- 1Stem Cell Transplant Program, University Hospitals Cleveland Medical Center and Case Western Reserve University, Cleveland, OH, United States
- 2Divsion of Pediatric Hematology-Oncology, Department of Pediatrics, Case Western Reserve University, Cleveland, OH, United States
- 3Angie Fowler AYA Cancer Institute, UH Rainbow Babies & Children’s Hospital, Cleveland, OH, United States
- 4Pluristem LTD, Haifa, Israel
- 5Department of Pathology, Case Western Reserve University School of Medicine, Cleveland, OH, United States
- 6University Hospitals Cleveland Medical Center, Cleveland, OH, United States
Late-term complications of hematopoietic cell transplantation (HCT) are numerous and include incomplete engraftment. One possible mechanism of incomplete engraftment after HCT is cytokine-mediated suppression or dysfunction of the bone marrow microenvironment. Mesenchymal stromal cells (MSCs) elaborate cytokines that nurture or stimulate the marrow microenvironment by several mechanisms. We hypothesize that the administration of exogenous MSCs may modulate the bone marrow milieu and improve peripheral blood count recovery in the setting of incomplete engraftment. In the current study, we demonstrated that posttransplant intramuscular administration of human placental derived mesenchymal-like adherent stromal cells [PLacental eXpanded (PLX)-R18] harvested from a three-dimensional in vitro culture system improved posttransplant engraftment of human immune compartment in an immune-deficient murine transplantation model. As measured by the percentage of CD45+ cell recovery, we observed improvement in the peripheral blood counts at weeks 6 (8.4 vs. 24.1%, p < 0.001) and 8 (7.3 vs. 13.1%, p < 0.05) and in the bone marrow at week 8 (28 vs. 40.0%, p < 0.01) in the PLX-R18 cohort. As measured by percentage of CD19+ cell recovery, there was improvement at weeks 6 (12.6 vs. 3.8%) and 8 (10.1 vs. 4.1%). These results suggest that PLX-R18 may have a therapeutic role in improving incomplete engraftment after HCT.
Introduction
The late-term complications of hematopoietic cell transplantation (HCT) are numerous and include, but are not limited to, chronic graft-vs.-host disease (cGVHD), immunodeficiency, opporunistic infections, liver, pulmonary, and endocrine dysfunction, gonadal failure, and secondary malignancy (1, 2). Slow recovery of blood counts is also a well-recognized complication of transplantation, as well as incomplete engraftment, especially after umbilical cord blood (UCB) grafts and T-cell depleted or in vitro manipulated grafts (3). The incidence of long-term thrombocytopenia varies from 5 to 20% of HCT patients (4–10). Cytopenia may be related to transplant specific complications, such as cGVHD, inadequate cell dose of the graft, marrow dysfunction, immunosuppressive medications, and late chemotherapeutic effects (11, 12). Cytopenia after transplant is a predictor of non-relapse mortality (13–15). Possible mechanisms of cytopenia after HCT include cytokine-mediated suppression of megakaryopoiesis, erythropoiesis, or lymphopoiesis, and dysfunction of the bone marrow microenvironment (16, 17). Bone marrow stromal cells such as mesenchymal stromal cells (MSCs) elaborate cytokines that nurture or stimulate the marrow microenvironment by several mechanisms (18–20). MSCs can act as pericytes, wrapping around the endothelial cells of capillaries and venules and secrete bioactive products that contribute to tissue regeneration (21, 22). MSCs could also be selectively immune-suppressive and could affect the production of inhibitory cytokines (23, 24). Hence, it is possible that administration of MSCs in the setting of incomplete or delayed engraftment can modulate the milieu of the bone marrow microenvironment, through both direct interaction with hematopoietic stem cells (HSCs) and through secretion of cytokines, to improve blood counts posttransplant (25–27).
Mesenchymal stromal cells given at the time of HCT have been shown to improve engraftment, as well as have immunomodulatory effects in murine stem cell transplantation models (28, 29). MSCs can be found within multiple site, including adipose tissue and bone marrow (30, 31). The placenta is also an easily available source of cells from mesenchymal origin (32). PLacental eXpanded (PLX)-R18 (Pluristem Ltd., Haifa, Israel) is a human placental derived mesenchymal-like adherent stromal cells grown in vitro in a three-dimensional system. These cells secrete cytokines which contribute to hematopoietic reconstitution and differentiation, including IL-6, MCP-3, HGF, IL-8, FGF-7, GM-CSF, IL-10, and bFGF (33). Previous studies have demonstrated that intramuscular (IM) injections of PLX-R18 can mitigate mortality from acute radiation syndrome in murine models (33). PLX-R18 is being developed under the FDA’s animal rule for hematopoietic rescue from radiation syndrome. The effects of IM PLX-R18 appear to be related to transient secretion of pro-differentiation and pro-growth cytokines (33, 34).
Because there is no mouse model of delayed or incomplete engraftment, we proposed to administer PLX-R18 posttransplant in a well-established murine model of transplant utilizing sub-optimal doses of human CD34-selected UCB after radiation conditioning. Our hypothesis is that posttransplant IM administration of PLX-R18 will improve human hematopoietic engraftment, as measured by a quantitative improvement in human hematopoietic (CD45), B-cell (CD19), T-cell (CD3), megakaryocytic (CD41), and granulocyte (CD13, CD14) lineages in the peripheral blood and bone marrow.
Materials and Methods
Mice
Non-obese Diabetic–Severe Combined Immunodeficiency–IL2Rgammanull (NSG) mice were obtained from breeding pairs originally purchased from Jackson Laboratories (Bar Harbor, ME, USA). NSG mice were bred in a pathogen-free unit and maintained in sterile cages. Mice were handled and cared with strict adherence to guidelines as established by the Animal Resource Center and following study protocols as approved by the Institutional Animal Care and Use Committee at Case Western Reserve University School of Medicine (IACUC protocol 2015-0118).
PLX-18
PLacental eXpanded-R18 cells were produced and supplied by Pluristem Therapeutics, Inc. (Haifa, Israel). The PLX-R18 cells are mesenchymal-like adherent stromal cells derived from full-term placentas following Cesarean section. The PLX-R18 production process is composed of two major steps of isolation and culturing of the adherent stromal cells. In the first stage, adherent stromal cells are isolated from the placenta and passaged under two-dimensional cell growth conditions. Cells are then concentrated and cryopreserved. This intermediate cell stock is later thawed, passaged, and subsequently seeded for further expansion in three-dimensional growth in a bioreactor on non-woven fiber-made carriers, from which cells are subsequently harvested and cryopreserved.
PLacental eXpanded-R18 cells have a spindle-like morphology and are characterized by a high expression of typical MSC markers, such as CD105, CD73 and CD29, and lack surface expression of CD45, CD34, CD14, CD19, and HLA-DR. In addition, PLX-R18 does not express CD31 (an endothelial marker) and GlyA (an erythrocyte cell marker) on their surface. PLX-R18 cells exhibit limited capacity to differentiate in vitro into osteocytes and adipocytes compared to bone marrow-derived MSCs.
The cells are harvested and cryopreserved in liquid nitrogen as an “off the shelf” allogeneic adult cell source product. Prior to their administration the cells were thawed washed and suspended in Plasmalyte A solution (Baxter, Deerfield, IL, USA).
CD34+ Umbilical Cord Cell Isolation
Umbilical cord blood units were received from the Cleveland Cord Blood Center (Cleveland, OH, USA). Each unit was diluted 1:3 with phosphate buffered saline (PBS) + 0.5% Human Serum Albumin (HSA) and layered onto Ficoll Paque PLUS to isolate the mononuclear cells by density gradient. After a cell count and washes, the mononuclear cells were labeled per protocol using the Miltenyi Biotec (Cologne, Germany) CD34 Microbead Kit. The CD34 cells were then isolated using a positive selection column in a magnet and washed three times with MACS buffer. The CD34 cells from both UCB units were combined, counted, and re-suspended in PBS for injection into mice.
Murine Transplantation
NSG mice aged 8–12 weeks were used in all transplant experiments. Prior to transplantation with UCB, recipient mice were ear punched for individual identification. NSG mice received 300 cGy total body irradiation prior to receiving 5 × 105 CD34+ selected cells from human UCB, which has been shown to lead to incomplete human hematopoietic recovery (35). Following non-lethal irradiation (300 cGy), two groups of NSG mice were studied: (1) intravenous (IV) 5 × 105 UCB CD34+ cells (N = 10) and (2) IV 5 × 105 UBC CD34+ cells and 1 × 106 IM PLX-R18 on day 2 (D2) and day 7 (D7) (N = 14) (Figure 1). Initially, IV and IM injection of PLX-R18 were attempted; however, mice immediately developed fatal acute pulmonary toxicity after IV infusion (even with slow infusion) and thus the IM route obviously was preferred.
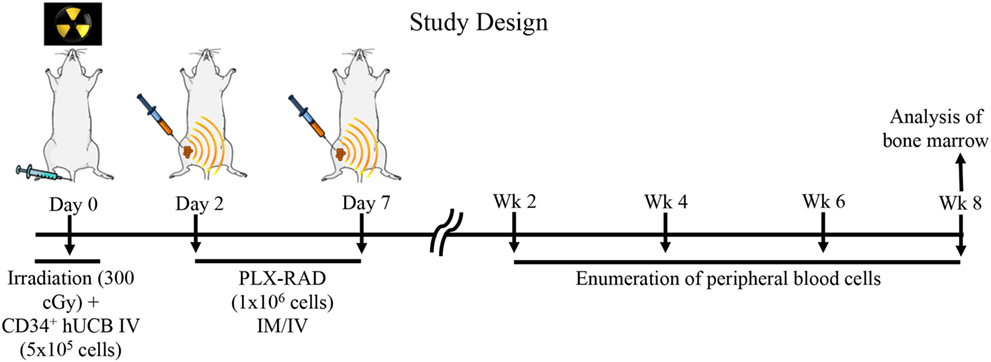
Figure 1. Study design: NSG mice received 300 cGy total body irradiation prior to receiving 5 × 105 CD34+ selected cells from human umbilical cord blood (UCB). Following non-lethal irradiation (300 cGy), two groups of NSG mice were studied: (1) intravenous (IV) 5 × 105 UCB CD34+ cells and (2) IV 5 × 105 UBC CD34+ cells and 1 × 106 intramuscular (IM) PLacental eXpanded (PLX)-R18 on D2 and D7. Peripheral blood from transplant recipient mice were analyzed on weeks 2, 4, 6, and 8. Bone marrow from transplant recipient mice was analyzed on week 8.
Injections
Umbilical cord blood cells were administered via tail vein injection suspended in a total volume of 200 µL. IM injections: PLX-18 cells were administered via IM right thigh injections suspended in a total volume of 100 µL.
Tissue Harvest and Preparation
The liver, ileum, ascending colon, and right tibia were harvested and were fixed in 10% buffered formalin, embedded in paraffin, cut into 5-μm thick sections and stained with hematoxylin and eosin for histologic examination. Slides were coded without reference to transplant group or treatment and reviewed in blinded fashion by an independent pathologist (HM).
Flow Cytometry
Peripheral blood samples from transplant recipient mice were analyzed on weeks 2, 4, 6, and 8. Bone marrow from transplant recipient mice was analyzed on week 8. Peripheral blood and bone marrow were stained for T cell markers (CD3), myeloid markers (CD45, CD13, CD14, CD41) along with B-cell markers (CD19). All monoclonal antibodies (mAbs) are human specific and were purchased from BD Biosciences Pharmingen (San Diego, CA, USA) or eBioscience (San Diego, CA, USA). At least 1 × 105 events were analyzed per conjugated MAb stain condition. Data were analyzed using CFlow software (Accuri, Ann Arbor, MI, USA). Human engraftment was expressed as percentage of CD45+ cells within the gated population of bone marrow or peripheral blood cells. Engraftment of CD45+ cell subsets (CD3, CD13, CD14, CD19) was expressed as a percentage within the gated population of bone marrow or peripheral blood cells. Platelet engraftment was expressed as a percentage of CD41+ events compared to total peripheral blood or bone marrow cells.
Histology
An independent hematopathologist, blinded to cohort characteristics, evaluated all tibial sections. The analysis included sections from each mouse in every cohort and was evaluated for myeloid-to-erythroid ratio, cellularity, and megakaryocyte percentages.
Statistics
All values are expressed as the mean ± SEM. Statistical comparisons between groups were completed using Mann and Whitney test (nonparametric data).
Results
Posttransplant IM Administration of PLX-R18 Improves Overall Human Hematopoietic Cell Engraftment (CD45) and B-Cell Engraftment (CD19) in the Peripheral Blood
To create a mouse model of incomplete engraftment, we injected sub-optimal dose (1 × 105 cells/mouse) of human UCB-derived CD34+ cells into NSG mice following 300 cGy whole-body irradiation. IM of 1 × 106 PLX-R18 were administered on days 2 and 7 following hUCB transplantation (Figure 1). Hematopoietic reconstitution analyses were performed every 2 weeks starting 2 weeks following hUCB transplantation. Bi-weekly flow cytometry analysis of the peripheral blood revealed the highest level of human CD45+ cells and B-cell engraftment in the IM PLX-R18 cohort at weeks 6 and 8, but not at weeks 2 and 4 (Figure 2). Specifically, there was a gradual increase in human CD45 percentage in the IV UCB cohort from week to week, the percentage of human CD45 engraftment in the peripheral blood spiked at week 6 in the IM PLX-R18 cohort, which was statistically significant (8.4 vs. 24.1%, p < 0.001; Figure 2A; Table 1). While a relative decrease in the percentage of human CD45 cells occurred in the peripheral blood of those mice treated with PLX-R18 at week 8 when compared to week 6, it was still superior when compared to the IV hUCB cohort (7.3 vs. 13.1%, p < 0.05, Figure 2A; Table 1). In contrast, mice treated with PLX-R18 demonstrated sustained B-cell engraftment superior to the control cohort at weeks 6 (3.8 vs. 12.6%, p < 0.01, Figure 2B; Table 1) and 8 posttransplant (4.1 vs. 10.1%, p < 0.01; Figure 2E; Table 1). Similar rises in the percentage of human T-cell, granulocyte, and platelet engraftment occurred in the peripheral blood of the PLX-R18 cohort, but these measurements were not statistically different from the IV hUCB cohort (Figures 2B–D,F; Table 1). Representative flow cytometry plots of peripheral blood analysis at 8 weeks posttransplant are shown in Figure 3.
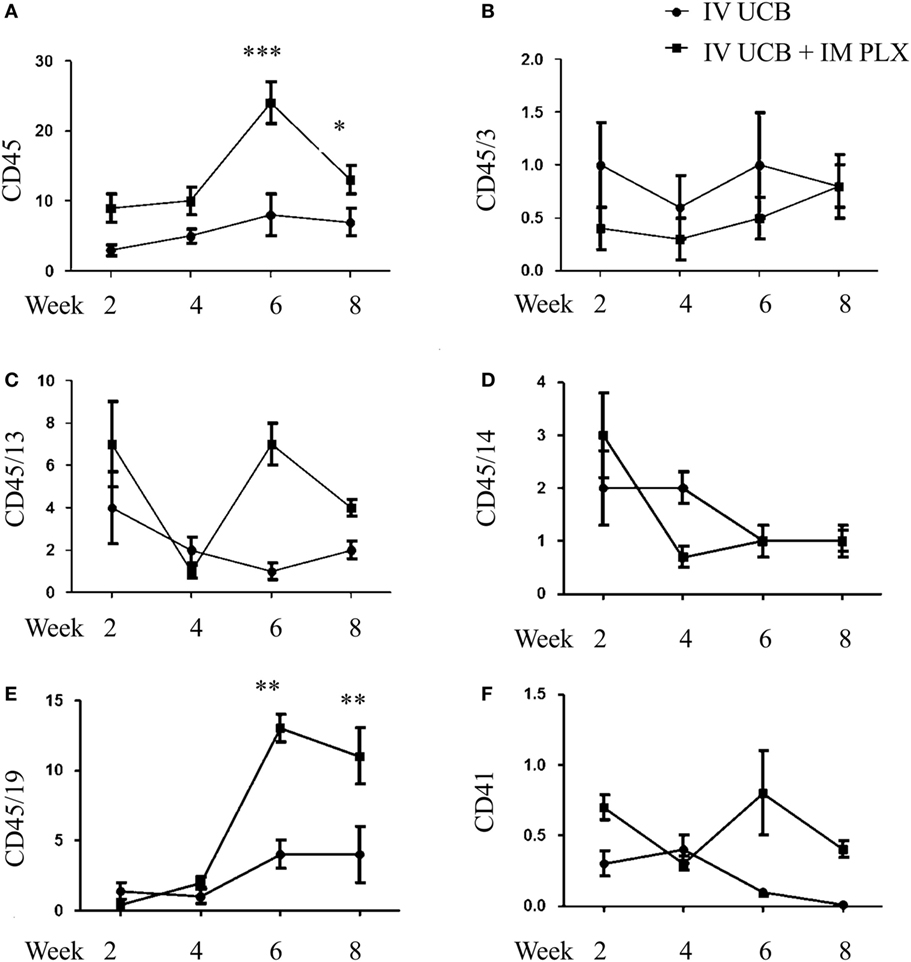
Figure 2. Posttransplant PLacental eXpanded (PLX)-R18 IM injection improves human leukocyte (CD45) and B-cell (CD45/19) engraftment in the peripheral blood. Flow cytometry results of CD45 (A), CD45/CD3 (B), CD45/CD13 (C), CD45/CD14 (D), CD45/CD19 (E), and CD41 (F) in the peripheral blood at weeks 2, 4, 6, and 8 weeks are shown. IV UCB, n = 9; IV UCB/IM PLX, n = 14; IV, intravenous; UCB, umbilical cord blood; IM, intramuscular; *p < 0.05; **p < 0.01; ***p < 0.001.
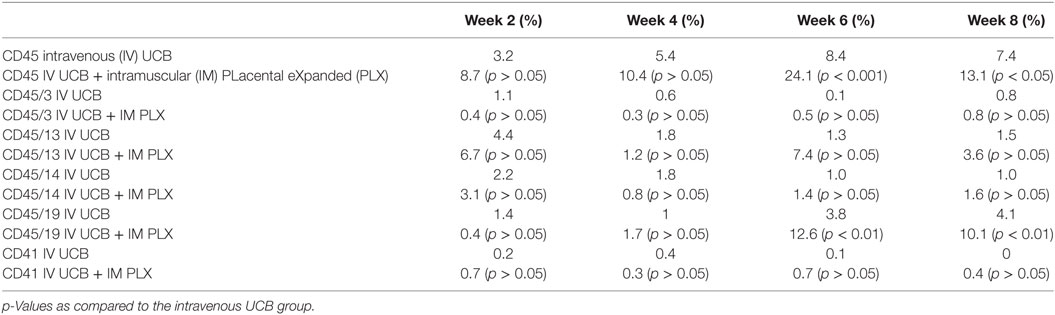
Table 1. Summary of the percent CD45, CD45/3, CD45/13, CD45/14, CD45/19, and CD41 in the peripheral blood at 2, 4, 6, and 8 weeks post umbilical cord blood (UCB) infusion.
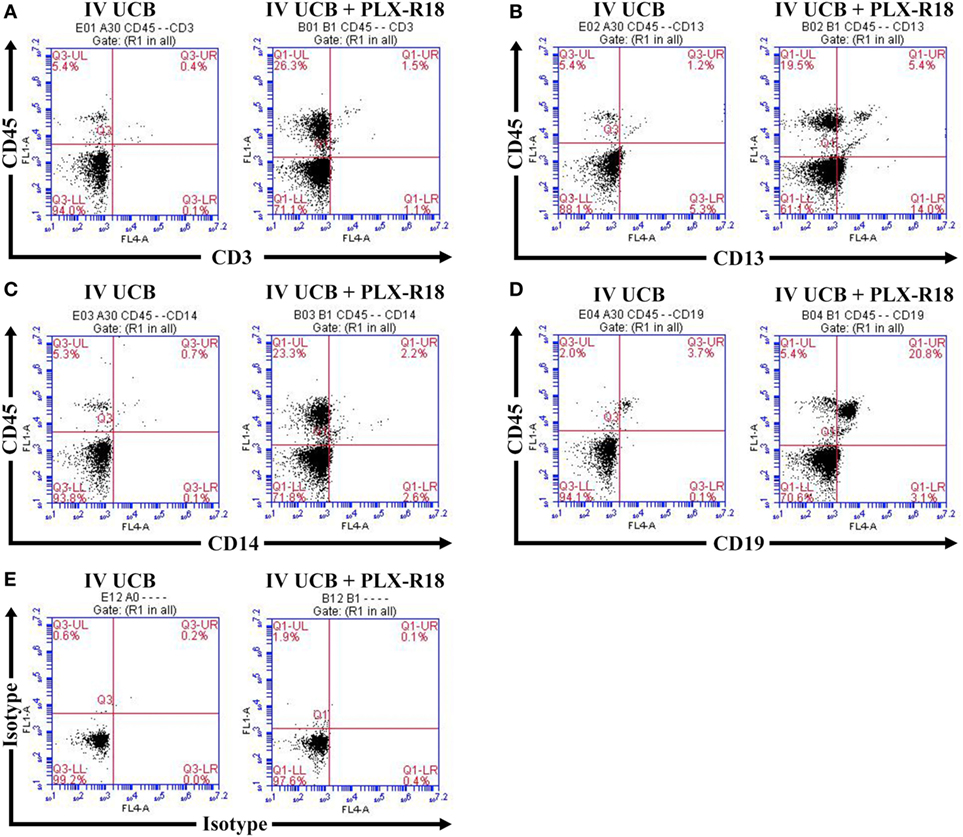
Figure 3. Representative flow cytometry plot of peripheral blood at 8 weeks posttransplant. CD45/CD3 (A) CD45/CD13 (B), CD45/CD14 (C), CD45/CD19 (D), and isotype control (E).
Posttransplant IM Administration of PLX-R18 Improves Overall Human CD45 Lineage Cell Engraftment in the Bone Marrow
Within the bone marrow, the IV UCB/IM PLX-R18 cohort demonstrated similar increases in CD45+ lineage cells at 8 weeks as compared with the IV hUCB cohort control (28 vs. 40.0%, p < 0.01; Figure 4A; Table 2). In contrast with the peripheral blood, there was no significant difference in B-cells within the bone marrow between cohorts (Figure 4E); however, the majority of the CD45+ cells were also CD19+. The differences between megakaryocytes and platelets were not significance (2.6 vs. 10.2%, p > 0.05; Figure 4F; Table 2), nor were the differences between T-cells, granulocytes, and platelets or megakaryocytes in the bone marrow between either cohorts (Figures 4B–D; Table 2). Representative flow cytometry plots of bone marrow at 8 weeks posttransplant are shown in Figure 5. Concurrent histopathologic evaluation demonstrated no difference in overall cellularity, myeloid-to-erythroid ratio, or megakaryocytic percentages between the bone marrow samples of the cohorts at 8 weeks posttransplant (Figure 6).
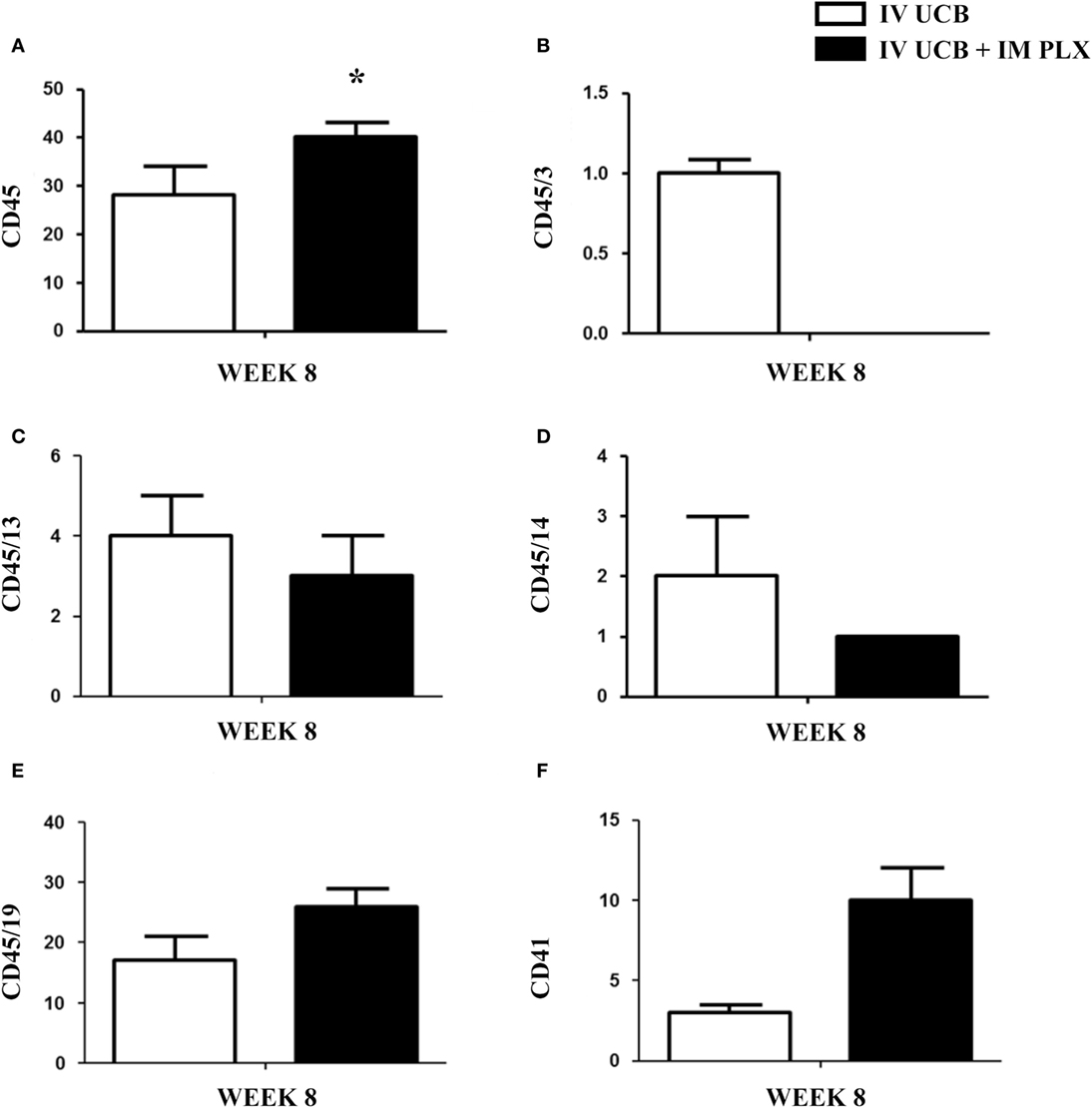
Figure 4. Posttransplant PLacental eXpanded (PLX)-R18 IM injection improves human leukocyte (CD45) in the bone marrow at 8 weeks. Flow cytometry results of the right tibia bone marrow CD45 (A), CD45/CD3 (B), CD45/CD13(C), CD45/CD14 (D), CD45/19 (F), and CD41 (E). IV UCB, n = 10; IV UCB/IM PLX, n = 14. IV, intravenous; UCB, umbilical cord blood; IM, intramuscular; *p < 0.05.

Table 2. Summary of the percent CD45, CD45/3, CD45/13, CD45/14, CD45/19, and CD41 in the bilateral tibial and femoral bone marrow at 8 weeks post umbilical cord blood (UCB) infusion.
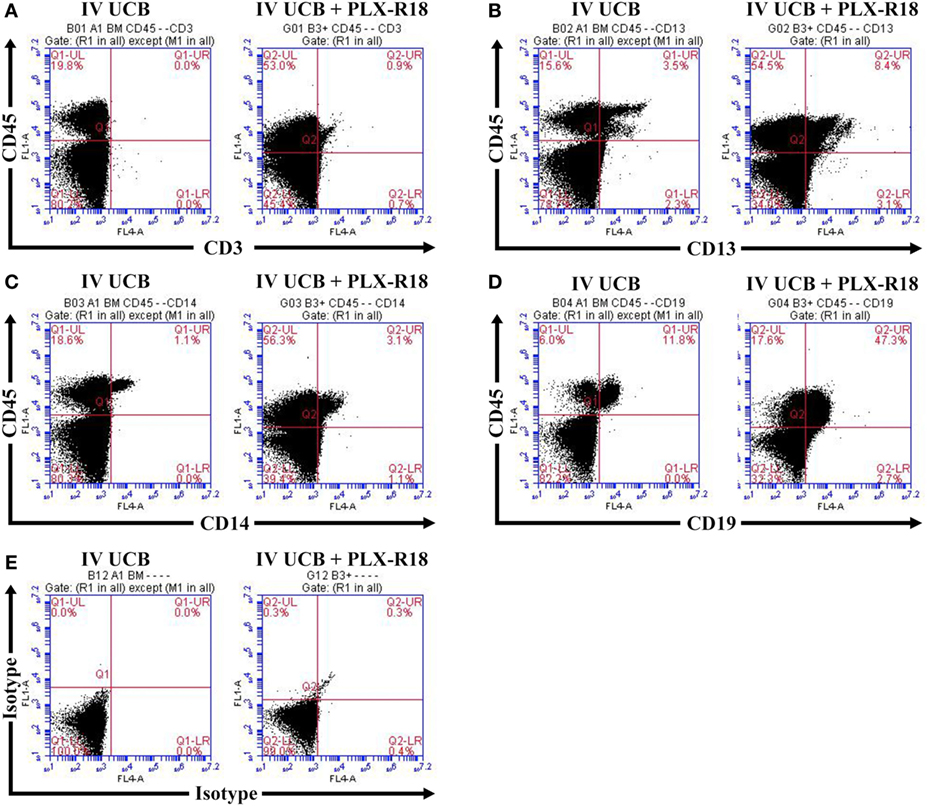
Figure 5. Representative flow cytometry plots of bone marrow at 8 weeks posttransplant. CD45/CD3 (A) CD45/CD13 (B), CD45/CD14 (C), CD45/CD19 (D), and isotype control (E).
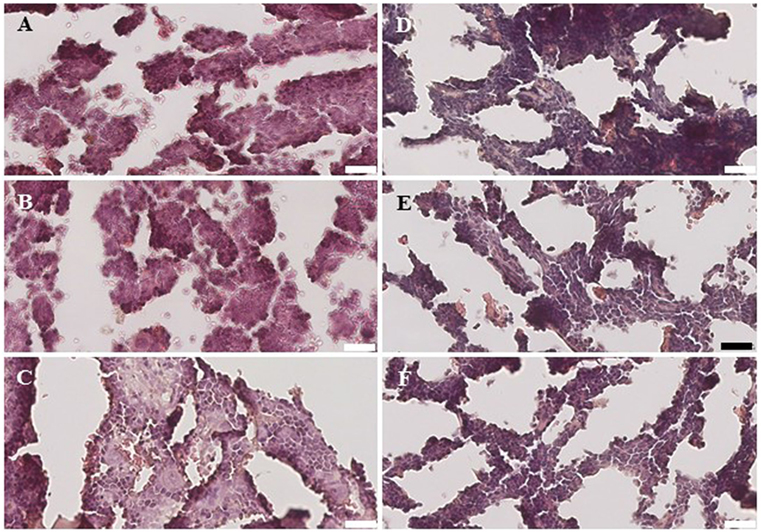
Figure 6. Histology of the bone marrow from NSG mice treated with intravenous (IV) 5 × 105 umbilical cord blood CD34+ cells (A–C) and NSG mice treated with IV 5 × 105 UBC CD34+ cells and 1 × 106 intramuscular PLacental eXpanded-R18 on D2 and D7 (D–F).
Conclusion
Our results support the hypothesis that IM injection of PLX-R18 improves the rapidity of human UCB engraftment in NSG mice when given in the posttransplant setting. It is likely that PLX-R18 exert their effect through secretion of pro-differentiation and proliferative cytokines that subsequently exert systemic effects, given that the PLX-R18 cells were injected intramuscularly and not intravenously or intra-osseously (36). As such, the PLX-R18 cells are unlikely to track into the bone marrow microenvironment. Additional bio-distribution studies of IM PLX-R18 demonstrate that the cells remain local, but few survive beyond 13 weeks past administration (in press).
Consistent with previous experiments by Prather et al., the effect of the PLX-R18 appeared to be transient, as the percentage of CD45+ cells declined within the peripheral blood after week 6. This drop off after week 6 likely is the result of exhaustion, death, or clearance of the PLX-R18 cells (34). Interestingly, though the effect within the peripheral blood appeared transient, the week 8 analysis of the bone marrow did show a significant increase in human engraftment as expressed by CD45+ cells, suggesting that marrow engraftment can be enhanced and may be permanent. Cytokine analyses of the serum are ongoing, but in vivo experiments demonstrate that PLX-R18 secretes granulocyte-colony stimulating factor, CXCL1, monocyte chemo-attractant protein-1, and interleukin-6; these concentrations peak in the serum at day 9 post-IM injection (in press).
Intravenous instead of IM injection of placental derived MSC combined with hUCB seems to result in similar engraftment outcomes. In an NOD/SCID mouse model of transplant utilizing sub-lethal conditioning, placental derived MSCs grown in a 3D-culture (PLX-I) combined with hUCB resulted in improved engraftment rates in the bone marrow at 6 weeks as measured by CD45+ human cells (37). Experiments involving IV cotransplant of hUCB and MSC has demonstrated improved engraftment rates of CD45+ human cells in the peripheral blood and bone marrow of NOD/SCID mice at 6–8 weeks posttransplant (20, 38). Similar to IM PLX-R18 cohort, the majority of the CD45+ population of cells in both the bone marrow and the peripheral blood were of the lymphoid lineage when hUCB was co-transplant with MSC. This finding is also consistent with other studies and suggests that PLX-R18 may also improve immune reconstitution following transplant (28, 39).
A detailed histological analysis of the bone marrow did not demonstrate any differences in overall cellularity, megakaryocytic:erythrocytic (M:E) ratio, or the percentages of megakaryocytes among cohorts. In the setting of non-myeloablative radiation, autologous recovery of murine bone marrow cells along with hUCB engraftment would be expected, and PLX-R18 cells may have enhanced the rate of this recovery.
The current therapeutic strategy is intriguing in that it may improve engraftment in patients who have incomplete engraftment after HCT. There is significant evidence in mice that MSC improve hUCB hematopoietic cell engraftment (18, 19). In theory, the observed experimental benefit could be extended to patients whose marrows have experienced delayed or incomplete engraftment. The safety and potential efficacy of IV administration of MSCs has been demonstrated in humans (25, 26). In autologous transplants, autologous MSCs co-infused with HSCs leads to rapid recovery of blood counts (26). In allogeneic transplantation, Lazarus et al. published a study in which 46 matched related donor transplant patients were infused with matched related culture-expanded MSCs prior to the hematopoietic graft. There were no toxicities associated with infusion of the MSC, and engraftment of neutrophils and platelets occurred at a median time of 14 and 20 days, respectively. In contrast with IV administration of MSC, IM administration of mesenchymal-like PLX cells has been documented to improve hematopoietic recovery in a three-patient cohort study (40). As such, a translational, a phase I international trial is now underway [ClinicalTrials.gov number NCT03002519].
Ethics Statement
Non-obese Diabetic–Severe Combined Immunodeficiency–IL2Rgammanull (NSG) mice were obtained from breeding pairs originally purchased from Jackson Laboratories (Bar Harbor, ME, USA). NSG mice were bred in a pathogen-free unit and maintained in sterile cages. Mice were handled and cared with strict adherence to guidelines as established by the Animal Resource Center and following study protocols as approved by the Institutional Animal Care and Use Committee at Case Western Reserve University School of Medicine (IACUC protocol 2015-0118).
Author Contributions
Concept and design (LM, HL, and AH), collection and/or assembly of data (LM, SE, KL, and HM), data analysis and interpretation (LM, HM, HL, and AH), manuscript writing (LM, RO, LP, HL, and AH), and final approval of manuscript (LM, HL, and AH).
Conflict of Interest Statement
The authors declare that the research was conducted in the absence of any commercial or financial relationships that could be construed as a potential conflict of interest.
The reviewer KCA and the handling editor declared their shared affiliation.
Acknowledgments
The authors thank Jane Reese and the Cellular Therapy Integrated Service of the National Center for Regenerative Medicine at Case Western Reserve University for their assistance. This study was supported in part by a grant from the National Institute of Health (Paul Calabresi Career Development Award for Clinical Oncology (K12): Clinical Oncology Research Career Development Program, 5K12CA076917-18), Pluristem Therapeutics, Inc., and the Clinical and Translational Science Collaborative of Cleveland (UL1TR000439 from the National Center for Advancing Translational Sciences (NCATS) component of the National Institutes of Health and NIH roadmap for Medical Research). Its contents are solely the responsibility of the authors and do not necessarily represent the official views of the NIH. The authors declare no conflict of interest.
Funding
National Institute of Health, Paul Calabresi Career Development Award for Clinical Oncology (K12): Clinical Oncology Research Career Development Program, 5K12CA076917-18. Clinical and Translational Science Collaborative of Cleveland (UL1TR000439 from the National Center for Advancing Translational Sciences (NCATS) component of the National Institutes of Health and NIH roadmap for MedicalResearch).
Abbreviations
MSCs, human mesenchymal stromal cells; HSC, Hematopoietic stem cells; UCB, Umbilical cord blood; IV, Intravenous; IM, Intramuscular; NSG, non-obese diabetic severe combined immunodeficiency- IL2Rgammanull; HCT, Hematopoietic cell transplant; mAbs, Monoclonal antibodies; PBS, Phosphate Buffered Saline; HSA, Human Serum Albumin; IACUC, Institutional Animal Care and Use Committee.
References
1. Copelan EA. Hematopoietic stem-cell transplantation. N Engl J Med (2006) 354(17):1813–26. doi:10.1056/NEJMra052638
2. Passweg JR, Baldomero H, Peters C, Gaspar HB, Cesaro S, Dreger P, et al. Hematopoietic SCT in Europe: data and trends in 2012 with special consideration of pediatric transplantation. Bone Marrow Transplant (2014) 49(6):744–50. doi:10.1038/bmt.2014.55
3. Norkin M, Lazarus HM, Wingard JR. Umbilical cord blood graft enhancement strategies: has the time come to move these into the clinic? Bone Marrow Transplant (2013) 48(7):884–9. doi:10.1038/bmt.2012.163
4. Bruno B, Gooley T, Sullivan KM, Davis C, Bensinger WI, Storb R, et al. Secondary failure of platelet recovery after hematopoietic stem cell transplantation. Biol Blood Marrow Transplant (2001) 7(3):154–62. doi:10.1053/bbmt.2001.v7.pm11302549
5. Bentley SA, Brecher ME, Powell E, Serody JS, Wiley JM, Shea TC. Long-term engraftment failure after marrow ablation and autologous hematopoietic reconstitution: differences between peripheral blood stem cell and bone marrow recipients. Bone Marrow Transplant (1997) 19(6):557–63. doi:10.1038/sj.bmt.1700717
6. Cassileth PA, Andersen J, Lazarus HM, Colvin OM, Bennett JM, Stadtmauer EA, et al. Autologous bone marrow transplant in acute myeloid leukemia in first remission. J Clin Oncol (1993) 11(2):314–9. doi:10.1200/JCO.1993.11.2.314
7. Mick R, Williams SF, Bitran JD. Patients at increased risk for late engraftment after transplantation: a novel method for their identification. Bone Marrow Transplant (1990) 6(3):185–91.
8. Sivakumaran M, Hutchinson RM, Pringle H, Graham S, Primrose L, Wood JK, et al. Thrombocytopenia following autologous bone marrow transplantation: evidence for autoimmune aetiology and B cell clonal involvement. Bone Marrow Transplant (1995) 15(4):531–6.
9. Sheridan WP, Begley CG, Juttner CA, Szer J, To LB, Maher D, et al. Effect of peripheral-blood progenitor cells mobilised by filgrastim (G-CSF) on platelet recovery after high-dose chemotherapy. Lancet (1992) 339(8794):640–4. doi:10.1016/0140-6736(92)90795-5
10. Bielski M, Yomtovian R, Lazarus HM, Rosenthal N. Prolonged isolated thrombocytopenia after hematopoietic stem cell transplantation: morphologic correlation. Bone Marrow Transplant (1998) 22(11):1071–6. doi:10.1038/sj.bmt.1701499
11. Pulanic D, Lozier JN, Pavletic SZ. Thrombocytopenia and hemostatic disorders in chronic graft versus host disease. Bone Marrow Transplant (2009) 44(7):393–403. doi:10.1038/bmt.2009.196
12. Dominietto A, Lamparelli T, Raiola AM, Van Lint MT, Gualandi F, Berisso G, et al. Transplant-related mortality and long-term graft function are significantly influenced by cell dose in patients undergoing allogeneic marrow transplantation. Blood (2002) 100(12):3930–4. doi:10.1182/blood-2002-01-0339
13. Anasetti C, Rybka W, Sullivan KM, Banaji M, Slichter SJ. Graft-v-host disease is associated with autoimmune-like thrombocytopenia. Blood (1989) 73(4):1054–8.
14. Akpek G, Lee SJ, Flowers ME, Pavletic SZ, Arora M, Lee S, et al. Performance of a new clinical grading system for chronic graft-versus-host disease: a multicenter study. Blood (2003) 102(3):802–9. doi:10.1182/blood-2002-10-3141
15. Bolwell B, Pohlman B, Sobecks R, Andresen S, Brown S, Rybicki L, et al. Prognostic importance of the platelet count 100 days post allogeneic bone marrow transplant. Bone Marrow Transplant (2004) 33(4):419–23. doi:10.1038/sj.bmt.1704330
16. Sakamaki S, Hirayama Y, Matsunaga T, Kuroda H, Kusakabe T, Akiyama T, et al. Transforming growth factor-beta1 (TGF-beta1) induces thrombopoietin from bone marrow stromal cells, which stimulates the expression of TGF-beta receptor on megakaryocytes and, in turn, renders them susceptible to suppression by TGF-beta itself with high specificity. Blood (1999) 94(6):1961–70.
17. Chatterjee S, Dutta RK, Basak P, Das P, Das M, Pereira JA, et al. Alteration in marrow stromal microenvironment and apoptosis mechanisms involved in aplastic anemia: an animal model to study the possible disease pathology. Stem Cells Int (2010) 2010:932354. doi:10.4061/2010/932354
18. in’t Anker PS, Noort WA, Kruisselbrink AB, Scherjon SA, Beekhuizen W, Willemze R, et al. Nonexpanded primary lung and bone marrow-derived mesenchymal cells promote the engraftment of umbilical cord blood-derived CD34(+) cells in NOD/SCID mice. Exp Hematol (2003) 31(10):881–9. doi:10.1016/S0301-472X(03)00202-9
19. Noort WA, Kruisselbrink AB, in’t Anker PS, Kruger M, van Bezooijen RL, de Paus RA, et al. Mesenchymal stem cells promote engraftment of human umbilical cord blood-derived CD34(+) cells in NOD/SCID mice. Exp Hematol (2002) 30(8):870–8. doi:10.1016/S0301-472X(02)00820-2
20. Metheny L 3rd, Eid S, Lingas K, Reese J, Meyerson H, Tong A, et al. Intra-osseous co-transplantation of CD34-selected umbilical cord blood and mesenchymal stromal cells. Hematol Med Oncol (2016) 1(1):41–5. doi:10.15761/HMO.1000105
21. da Silva Meirelles L, Caplan AI, Nardi NB. In search of the in vivo identity of mesenchymal stem cells. Stem Cells (2008) 26(9):2287–99. doi:10.1634/stemcells.2007-1122
22. Crisan M, Yap S, Casteilla L, Chen CW, Corselli M, Park TS, et al. A perivascular origin for mesenchymal stem cells in multiple human organs. Cell Stem Cell (2008) 3(3):301–13. doi:10.1016/j.stem.2008.07.003
23. Caplan AI. Adult mesenchymal stem cells: when, where, and how. Stem Cells Int (2015) 2015:628767. doi:10.1155/2015/628767
24. Ringden O. Mesenchymal stromal cells as first-line treatment of graft failure after hematopoietic stem cell transplantation. Stem Cells Dev (2009) 18(9):1243–6. doi:10.1089/scd.2009.1809.edi
25. Lazarus HM, Koc ON, Devine SM, Curtin P, Maziarz RT, Holland HK, et al. Cotransplantation of HLA-identical sibling culture-expanded mesenchymal stem cells and hematopoietic stem cells in hematologic malignancy patients. Biol Blood Marrow Transplant (2005) 11(5):389–98. doi:10.1016/j.bbmt.2005.02.001
26. Koç ON, Gerson SL, Cooper BW, Dyhouse SM, Haynesworth SE, Caplan AI, et al. Rapid hematopoietic recovery after coinfusion of autologous-blood stem cells and culture-expanded marrow mesenchymal stem cells in advanced breast cancer patients receiving high-dose chemotherapy. J Clin Oncol (2000) 18(2):307–16. doi:10.1200/JCO.2000.18.2.307
27. Fouillard L, Bensidhoum M, Bories D, Bonte H, Lopez M, Moseley AM, et al. Engraftment of allogeneic mesenchymal stem cells in the bone marrow of a patient with severe idiopathic aplastic anemia improves stroma. Leukemia (2003) 17(2):474–6. doi:10.1038/sj.leu.2402786
28. Carrancio S, Romo C, Ramos T, Lopez-Holgado N, Muntion S, Prins HJ, et al. Effects of MSC coadministration and route of delivery on cord blood hematopoietic stem cell engraftment. Cell Transplant (2013) 22(7):1171–83. doi:10.3727/096368912X657431
29. Li ZY, Wang CQ, Lu G, Pan XY, Xu KL. Effects of bone marrow mesenchymal stem cells on hematopoietic recovery and acute graft-versus-host disease in murine allogeneic umbilical cord blood transplantation model. Cell Biochem Biophys (2014) 70(1):115–22. doi:10.1007/s12013-014-9866-y
30. Pontikoglou C, Deschaseaux F, Sensebe L, Papadaki HA. Bone marrow mesenchymal stem cells: biological properties and their role in hematopoiesis and hematopoietic stem cell transplantation. Stem Cell Rev (2011) 7(3):569–89. doi:10.1007/s12015-011-9228-8
31. Minteer D, Marra KG, Rubin JP. Adipose-derived mesenchymal stem cells: biology and potential applications. Adv Biochem Eng Biotechnol (2013) 129:59–71. doi:10.1007/10_2012_146
32. Parolini O, Alviano F, Bagnara GP, Bilic G, Bühring HJ, Evangelista M, et al. Concise review: isolation and characterization of cells from human term placenta: outcome of the first international Workshop on Placenta Derived Stem Cells. Stem Cells (2008) 26(2):300–11. doi:10.1634/stemcells.2007-0594
33. Gaberman E, Pinzur L, Levdansky L, Tsirlin M, Netzer N, Aberman Z, et al. Mitigation of lethal radiation syndrome in mice by intramuscular injection of 3D cultured adherent human placental stromal cells. PLoS One (2013) 8(6):e66549. doi:10.1371/journal.pone.0066549
34. Prather WR, Toren A, Meiron M. Placental-derived and expanded mesenchymal stromal cells (PLX-I) to enhance the engraftment of hematopoietic stem cells derived from umbilical cord blood. Expert Opin Biol Ther (2008) 8(8):1241–50. doi:10.1517/14712598.8.8.1241
35. McDermott SP, Eppert K, Lechman ER, Doedens M, Dick JE. Comparison of human cord blood engraftment between immunocompromised mouse strains. Blood (2010) 116(2):193–200. doi:10.1182/blood-2010-02-271841
36. Ofir RP, Pinzur L, Levent A, Aberman Z, Gorodetsky R, Volk HD. Mechanism of action of PLX-R18, a placental-derived cellular therapy for the treatment of radiation-induced bone marrow failure. Blood (2015) 126(23):2417.
37. Burger OA, Ashtamker GF, Bercovich N, Rusanovsky M, Pinzur L, Jacov OM. Human placental derived mesenchymal stromal cells (MSC) grown in 3D-culture (PLX-I), promotes engraftment of human umbilical cord blood (hUCB) derived CD34+ cells in NOD/SCID mice. Blood (2007) 110(11):1416.
38. Hiwase SD, Dyson PG, To LB, Lewis ID. Cotransplantation of placental mesenchymal stromal cells enhances single and double cord blood engraftment in nonobese diabetic/severe combined immune deficient mice. Stem Cells (2009) 27(9):2293–300. doi:10.1002/stem.157
39. Allen HS, Sher N, Rekhes S, Pinzur L, Prezma T, Gorodetsky R, et al. Human placenta-derived stromal cells rescue mice from radiation-induced bone marrow failure: a Cytof-based mechanistic analysis. Blood (2016) 128(22):2677.
Keywords: cord blood stem cell transplantation, mesenchymal stromal cells, hematopoietic stem cell transplantation, engraftment, posttransplant complications
Citation: Metheny L, Eid S, Lingas K, Ofir R, Pinzur L, Meyerson H, Lazarus HM and Huang AY (2018) Posttransplant Intramuscular Injection of PLX-R18 Mesenchymal-Like Adherent Stromal Cells Improves Human Hematopoietic Engraftment in A Murine Transplant Model. Front. Med. 5:37. doi: 10.3389/fmed.2018.00037
Received: 11 October 2017; Accepted: 31 January 2018;
Published: 22 February 2018
Edited by:
Meral Beksac, Ankara University, TurkeyReviewed by:
Pinar Yurdakul, TOBB University of Economics and Technology, TurkeyKamil Can Akcali, Ankara University Medical School, Turkey
Copyright: © 2018 Metheny, Eid, Lingas, Ofir, Pinzur, Meyerson, Lazarus and Huang. This is an open-access article distributed under the terms of the Creative Commons Attribution License (CC BY). The use, distribution or reproduction in other forums is permitted, provided the original author(s) and the copyright owner are credited and that the original publication in this journal is cited, in accordance with accepted academic practice. No use, distribution or reproduction is permitted which does not comply with these terms.
*Correspondence: Leland Metheny, TGVsYW5kLm1ldGhlbnkmI3gwMDA0MDt1aGhvc3BpdGFscy5vcmc=
†Both senior authors contributed equally to this manuscript.