginal community stability (less likely to transit to dysbiosis) and health (19, 52, 53). L. iners exhibits pathogenic propensity via its pore-forming cholesterol-dependent cytolysin (CDC, inerolysin) (54, 55). It has a small genome and is unable to produce D-lactic acid and H2O2 required to promote eubiosis, unlike the other Lactobacillus species (3, 13). Also, we recently observed that preponderance of L. jensenii (which produces only the D-Lactic acid and lesser protective capacity compared to L. crispatus) (27), was associated with decrease in lactate, succinate and increased risk of premature delivery (56).
The Influence of Microbial Activity on the Vaginal Mucosal Barrier Function
The mucosal surface of the vagina is an immunological and physical barrier that prevents potential pathogens from coming in contact with vaginal epithelial cells. It contains glycosylated mucus proteins (sialoglycoproteins) such as mucin that provide a dense lubricated physical barrier, which inhibits epithelial cell-pathogen contact; and secretory immunoglobulin A (sIgA) and IgG that recognize and neutralize antigenic microbial products. Anaerobes associated with vaginal infection such as G. vaginalis secrete sialidase that degrades mucus by cleaving sialic acid from the glycoproteins. Sialic acid is taken up and neutralized by G. vaginalis (foraging) to further circumvent the host response. A significant depletion of mucus sialic acids is seen in BV-infected women compared to their healthy counterparts with Lactobacillus-dominated microbiota (57). Degradation and depletion of the components of the mucosal protective barrier permits ascending upper genital tract infection. In addition, like L. iners, G. vaginalis also produce a CDC called vaginolysin with which it forms pores in the vaginal epithelium, which further obliterates the protective barrier. Contrarily, the protective function of the mucus layer is enhanced by L. crispatus-dominated microbiota thereby hindering the penetration of pathogens like HIV, whereas L. iners-dominated microbiota facilitates the penetration of HIV. Therefore, alterations in the composition of the vaginal microbial community significantly affects the integrity of the protective mucosal surface layer (5).
Stress and Vaginal Health
The influence of stress on vaginal immunity has been the subject of much speculation. Immune response may be impaired by stress-related activation of the hypothalamic-pituitary adrenal (HPA) axis and secretion of corticotropin-releasing hormone (CRH) from the hypothalamus, which activates the release of cortisol from the adrenal cortex and noradrenaline from sympathetic nerve terminals (58). Cortisol inhibits the estrogen-associated vaginal epithelial maturation and accumulation of glycogen and consequently reduces lactobacilli dominance, while noradrenaline acts synergistically with immune mediators to potentiate the release of cytokines. The stress-induced increase in cortical hormones - cortisol and deoxycorticosterone - and the resultant decrease in lactobacilli abundance can worsen vulvovaginal symptoms of infection (59). Reduced vaginal epithelial glycogen decreases the production of lactic acid and loss of its anti-inflammatory activities. Hence, a dysbiotic vaginal flora is created characterized by a reduction or loss of lactobacillus dominance. Concomitant increase in noradrenaline potentiates the pro-inflammatory response and proliferation of pathogenic strict and facultative anaerobes as well as other STIs. Ultimately, stress exacerbates the susceptibility and severity of vaginal infection (3).
Aberrant Vaginal Microbiota (Dysbiosis)
The vaginal microbiota is a dynamic community of diverse bacterial species repeatedly subjected to both internal and external manipulative stimuli such as changes in sex hormone levels and stage of the menstrual cycle, sexual activity, antibiotic therapy and the use of oral contraceptives, vaginal douching, menopause, pregnancy, lactation, diabetes mellitus and stress. The composition of the vaginal microbiota is also determined by gene-environment interactions. Vaginal bacterial communities devoid of Lactobacillus dominance with higher pH and lower H2O2 have been observed to be normal in Black and Hispanic women (11, 52, 60, 61).
The most common vaginal infection in reproductive-aged women is bacterial vaginosis (BV). BV, with a prevalence rate of 5–70% (62), is characterized by a depletion of lactobacilli in favor of potentially pathogenic mixed anaerobes such as Gardnerella, Atopobium, Mobiluncus, Prevotella, Streptococcus, Mycoplasma, Ureaplasma, Dialister, Bacteroides etc. (63–65). It creates a more heterogeneous vaginal environment associated with decreased lactic acid levels, pH > 4.5, and high amounts of short chain fatty acids (SCFAs) such as acetate, butyrate, propionate and succinate produced by anaerobes. Although an overt inflammatory response is often not manifest, it has been associated with increased levels of immune mediators such as IL-1β, IL-2, IL-6, IL-8, IL-10, TNF-α, Interferon (IFN)-γ, RANTES etc. (1, 5), and decreased concentration of antimicrobial peptides like NGAL (47). BV is an enigmatic syndrome with unidentified etiology. Most BV-positive women are usually asymptomatic. However, symptoms could appear in the form of a non-itchy but irritating, creamy vaginal discharge with a fishy odor that may be more prominent after sexual intercourse and during menstruation.
The Amsel criteria are used to diagnose BV in most clinical settings. The criteria include the evaluation of vaginal acidity, the presence of vaginal discharge, the appearance of clue cells (desquamated vaginal epithelial cells studded with anaerobic bacteria), and a positive “whiff test” (a characteristic “fishy” odor perceived when 10% potassium hydroxide is added to a microscopic slide of vaginal discharge) (66). The most sensitive criteria are the vaginal pH (>4.5) and the detection of thin, homogenous, milky and adherent discharge (97%), when the criteria are assessed individually (67). However, the detection of discharge had a low specificity (26%) and positive predictive value (27%), while the criterion with the highest specificity was the presence of clue cells (86%). When combined together, the presence of at least three criteria significantly increases the likelihood of making an accurate diagnosis of BV, yielding a sensitivity and specificity of 97 and 90% respectively (67, 68).
In the research space, BV is commonly diagnosed with the Nugent scoring system (69). Although the diagnosis requires experienced laboratory staff to evaluate the slides, it is more objective and reliable and has a higher reproducibility and sensitivity compared to the Amsel criteria (70). It employs the Gram stain to microscopically identify a shift in vaginal microbiota from healthy lactobacilli dominance (Gram-positive rods) to an intermediate level of mixed Gram negative/variable microbiota (Gardnerella and Bacteroides), to absence of lactobacilli and predominance of Gram negative/variable rods or curved rods (Mobiluncus) (68, 69). A score of 0–3 is consistent with lactobacillus dominance and vaginal health, a score of 4–6 indicates an intermediate, mixed vaginal bacterial community, whereas >7 indicates BV infection. There is a good correlation between clinical features of BV and Gram stain scores (70).
BV is associated with increased risk of acquisition of STI such as N. gonorrhoea, C. trachomatis, T. vaginalis, HSV, HPV, and HIV, and other infections such as pelvic inflammatory disease, endometritis, chorioamnionitis, and amniotic fluid infection. In relation to pregnancy, BV appears to be associated with preterm premature rupture of membranes (PPROM), preterm labor (PTL) and preterm birth (PTB, i.e., delivery before 37 completed weeks of gestation) (62, 71–81).
Vaginal dysbiosis can also manifest as aerobic vaginitis (AV). It is an equally disruptive infection of the normal vaginal Lactobacillus-dominated microbiota but is characterized by overt inflammation, leukocyte and parabasal cell infiltration and proliferation of enteric aerobic bacterial organisms including Escherichia coli, Enteroccoci, Staphylococcus aureus, and group B Streptococcus (21, 82). It has been described as the aerobic equivalent of BV, due to decreased lactic acid concentration secondary to depleted Lactobacillus dominance. However, because anaerobes are absent, succinate concentration is low. AV has also been associated with STIs such as C. trachomatis, N. gonorrhoeae, and T. vaginalis. The clinical features of AV include: red vaginal mucosal inflammation, increased IL-1β and IL-6, vaginal pH > 6, itching and burning (pruritus), dyspareunia and yellowish sticky discharge devoid of fishy odor. AV is present in 2–25% of women and has been associated with severe adverse gynecological and obstetric outcomes including ascending genital infection/inflammation, PPROM, 83, PTL and PTB (84).
Implications of Abnormal Vaginal Microbiota for Human Pregnancy
The physiological status of the vaginal milieu is crucial not just to the general wellbeing of the host but also for conception and eventual success of pregnancy. The ability of Lactobacillus to preclude the invasion and colonization of the vaginal space by pathogens without triggering an overt inflammatory response is termed Tolerance and is particularly beneficial for reproduction (3). Apart from increasing the host's susceptibility to STIs and other gynecological conditions including cervical intraepithelial neoplasia and cervical cancer (85–87), a dysbiotic vaginal microenvironment with degraded Lactobacilli-mediated tolerance and anti-inflammatory mechanisms also influence the course and outcome of pregnancy. In essence, Lactobacillus spp. is invaluable to the preservation of a homeostatic vaginal milieu.
Conception and miscarriage
Diminished vaginal Lactobacillus dominance has been linked to failure of in vitro fertilization (IVF) and miscarriage (88). Decreased conception rates and high rates of early pregnancy loss due to reduced concentration of H2O2-producing Lactobacilli and BV infection have been observed. The percentage of women who deliver a live birth after undergoing embryo transfer is greatly influenced by the microbial composition of the cervicovaginal space. Increased pregnancy loss at 10–16 weeks in spontaneous pregnancies (88) and early pregnancy loss (before 6 weeks) after IVF (89, 90) are associated with the presence of a dysbiotic vaginal microbiota during gestation. There is a high (30–40%) prevalence of abnormal vaginal microbiota in women undergoing IVF (89, 91, 92). Low pregnancy rate (18%) and increased pregnancy loss (up to 66%) was recorded in women undergoing IVF when S. viridans was present in the transfer catheter tip (92), while high live birth rates (up to 70%) and low or no pregnancy loss were observed with the presence of H2O2-producing Lactobacillus in the vagina and transfer catheter tip. An even lower clinical pregnancy rate (9%) associated with abnormal vaginal microbiota was observed in a recent study (93). The role of infection-induced inflammation of the endometrial lining and gestational tissues (chorioamniotic membranes and placenta) was implicated (88). However, this plausible association requires further investigation to unravel its pathogenesis in order to improve conception and live birth rates.
Prematurity
Pregnancy is characterized by increased vaginal Lactobacillus dominance due to heightened estrogen-stimulated glycogen deposition and subsequent breakdown to lactic acid (Figure 1) (6, 12, 17). Also, tolerance mechanisms including lactic acid-induced autophagy of bacteria, viruses and protozoa, and lactobacilli inhibition of pro-inflammatory mediators are up-regulated during gestation (94, 95). Therefore, in the event of disturbance of this homeostatic vaginal host-microbial interaction during gestation (e.g., due to BV or AV), these protective mechanisms are diminished and invasion and colonization of the genital tract by pathogenic organisms can occur. These organisms can stimulate the degradation of the host mucosal epithelial barrier and cervical plug via the release of proteolytic enzymes such as elastase, mucinase, sialidase, protease, prolidase, and collagenases; ascend through the cervical canal and gain access into the uterine cavity and compromise the integrity of the fetal membranes (96–100). Ascending intrauterine infection earlier in gestation accounts for about 50% of spontaneous PTBs (2, 77), as does chorioamniotic-decidual inflammation (101). This occurs via the release of pro-inflammatory cytokines and chemokines (e.g., TNF-α and β, IL-1α and β, IL-2, IL-6, IL-8, RANTES etc.), prostaglandins (PGE2 and PGF2α), matrix metalloproteinases (MMPs- 1, 2, 3, 8, and 9), CRH, which trigger uterine contraction, membrane activation (i.e., separation of fetal membranes from the decidua) and cervical remodeling and effacement. An undesirable positive feed forward mechanism is established which culminates in PPROM, PTL and PTB (Figure 2) (49, 102). Central to these labor-associated processes (at both term and preterm) is the pleotropic transcription factor—NF-κB. It is activated by the recognition of microbial products (e.g., LPS) and non-microbial products by TLR/NLR, and regulates the expression of pro-inflammatory cytokines, prostaglandins and MMPs (15, 103).
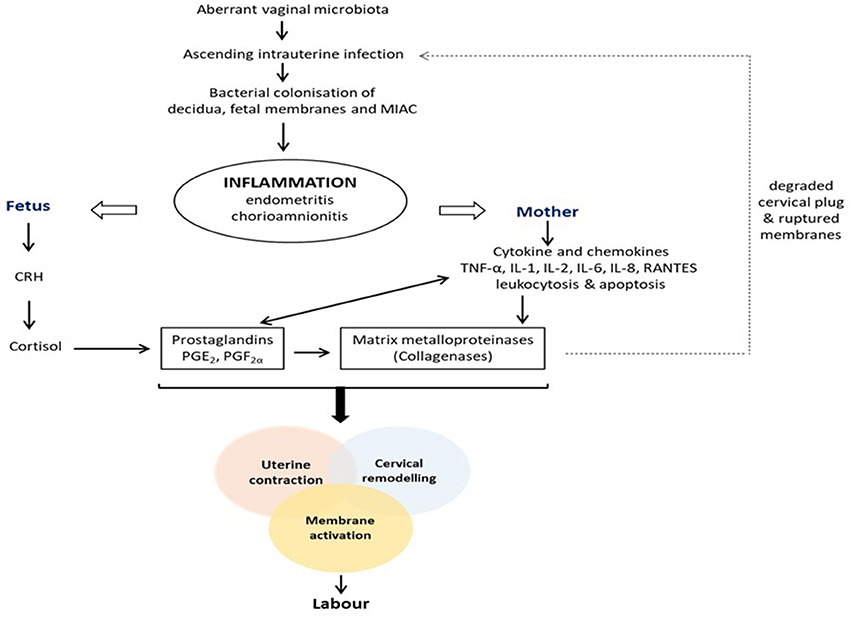
Figure 2. Pathogenesis of infection/inflammation-associated preterm labor and birth. Colonization of the female genital tract by pathogenic anaerobic bacteria due to an altered vaginal microbiota triggers a feed forward inflammatory response that ultimately stimulates the pathways to preterm labor and delivery, i.e., uterine contraction, cervical remodeling and membrane activation. CRH, corticotropin-releasing hormone; IL, interleukin; MIAC, microbial invasion of amniotic cavity; PGE2, prostaglandin E2; PGF2α, prostaglandin F2α; TNF, tumor necrosis factor.
Interestingly, D-lactic acid produced exclusively by Lactobacillus spp.—L. crispatus, L. jensenii, and L. gasseri—in the vagina, inhibits the synthesis of MMP-8 by modulating the action of EMMPRIN produced by vaginal epithelial cells (27). This prevents cervical tissue digestion and decreases the possibility of an ascending intrauterine infection and microbial invasion of the amniotic cavity (MIAC). Also, the protective function of the vaginal epithelial mucus layer is enhanced (5) and heightened autophagy activity (3) is observed when the vaginal microbiota is predominated by L. crispatus. An L. crispatus-dominated vaginal microbiome as seen in most healthy pregnancies is also inhibitory to E. coli colonization of the genital tract (104), and can prevent AV (83, 84). This is indicated to be via proteins produced by L. crispatus – S-layer, bacterial surface layer and cell separation proteins; and L. jensenii – adhesion exoprotein (105). In contrast, a low E. coli inhibitory activity was observed in women with L. iners dominance irrespective of pregnancy status (104), as this does not produce D-lactic acid. However, this observation still requires further investigation because in African women in whom the relative abundance of L. crispatus is low (11, 104), E. coli inhibitory activity is indicative of inflammation and increased susceptibility to HIV (106, 107). It is also worth noting that even in healthy women, there are ethnicity-dependent significant variations in the vaginal microbiome (108). Additionally, L. crispatus and L. jensenii inhibit the expression of pro-inflammatory mediators in the presence of TLR agonists (1). However, the mechanism through which this is achieved is unclear and the implications for reproductive outcomes may differ between these two species. Indeed, we recently observed an association between L. jensenii dominance, decreased lactate and succinate, and spontaneous PTB, while L. crispatus and L. gasseri were associated with elevated lactate and succinate, and delivery at term (56). We have also demonstrated a decrease in IL-8 and acetate levels (a marker of vaginal dysbiosis and imminent PTB) (1, 109–111) when L. crispatus is co-cultured with either G. vaginalis or M. curtisii (112).
A plausible explanation for these species-dependent varied outcomes could be the differential expression of lactic acid isomers by L. crispatus, L. jensenii, and L. iners in synergy with vaginal epithelial cells as described in earlier sections of this article. In addition to acidifying the vaginal milieu and inhibiting the growth of pathogenic anaerobes, both L- and D-lactic acid isomers (protonated form) exhibit anti-inflammatory properties by stimulating increased levels of IL-1RA without a concomitant increase in IL-1β, IL-6, IL-8, TNFα, RANTES, and MIP-3 α (41). They also attenuate the production of pro-inflammatory mediators stimulated by TLR agonist (41, 113, 114). Though both isomers exhibit similar virucidal and bactericidal activity against HIV-1 and BV-associated bacteria respectively (41, 42), D-lactic acid shows superior microbicidal capacity than the L-isomer (27, 115), and has been advocated as a prebiotic in the treatment of BV and prevention of PTB (2). The superior protective capacity of L. crispatus is attributable to its ability to produce both isomers of lactic acid. Taken together, the protective functions of L. crispatus and/or its products could be annexed through more comprehensive studies at different climates to develop therapeutic strategies for maintaining vaginal health and improving reproductive outcomes.
Conclusion
A healthy vaginal milieu requires an optimum balance of the host-microbial interaction despite multiple and sometimes inevitable internal and external stimulations it receives. Alteration of this homeostatic state, perhaps due to failure of the host adaptive responses results in dysbiosis with reproductive consequences if left unattended. These manifest as decreased conception rates especially with assisted reproductive technology, early pregnancy loss, and premature labor and delivery. Though dysbiotic conditions such as BV also increase the rate of PTB up to 7-fold, especially when diagnosed before 16 weeks of gestation (70), most BV-infected pregnant women do not deliver prematurely (116). Also, a considerable proportion of women with inflammation of the gestational tissues who deliver preterm do not have BV (100). There is a growing body of evidence suggesting that it is the relative quantities (abundance) of bacteria, rather than just their presence (64, 117), and the unique host immune response to the infectious stimuli, that are associated with increased risk of infection and PTB (64, 118). Host differences in distribution of Lactobacillus spp. innate and adaptive immunity, volume and composition of vaginal fluid, expression of epithelia cells surface ligands, as well as other human behaviors and practices (such as smoking, sexual intercourse, douching, contraceptive and antibiotics use etc.) have been implicated in the variations in the composition of the “normal” vaginal microbiota. Risk of infection and PTB is modified by variations in maternal/fetal genetic composition and epigenetics, which ultimately determines the intensity of immune response to altered vaginal microbiota during gestation (119–125). These discrepancies have created a huge burden on the development of effective preventive and therapeutic strategies to improved women's health. As inflammation may continue optimally even after the stimuli (e.g., bacterial infection) has been treated, dual administration (cotreatment) of antibiotics and anti-inflammatory agents such as TNF biologics, CSAIDs – cytokine suppressive anti-inflammatory drugs (NF-κB and MAPK inhibitors) (126–128), PDE inhibitors, glucocorticoids, NSAIDs (15, 129, 130) should be explored.
Author Contributions
This review was conceived, conducted, written and reviewed by both EA and DA. Both authors approved the final manuscript.
Conflict of Interest Statement
The authors declare that the research was conducted in the absence of any commercial or financial relationships that could be construed as a potential conflict of interest.
Acknowledgments
Parts of this work are included in EA's PhD thesis titled Analysis of cervicovaginal fluid metabolome and microbiome in relation to preterm birth, published by the University of Sheffield in the White Rose eTheses Online, WREO: 13862. http://etheses.whiterose.ac.uk/13862/
Abbreviations
CDC, cholesterol-dependent cytolysin; CRH, corticotropin-releasing hormone; CSAIDs, cytokine suppressive anti-inflammatory drugs; CVF, cervicovaginal fluid; EMMPRIN, extracellular matrix metalloproteinase inducer; HIV, human immunodeficiency virus; HPA, hypothalamic-pituitary axis; HPV, human papillomavirus; HSV, herpes simplex virus; IL, interleukin; LPS, lipopolysaccharide; MAPK, mitogen-activated protein kinase; MIAC, microbial invasion of the amniotic cavity; MIP, macrophage inflammatory protein; MMP, matrix metalloproteinase; NF-κB, nuclear factor-κB; NGAL, neutrophil gelatinase-associated lipocalin; NK, natural killer; NLR, nod-like receptor; NSAIDs, non-steroidal anti-inflammatory drugs; PDE, phosphodiesterase; PGE2, prostaglandin E2; PGF2α, prostaglandin F2α; PROM, premature rupture of membranes; PRRs, pattern recognition receptors; PTB, preterm birth; PTL, preterm labor; RANTES, regulated on activation normal T cell expressed and secreted; SLPI, secretory leucocyte protease inhibitor; TLR, toll-like receptor; TNF, tumor necrosis factor.
References
1. Aldunate M, Srbinovski D, Hearps AC, Latham CF, Ramsland PA, Gugasyan R, et al. Antimicrobial and immune modulatory effects of lactic acid and short chain fatty acids produced by vaginal microbiota associated with eubiosis and bacterial vaginosis. Front Physiol. (2015) 6:164. doi: 10.3389/fphys.2015.00164
2. Witkin SS. The vaginal microbiome, vaginal anti-microbial defence mechanisms and the clinical challenge of reducing infection-related preterm birth. BJOG (2015) 122:213–8. doi: 10.1111/1471-0528.13115
3. Witkin S, Linhares I. Why do lactobacilli dominate the human vaginal microbiota? BJOG (2016) 124:606–11. doi: 10.1111/1471-0528.14390
4. Marchesi JR, Ravel J. The vocabulary of microbiome research: a proposal. Microbiome (2015) 3:31. doi: 10.1186/s40168-015-0094-5
5. Smith SB, Ravel J. The vaginal microbiota, host defence and reproductive physiology. J Physiol. (2017) 595:451–63. doi: 10.1113/JP271694
6. Weinstein L, Bogin M, Howard J, Finkelstone B. A survey of the vaginal flora at various ages with special reference to the Doderlein bacillus. Am J Obstet Gynecol. (1936) 32:211–8. doi: 10.1016/S0002-9378(36)90120-5
7. Cook RL, Sobel JD. Emerging role of lactobacilli in the control and maintenance of the vaginal bacterial microflora. Rev Infect Dis. (1990) 12:856–72.
8. Stumpf RM, Wilson BA, Rivera A, Yildirim S, Yeoman CJ, Polk JD, et al. The primate vaginal microbiome: comparative context and implications for human health and disease. Am J Phys Anthropol. (2013) 152:119–34. doi: 10.1002/ajpa.22395
9. Miller E, Beasley D, Dunn R, Archie E. Lactobacilli dominance and vaginal pH: why is the human vaginal microbiome unique? Front Microbiol. (2016) 7:1936. doi: 10.3389/fmicb.2016.01936
10. Rosenberg K, Trevathan W. Birth, obstetrics and human evolution. BJOG (2002) 109:1199–206. doi: 10.1046/j.1471-0528.2002.00010.x
11. Ravel J, Gajer P, Abdo Z, Schneider GM, Koenig SSK, McCulle SL, et al. Vaginal microbiome of reproductive-age women. Proc Natl Acad Sci USA. (2011) 108:4680–7. doi: 10.1073/pnas.1002611107
12. MacIntyre DA, Chandiramani M, Lee YS, Kindinger L, Smith A, Angelopoulos N, et al. The vaginal microbiome during pregnancy and the postpartum period in a European population. Sci Rep. (2015) 5:8988. doi: 10.1038/srep08988
13. Petrova MI, Reid G, Vaneechoutte M, Lebeer S. Lactobacillus iners: friend or foe? Trends Microbiol. (2017) 25:182–91. doi: 10.1016/j.tim.2016.11.007
14. Lee SK, Kim CJ, Kim DJ, Kang JH. Immune Cells in the female reproductive tract. Immune Network (2015) 15:16–26. doi: 10.4110/in.2015.15.1.16
15. Keelan JA. Pharmacological inhibition of inflammatory pathways for the prevention of preterm birth. J Reprod Immunol. (2011) 88:176–84. doi: 10.1016/j.jri.2010.11.003
16. Gupta S, Kumar N, Singhal N, Kaur R, Manektala U. Vaginal microflora in postmenopausal women on hormone replacement therapy. Indian J Pathol Microbiol. (2006) 49:457–61.
17. Romero R, Hassan SS, Gajer P, Tarca AL, Fadrosh DW, Nikita L, et al. The composition and stability of the vaginal microbiota of normal pregnant women is different from that of non-pregnant women. Microbiome (2014) 2:4. doi: 10.1186/2049-2618-2-4
18. DiGiulio DB, Callahan BJ, McMurdie PJ, Costello EK, Lyell DJ, Robaczewska A, et al. Temporal and spatial variation of the human microbiota during pregnancy. Proc Natl Acad Sci USA. (2015) 112:11060–5. doi: 10.1073/pnas.1502875112
19. Srinivasan S, Liu C, Mitchell CM, Fiedler TL, Thomas KK, Agnew KJ, et al. Temporal variability of human vaginal bacteria and relationship with bacterial vaginosis. PLoS ONE (2010) 5:e10197. doi: 10.1371/journal.pone.0010197
20. Lopes dos Santos Santiago G, Tency I, Verstraelen H, Verhelst R, Trog M, Temmerman M, et al. Longitudinal qPCR Study of the dynamics of L. crispatus, L. iners, A. vaginae, (Sialidase Positive) G. vaginalis, and P. bivia in the vagina. PLoS ONE (2012) 7:e45281. doi: 10.1371/journal.pone.0045281
21. Donders GGG. Definition and classification of abnormal vaginal flora. Best Pract Res Clin Obstet Gynaecol. (2007) 21:355–73. doi: 10.1016/j.bpobgyn.2007.01.002
22. Danielsson D, Teigen PK, Moi H. The genital econiche: focus on microbiota and bacterial vaginosis. Ann NY Acad Sci. (2011) 1230:48–58. doi: 10.1111/j.1749-6632.2011.06041.x
23. O'Hanlon DE, Moench TR, Cone RA. Vaginal pH and microbicidal lactic acid when lactobacilli dominate the microbiota. PLoS ONE (2013) 8:e80074. doi: 10.1371/journal.pone.0080074
24. Dennerstein GJ, Ellis DH. Oestrogen, glycogen and vaginal candidiasis. Aust N Z J Obstet Gynaecol. (2001) 41:326–8. doi: 10.1111/j.1479-828X.2001.tb01238.x
25. Nasioudis D, Beghini J, Bongiovanni AM, Giraldo PC, Linhares IM, Witkin SS. α-Amylase in vaginal fluid:association with conditions favorable to dominance of Lactobacillus. Reprod Sci. (2015) 22:1393–8. doi: 10.1177/1933719115581000
26. Boskey E, Cone R, Whaley K, Moench T. Origins of vaginal acidity: high D/L lactate ratio is consistent with bacteria being the primary source. Hum Reprod. (2001) 16:1809–13. doi: 10.1093/humrep/16.9.1809
27. Witkin SS, Mendes-Soares H, Linhares IM, Jayaram A, Ledger WJ, Forney LJ. Influence of vaginal bacteria and D-and L-lactic acid isomers on vaginal extracellular matrix metalloproteinase inducer: implications for protection against upper genital tract infections. MBio (2013) 4:e00460–13. doi: 10.1128/mBio.00460-13
28. Borgdorff H, Tsivtsivadze E, Verhelst R, Marzorati M, Jurriaans S, Ndayisaba GF, et al. Lactobacillus-dominated cervicovaginal microbiota associated with reduced HIV/STI prevalence and genital HIV viral load in African women. ISME J. (2014) 8:1781–93. doi: 10.1038/ismej.2014.26
29. van de Wijgert JHHM, Borgdorff H, Verhelst R, Crucitti T, Francis S, Verstraelen H, et al. The Vaginal microbiota: what have we learned after a decade of molecular characterization? PLoS ONE (2014) 9:e105998. doi: 10.1371/journal.pone.0105998
30. Kindinger LM, Bennett PR, Lee YS, Marchesi JR, Smith A, Cacciatore S, et al. The interaction between vaginal microbiota, cervical length, and vaginal progesterone treatment for preterm birth risk. Microbiome (2017) 5:6. doi: 10.1186/s40168-016-0223-9
31. Cadieux P, Burton J, Devillard E, Reid G. Lactobacillus by-products inhibit the growth and virulence of uropathogenic Escherichia coli. J Physiol Pharmacol. (2009) 60:13–8.
32. Thoma ME, Gray RH, Kiwanuka N, Aluma S, Wang MC, Sewankambo N, et al. Longitudinal changes in vaginal microbiota composition assessed by Gram stain among never sexually active pre-and postmenarcheal adolescents in Rakai, Uganda. J Pediatr Adolesc Gynecol. (2011) 24:42–7. doi: 10.1016/j.jpag.2010.07.002
33. Aldunate M, Tyssen D, Johnson A, Zakir T, Sonza S, Moench T, et al. Vaginal concentrations of lactic acid potently inactivate HIV. J Antimicrob Chemother. (2013) 68:2015–25. doi: 10.1093/jac/dkt156
34. Gong Z, Luna Y, Yu P, Fan H. Lactobacilli inactivate chlamydia trachomatis through lactic acid but not H2O2. PLoS ONE (2014) 9:e107758. doi: 10.1371/journal.pone.0107758
35. Edwards V, McComb E, Guttman H, Humphrys M, Forney L, Bavoil P, et al. P08.06 lactic acid isomers differentially reduce chlamydia trachomatis infection in a ph dependent manner. Sex Trans Infect. (2015) 91:A134. doi: 10.1136/sextrans-2015-052270.352
36. O'Hanlon DE, Moench TR, Cone RA. In vaginal fluid, bacteria associated with bacterial vaginosis can be suppressed with lactic acid but not hydrogen peroxide. BMC Infect Dis. (2011) 11:200. doi: 10.1186/1471-2334-11-200
37. Ahmed K, Tunaru S, Offermanns S. GPR109A, GPR109B and GPR81, a family of hydroxy-carboxylic acid receptors. Trends Pharmacol Sci. (2009) 30:557–62. doi: 10.1016/j.tips.2009.09.001
38. Kuchiiwa T, Nio-Kobayashi J, Takahashi-Iwanaga H, Yajima T, Iwanaga T. Cellular expression of monocarboxylate transporters in the female reproductive organ of mice: implications for the genital lactate shuttle. Histochem Cell Biol. (2011) 135:351–60. doi: 10.1007/s00418-011-0794-2
39. Kashket ER. Bioenergetics of lactic acid bacteria: cytoplasmic pH and osmotolerance. FEMS Microbiol Lett. (1987) 46:233–44. doi: 10.1111/j.1574-6968.1987.tb02463.x
40. Alakomi HL, Skyttä E, Saarela M, Mattila-Sandholm T, Latva-Kala K, Helander IM. Lactic acid permeabilizes gram-negative bacteria by disrupting the outer membrane. Appl Environ Microbiol. (2000) 66:2001–5. doi: 10.1128/aem.66.5.2001-2005.2000
41. Hearps AC, Tyssen D, Srbinovski D, Bayigga L, Diaz DJD, Aldunate M, et al. Vaginal lactic acid elicits an anti-inflammatory response from human cervicovaginal epithelial cells and inhibits production of pro-inflammatory mediators associated with HIV acquisition. Mucosal Immunol. (2017) 10:1480–90. doi: 10.1038/mi.2017.27
42. Tachedjian G, Aldunate M, Bradshaw CS, Cone RA. The role of lactic acid production by probiotic Lactobacillus species in vaginal health. Res Microbiol. (2017) 168:782–92. doi: 10.1016/j.resmic.2017.04.001
43. Ménard S, Candalh C, Bambou JC, Terpend K, Cerf-Bensussan N, Heyman M. Lactic acid bacteria secrete metabolites retaining anti-inflammatory properties after intestinal transport. Gut (2004) 53:821–8. doi: 10.1136/gut.2003.026252
44. Lee JM, Hwang KT, Jun WJ, Park CS, Lee MY. Antiinflammatory effect of lactic acid bacteria: inhibition of cyclooxygenase-2 by suppressing nuclear factor-kappaB in Raw264.7 macrophage cells. J Microbiol Biotechnol. (2008) 18:1683–8.
45. Latham T, Mackay L, Sproul D, Karim M, Culley J, Harrison DJ, et al. Lactate, a product of glycolytic metabolism, inhibits histone deacetylase activity and promotes changes in gene expression. Nucleic Acids Res. (2012) 40:4794–803. doi: 10.1093/nar/gks066
46. Wagner W, Ciszewski WM, Kania KD. L- and D-lactate enhance DNA repair and modulate the resistance of cervical carcinoma cells to anticancer drugs via histone deacetylase inhibition and hydroxycarboxylic acid receptor 1 activation. Cell Commun Signal. (2015) 13:36. doi: 10.1186/s12964-015-0114-x
47. Joziani B, Paulo CG, Iara ML, William JL, Steven SW. Neutrophil gelatinase-associated lipocalin concentration in vaginal fluid: relation to bacterial vaginosis and vulvovaginal candidiasis. Reprod Sci. (2015) 22:964–8. doi: 10.1177/1933719115570914
48. Lamont RF. Infection in the prediction and antibiotics in the prevention of spontaneous preterm labour and preterm birth. BJOG (2003) 110:71–5.
49. Pararas MV, Skevaki CL, Kafetzis DA. Preterm birth due to maternal infection: causative pathogens and modes of prevention. Eur J Clin Microbiol Infect Dis. (2006) 25:562–9. doi: 10.1007/s10096-006-0190-3
50. Rodriguez Jovita M, Collins, MD, Sjoden, B, Falsen E. Characterization of a novel Atopobium isolate from the human vagina: description of Atopobium vaginae sp. nov. Int J Syst Bacteriol. (1999) 4:1573–6. doi: 10.1099/00207713-49-4-1573
51. Zhou X, Bent SJ, Schneider MG, Davis CC, Islam MR, Forney LJ. Characterization of vaginal microbial communities in adult healthy women using cultivation-independent methods. Microbiology-Sgm (2004) 150:2565–73. doi: 10.1099/mic.0.26905-0
52. Petrova MI, Lievens E, Malik S, Imholz N, Lebeer S. Lactobacillus species as biomarkers and agents that can promote various aspects of vaginal health. Front Physiol. (2015) 6:81. doi: 10.3389/fphys.2015.00081
53. Borgdorff H, Armstrong SD, Tytgat HL, Xia D, Ndayisaba GF, Wastling JM, et al. Unique insights in the cervicovaginal Lactobacillus iners and L. crispatus Proteomes and their associations with microbiota dysbiosis. PLoS ONE (2016) 11:e0150767. doi: 10.1371/journal.pone.0150767
54. Macklaim JM, Gloor GB, Anukam KC, Cribby S, Reid G. At the crossroads of vaginal health and disease, the genome sequence of Lactobacillus iners AB-1. Proc Natl Acad Sci USA. (2011) 108(Suppl. 1):4688–95. doi: 10.1073/pnas.1000086107
55. Macklaim JM, Fernandes AD, Di Bella JM, Hammond JA, Reid G, Gloor GB. Comparative meta-RNA-seq of the vaginal microbiota and differential expression by Lactobacillus iners in health and dysbiosis. Microbiome (2013) 1:12. doi: 10.1186/2049-2618-1-12
56. Stafford GP, Parker JL, Amabebe E, Kistler J, Reynolds S, Stern V, et al. Spontaneous preterm birth is associated with differential expression of vaginal metabolites by Lactobacilli-dominated microflora. Front Physiol. (2017) 8:615. doi: 10.3389/fphys.2017.00615
57. Lewis WG, Robinson LS, Gilbert NM, Perry JC, Lewis AL. Degradation, foraging, and depletion of mucus sialoglycans by the vagina-adapted actinobacterium gardnerella vaginalis. J Biol Chem. (2013) 288:12067–79. doi: 10.1074/jbc.M113.453654
58. Padgett DA, Glaser R. How stress influences the immune response. Trends Immunol. (2003) 24:444–8. doi: 10.1016/S1471-4906(03)00173-X
59. Wrenn TR, Wood JR, Bitman J, Brinsfield TH. Vaginal glycogen assay for oestrogen: specificity and application to blood and urine. J Reprod Fertil. (1968) 16:301–4. doi: 10.1530/jrf.0.0160301
60. Zhou X, Brown CJ, Abdo Z, Davis CC, Hansmann MA, Joyce P, et al. Differences in the composition of vaginal microbial communities found in healthy Caucasian and black women. ISME J. (2007) 1:121–33. doi: 10.1038/ismej.2007.12
61. Zhou X, Hansmann MA, Davis CC, Suzuki H, Brown CJ, Schütte U, et al. The vaginal bacterial communities of Japanese women resemble those of women in other racial groups. FEMS Immunol Med Microbiol. (2010) 58:169–81. doi: 10.1111/j.1574-695X.2009.00618.x
62. Kenyon C, Colebunders R, Crucitti T. The global epidemiology of bacterial vaginosis: a systematic review. Am J Obstet Gynecol. (2013) 209:505–23. doi: 10.1016/j.ajog.2013.05.006
63. Cauci S, McGregor J, Thorsen P, Grove J, Guaschino S. Combination of vaginal pH with vaginal sialidase and prolidase activities for prediction of low birth weight and preterm birth. Am J Obstet Gynecol. (2005) 192:489–96. doi: 10.1016/j.ajog.2004.07.023
64. Foxman B, Wen A, Srinivasan U, Goldberg D, Marrs CF, Owen J, et al. Mycoplasma, bacterial vaginosis–associated bacteria BVAB3, race, and risk of preterm birth in a high-risk cohort. Am J Obstet Gynecol. (2014) 210:e221–226. doi: 10.1016/j.ajog.2013.10.003
65. Srinivasan S, Morgan MT, Fiedler TL, Djukovic D, Hoffman NG, Raftery D, et al. Metabolic signatures of bacterial vaginosis. MBio (2015) 6:e00204–15. doi: 10.1128/mBio.00204-15
66. Amsel R, Totten PA, Spiegel CA, Chen KCS, Eschenbach D, Holmes KK. Nonspecific vaginitis - diagnostic-criteria and microbial and epidemiologic associations. Am J Med. (1983) 74:14–22. doi: 10.1016/0002-9343(83)91112-9
67. Simoes JA, Discacciati MG, Brolazo EM, Portugal PM, Dini DV, Dantas MCM. Clinical diagnosis of bacterial vaginosis. Int J Gynecol Obstet. (2006) 94:28–32. doi: 10.1016/j.ijgo.2006.04.013
68. Muhleisen AL, Herbst-Kralovetz MM. Menopause and the vaginal microbiome. Maturitas (2016) 91:42–50. doi: 10.1016/j.maturitas.2016.05.015
69. Nugent RP, Krohn MA, Hillier S. Reliability of diagnosing bacterial vaginosis is improved by a standardized method of gram stain interpretation. J Clin Microbiol. (1991) 29:297–301.
70. Guaschino S, De Seta F, Piccoli M, Maso G, Alberico S. Aetiology of preterm labour: bacterial vaginosis. BJOG (2006) 113:46–51. doi: 10.1111/j.1471-0528.2006.01122.x
71. Hillier SL, Krohn MA, Cassen E, Easterling TR, Rabe LK, Eschenbach DA. The role of bacterial vaginosis and vaginal bacteria in amniotic fluid infection in women in preterm labor with intact fetal membranes. Clin Infect Dis. (1995) S276–8.
72. Hillier SL, Nugent RP, Eschenbach DA, Krohn MA, Gibbs RS, Martin DH, et al. Association between bacterial vaginosis and preterm delivery of a low-birth-weight infant. N Engl J Med. (1995) 333:1737–42. doi: 10.1056/nejm199512283332604
73. Schwebke JR. Role of vaginal flora as a barrier to HIV acquisition. Curr Infect Dis Rep. (2001) 3:152–5.
74. Cherpes TL, Meyn LA, Krohn MA, Lurie JG, Hillier SL. Association between acquisition of herpes simplex virus type 2 in women and bacterial vaginosis. Clin Infect Dis. (2003) 37:319–25. doi: 10.1086/375819
75. Haggerty CL, Hillier SL, Bass DC, Ness RB. Bacterial vaginosis and anaerobic bacteria are associated with endometritis. Clin Infect Dis. (2004) 39:990–5. doi: 10.1086/423963
76. Atashili J, Poole C, Ndumbe PM, Adimora AA, Smith JS. Bacterial vaginosis and HIV acquisition: a meta-analysis of published studies. AIDS (2008) 22:1493–501. doi: 10.1097/QAD.0b013e3283021a37
77. Goldenberg RL, Culhane JF, Iams JD, Romero R. Epidemiology and causes of preterm birth. Lancet (2008) 371:75–84. doi: 10.1016/S0140-6736(08)60074-4
78. Donders GG, Van Calsteren K, Bellen G, Reybrouck R, Van den Bosch T, Riphagen I, et al. Predictive value for preterm birth of abnormal vaginal flora, bacterial vaginosis and aerobic vaginitis during the first trimester of pregnancy. BJOG (2009) 116:1315–24. doi: 10.1111/j.1471-0528.2009.02237.x
79. Capoccia R, Greub G, Baud D. Ureaplasma urealyticum, Mycoplasma hominis and adverse pregnancy outcomes. Curr Opin Infect Dis. (2013) 26:231–40. doi: 10.1097/QCO.0b013e328360db58
80. Combs CA, Gravett M, Garite TJ, Hickok DE, Lapidus J, Porreco R, et al. Amniotic fluid infection, inflammation, and colonization in preterm labor with intact membranes. Am J Obstet Gynecol. (2014) 210:125.e121–15. doi: 10.1016/j.ajog.2013.11.032
81. Mitchell C, Marrazzo J. Bacterial vaginosis and the cervicovaginal immune response. Am J Reprod Immunol. (2014) 71:555–63. doi: 10.1111/aji.12264
82. Donders GGG, Vereecken A, Bosmans E, Dekeersmaecker A, Salembier G, Spitz B. Definition of a type of abnormal vaginal flora that is distinct from bacterial vaginosis: aerobic vaginitis. BJOG (2002) 109:34–43. doi: 10.1111/j.1471-0528.2002.00432.x
83. Donders G, Bellen G, Rezeberga D. Aerobic vaginitis in pregnancy. BJOG (2011) 118:1163–70. doi: 10.1111/j.1471-0528.2011.03020.x
84. Vieira-Baptista P, Lima-Silva J, Pinto C, Saldanha C, Beires J, Martinez-de-Oliveira J, et al. Bacterial vaginosis, aerobic vaginitis, vaginal inflammation and major Pap smear abnormalities. Eur J Clin Microbiol Infect Dis. (2016) 35:657–64. doi: 10.1007/s10096-016-2584-1
85. Mitra A, MacIntyre DA, Lee YS, Smith A, Marchesi JR, Lehne B, et al. Cervical intraepithelial neoplasia disease progression is associated with increased vaginal microbiome diversity. Sci Rep. (2015) 5:16865. doi: 10.1038/srep16865
86. Audirac-Chalifour A, Torres-Poveda K, Bahena-Román M, Téllez-Sosa J, Martínez-Barnetche J, Cortina-Ceballos B, et al. Cervical microbiome and cytokine profile at various stages of cervical cancer: a pilot study. PLoS ONE (2016) 11:e0153274. doi: 10.1371/journal.pone.0153274
87. Motevaseli E, Azam R, Akrami SM, Mazlomy M, Saffari M, Modarressi MH, et al. The effect of Lactobacillus crispatus and Lactobacillus rhamnosusculture supernatants on expression of autophagy genes and HPV E6 and E7 oncogenes in the HeLa cell line. Cell J. (2016) 17:601–7. doi: 10.22074/cellj.2016.3833
88. Eckert LO, Moore DE, Patton DL, Agnew KJ, Eschenbach DA. Relationship of vaginal bacteria and inflammation with conception and early pregnancy loss following in vitro fertilization. Infect Dis Obstet Gynecol. (2003) 11:11–7. doi: 10.1155/s1064744903000024
89. Ralph S, Rutherford A, Wilson J. Influence of bacterial vaginosis on conception and miscarriage in the first trimester: cohort study. BMJ (1999) 319:220–3. doi: 10.1136/bmj.319.7204.220
90. van Oostrum N, De Sutter P, Meys J, Verstraelen H. Risks associated with bacterial vaginosis in infertility patients: a systematic review and meta-analysis. Hum Reprod. (2013) 28:1809–15. doi: 10.1093/humrep/det096.
91. Gaudoin M, Rekha P, Morris A, Lynch J, Acharya U. Bacterial vaginosis and past chlamydial infection are strongly and independently associated with tubal infertility but do not affect in vitro fertilization success rates. Fertil Steril. (1999) 72:730–2. doi: 10.1016/S0015-0282(99)00310-6
92. Moore DE, Soules, MR, Klein NA, Fujimoto VY, Agnew KJ, Eschenbach DA. Bacteria in the transfer catheter tip influence the live-birth rate after in vitro fertilization. Fertil Steril. (2000) 74:1118–24. doi: 10.1016/S0015-0282(00)01624-1
93. Haahr T, Jensen JS, Thomsen L, Duus L, Rygaard K, Humaidan P. Abnormal vaginal microbiota may be associated with poor reproductive outcomes: a prospective study in IVF patients. Hum Reprod. (2016) 31:795–803. doi: 10.1093/humrep/dew026
94. Witkin SS, Linhares IM, Bongiovanni AM, Herway C, Skupski D. Unique alterations in infection-induced immune activation during pregnancy. BJOG (2011) 118:145–53. doi: 10.1111/j.1471-0528.2010.02773.x
95. Ramos Bde A, Kanninen, TT, Sisti, G, Witkin SS. Microorganisms in the female genital tract during pregnancy: tolerance versus pathogenesis. Am J Reprod Immunol. (2015) 73:383–9. doi: 10.1111/aji.12326
96. Howe L, Wiggins R, Soothill PW, Millar MR, Horner PJ, Corfield AP. Mucinase and sialidase activity of the vaginal microflora: implications for the pathogenesis of preterm labour. Int J STD AIDS (1999) 10:442–7. doi: 10.1258/0956462991914438
97. Cauci S, Hitti J, Noonan C. Vaginal hydrolytic enzymes immunoglobulin A against Gardenerella vaginalis toxin and risk of early preterm birth among women in preterm labor with bacterial vaginosis or intermediate flora. Am J Obstet Gynecol. (2002) 187:877–81. doi: 10.1067/mob.2002.127454
98. Cauci S, Culhane JF. Modulation of vaginal immune response among pregnant women with bacterial vaginosis by Trichomonas vaginalis, Chlamydia trachomatis, Neisseria gonorrhoeae, and yeast. Am J Obstet Gynecol. (2007) 196:133.e131–7. doi: 10.1016/j.ajog.2006.08.033
99. Cauci S, Culhane JF, Di, Santolo M, McCollum K. Among pregnant women with bacterial vaginosis, the hydrolytic enzymes sialidase and prolidase are positively associated with interleukin-1beta. Am J Obstet Gynecol. (2008) 198:132.e131–7. doi: 10.1016/j.ajog.2007.05.035
100. Srinivasan U, Misra D, Marazita ML, Foxman B. Vaginal and oral microbes, host genotype and preterm birth. Med hypotheses (2009) 73:963–75. doi: 10.1016/j.mehy.2009.06.017
101. Lockwood CJ, Kuczynski E. Risk stratification and pathological mechanisms in preterm delivery. Paed Perinat Epidemiol. (2001) 15:78–89. doi: 10.1046/j.1365-3016.2001.00010.x
102. Goldenberg RL, Andrews WW, Hauth JC. Choriodecidual infection and preterm birth. Nutr Rev. (2002) 60:S19–25.
103. Boyle AK, Rinaldi SF, Norman JE, Stock SJ. Preterm birth: Inflammation, fetal injury and treatment strategies. J Reprod Immunol. (2017) 119:62–6. doi: 10.1016/j.jri.2016.11.008
104. Ghartey JP, Smith BC, Chen Z, Buckley N, Lo Y, Ratner AJ, et al. Lactobacillus crispatus dominant vaginal microbiome is associated with inhibitory activity of female genital tract secretions against Escherichia coli. PLoS ONE (2014) 9:e96659. doi: 10.1371/journal.pone.0096659
105. Kalyoussef S, Nieves E, Dinerman E, Carpenter C, Shankar V, Oh J, et al. Lactobacillus proteins are associated with the bactericidal activity against E. coli of Female Genital Tract Secretions. PLos ONE (2012) 7:e49506. doi: 10.1371/journal.pone.0049506
106. Dezzutti CS, Richardson BA, Marrazzo JM, Tugetman J, Ramjee G, Taha T, et al. Mucosal Escherichia coli bactericidal activity and immune mediators are associated with HIV-1 seroconversion in women participating in the HPTN 035 trial. J Infect Dis. (2012) 206:1931–5. doi: 10.1093/infdis/jis555
107. Pellett Madan R, Masson L, Tugetman J, Werner L, Grobler A, Mlisana K, et al. Innate antibacterial activity in female genital tract secretions is associated with increased risk of HIV acquisition. AIDS Res Hum Retroviruses (2015) 31:1153–9. doi: 10.1089/aid.2015.0011
108. Fettweis JM, Brooks JP, Serrano MG, Sheth NU, Girerd PH, Edwards DJ, et al. Differences in vaginal microbiome in African American women versus women of European ancestry. Microbiology (2014) 160:2272–82. doi: 10.1099/mic.0.081034-0
109. Amabebe E. Analysis of Cervicovaginal Fluid Metabolome Microbiome in Relation to Preterm Birth. PhD thesis, University of Sheffield. White Rose eTheses Online, WREO: 13862 (2016). Available online at: http://etheses.whiterose.ac.uk/13862/
110. Amabebe E, Reynolds S, Stern V, Stafford G, Paley M, Anumba DOC. Cervicovaginal fluid acetate: a metabolite marker of preterm birth in symptomatic pregnant women. Front Med. (2016) 3:48. doi: 10.3389/fmed.2016.00048
111. Amabebe E, Reynolds S, Stern VL, Parker JL, Stafford GP, Paley MN, et al. Identifying metabolite markers for preterm birth in cervicovaginal fluid by magnetic resonance spectroscopy. Metabolomics (2016) 12:1–11. doi: 10.1007/s11306-016-0985-x
112. Amabebe E, Qiao H, Murdoch C, Anumba D. Cervicovaginal epithelial cells differentially express acetate and CXCL8 in co-culture with preterm birth-associated bacteria and protective lactobacillus species. Reprod Sci. (2018) 25(Suppl 1):187A–187A(F014).
113. Mares D, Simoes JA, Novak RM, Spear GT. TLR2-mediated cell stimulation in bacterial vaginosis. J Reprod Immunol. (2008) 77:91–9. doi: 10.1016/j.jri.2007.04.004
114. Nazli A, Kafka JK, Ferreira VH, Anipindi V, Mueller K, Osborne BJ, et al. HIV-1 gp120 induces TLR2- and TLR4-mediated innate immune activation in human female genital epithelium. J Immunol. (2013) 191:4246–58. doi: 10.4049/jimmunol.1301482
115. Beghini J, Linhares I, Giraldo P, Ledger W, Witkin S. Differential expression of lactic acid isomers, extracellular matrix metalloproteinase inducer, and matrix metalloproteinase-8 in vaginal fluid from women with vaginal disorders. BJOG (2014) 122:1580–5. doi: 10.1111/1471-0528.13072
116. Romero R, Chaiworapongsa T, Kuivaniemi H, Tromp G. Bacterial vaginosis, the inflammatory response and the risk of preterm birth: a role for genetic epidemiology in the prevention of preterm birth. Am J Obstet Gynecol. (2004) 190:1509–19. doi: 10.1016/j.ajog.2004.01.002
117. Steel JH, Malatos S, Kennea N, Edwards AD, Miles L, Duggan P, et al. Bacteria and inflammatory cells in fetal membranes do not always cause preterm labor. Pediatr Res. (2005) 57:404–11. doi: 10.1203/01.PDR.0000153869.96337.90
118. Choi SJ, Park SD, Jang IH, Uh Y, Lee A. The prevalence of vaginal microorganisms in pregnant women with preterm labor and preterm birth. Ann Lab Med. (2012) 32:194–200. doi: 10.3343/alm.2012.32.3.194
119. Menon R, Merialdi M, Betrán AP, Dolan S, Jiang L, Fortunato SJ, et al. Analysis of association between maternal tumor necrosis factor-α promoter polymorphism (−308), tumor necrosis factor concentration, and preterm birth. Am J Obstet Gynecol. (2006) 195:1240–8. doi: 10.1016/j.ajog.2006.05.037
120. Holst D, Garnier Y. Preterm birth and inflammation—The role of genetic polymorphisms. Eur J Obstet Gynecol Reprod Biol. (2008) 141:3–9. doi: 10.1016/j.ejogrb.2008.07.020
121. Gómez LM, Sammel MD, Appleby DH, Elovitz MA, Baldwin DA, Jeffcoat MK, et al. Evidence of a gene-environment interaction that predisposes to spontaneous preterm birth: a role for asymptomatic bacterial vaginosis and DNA variants in genes that control the inflammatory response. Am J Obstet Gynecol. (2010) 202:386.e381–6. doi: 10.1016/j.ajog.2010.01.042
122. Gracie SK, Lyon AW, Kehler HL, Pennell CE, Dolan SM, McNeil DA, et al. All Our Babies Cohort Study: recruitment of a cohort to predict women at risk of preterm birth through the examination of gene expression profiles and the environment. BMC Pregnancy Childbirth (2010) 10:87. doi: 10.1186/1471-2393-10-87
123. Romero R, Friel LA, Velez Edwards DR, Kusanovic JP, Hassan SS, Mazaki-Tovi S, et al. A genetic association study of maternal and fetal candidate genes that predispose to preterm prelabor rupture of membranes (PROM). Am J Obstet Gynecol. (2010) 203:361.e361–30. doi: 10.1016/j.ajog.2010.05.026
124. Menon R, Conneely KN, Smith AK. DNA methylation: an epigenetic risk factor in preterm birth. Reprod Sci. (2012) 19:6–13. doi: 10.1177/1933719111424446
125. Lee W-L, Tsui K-H, Wang P-H. Lactobacillus and health. J Chinese Med Assoc. (2016) 79:639–41. doi: 10.1016/j.jcma.2016.05.002
126. Lamont RF. Advances in the prevention of infection-related preterm birth. Front Immunol. (2015) 6:566. doi: 10.3389/fimmu.2015.00566
127. Ng PY, Ireland DJ, Keelan JA. Drugs to block cytokine signaling for the prevention and treatment of inflammation-induced preterm birth. Front Immunol. (2015) 6:166. doi: 10.3389/fimmu.2015.00166
128. Demelza JI, Elizabeth AN, Shaofu L, Adrian KC, Lisa FS, Matthew WK, et al. Preclinical evaluation of drugs to block inflammation-driven preterm birth. Innate Immunity (2016) 23:20–33. doi: 10.1177/1753425916672313
129. Gravett MG, Adams KM, Sadowsky DW, Grosvenor AR, Witkin SS, Axthelm MK, et al. Immunomodulators plus antibiotics delay preterm delivery after experimental intra-amniotic infection in a nonhuman primate model. Am J Obstet Gynecol. (2007) 197:518.e511–8. doi: 10.1016/j.ajog.2007.03.064
130. Grigsby PL, Novy MJ, Sadowsky DW, Morgan TK, Long M, Acosta E, et al. Maternal azithromycin therapy for Ureaplasma intraamniotic infection delays preterm delivery and reduces fetal lung injury in a primate model. Am J Obstet Gynecol. (2012) 207:475.e471–475.e414. doi: 10.1016/j.ajog.2012.10.871
Keywords: Lactobacillus, lactic acid, glycogen, estrogen, vaginal microbiota, bacterial vaginosis, preterm birth
Citation: Amabebe E and Anumba DOC (2018) The Vaginal Microenvironment: The Physiologic Role of Lactobacilli. Front. Med. 5:181. doi: 10.3389/fmed.2018.00181
Received: 01 May 2018; Accepted: 29 May 2018;
Published: 13 June 2018.
Edited by:
Sinuhe Hahn, Universität Basel, SwitzerlandReviewed by:
Zaleha Abdullah Mahdy, National University of Malaysia, MalaysiaMehmet Ali Vardar, Çukurova University, Turkey
Copyright © 2018 Amabebe and Anumba. This is an open-access article distributed under the terms of the Creative Commons Attribution License (CC BY). The use, distribution or reproduction in other forums is permitted, provided the original author(s) and the copyright owner are credited and that the original publication in this journal is cited, in accordance with accepted academic practice. No use, distribution or reproduction is permitted which does not comply with these terms.
*Correspondence: Dilly O. C. Anumba, ZC5vLmMuYW51bWJhQHNoZWZmaWVsZC5hYy51aw==