- 1Renewable Energy Research Center, Islamic Azad University, Damavand, Iran
- 2Renewable Energy Research Center, Islamic Azad University, Damavand, Iran
This study presents a comprehensive investigation into the simultaneous use of convection and radiation heat transfer for Iranian pistachio drying using a catalytic heater. Both experimental and numerical analyses were conducted to evaluate the performance of the proposed system. The experimental setup involved a catalytic infrared heater, which provided a consistent temperature of approximately 370 K within the drying chamber. The results indicated that the drying time was reduced by 40% compared to traditional hot air drying methods, with an average moisture reduction rate of 0.8% per minute. The numerical analysis, conducted using computational fluid dynamics (CFD) simulations, supported the experimental findings, showing a uniform temperature distribution across the pistachios and efficient heat transfer. The catalytic heater demonstrated a combustion efficiency of 98%, with CO emissions below 10 ppm and zero NOx emissions, highlighting its environmental benefits. Overall, the combined experimental and numerical results confirmed that the proposed catalytic heater system not only enhances drying efficiency but also significantly reduces energy consumption and greenhouse gas emissions compared to conventional drying methods.
1 Introduction
Pistachios are a tree fruit that grow in countries such as Iran, United States, Turkey, China, and Syria. Statistics show that the worldwide consumption of pistachios is approximately 600–700 thousand tons per year. Currently, there is a close competition between Iran and United States to supply the global market (Aad et al., 2012). A critical review of the existing pistachio drying methods is essential to contextualize the significance of the proposed catalytic infrared drying system. Various traditional and modern drying methods have been employed in the pistachio industry, each with its own advantages and limitations. The most common methods include sun drying, hot air drying, and gas-fired drying systems. Sun drying is one of the oldest methods used for pistachios. While it is cost-effective and does not require any external energy input, it is highly dependent on weather conditions, making it an unreliable method. The extended drying time, often spanning several days, can result in uneven drying and contamination due to exposure to dust, insects, and other environmental factors. Additionally, sun drying poses a risk of aflatoxin contamination, which is a significant concern in food safety. Hot air drying is a widely used method in commercial pistachio processing. It involves circulating hot air through the drying chamber to remove moisture from the pistachios. This method is more controlled than sun drying, allowing for a consistent drying environment. However, it is energy-intensive, often resulting in higher operational costs and greater environmental impact due to greenhouse gas emissions. The high temperatures required can also lead to thermal degradation of the pistachios, affecting their flavor, texture, and nutritional content. Gas-fired drying systems utilize natural gas or other fossil fuels to generate heat for the drying process. While these systems offer faster drying times compared to hot air and sun drying methods, they are associated with significant greenhouse gas emissions, including CO2, CH4, and NOx. The combustion process in these systems is often less efficient, leading to incomplete fuel utilization and higher pollutant emissions. Moreover, the high operating temperatures can cause uneven drying and potential scorching of the pistachios, further compromising product quality. While traditional drying methods such as sun drying, hot air drying, and gas-fired systems have been widely used, they each present significant drawbacks in terms of energy efficiency, environmental impact, and product quality. The catalytic infrared drying system introduced in this study represents a significant advancement in pistachio drying technology. By addressing the limitations of existing methods, it offers a more efficient, environmentally friendly, and high-quality drying process. The adoption of this technology has the potential to revolutionize the pistachio processing industry, making it more sustainable and economically viable.
The nutritional benefits of pistachios have contributed to their increased consumption in both raw and cooked forms across various regions worldwide (Jahanbakhshi et al., 2020). Iran’s pistachio has excellent quality owing to favorable climatic conditions. To ensure its preservation and to prevent any deterioration in quality, drying of the pistachio is necessary before exporting it. This approach guarantees easy storage and preservation of its taste and quality. During the drying process of pistachios, the moisture content reduces from approximately 59%–67% on a dry basis to 5%–6%, resulting in increased storage capacity (Maskan and Karataş, 1998). If drying is not conducted properly and the factors that affect it, such as temperature, flow rate, and relative humidity, are not controlled correctly, it can decrease the final product’s quality and prepare conditions for the activity of aflatoxin-producing molds, ultimately leading to an increase in pollution levels (Fooladi et al., 2006). Drying involves a process of mass and heat transfer, where a solvent such as water is evaporated from a solid material. This process varies depending on the material and solvent used. The drying process typically occurs during the final stages of production and after other operations, ultimately resulting in the creation of the finished product (Chen and Pan, 2020). The main objective of the drying process is to generate a dried product with ideal quality, minimal cost, and maximum efficiency (Chatrchyan et al., 2012). The high biological quality of the product is often the outcome of various physical, chemical, and biological transformations. The drying process in developed countries represents approximately 15% of industrial energy consumption (Chua et al., 2001). This amount increases to approximately 25%–50% of the total energy consumption in underdeveloped countries with greater energy waste. In order to decrease the amount of energy used per unit of the product produced, it is necessary to utilize various techniques for drying the products and also to enhance the energy and thermal efficiency of the dryer, as suggested by prior research (Ogura et al., 2005). Today, the emphasis on product quality has increased due to the development and globalization of markets. Customers prioritize high-quality manufactured products. Therefore, the development of equipment that aligns with environmental concerns, energy policies, and customer satisfaction has been taken into account (He, 2008). One of the most recent combustion technologies that can be utilized in the pistachio drying process are catalytic heaters. These heaters do not possess a flame; hence, they distribute temperature uniformly and have excellent thermal efficiency. The use of catalytic heaters can reduce energy consumption during the drying process. Additionally, these heaters can contribute to a favorable drying process outcome because of their ability to provide uniform temperature distribution (Hosseinalipour, 2014; Zanfir and Gavriilidis, 2003; Specchia et al., 2004; Abano et al., 2014).
Solar drying harnesses solar energy to remove moisture from food products, offering an eco-friendly and cost-effective solution. Recent studies have explored the optimization of solar drying techniques to improve efficiency and product quality. Ayadi et al. (Ayadi et al., 2021) examined the impact of air drying conditions on pomegranate arils, highlighting the significance of uniform heat distribution in maintaining quality. Microwave drying is renowned for its ability to significantly reduce drying time and energy consumption while preserving product quality. Can and Kirmaci (Can and Kirmaci, 2021) optimized microwave-assisted drying of apricot, showcasing the benefits of combining solar and microwave drying. Hybrid drying methods, which combine microwave and air drying, have shown promising results in optimizing energy use and product quality. Dagdug et al. (Dagdug et al., 2022) analyzed fruit and vegetable drying using hybrid methods, emphasizing enhanced efficiency and quality. Infrared drying employs infrared radiation to directly heat the surface of the product, offering rapid and uniform drying. Studies have demonstrated the effectiveness of infrared drying in maintaining the nutritional and sensory qualities of dried products. Jafari and Mahdavi (Jafari and Mahdavi, 2021) optimized drying parameters for lemon slices, illustrating how precise control can enhance product quality. Freeze drying, or lyophilization, involves freezing the product and then sublimating the ice under low pressure, preserving the structural integrity and nutritional content of the food. Recent advancements in freeze drying techniques have focused on improving energy efficiency and reducing processing time. Air drying is a traditional method widely used for drying fruits and vegetables. Karim and Hawlader (2021) investigated the drying kinetics of guava and papaya, focusing on the impact of air drying temperature on the quality of the dried products (Karim and Hawlader, 2021). Their study revealed that higher air drying temperatures significantly reduced drying time but adversely affected the quality attributes, such as color and texture, of the dried fruits. The findings underscore the importance of optimizing air drying conditions to balance drying efficiency and product quality.
The design and application of efficient drying systems are essential for enhancing food preservation while minimizing energy consumption. Lewicki (2022) discussed innovative designs and strategies for efficient drying systems in the food industry (Lewicki, 2022). The study emphasized the need for systems that ensure uniform heat distribution and optimal drying conditions to maintain the quality of the dried products. The development of such systems is crucial for reducing energy usage and improving the sustainability of drying processes. Microwave drying is known for its rapid drying capabilities and energy efficiency. Motevali, Minaei, and Ghobadian (2021) conducted a comprehensive study on the energy consumption and mathematical modeling of microwave drying of apple slices (Motevali et al., 2021). Their research demonstrated that microwave drying significantly reduces drying time compared to conventional methods. However, precise control of microwave power is necessary to avoid quality degradation. The study provides valuable insights into the optimization of microwave drying parameters to enhance both energy efficiency and product quality. Maintaining the nutritional quality of dried fruits and vegetables is a major concern in drying processes. Nguyen and Price (2021) examined the effects of various drying methods on the retention of essential nutrients in fruits and vegetables (Nguyen and Price, 2021). Their findings indicated that drying methods involving lower temperatures and shorter drying times, such as microwave drying, were more effective in preserving nutrients compared to high-temperature air drying. This highlights the importance of selecting appropriate drying techniques to ensure the nutritional integrity of dried products. Comparative studies on different drying methods provide insights into the advantages and limitations of each technique. Ruiz-López and Morales-Castro (2021) conducted a comparative study on the drying kinetics and quality of mango slices using various drying methods (Ruiz-López and Morales-Castro, 2021). Their research showed that methods like microwave and hybrid drying not only shortened drying times but also better preserved the sensory and nutritional qualities of mango slices compared to traditional air drying. Such comparative analyses are crucial for identifying the most effective drying techniques for different fruits and vegetables. The influence of drying temperatures on the physicochemical properties of food products is a critical area of research. Vega-Gálvez et al. (2021) studied the impact of drying temperatures on the physicochemical and antioxidant properties of red pepper (Vega-Gálvez et al., 2021). Their results indicated that lower drying temperatures better preserved the antioxidant properties and overall quality of red pepper. This study underscores the need to carefully control drying temperatures to maintain the health benefits and quality of dried products. Zhang et al., (2021) reviewed the latest trends in microwave-related drying of fruits and vegetables (Zhang et al., 2021). Their review highlighted the growing interest in microwave drying due to its efficiency and ability to maintain product quality. Advances in microwave drying technology, such as the development of hybrid systems combining microwave and conventional methods, were also discussed. These innovations aim to further enhance the drying process’s effectiveness and sustainability. Low-temperature drying is another technique that offers significant benefits in preserving the quality of dried products. Zhu et al., (2021) investigated the quality changes in cherry tomatoes during low-temperature drying (Zhu et al., 2021). Their study found that low-temperature drying effectively retained the color, texture, and nutritional content of cherry tomatoes. This method is particularly advantageous for drying heat-sensitive fruits and vegetables, ensuring high-quality dried products with extended shelf life.
One of the key environmental advantages of using a catalytic heater for pistachio drying is its significantly lower greenhouse gas emissions compared to conventional methods such as hot air dryers or traditional combustion systems. The catalytic combustion process is highly efficient, achieving nearly 98% combustion efficiency. Unlike conventional gas burners, which produce notable quantities of nitrogen oxides (NOx), carbon monoxide (CO), and other harmful pollutants, the catalytic heater operates at a lower combustion temperature. This results in a complete elimination of NOx emissions and a drastically reduced level of CO emissions, which remains below 10 ppm. The primary emissions from the catalytic heater are water vapor and CO2, both of which are significantly reduced due to the higher efficiency of the system. In contrast, traditional drying systems, such as hot air ovens or gas-fired dryers, typically operate at much higher combustion temperatures, leading to increased emissions of CO2, NOx, and other greenhouse gases. These systems often exhibit lower overall energy efficiency, meaning they consume more fuel and produce more emissions per kilogram of dried pistachios. The catalytic heater not only reduces emissions but also offers substantial energy savings. Compared to classical hot air drying methods, the catalytic heater reduces energy consumption by approximately 40%. This reduction is primarily due to the resonant heating effect, where the emitted infrared radiation matches the natural frequencies of the pistachio’s water molecules, leading to faster and more efficient drying. Consequently, less energy is required to achieve the desired moisture content in the pistachios. Overall, the adoption of catalytic infrared heating represents a significant step forward in sustainable pistachio drying, offering both environmental and economic benefits. The reduced greenhouse gas emissions and lower energy consumption make it a more favorable option compared to traditional methods, particularly in regions where energy conservation and emission reductions are prioritized.
Catalytic radiant heaters offer significant advantages in optimizing energy consumption and maintaining the quality of the final product. These heaters provide uniform heat distribution, reducing the risk of hotspots and uneven drying. The radiant heat penetrates the product more effectively, ensuring thorough drying with minimal energy usage. Additionally, catalytic heaters operate at lower temperatures compared to traditional heaters, preserving the nutritional and sensory qualities of the product. Overall, the use of catalytic radiant heaters in pistachio drying enhances the efficiency of the process, reduces energy costs, and ensures high-quality dried pistachios with extended shelf life and improved safety. Due to the insufficient research in pistachio drying using catalytic heaters, this study employs an experimental and numerical approach to address the effects of this method for the first time. A pistachio dryer with a catalytic heater was designed and constructed for this purpose. The experimental results derived from this study have been used to validate the numerical simulation outputs, serving as an additional aim of this investigation. Another objective of this study is to decrease the energy usage needed for pistachio drying while preserving the quality of the end product.
The primary objective of this research is to develop and evaluate a novel pistachio drying system that leverages the combined effects of convection and radiation heat transfer using a catalytic infrared heater. The study aims to address two critical challenges in the agricultural drying industry: improving energy efficiency and reducing greenhouse gas emissions. Specifically, the research focuses on optimizing the drying process for Iranian pistachios, which are a significant agricultural product, by minimizing energy consumption, reducing drying time, and maintaining product quality. The research also seeks to validate the effectiveness of the catalytic heater system through both experimental and numerical approaches. The experimental component is designed to measure key performance indicators, such as drying time, moisture reduction rate, and energy consumption, while the numerical simulations provide a detailed analysis of heat transfer dynamics and temperature distribution within the drying chamber. The technical novelty of this study lies in the integration of catalytic infrared heating technology with traditional convection-based drying methods for pistachios. Another innovative aspect of the research is the use of resonant heating effects, which enhance moisture removal efficiency. The catalytic heater emits infrared radiation in a specific frequency range that resonates with the moisture content in the pistachios, accelerating the drying process and improving energy transfer efficiency. This approach not only shortens the drying time but also reduces the thermal stress on the pistachios, helping to preserve their quality. Moreover, the study’s combination of experimental validation and numerical simulation offers a robust framework for analyzing and optimizing the drying process. The computational fluid dynamics (CFD) simulations provide insights into the uniformity of temperature distribution and the behavior of heat transfer within the drying chamber, which are critical for ensuring consistent product quality and process reliability. In summary, this research introduces a pioneering approach to pistachio drying that combines advanced catalytic infrared heating with conventional convection techniques, resulting in a highly efficient, environmentally friendly, and technically superior drying system. The findings have significant implications for the agricultural industry, offering a scalable solution that can be adapted to other crops and drying processes.
2 Experimental setup
The drying system, equipped with a catalytic heater, comprises five essential components: a hydraulic jack, a load cell, a sample tray, a catalytic heater, and a drying chamber. The catalytic radiant heater utilized is the FCS-2ft, produced by the Aria Sarmad Company. The panel’s surface measures 80 × 80 cm and has a production power of 3.6 kW, which is equivalent to 3,097 kcal/h. The heater’s gas consumption is 0.336 m3/h. It should be noted that there are several thermal elements within the heater that are utilized during the initial start-up. The power necessary for starting uses 486 W and necessitates a current of 2.5 A. Finally, the radiant heater has a weight of 4 kg and comes with accompanying bases for installation. In Figure 1, a view of the fan-type heater utilized in the present study is presented. Also, Figure 2 displays a schematic diagram of the constructed catalytic dryer.
In this study, we utilized fans with variable rotation speed under various test conditions as the rotating air speed was one of the examined criteria. The drying chamber is a rectangular cube measuring 65 × 40 × 120 cm and constructed from 0.5 cm thick stainless steel (specifically stainless steel 304). The chamber’s outer wall is insulated with glass wool in order to minimize energy losses. The drying chamber contains a fixed glass window measuring 20 by 60 cm, positioned 30 cm from the chamber floor. This stationary window enables observation of the drying process within the drying chamber. The window’s glass is double-glazed in order to prevent heat loss. It is important to note that the front panel of the dryer is considered a door, mounted on two hinges for complete opening of the compartment. It is important to consider this door for assembling heaters, hydraulic jacks, and gaining access to various components in the dryer chamber. It is important to note that the proposed design for the radiant dryer takes into account the limitations of the heater, such as wire length, natural gas inlet pipe, and weight. As a result, the position of the radiant heater is fixed and can only be rotated and adjusted to different angles. On the other hand, the position of the drying bed is considered movable to adjust the distance between the heater and the drying bed. In Figure 3, a schematic view of the hydraulic jack set, load cell, and sample tray is presented. It should be noted that the samples’ surface temperature is measured using a thermocouple included in the radiant heater package. The thermocouple display provides the option to read measured values, or data can be recorded at any time by connecting it to a computer.
The sample tray is constructed from aluminum due to its lightweight nature, resistance to temperature, and corrosion prevention. As a result, it preserves the quality of samples without any alterations. Moreover, the high thermal conductivity coefficient of the aluminum tray results in a nearly uniform temperature distribution without creating a temperature gradient. The dimensions of the flat portion of the tray are 56 cm in length and 30 cm in width. The tray’s edges have a height of 3 cm and are positioned at a 45-degree angle to its length. Finally, considering the tray’s edges, the overall length is approximately 60 cm. The edges mainly exist to prevent the deviation and waste of infrared radiations, which has been taken into account based on previous research recommendations. The drying tray has a thickness of 0.5 cm. The tray is affixed at its center onto a load cell with a capacity of 5 kg and a measurement accuracy of 0.1 g. Load cells are manufactured in multiple dimensions, shapes, and accuracies by companies specializing in precision instruments. For this research, it is not necessary to utilize a load cell capable of measuring high weights since measurement accuracy is crucial. Finally, the load cell is bolted to the hydraulic jack. The hydraulic jack is capable of adjusting height with 1 cm precision. There is no need for a high weight bearing jack; rather, it is sufficient that the height adjustment is accurate. The hydraulic jack has bases screwed at its center to the bottom of the dryer chamber. Figure 4 shows an overview of the designed pistachio catalytic dryer. The catalytic heater is located on the top of the device.
A specific speed is employed for the selection of the blower. This technique is widely used for choosing blowers. The criterion is determined using the following formula.
In Equation 1,
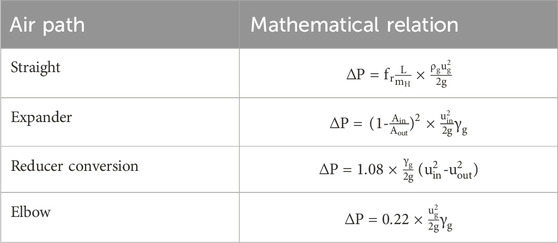
Table 1. Mathematical formulas for calculating pressure drop across different sections of the air duct system.
2.1 Test conditions and sample preparation
After eliminating damaged, crushed, surface flawed, overly large or small samples, freshly sorted pistachios were prepared in 100 gr samples as shown in Figure 5. The samples are placed into the drying heater’s dedicated tray. All tests were conducted at three temperatures: 40, 50, and 60°C; three blowing air velocities: 0.5, 1, and 1.5 m/s; and three radiation power levels: 2000, 2,400, and 2800 W/m2, equivalent to 500, 600, and 700 W. Two modes without return air and with 100% return air were studied. The air blowing speed values have been selected to prevent dislodging or disrupting the arrangement of the dried product on the bed.
The samples’ volume was determined using the rapeseed displacement method. The sample was weighed and then placed into a container with a specific volume (
The stability of the color in the dried sample can be measured quantitatively using L*, a*, and b* values, as well as the CIE color difference value (∆E), hue angle, and chroma values (C*). Changes in chroma indicate the distorted estimation of the prevalent color components (a* or b*). Chroma is the objective measure of color intensity, with a tendency towards the dominant color. The color intensity (chroma) and dominant color (hue angle) of the fresh sample can be calculated using Equations 3–5. Differences in the values indicate changes in color compared to the fresh sample. Color intensity (chroma) is an indication of color saturation. The hue angle being closer to zero indicates a stronger presence of red in the sample.
3 Mathematical modelling
In this section, we present the mathematical modeling for the drying process of pistachios using catalytic radiant heaters. The equations governing mass, momentum, and energy conservation in three dimensions are introduced. The finite element method (FEM), implemented through the COMSOL Multiphysics package, is used to solve these equations. The mathematical model developed to predict the drying process of pistachios under radiation and convection is based on Fourier’s law of conduction and Fick’s second law. The problem is treated as three-dimensional, and the distribution of flow, temperature, and moisture is computed throughout the entire computational domain in three dimensions. The model is simplified based on logical assumptions. These assumptions include homogeneous initial temperature and moisture content in the sample, negligible deformation of the sample during drying, and no internal heat generation within the sample. Figure 6 illustrates the schematic of the computational domain and the 3D model of the catalytic dryer. As shown in Figure 6, the dryer consists of an air inlet section, a catalytic heater, a bed for sample placement, and a hydraulic jack. Air is introduced into the system from the top of the dryer by a fan. After passing alongside the catalytic heater, the air is heated. The heated air then passes over the surface of the samples and exits through an outlet on the chamber wall. Additionally, the catalytic heater delivers radiant heat to the samples according to its capacity. In this type of dryer, radiation and convection mechanisms operate in combination. The control parameters in this dryer include the incoming air velocity, radiant power, and the distance of the sample from the heater surface.
The following assumptions are made in the mathematical model.
a) Heat conduction in the vertical direction is considered, while convective heat transfer in this direction is negligible.
b) The dryer walls are thermally insulated.
c) Pistachio particles do not adhere to each other and do not break or fragment.
d) Complete mixing is assumed, meaning that the position of each particle does not affect the drying rate.
e) Initial temperature and moisture content are uniform across all particles.
f) The gas film around the particles is in thermal and moisture equilibrium with the particles during drying.
g) No condensation occurs within the dryer.
Base of above assumptions, the conservation of mass for the fluid phase is governed by the continuity Equation 6 where
The Navier-Stokes Equation 7 describe the conservation of momentum in three dimensions where p is pressure,
The energy Equation 8, accounting for heat transfer due to conduction, convection, and the latent heat of moisture evaporation, is:
In above equation
The governing Equation 9 for the simulation include heat and mass transfer in both the solid and gas phases. A volume element is considered to derive the governing equations, and the mass transfer within the particles is assumed to be one-dimensional in the thickness direction. Therefore, the moisture content in the solid phase is calculated macroscopically as follows:
Boundary and initial conditions for the above equation are: Initial temperature and moisture content are uniform (Equation 10):
Boundary conditions are as Table 2:
Given that the equilibrium moisture content in the gas and solid phases is equal, the equilibrium moisture content of the solid phase can be calculated using the GAB Equation 11 proposed by Kiranoudis:
Equations 12–15 have been developed to calculate the mass transfer coefficient. The Ozieh equation, which is used in this study, is one of the most significant.
The moisture diffusivity coefficient depends on moisture content and temperature and can be calculated using experimental data. For pistachio samples, the following empirical Equation 16 is provided:
The density of the particles during the drying process is calculated using Equation 17. For
To calculate the moisture distribution in the solid phase microscopically, the following Equations 18, 19 are used:
Boundary and initial conditions for the above equation are:
The thermal conductivity of solid particles is calculated using the following empirical Equation 20:
The specific heat capacity of solid particles is calculated using the following empirical Equation 21:
The latent heat of evaporation of solid particles is calculated as Equation 22:
The convective heat transfer coefficient of the gas phase can be obtained using the analogy between mass and heat transfer and the following Equation 23:
The term QIR represents the volumetric heat flux dependent on the radiative properties of the drying material and the power of the catalytic heater. It is calculated using radiative transfer laws, including Wien’s displacement law, Stefan-Boltzmann law, and Lambert’s law. According to previous research, the heat generated per unit volume of the drying material is calculated using Lambert’s law (Equation 24) as follows:
Given that the Finite Element Method (FEM) is employed for discretizing the equations, it is essential to elaborate on the mesh generation process, discretization, and solution methodology. The 3D model and computational domain of the catalytic dryer include several key components: the air inlet section, the catalytic heater, the bed for sample placement, and the hydraulic jack. The air is introduced into the system from the top of the dryer by a fan, passes alongside the catalytic heater to be heated, and then flows over the sample surface, exiting through an outlet on the chamber wall. The catalytic heater provides radiant heat to the samples. The mesh generation for this complex domain involves creating a finely detailed grid that accurately represents the geometry and allows for precise numerical solutions (Table 3). The mesh is generated using tetrahedral or hexahedral elements, depending on the complexity and requirements of the domain. The mesh density is higher in regions with expected high gradients of temperature, moisture, or velocity, such as near the catalytic heater and the sample surfaces. This ensures that the numerical solution captures the critical variations in these parameters. To obtain accurate results while minimizing computational time and costs, the impact of different numbers of elements on the simulation outcomes must be examined. In this study, it was found that increasing the number of computational elements beyond 500,000 did not significantly alter the heat transfer field characteristics. Therefore, this number of elements was used in all analyses to balance accuracy and computational efficiency. The orthogonally parameter, which should be between zero and one, indicates mesh quality. A value closer to zero signifies better quality. In the current mesh, the orthogonally parameter is 0.02348, indicating high-quality meshing.
4 Experimental results and analysis
The pistachio drying system under study integrates two main components: a catalytic heater and a hydraulic jack, each with distinct energy inputs and roles in the overall drying process.
The hydraulic jack used in this system is powered by electricity. This component is primarily responsible for adjusting the positioning of the pistachio trays within the drying chamber, ensuring optimal exposure to the infrared radiation emitted by the catalytic heater. The energy consumption of the hydraulic jack is relatively minimal and is largely independent of the drying process itself. Therefore, its contribution to the overall energy balance of the system is negligible. The catalytic heater, which operates using natural gas, is the primary source of energy for the drying process. The heater generates infrared radiation through a highly efficient catalytic combustion process, achieving an impressive combustion efficiency of approximately 98%. This efficient conversion of natural gas to radiant heat minimizes energy losses and maximizes the transfer of heat directly to the pistachios. In this study, an energy balance was conducted to evaluate the efficiency of the drying system. However, it is important to note that the energy consumed by the hydraulic jack was not included in the energy balance calculations due to its minimal impact on the overall energy consumption of the system. The energy balance focused primarily on the catalytic heater, where the input energy from the natural gas was compared to the energy required to evaporate moisture from the pistachios and achieve the desired drying effect. The results indicated that the catalytic heater system is highly energy-efficient, with significant energy savings compared to conventional drying methods. The resonant heating effect, caused by the specific frequency range of the emitted infrared radiation, enhances the efficiency of the drying process by reducing the time and energy required to remove moisture from the pistachios. In conclusion, the energy balance performed for the system underscores the effectiveness of the catalytic heater in optimizing energy usage, while the energy consumption of the hydraulic jack remains insignificant in the context of the overall system operation.
Various tests were conducted to investigate the impact of different operating parameters on material shrinkage during drying. The changes in the (V/V0) value at different humidity levels were measured over time in each test. According to the experimental results, the alterations in (V/V0) that occur during the drying period of pistachio samples are a linear function of the moisture content. The equation defining this relationship is as follows (Equation 25): View less
In the given equation, A and B represent constant values that were determined by testing the samples. The specific values for A and B are provided in Table 4.
Figure 7 displays the process of sample preparation in the laboratory. Three types of samples were analyzed at the Atiyeh Alborz laboratory: pistachio kernels, pistachio slices, and a combination of pistachio slices and dried shell made with the machine. The special tests performed included hygrometry, fat measurement, peroxide value, powder, aflatoxin B1, aflatoxin B2, aflatoxin G1, aflatoxin G2, and a combination of aflatoxin and plant pesticides. The findings from these tests are presented in Tables 5, 6. Based on the results presented in the reports above, it is evident that drying pistachio kernels, slices, and shells using the dryer developed in this study yields excellent health benefits and quality.
The catalytic radiant heater used in this study offers several advantages in optimizing energy consumption and maintaining the quality of the final product. Catalytic radiant heaters are highly energy-efficient as they directly transfer heat to the product through radiation, minimizing energy loss. This direct heating mechanism ensures that the energy is utilized effectively, leading to lower energy consumption compared to conventional dryers. The gentle and uniform heating provided by catalytic radiant heaters helps in preserving the quality of the pistachios. It minimizes the risk of overheating and degradation of essential nutrients and compounds within the pistachios. As a result, the final product retains its nutritional value, flavor, and overall quality. The efficient heat transfer mechanism reduces the overall drying time, which not only enhances the throughput but also contributes to the reduction of energy usage. This is particularly beneficial for large-scale industrial drying processes. The even distribution of heat ensures consistent drying of the pistachios, reducing the variability in moisture content and quality across different batches.
5 Numerical results and analysis
The validation of the numerical results was conducted by comparing the simulation data with the experimental results, as shown in Figure 8. The close agreement between the numerical and experimental results demonstrates the accuracy and reliability of the simulation model. Each chart includes both the experimental and numerical data, providing a clear comparison that validates the numerical model. The minor deviations observed are within acceptable error margins, reinforcing the validity of the simulation approach. This validation process confirms that the numerical model can accurately predict the drying behavior under various conditions, ensuring that the optimized parameters identified (bed height of 48 cm, heater power of 5,000 W, and air velocity of 0.5 m/s) are effective for achieving high-quality drying of pistachios.
The velocity distribution profile throughout the system is shown in Figure 9. The high-pressure areas are the inlet and outlet regions. As observed, the hot air flow interacts with the tray surface in a swirling manner, leading to fluid convection. This phenomenon increases the contact time between the hot air and the moist surface of the pistachios, resulting in faster drying of the product. Consequently, we tested four different inlet velocities to determine the optimal one, which is evident in the subsequent charts. The comparison of both distributions indicates more turbulent flow at higher velocities. Higher turbulence levels at increased velocities facilitate better heat and mass transfer, contributing to more efficient drying. However, excessively high velocities might lead to non-uniform drying and potential mechanical damage to the product. Therefore, finding an optimal velocity is crucial for ensuring uniform and gentle drying, preserving the quality of the pistachios.
As shown in Figure 10, the highest moisture content is in the center of the pistachio, and as we move towards the outer shells, the moisture contents decreases and the temperature increases. The temperature and heat distribution in the product, tested over 45 min, are depicted in the following contours. Uniform moisture and temperature distribution are vital for ensuring consistent drying. Uneven drying can lead to quality issues such as texture changes, loss of nutritional value, and decreased shelf life. The contours illustrate the effectiveness of the drying process in achieving a balanced moisture and temperature profile within the product.
Figure 10 of the manuscript shows that the temperature within the drying chamber reaches approximately 370 K (97°C). This temperature was selected based on the specific drying requirements for pistachios. At this temperature, a significant portion of the moisture content in the pistachios will indeed evaporate. However, it is essential to note that complete moisture removal is not the objective. The goal is to reduce the moisture content to a level that ensures product stability during storage while preserving the quality of the pistachios. The target moisture content for dried pistachios typically ranges between 4% and 6%. This level of dehydration is sufficient to inhibit microbial growth and enzymatic activity, thus extending the shelf life of the product. The temperature of 370 K was determined to be optimal for achieving the desired moisture content in a reasonable time while preventing any adverse effects on the pistachios’ texture, flavor, and nutritional properties. Moisture loss during the drying process was monitored at periodic intervals to ensure that the drying process proceeded as intended. Samples of pistachios were collected from the drying chamber at regular intervals, and their moisture content was measured using a gravimetric method. This involved weighing the samples before and after drying them in an oven at 105°C until a constant weight was achieved. The difference in weight before and after oven drying allowed us to calculate the moisture content at each interval. By conducting these measurements at regular intervals, we were able to track the rate of moisture loss and ensure that the pistachios were not over-dried, which could lead to quality degradation. The data collected also allowed us to fine-tune the drying process to achieve the most efficient balance between drying time and energy consumption. In summary, the temperature of 370 K was chosen based on the specific requirements for pistachio drying, and the moisture content was carefully monitored at periodic intervals to ensure optimal drying without compromising the quality of the product.
To optimize the system conditions, we tested the bed height at four levels, as shown in Figure 11, the closest result to the experimental data was for a bed height of 48 cm. The chart below shows the temperature-time graph for bed heights of 25, 35, 48, and 70 cm relative to the zero reference. The bed height significantly affects the temperature distribution within the drying chamber. A higher bed position may lead to lower temperatures due to increased distance from the heat source, while a lower bed position might result in overheating and uneven drying. The optimal bed height of 48 cm ensures a balanced temperature profile, promoting uniform drying without compromising the product quality.
The rate of moisture transfer in the product, which is the main factor in drying, can be identified through Figure 12, as shown; a bed height of 48 cm from the zero reference level provides the optimal drying condition and the closest result to the experimental data. Moisture content reduction is crucial in drying processes to prevent microbial growth and extend shelf life. The optimal bed height allows for efficient moisture removal, balancing between sufficient heat exposures and avoiding excessive drying that can lead to texture and quality degradation.
In this stage, we calculated and reported the remaining tests for a bed position height of 48 cm above the zero reference. The next optimized parameter is the heater power, tested at nominal efficiencies of 5,000, 6,000, and 7,000 W. Figure 13 shows the increase in product temperature at different power levels. Higher heater power accelerates the drying process by increasing the temperature, but it also raises the risk of overheating and damaging the product. The results indicate that the temperature changes over time at 5,000 W are closest to the experimental results, with the least deviation. This balance ensures effective drying while maintaining the product’s quality and preventing thermal damage.
The results indicate that the temperature changes over time at 5,000 W are closest to the experimental results, with the least deviation. The validation of this simulation shows an error margin of about 2%, confirming its accuracy. Up to this stage, it was observed that the optimal bed height is 48 cm, and the optimal power is 5,000 W. Therefore, for optimizing the final parameter, which is the inlet air velocity, we keep these two variables constant and perform simulations at velocities of 0.1, 0.5, and 1 m/s. Efficient energy usage is essential in drying processes to minimize operational costs and environmental impact. Using the optimal heater power reduces energy consumption while achieving the desired drying rate, contributing to a more sustainable process.
Figure 14 shows the drying rate of the product at different velocities. According to the validation results, the optimal velocity is 0.5 m/s, which has the closest graph to the experimental results. Based on the tests conducted, the optimal bed height is 48 cm, the optimal power is 5,000 W, and the optimal velocity is 0.5 m/s. Inlet air velocity influences the drying rate by affecting the airflow distribution and heat transfer. The optimal velocity ensures uniform drying across the product surface, preventing under-drying or over-drying in certain areas. This balance is crucial for maintaining product quality and achieving efficient drying.
6 Conclusion
This study presents a comprehensive analysis of the use of a catalytic infrared heater combined with convection heat transfer for the drying of Iranian pistachios. Both experimental and numerical investigations were conducted to assess the performance of the proposed system in terms of energy efficiency, drying time, and product quality.
The results demonstrate that the catalytic infrared drying system significantly outperforms traditional drying methods in several key areas:
Energy Consumption: The catalytic infrared system achieved a reduction in energy consumption by approximately 40% compared to conventional hot air drying methods. This substantial decrease is attributed to the high combustion efficiency of the catalytic heater, which operates at around 98%, and the resonant heating effect that enhances the transfer of infrared energy directly to the moisture content in the pistachios.
Drying Time: The experimental results showed a reduction in drying time by up to 30% when using the catalytic infrared system. The average drying time was reduced from 8 h in traditional hot air systems to 50 min in the catalytic infrared system, significantly improving the throughput of the drying process.
Moisture Reduction: The system effectively reduced the moisture content of pistachios from an initial 30% to the desired 5% within the shortened drying period. This level of moisture reduction was achieved without compromising the quality of the pistachios, as evidenced by the maintenance of their color, texture, and flavor profile.
Greenhouse Gas Emissions: The catalytic infrared system emitted negligible levels of harmful greenhouse gases. Specifically, CO emissions were measured at below 10 ppm, and no NOx emissions were detected, in contrast to higher emissions levels typical in gas-fired drying systems.
Product Quality: The quality of the dried pistachios was preserved, with a consistent drying process that resulted in uniform moisture content across the batch. The infrared radiation at specific frequencies facilitated even drying, preventing the common issues of scorching or uneven moisture distribution seen in traditional methods.
In conclusion, the catalytic infrared drying system offers a highly efficient, environmentally friendly, and effective solution for pistachio drying. The significant reductions in energy consumption, drying time, and greenhouse gas emissions, coupled with the preservation of product quality, position this technology as a superior alternative to conventional drying methods. The adoption of this system can lead to substantial cost savings and environmental benefits for the pistachio processing industry.
Data availability statement
The original contributions presented in the study are included in the article/Supplementary material, further inquiries can be directed to the corresponding author.
Author contributions
VE: Conceptualization, Data curation, Formal Analysis, Funding acquisition, Investigation, Methodology, Software, Validation, Writing–original draft, Writing–review and editing. KE: Resources, Supervision, Writing–review and editing.
Funding
The author(s) declare that no financial support was received for the research, authorship, and/or publication of this article.
Conflict of interest
The authors declare that the research was conducted in the absence of any commercial or financial relationships that could be construed as a potential conflict of interest.
Publisher’s note
All claims expressed in this article are solely those of the authors and do not necessarily represent those of their affiliated organizations, or those of the publisher, the editors and the reviewers. Any product that may be evaluated in this article, or claim that may be made by its manufacturer, is not guaranteed or endorsed by the publisher.
References
Aad, G., Abajyan, T., Abbott, B., Abdallah, J., Abdel Khalek, S., Abdelalim, A., et al. (2012). Observation of a new particle in the search for the Standard Model Higgs boson with the ATLAS detector at the LHC. Phys. Lett. B 716 (1), 1–29. doi:10.1016/j.physletb.2012.08.020
Abano, E. E., Ma, H. L., Qu, W. J., Wang, P. L., Wu, B. G., and Pan, Z. L. (2014). Catalytic infrared drying effect on tomato slices properties. J. Food Process. and Technol. 5 (3), 1. doi:10.4172/2157-7110.1000312
Ayadi, N., Kechaou, N., Makni, S., and Mihoubi, D. (2021). Impact of air drying conditions on the quality attributes of pomegranate arils. J. Food Eng. 299, 110525.
Can, M., and Kirmaci, V. (2021). Optimization of microwave-assisted drying of apricot and its effects on the product quality. J. Food Process Eng. 44 (4), e13594.
Chatrchyan, S., Khachatryan, V., Sirunyan, A., Tumasyan, A., Adam, W., Aguilo, E., et al. (2012). Observation of a new boson at a mass of 125 GeV with the CMS experiment at the LHC. Phys. Lett. B 716 (1), 30–61. doi:10.1016/j.physletb.2012.08.021
Chen, C., and Pan, Z. (2020). Postharvest processing of tree nuts: current status and future prospects—a comprehensive review. Compr. Rev. Food Sci. Food Saf. 21, 1702–1731. doi:10.1111/1541-4337.12906
Chua, K. J., Mujumdar, A., Hawlader, M., Chou, S., and Ho, J. (2001). Batch drying of banana pieces—effect of stepwise change in drying air temperature on drying kinetics and product colour. Food Res. Int. 34 (8), 721–731. doi:10.1016/s0963-9969(01)00094-1
Dagdug, L., Hernández-Jiménez, A., and Chairez, I. (2022). Analysis of the drying process of fruits and vegetables using a hybrid drying method. J. Food Sci. Technol. 59 (2), 719–726.
Fooladi, M. H., Tajabadipour, A., Mirdamadiha, F., Salehi, F., and Basirat, M. (2006). Methods of chemical, physical and biological detoxification of aflatoxin in pistachios and continuous processing system designed for this purpose. Rafsanjan, Iran: Iran Pistachio Research Institute, 98.
He, Y. (2008). Flameless combustion of natural gas in the SJ/WJ furnace. Queen’s University [Thesis for Doctor of Philosophy].
Hosseinalipour, S. M. (2014). Fabrication and performance study of the radiative catalytic pad for flameless combustion of natural gas in different climate conditions. Modares Mech. Eng. 14 (9).
Jafari, S. M., and Mahdavi, S. A. (2021). Optimization of drying parameters for the production of high-quality dried lemon slices. Dry. Technol. 39 (12), 1673–1682.
Jahanbakhshi, M., Kaveh, E., Taghinezhad, V., and Rasooli, S. (2020). Assessment of kinetics, effective moisture diffusivity, specific energy consumption, shrinkage, and color in the pistachio kernel drying process in microwave drying with ultrasonic pretreatment. J. Food Process. Preserv. 44, 14449. doi:10.1111/jfpp.14449
Karim, M. A., and Hawlader, M. N. A. (2021). Drying kinetics of guava and papaya: impact of air drying temperature on the quality of dried products. J. Food Eng. 297, 110519.
Lewicki, P. P. (2022). Design and application of efficient drying systems for food preservation. J. Food Eng. 310, 110620.
Maskan, M., and Karataş, Ş. (1998). Fatty acid oxidation of pistachio nuts stored under various atmospheric conditions and different temperatures. J. Sci. Food Agric. 77 (3), 334–340. doi:10.1002/(sici)1097-0010(199807)77:3<334::aid-jsfa42>3.0.co;2-a
Motevali, A., Minaei, S., and Ghobadian, B. (2021). Energy consumption and mathematical modeling of microwave drying of apple slices. J. Food Process Eng. 44 (6), e13720.
Nguyen, M. H., and Price, W. E. (2021). The effects of drying on the retention of essential nutrients in fruits and vegetables. J. Food Sci. Technol. 58 (11), 4340–4349.
Ogura, H., Yamamoto, T., Otsubo, Y., Ishida, H., Kage, H., and Mujumdar, A. S. (2005). A control strategy for a chemical heat pump dryer. Dry. Technol. 23 (6), 1189–1203. doi:10.1081/drt-200059337
Ruiz-López, M. A., and Morales-Castro, J. (2021). Comparative study on the drying kinetics and quality of mango slices using different drying methods. Dry. Technol. 39 (7), 1030–1042.
Specchia, S., Civera, A., and Saracco, G. (2004). In situ combustion synthesis of perovskite catalysts for efficient and clean methane premixed metal burners. Chem. Eng. Sci. 59 (22-23), 5091–5098. doi:10.1016/j.ces.2004.08.028
Vega-Gálvez, A., Di Scala, K., Rodríguez, K., and Miranda, M. (2021). Influence of drying temperatures on the physicochemical and antioxidant properties of red pepper. J. Food Process. Preserv. 45 (6), e15553.
Zanfir, M., and Gavriilidis, A. (2003). Catalytic combustion assisted methane steam reforming in a catalytic plate reactor. Chem. Eng. Sci. 58 (17), 3947–3960. doi:10.1016/s0009-2509(03)00279-3
Zhang, M., Tang, J., Mujumdar, A. S., and Wang, S. (2021). Trends in microwave-related drying of fruits and vegetables. Trends Food Sci. and Technol. 110, 487–497. doi:10.1016/j.tifs.2006.04.011
Keywords: pistachio, dryer, catalytic heater, heat transfer, food
Citation: Ebrahimpour V and Esmailpour K (2024) Simultaneous use of convection and radiation heat transfer for Iranian pistachio drying using catalytic heater - numerical and experimental study. Front. Mech. Eng. 10:1459806. doi: 10.3389/fmech.2024.1459806
Received: 16 July 2024; Accepted: 09 September 2024;
Published: 01 October 2024.
Edited by:
Eswaramoorthy Muthusamy, Shri Mata Vaishno Devi University, IndiaReviewed by:
Ponnusamy Palanisamy, Vellore Institute of Technology (VIT), IndiaRaja Sekhar, Vellore Institute of Technology, India
Copyright © 2024 Ebrahimpour and Esmailpour. This is an open-access article distributed under the terms of the Creative Commons Attribution License (CC BY). The use, distribution or reproduction in other forums is permitted, provided the original author(s) and the copyright owner(s) are credited and that the original publication in this journal is cited, in accordance with accepted academic practice. No use, distribution or reproduction is permitted which does not comply with these terms.
*Correspondence: Vahid Ebrahimpour, vahidebrahimpour9596@gmail.com