- 1Laboratory of Cellular and Molecular Immunology, GIGA-Research, University of Liège, Liège, Belgium
- 2Faculty of Veterinary Medicine, University of Liège, Liège, Belgium
- 3WELBIO, Walloon Excellence in Life Sciences and Biotechnology, Wallonia, Belgium
Eosinophils are typically considered to be specialized effector cells that are recruited to the tissues as a result of T helper type 2 (Th2) cell responses associated with helminth infections or allergic diseases such as asthma. Once at the site of injury, eosinophils release their cytotoxic granule proteins as well as preformed cytokines and lipid mediators, contributing to parasite destruction but also to exacerbation of inflammation and tissue damage. Accumulating evidence indicates that, besides their roles in Th2 responses, eosinophils also regulate homeostatic processes at steady state, thereby challenging the exclusive paradigm of the eosinophil as a destructive and inflammatory cell. Indeed, under baseline conditions, eosinophils rapidly leave the bloodstream to enter tissues, mainly the gastrointestinal tract, lungs, adipose tissue, thymus, uterus, and mammary glands, where they regulate a variety of important biological functions, such as immunoregulation, control of glucose homeostasis, protection against obesity, regulation of mammary gland development, and preparation of the uterus for pregnancy. This article provides an overview of the characteristics and functions of these homeostatic eosinophils.
Introduction
Eosinophils have long been perceived as terminally differentiated cytotoxic and destructive cells that play an effector role mainly in helminthic infections and allergic reactions, such as asthma (1). However, several recent studies have challenged the simplistic view of eosinophils as being exclusively involved in parasite destruction and allergic inflammation. Indeed, at steady state, blood eosinophils rapidly migrate into the gastrointestinal tract, lungs, adipose tissue, thymus, uterus, and mammary glands, where they are now known to exert a variety of essential homeostatic functions (2, 3). In this Mini Review, we summarize the advances in our understanding of the biology (distribution, phenotypic and morphological features, and ontogeny) and functions of these homeostatic eosinophils (hEos).
Distribution of hEos
In both humans and mice, most hEos are found in the non-esophageal portions of the gastrointestinal tract, where they principally reside in the lamina propria of the small intestine (4–7). Depending on the bibliographic source, the numbers of hEos in the gastrointestinal tract of mice are estimated to be 1.5- to 10-fold higher than in the blood (i.e., ranging from 3 × 105 to 2 × 106 cells) (8, 9). Pulmonary hEos are located in the lung parenchyma of both humans and mice (10). In C57BL/6 mice, the numbers of lung hEos exceed 4 × 105, which corresponds to two times the numbers of eosinophils present in the entire circulation (10). In the adipose tissue of mice, hEos account for 4–5% of the stromal/vascular fraction cells (11). In the other organs, hEos reside only transiently (8, 12–16). In mice, the numbers of thymic hEos increase drastically after birth to reach a peak at 2 weeks of age (15). Their numbers then diminish significantly but rise again at 16 weeks when thymic involution starts (15). During the first recruitment phase, hEos concentrate in the cortico-medullary region of the thymus, whereas they are more prominent in the medulla at latter time points (15). It is noteworthy that, in humans, hEos seem to be already present in the thymus of fetuses (14). In rodents, infiltration of the uterus by hEos coincides with the estrus cycle (12, 13). Numerous hEos are indeed observed in the uterus just prior to estrus, during estrus and 1 day postestrus, whereas only few hEos are present during diestrus (12, 13). The vast majority of these cells are located in the endometrium adjacent to the muscular layer (16). In mice, hEos also home to the mammary gland during postnatal development (17). Mammary hEos are principally found around the growing terminal end buds from 3 weeks until 8 weeks of age (17).
In vivo studies in humans and mice have shown that eosinophils spend only a short time (i.e., half-life between 3 and 24 h) in the circulation (8, 18, 19). By contrast, hEos remain for a longer time in the tissues. Indeed, their half-life is about 36 h in the lung and up to 6 days in the intestines, thymus, and uterus (8) (Figure 1). The longevity of tissue hEos seems to correlate with CD11c expression. Indeed, while intestine, uterus, and thymus hEos express CD11c, lung, and blood hEos do not express this marker (8, 10) (Figure 1).
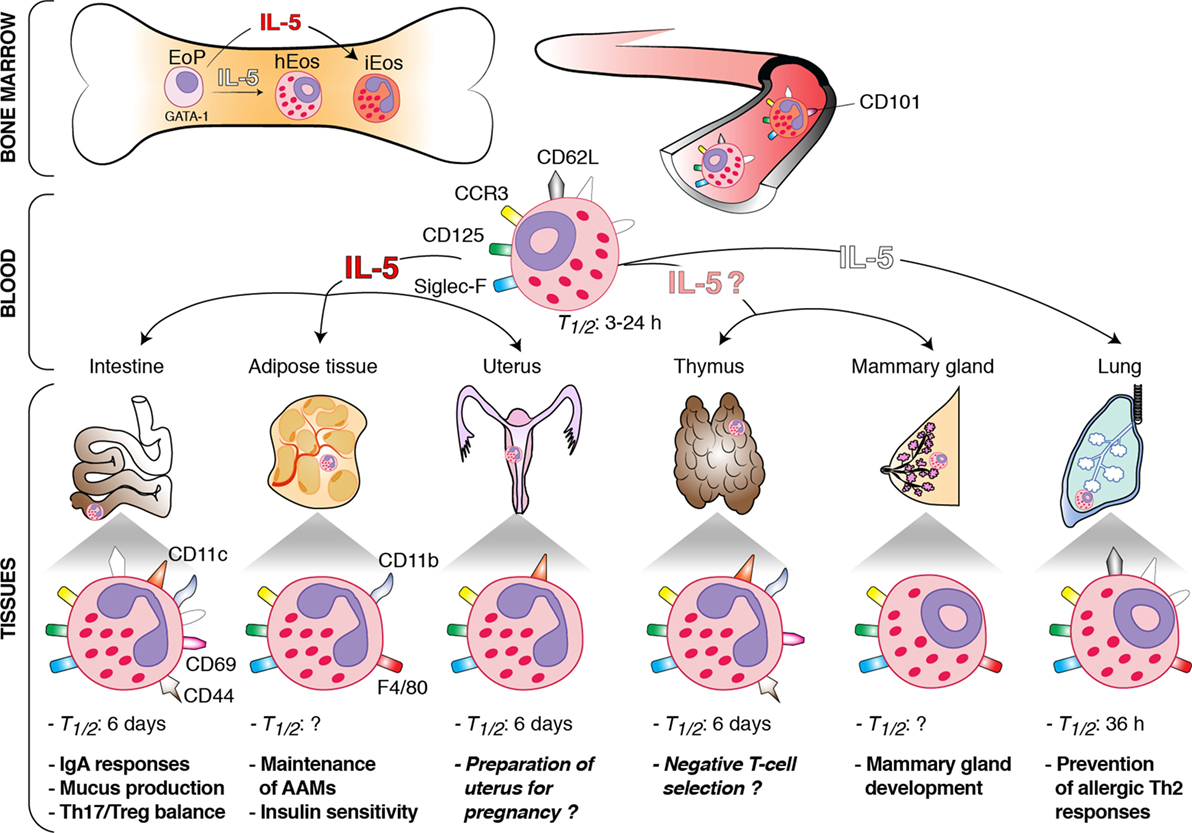
Figure 1. Schematic overview of the origin, interleukin (IL)-5 dependence, phenotype, and functions of homeostatic eosinophils (hEos) in mice. hEos are produced in the bone marrow from the EoP precursor independently of IL-5. Conversely, inflammatory eosinophils (iEos) require IL-5 for their production. hEos are uniformly characterized by expression of Siglec-F, F4/80, CD125, and CCR3. hEos transit through the blood circulation to home into tissues at baseline. Blood hEos have a ring-shaped nucleus and express CD62L, while iEos have a segmented nucleus and do not express CD62L but express CD101. hEos homing to the tissues is either dependent (dark red) or independent (white) on IL-5. The IL-5-(in)dependence of thymic and mammary gland hEos remains unknown. Tissue hEos display distinct phenotype, half-life (T1/2), and homeostatic functions. The surface phenotype depicted shows whether hEos express (colored symbols) or do not express (white symbols) the indicated surface markers. When marker expression is undefined, the symbol is not present. The function described in italic has been suggested, but a clear demonstration is still lacking. h, hours.
Time-course studies in mice have revealed that hEos are not present in the lung at birth but gradually increase in numbers to reach a maximal density by day 7 (10). This observation suggests a link between the colonization of the lung by hEos and the development of the microbiota. Paradoxically, however, hEos recruitment to the gastrointestinal tract seems to be independent of the bacterial flora. Indeed, prenatal mice have detectable hEos in their intestines, and germ-free mice display hEos levels similar to those of control colonized mice (5).
The basal recruitment of hEos to tissues is mainly driven by eotaxin-1 (CCL11), a chemokine produced by local cells such as epithelial cells, endothelial cells, fibroblasts, and monocytes (20–23). Correspondingly, hEos numbers are drastically reduced in the gastrointestinal tract, thymus, and uterus of eotaxin-1-deficient mice (5, 16, 24). Loss of CCR3, the major eotaxin-1 receptor (25, 26), results in defective tissue homing of hEos to the intestines but has no effect on the numbers of lung and thymus hEos (27), which likely relates to the fact that eotaxin-1 may act through alternative receptors such as CCR5 (28). Interleukin (IL)-5 and IL-13, two T helper type 2 (Th2) cytokines, may also promote, although to a lesser extent than eotaxin-1, trafficking of hEos under normal conditions (3, 5). IL-13 enhances eotaxin-1 production (29), while IL-5 supports eosinophil generation from bone marrow progenitors, enhances their sensitivity to eotaxin-1, and sustains their survival (30–32). It has been recently shown that the major source of basal IL-5 and IL-13 in the gastrointestinal tract and the adipose tissue are type 2 innate lymphoid cells (ILC2s) (29, 33). Moreover, after food intake, the vasoactive intestinal peptide stimulates intestinal ILC2 to enhance their secretion of IL-5 and IL-13, linking eosinophil levels with metabolic cycling (29).
Morphological and Phenotypic Features of hEos
hEos have been mainly characterized in mice. They display most of the typical features of eosinophils, including red staining granules containing toxic cationic proteins (e.g., major basic proteins) and combined expression of CCR3, Siglec-F, and CD125 (i.e., the subunit α of the IL-5 receptor) (8, 9, 34) (Figure 1). They may also express CD11b (intestines, thymus, and adipose tissue), F4/80 (mammary glands, lung, and adipose tissue), CD69 (intestines and thymus), and CD44 (intestines and thymus) (6, 8, 10, 11, 15, 17, 35). In addition, most tissue hEos have a segmented nucleus and express CD11c (8, 13, 15, 16, 24, 35). Lung hEos represent an exception and rather resemble resting blood eosinophils. Indeed, both blood and lung eosinophils have a ring-shaped nucleus (as is the case for mammary hEos as well), express CD62L, display only intermediate levels of Siglec-F, and are negative for CD11c (8, 10, 17, 36, 37) (Figure 1). In mouse eosinophils, such characteristics, especially the presence of a ring-shaped nucleus, are considered a sign of cell immaturity (38, 39), suggesting that pulmonary hEos retain an immature phenotype when spreading into the lungs. However, they undergo piecemeal degranulation and are capable of phagocytosis, demonstrating their functionality (10). Interestingly, the number, localization, and morphological, phenotypic, and transcriptomic features of lung hEos remain unchanged, and differ from those of inflammatory eosinophils (iEos), during allergic airway inflammation (10). iEos, which are abundantly recruited to the lung during airway allergy, are indeed defined as SiglecFhiCD62L−CD101hi cells with a segmented nucleus (CD101 being an iEos marker that is not expressed by lung hEos) (10). These observations suggest that hEos and iEos represent distinct eosinophil subsets. In line with this hypothesis, hEos- and iEos-like eosinophils are present in the blood of asthmatic mice (10), indicating that the differentiation of both subsets occurs even before their recruitment to the tissues. Furthermore, the parenchymal hEos found in non-asthmatic human lungs (Siglec-8+CD62L+IL-3Rlo cells) are phenotypically distinct from the iEos isolated from the sputa of eosinophilic asthmatic patients (Siglec-8+CD62LloIL-3Rhi cells), confirming the mouse findings (10).
Origin of hEos
Eosinophil development depends on a complex interplay of several transcription factors, including GATA-binding protein-1 (GATA-1), CCAAT/enhancer-binding protein-α and -ε (C/EBP-α and -ε), E26 family transcription factor PU.1 (PU.1), and X-box-binding protein-1 (1, 3, 40–42). Among these transcription factors, GATA-1 is the most selective, as attested by the fact that ΔdblGATA mice, in which the double palindromic GATA-1-binding site in the Gata1 promoter has been genetically deleted, specifically lack eosinophils, including blood and tissue hEos (7, 10, 11, 36, 43). IL-5, which is the most specific cytokine for the eosinophil lineage, is dispensable for the steady-state production of eosinophils. Indeed, the basal numbers of blood eosinophils are only moderately reduced in IL-5-deficient mice, which are, however, unable to develop eosinophilia in the context of a Th2 response (44). Interestingly, recruitment of hEos to the tissues is independent (lungs), partly dependent (gastrointestinal tract and uterus), or entirely dependent (adipose tissue) on local IL-5 production (5, 10, 33, 44, 45) (Figure 1). Given that IL-5 enhances eosinophil survival following migration into the tissues, and that hEos that partly depend on IL-5 (gastrointestinal tract and uterus) have a higher half-life (see Distribution of hEos) than the IL-5-independent ones (lungs), one may speculate that the longevity of tissue hEos is directly linked to their dependence on IL-5. All these observations, if applicable to humans, could also explain why residual eosinophils are found in the blood and lungs of patients treated with anti-α-IL-5 antibodies (46–48).
Functions of hEos
Depending on the type of tissue they infiltrate, hEos are fulfilling completely different tasks, suggesting the local environment is able to drive hEos functions according to its specific needs. Here, we will review the tissue-specific homeostatic functions of hEos, also summarized in Figure 1.
Gastrointestinal Tract
Small intestinal hEos are now considered as actively contributing to intestinal homeostasis, allowing the host to cope with the constant and intense exposition to potentially pathogenic microorganisms and foreign and food antigens. In two independent studies, hEos have been shown to be required for the development and maintenance of immunoglobulin (Ig)A-producing plasma cells (7, 35), concordant with the function of bone marrow eosinophils in supporting plasma cell survival (49). They also promote class switching toward secretory IgA, components involved in the neutralization and regulation of intestinal microorganisms (7, 35). In addition, eosinophil deficiency has been associated with altered gut microbiota composition (7, 35), altered development of Peyer’s patches, and decreased mucus production in the small intestine (35), as well as increased numbers of Th17 cells (50) and decreased numbers of regulatory T cells and dendritic cells in gut-associated tissues (7). In vitro, Chen and colleagues have shown that small intestinal hEos are able to induce differentiation of naive T cells into Foxp3+ regulatory T cells through IL-1β- and retinoic acid-dependent mechanisms (51). More recently, small intestinal hEos have also been shown to suppress the in vitro differentiation of Th17 cells and intestinal T cell-derived IL-17 production by secreting large amounts of the IL-1 receptor antagonist IL-1Rα (50). Altogether, these findings are concordant with the idea that small intestinal hEos contribute to intestinal homeostasis by regulating adaptive humoral IgA responses and cellular T cell responses.
Adipose Tissue
Eosinophils have been emerging as central regulators of adipose tissue metabolism and metabolic health. In adipose tissues, hEos are present together with alternatively activated macrophages (AAMs), and such hEos produce IL-4, thereby favoring the polarization of adipose macrophages toward the alternatively activated phenotype (11). AAMs play a crucial role in glucose homeostasis and development of beige fat, which improves glucose tolerance, insulin reactivity, and, hence, protects against obesity (11, 52, 53). In the absence of adipose hEos, AAMs are greatly reduced and biogenesis of beige fat is impaired (11, 54). Moreover, eosinophil-deficient mice on high-fat diet develop obesity, insulin resistance, and impaired glucose tolerance (11). Conversely, wild-type mice on a high-fat diet but infected with the gastrointestinal nematode Nippostrongylus brasiliensis, which triggered a greater eosinophil recruitment in the adipose tissues, exhibit a long-lasting improved sensitivity to insulin and glucose tolerance (11).
This important hEos/macrophage axis is regulated by ILC2s, which sustain adipose hEos and AAMs (33), and is promoted by microbiota depletion (55) and caloric restriction (56).
Uterus
It is known for decades that hEos infiltrate the non-pregnant uterus of rodents and humans in a cyclic manner, with a peak during estrus (13, 57, 58), but few studies have assessed their potential contribution to the physiology of uterus and to reproductive functions. Gouon-Evans and Pollard examined eotaxin-deficient animals, in which recruitment of hEos to the uterus was impaired, and found a delay in the establishment of the first estrus cycle along with the first age of parturition in those animals compared to wild-type controls (16). While these observations point toward a potential role for hEos in preparing the uterus for pregnancy (16), they must be balanced by the fact that the timing of establishment of subsequent estrus cycles in mature mice is not affected by the absence of eosinophils (16). Most importantly, no fertility issues have been reported in constitutively eosinophil-deficient mice (36, 59), demonstrating that hEos are not essential for normal reproduction.
Thymus
The presence of thymic hEos in the close vicinity of immature double-negative thymocytes and their abundance in neonates suggest that they may contribute to the process of central tolerance and negative T-cell selection (15). Supporting this, thymic hEos numbers rapidly increase and hEos cluster with apoptotic bodies in an acute model of MHC-I-dependent negative selection (15). Another report proposes that hEos may contribute to the clearance of apoptotic cells, as eosinophil-deficient mice subjected to irradiation-induced thymocyte death are impaired in their ability to phagocyte apoptotic cells (43). However, the definitive proof of a homeostatic role for thymic eosinophils in the process of negative T-cell selection is currently lacking.
Mammary Gland
A role for eosinophils in regulating postnatal mammary gland development has been proposed in mice (17). Indeed, ablation of hEos recruitment to the mammary glands in eotaxin-deficient animals resulted in a reduced number of branches of the mammary ductal tree and of terminal end buds (i.e., the precursors of alveolar buds) (17). A similar phenotype was observed in the mammary tissue of IL-5-deficient mice as compared to the one from wild-type mice, although the specific contribution of IL-5 itself vs. IL-5-dependent eosinophils has not been assessed in this model (60). Nevertheless, such IL-5-mediated developmental events appear to have functional consequences, as IL-5-deficient nursing dams gave rise to decreased litter size and weanling survival, a phenomenon rescued when IL-5-deficient pups were nursed by IL-5-sufficient dams (60).
Lungs
Microarray analyses revealed that lung hEos, unlike lung iEos, express several genes, such as Anxa1, Nedd4, Runx3, Serpinb1a, and Ldlr, that are implicated in the maintenance of lung immune homeostasis, and especially in the negative regulation of Th2 cell responses (10). In line with this observation, eosinophil-deficient ΔdblGATA mice exhibit increased sensitivity to house dust mites (10), confirming that lung hEos are endowed with the capacity to prevent Th2-driven airway allergy. This immunosuppressive function of lung hEos is linked to their unique ability to inhibit the maturation, and therefore the pro-Th2 function, of allergen-loaded dendritic cells (10).
Conclusion
Although hEos are far from being fully characterized, it is fascinating to see how fast our understanding of the complexity of their phenotype and functions is growing. The fact that these cells exert crucial homeostatic roles at multiple levels merits further investigations and is of medical importance. Indeed, anti-eotaxin-1 and eosinophil-depleting agents, such as humanized anti-IL-5 receptor antibodies and anti-Siglec-8 molecules, are currently being developed to treat eosinophilic disorders such as allergic asthma (9, 61–66), and one has to keep in mind the possibility that such drugs may disrupt tissue homeostasis by preventing organ-specific homing of hEos or by affecting their survival or functions.
Author Contributions
TM, CM, and FB conducted a review of the literature. TM and FB wrote the manuscript.
Conflict of Interest Statement
The authors declare that the research was conducted in the absence of any commercial or financial relationships that could be construed as a potential conflict of interest.
Acknowledgments
The authors thank Raja Fares, Cédric François, and Ilham Sbai for excellent secretarial assistance.
Funding
This publication is supported by the F.R.S.-FNRS for the FRFS-WELBIO (Walloon Excellence in Life Sciences and Biotechnology) under grant CR-2012S-01R. TM is a research associate of the F.R.S.-FNRS and is supported by the Acteria Foundation.
References
1. Rothenberg ME, Hogan SP. The eosinophil. Annu Rev Immunol (2006) 24:147–74. doi:10.1146/annurev.immunol.24.021605.090720
2. Jacobsen EA, Helmers RA, Lee JJ, Lee NA. The expanding role(s) of eosinophils in health and disease. Blood (2012) 120(19):3882–90. doi:10.1182/blood-2012-06-330845
3. Travers J, Rothenberg ME. Eosinophils in mucosal immune responses. Mucosal Immunol (2015) 8(3):464–75. doi:10.1038/mi.2015.2
4. Kato M, Kephart GM, Talley NJ, Wagner JM, Sarr MG, Bonno M, et al. Eosinophil infiltration and degranulation in normal human tissue. Anat Rec (1998) 252(3):418–25. doi:10.1002/(SICI)1097-0185(199811)252:3<418::AID-AR10>3.0.CO;2-1
5. Mishra A, Hogan SP, Lee JJ, Foster PS, Rothenberg ME. Fundamental signals that regulate eosinophil homing to the gastrointestinal tract. J Clin Invest (1999) 103(12):1719–27. doi:10.1172/JCI6560
6. Chu DK, Jimenez-Saiz R, Verschoor CP, Walker TD, Goncharova S, Llop-Guevara A, et al. Indigenous enteric eosinophils control DCs to initiate a primary Th2 immune response in vivo. J Exp Med (2014) 211(8):1657–72. doi:10.1084/jem.20131800
7. Chu VT, Beller A, Rausch S, Strandmark J, Zanker M, Arbach O, et al. Eosinophils promote generation and maintenance of immunoglobulin-A-expressing plasma cells and contribute to gut immune homeostasis. Immunity (2014) 40(4):582–93. doi:10.1016/j.immuni.2014.02.014
8. Carlens J, Wahl B, Ballmaier M, Bulfone-Paus S, Forster R, Pabst O. Common gamma-chain-dependent signals confer selective survival of eosinophils in the murine small intestine. J Immunol (2009) 183(9):5600–7. doi:10.4049/jimmunol.0801581
9. Jung Y, Rothenberg ME. Roles and regulation of gastrointestinal eosinophils in immunity and disease. J Immunol (2014) 193(3):999–1005. doi:10.4049/jimmunol.1400413
10. Mesnil C, Raulier S, Paulissen G, Xiao X, Birrell MA, Pirottin D, et al. Lung-resident eosinophils represent a distinct regulatory eosinophil subset. J Clin Invest (2016) 126(9):3279–95. doi:10.1172/JCI85664
11. Wu D, Molofsky AB, Liang HE, Ricardo-Gonzalez RR, Jouihan HA, Bando JK, et al. Eosinophils sustain adipose alternatively activated macrophages associated with glucose homeostasis. Science (2011) 332(6026):243–7. doi:10.1126/science.1201475
12. Rytömaa T. Organ distribution and histochemical properties of eosinophil granulocytes in rat. Acta Pathol Microbiol Scand Suppl (1960) 50:1–118.
13. Ross R, Klebanoff SJ. The eosinophilic leukocyte. Fine structure studies of changes in the uterus during the estrous cycle. J Exp Med (1966) 124(4):653–60. doi:10.1084/jem.124.4.653
14. Muller E. Localization of eosinophils in the thymus by the peroxidase reaction. Histochemistry (1977) 52(3):273–9. doi:10.1007/BF00495862
15. Throsby M, Herbelin A, Pleau JM, Dardenne M. CD11c+ eosinophils in the murine thymus: developmental regulation and recruitment upon MHC class I-restricted thymocyte deletion. J Immunol (2000) 165(4):1965–75. doi:10.4049/jimmunol.165.4.1965
16. Gouon-Evans V, Pollard JW. Eotaxin is required for eosinophil homing into the stroma of the pubertal and cycling uterus. Endocrinology (2001) 142(10):4515–21. doi:10.1210/endo.142.10.8459
17. Gouon-Evans V, Rothenberg ME, Pollard JW. Postnatal mammary gland development requires macrophages and eosinophils. Development (2000) 127(11):2269–82.
18. Farahi N, Singh NR, Heard S, Loutsios C, Summers C, Solanki CK, et al. Use of 111-Indium-labeled autologous eosinophils to establish the in vivo kinetics of human eosinophils in healthy subjects. Blood (2012) 120(19):4068–71. doi:10.1182/blood-2012-07-443424
19. Wen T, Besse JA, Mingler MK, Fulkerson PC, Rothenberg ME. Eosinophil adoptive transfer system to directly evaluate pulmonary eosinophil trafficking in vivo. Proc Natl Acad Sci U S A (2013) 110(15):6067–72. doi:10.1073/pnas.1220572110
20. Mochizuki M, Bartels J, Mallet AI, Christophers E, Schroder JM. IL-4 induces eotaxin: a possible mechanism of selective eosinophil recruitment in helminth infection and atopy. J Immunol (1998) 160(1):60–8.
21. Rothenberg ME. Eotaxin. An essential mediator of eosinophil trafficking into mucosal tissues. Am J Respir Cell Mol Biol (1999) 21(3):291–5. doi:10.1165/ajrcmb.21.3.f160
22. Ahrens R, Waddell A, Seidu L, Blanchard C, Carey R, Forbes E, et al. Intestinal macrophage/epithelial cell-derived CCL11/eotaxin-1 mediates eosinophil recruitment and function in pediatric ulcerative colitis. J Immunol (2008) 181(10):7390–9. doi:10.4049/jimmunol.181.10.7390
23. Waddell A, Ahrens R, Steinbrecher K, Donovan B, Rothenberg ME, Munitz A, et al. Colonic eosinophilic inflammation in experimental colitis is mediated by Ly6C(high) CCR2(+) inflammatory monocyte/macrophage-derived CCL11. J Immunol (2011) 186(10):5993–6003. doi:10.4049/jimmunol.1003844
24. Matthews AN, Friend DS, Zimmermann N, Sarafi MN, Luster AD, Pearlman E, et al. Eotaxin is required for the baseline level of tissue eosinophils. Proc Natl Acad Sci U S A (1998) 95(11):6273–8. doi:10.1073/pnas.95.11.6273
25. Daugherty BL, Siciliano SJ, DeMartino JA, Malkowitz L, Sirotina A, Springer MS. Cloning, expression, and characterization of the human eosinophil eotaxin receptor. J Exp Med (1996) 183(5):2349–54. doi:10.1084/jem.183.5.2349
26. Ponath PD, Qin S, Post TW, Wang J, Wu L, Gerard NP, et al. Molecular cloning and characterization of a human eotaxin receptor expressed selectively on eosinophils. J Exp Med (1996) 183(6):2437–48. doi:10.1084/jem.183.6.2437
27. Humbles AA, Lu B, Friend DS, Okinaga S, Lora J, Al-Garawi A, et al. The murine CCR3 receptor regulates both the role of eosinophils and mast cells in allergen-induced airway inflammation and hyperresponsiveness. Proc Natl Acad Sci U S A (2002) 99(3):1479–84. doi:10.1073/pnas.261462598
28. Ogilvie P, Bardi G, Clark-Lewis I, Baggiolini M, Uguccioni M. Eotaxin is a natural antagonist for CCR2 and an agonist for CCR5. Blood (2001) 97(7):1920–4. doi:10.1182/blood.V97.7.1920
29. Nussbaum JC, Van Dyken SJ, von Moltke J, Cheng LE, Mohapatra A, Molofsky AB, et al. Type 2 innate lymphoid cells control eosinophil homeostasis. Nature (2013) 502(7470):245–8. doi:10.1038/nature12526
30. Yamaguchi Y, Hayashi Y, Sugama Y, Miura Y, Kasahara T, Kitamura S, et al. Highly purified murine interleukin 5 (IL-5) stimulates eosinophil function and prolongs in vitro survival. IL-5 as an eosinophil chemotactic factor. J Exp Med (1988) 167(5):1737–42. doi:10.1084/jem.167.5.1737
31. Clutterbuck EJ, Hirst EM, Sanderson CJ. Human interleukin-5 (IL-5) regulates the production of eosinophils in human bone marrow cultures: comparison and interaction with IL-1, IL-3, IL-6, and GMCSF. Blood (1989) 73(6):1504–12.
32. Mould AW, Matthaei KI, Young IG, Foster PS. Relationship between interleukin-5 and eotaxin in regulating blood and tissue eosinophilia in mice. J Clin Invest (1997) 99(5):1064–71. doi:10.1172/JCI119234
33. Molofsky AB, Nussbaum JC, Liang HE, Van Dyken SJ, Cheng LE, Mohapatra A, et al. Innate lymphoid type 2 cells sustain visceral adipose tissue eosinophils and alternatively activated macrophages. J Exp Med (2013) 210(3):535–49. doi:10.1084/jem.20121964
34. Rosenberg HF, Dyer KD, Foster PS. Eosinophils: changing perspectives in health and disease. Nat Rev Immunol (2013) 13(1):9–22. doi:10.1038/nri3341
35. Jung Y, Wen T, Mingler MK, Caldwell JM, Wang YH, Chaplin DD, et al. IL-1beta in eosinophil-mediated small intestinal homeostasis and IgA production. Mucosal Immunol (2015) 8(4):930–42. doi:10.1038/mi.2014.123
36. Yu C, Cantor AB, Yang H, Browne C, Wells RA, Fujiwara Y, et al. Targeted deletion of a high-affinity GATA-binding site in the GATA-1 promoter leads to selective loss of the eosinophil lineage in vivo. J Exp Med (2002) 195(11):1387–95. doi:10.1084/jem.20020656
37. Abdala Valencia H, Loffredo LF, Misharin AV, Berdnikovs S. Phenotypic plasticity and targeting of Siglec-F(high) CD11c(low) eosinophils to the airway in a murine model of asthma. Allergy (2016) 71(2):267–71. doi:10.1111/all.12776
38. el-Cheikh MC, Borojevic R. Extramedullar proliferation of eosinophil granulocytes in chronic schistosomiasis mansoni is mediated by a factor secreted by inflammatory macrophages. Infect Immun (1990) 58(3):816–21.
39. Lee JJ, Jacobsen EA, Ochkur SI, McGarry MP, Condjella RM, Doyle AD, et al. Human versus mouse eosinophils: “that which we call an eosinophil, by any other name would stain as red”. J Allergy Clin Immunol (2012) 130(3):572–84. doi:10.1016/j.jaci.2012.07.025
40. Nerlov C, Graf T. PU.1 induces myeloid lineage commitment in multipotent hematopoietic progenitors. Genes Dev (1998) 12(15):2403–12. doi:10.1101/gad.12.15.2403
41. Hirasawa R, Shimizu R, Takahashi S, Osawa M, Takayanagi S, Kato Y, et al. Essential and instructive roles of GATA factors in eosinophil development. J Exp Med (2002) 195(11):1379–86. doi:10.1084/jem.20020170
42. Bettigole SE, Lis R, Adoro S, Lee AH, Spencer LA, Weller PF, et al. The transcription factor XBP1 is selectively required for eosinophil differentiation. Nat Immunol (2015) 16(8):829–37. doi:10.1038/ni.3225
43. Kim HJ, Alonzo ES, Dorothee G, Pollard JW, Sant’Angelo DB. Selective depletion of eosinophils or neutrophils in mice impacts the efficiency of apoptotic cell clearance in the thymus. PLoS One (2010) 5(7):e11439. doi:10.1371/journal.pone.0011439
44. Kopf M, Brombacher F, Hodgkin PD, Ramsay AJ, Milbourne EA, Dai WJ, et al. IL-5-deficient mice have a developmental defect in CD5+ B-1 cells and lack eosinophilia but have normal antibody and cytotoxic T cell responses. Immunity (1996) 4(1):15–24. doi:10.1016/S1074-7613(00)80294-0
45. Robertson SA, Mau VJ, Young IG, Matthaei KI. Uterine eosinophils and reproductive performance in interleukin 5-deficient mice. J Reprod Fertil (2000) 120(2):423–32. doi:10.1530/jrf.0.1200423
46. Leckie MJ, ten Brinke A, Khan J, Diamant Z, O’Connor BJ, Walls CM, et al. Effects of an interleukin-5 blocking monoclonal antibody on eosinophils, airway hyper-responsiveness, and the late asthmatic response. Lancet (2000) 356(9248):2144–8. doi:10.1016/S0140-6736(00)03496-6
47. Flood-Page PT, Menzies-Gow AN, Kay AB, Robinson DS. Eosinophil’s role remains uncertain as anti-interleukin-5 only partially depletes numbers in asthmatic airway. Am J Respir Crit Care Med (2003) 167(2):199–204. doi:10.1164/rccm.200208-789OC
48. Kips JC, O’Connor BJ, Langley SJ, Woodcock A, Kerstjens HA, Postma DS, et al. Effect of SCH55700, a humanized anti-human interleukin-5 antibody, in severe persistent asthma: a pilot study. Am J Respir Crit Care Med (2003) 167(12):1655–9. doi:10.1164/rccm.200206-525OC
49. Chu VT, Frohlich A, Steinhauser G, Scheel T, Roch T, Fillatreau S, et al. Eosinophils are required for the maintenance of plasma cells in the bone marrow. Nat Immunol (2011) 12(2):151–9. doi:10.1038/ni.1981
50. Sugawara R, Lee EJ, Jang MS, Jeun EJ, Hong CP, Kim JH, et al. Small intestinal eosinophils regulate Th17 cells by producing IL-1 receptor antagonist. J Exp Med (2016) 213(4):555–67. doi:10.1084/jem.20141388
51. Chen HH, Sun AH, Ojcius DM, Hu WL, Ge YM, Lin X, et al. Eosinophils from murine lamina propria induce differentiation of naive T cells into regulatory T cells via TGF-beta1 and retinoic acid. PLoS One (2015) 10(11):e0142881. doi:10.1371/journal.pone.0142881
52. Lumeng CN, Bodzin JL, Saltiel AR. Obesity induces a phenotypic switch in adipose tissue macrophage polarization. J Clin Invest (2007) 117(1):175–84. doi:10.1172/JCI29881
53. Enerback S. Human brown adipose tissue. Cell Metab (2010) 11(4):248–52. doi:10.1016/j.cmet.2010.03.008
54. Qiu Y, Nguyen KD, Odegaard JI, Cui X, Tian X, Locksley RM, et al. Eosinophils and type 2 cytokine signaling in macrophages orchestrate development of functional beige fat. Cell (2014) 157(6):1292–308. doi:10.1016/j.cell.2014.03.066
55. Suarez-Zamorano N, Fabbiano S, Chevalier C, Stojanovic O, Colin DJ, Stevanovic A, et al. Microbiota depletion promotes browning of white adipose tissue and reduces obesity. Nat Med (2015) 21(12):1497–501. doi:10.1038/nm.3994
56. Fabbiano S, Suarez-Zamorano N, Rigo D, Veyrat-Durebex C, Stevanovic Dokic A, Colin DJ, et al. Caloric restriction leads to browning of white adipose tissue through type 2 immune signaling. Cell Metab (2016) 24(3):434–46. doi:10.1016/j.cmet.2016.07.023
57. Lucas FV, Neufeld HA, Utterback JG, Martin AP, Stotz E. The effect of estrogen on the production of a peroxidase in the rat uterus. J Biol Chem (1955) 214(2):775–80.
58. Salamonsen LA, Woolley DE. Menstruation: induction by matrix metalloproteinases and inflammatory cells. J Reprod Immunol (1999) 44(1–2):1–27. doi:10.1016/S0165-0378(99)00002-9
59. Lee JJ, Dimina D, Macias MP, Ochkur SI, McGarry MP, O’Neill KR, et al. Defining a link with asthma in mice congenitally deficient in eosinophils. Science (2004) 305(5691):1773–6. doi:10.1126/science.1099472
60. Colbert DC, McGarry MP, O’Neill K, Lee NA, Lee JJ. Decreased size and survival of weanling mice in litters of IL-5-/- mice are a consequence of the IL-5 deficiency in nursing dams. Contemp Top Lab Anim Sci (2005) 44(3):53–5.
61. Ding C, Li J, Zhang X. Bertilimumab Cambridge Antibody Technology Group. Curr Opin Investig Drugs (2004) 5(11):1213–8.
62. Kiwamoto T, Kawasaki N, Paulson JC, Bochner BS. Siglec-8 as a drugable target to treat eosinophil and mast cell-associated conditions. Pharmacol Ther (2012) 135(3):327–36. doi:10.1016/j.pharmthera.2012.06.005
63. Fulkerson PC, Rothenberg ME. Targeting eosinophils in allergy, inflammation and beyond. Nat Rev Drug Discov (2013) 12(2):117–29. doi:10.1038/nrd3838
64. Bochner BS. “Siglec”ting the allergic response for therapeutic targeting. Glycobiology (2016) 26(6):546–52. doi:10.1093/glycob/cww024
65. Rothenberg ME. Humanized anti-IL-5 antibody therapy. Cell (2016) 165(3):509. doi:10.1016/j.cell.2016.04.020
Keywords: eosinophils, homeostasis, immunomodulation, mucosae, innate immunity
Citation: Marichal T, Mesnil C and Bureau F (2017) Homeostatic Eosinophils: Characteristics and Functions. Front. Med. 4:101. doi: 10.3389/fmed.2017.00101
Received: 31 March 2017; Accepted: 26 June 2017;
Published: 11 July 2017
Edited by:
Florence Emmanuelle Roufosse, Free University of Brussels, BelgiumReviewed by:
Josiane Sabbadini Neves, Federal University of Rio de Janeiro, BrazilWei Li, Marshall University, United States
Copyright: © 2017 Marichal, Mesnil and Bureau. This is an open-access article distributed under the terms of the Creative Commons Attribution License (CC BY). The use, distribution or reproduction in other forums is permitted, provided the original author(s) or licensor are credited and that the original publication in this journal is cited, in accordance with accepted academic practice. No use, distribution or reproduction is permitted which does not comply with these terms.
*Correspondence: Thomas Marichal, dC5tYXJpY2hhbEB1bGcuYWMuYmU=;
Fabrice Bureau, ZmFicmljZS5idXJlYXVAdWxnLmFjLmJl