- 1Department of Biological Sciences, University of Calgary, Calgary, AB, Canada
- 2Integrated Bioscience Program, Department of Biology, The University of Akron, Akron, OH, United States
Recent years have witnessed a multitude of studies focusing on gekkotan adhesion. Intense interest in this phenomenon was triggered by the discovery of the manner and magnitude of the forces generated by the hair-like filaments (setae) on the toe pads and inspired the development of the next generation of smart, reversible synthetic adhesives. Most studies pursuing these goals have concentrated on the generalized form and properties of gekkotan setae outlined in those key early studies, resulting in the fabrication of synthetic filaments of uniform dimensions. Although there are over 1,800 species of extant geckos, and hundreds of species of anoles (a separate lizard lineage that has convergently evolved adhesive toe pads), most investigations have used relatively few species as the source of basic information, the Tokay gecko (Gekko gecko) being the most prominent among these. Such exemplar taxa generally exhibit structurally intricate setae and morphologically complex configurations of the adhesive apparatus. Setal structure taken to be characteristic of these taxa is generally reported by singular statements of maximal length, diameter, density and branching pattern. Contemporaneous work focusing on the configuration of setae at locations across the toe pads and upon the evolutionary origin of adhesively competent digits in anoles and specific lineages of geckos, however, has revealed extensive variation of setal structure within individuals, information about how setae may have arisen from non-adhesive filamentous precursors, and how newly adhesively competent digits have been integrated into pre-existing patterns of locomotor mechanics and kinematics. Such observations provide insights into what is minimally necessary for adhesively competent digits to function and reveal the simplest configuration of components that make this possible. We contend that information gleaned from such studies will assist those seeking to employ the principles of fibrillar-based adhesion, as exemplified by lizards, for bio-inspired applications.
Introduction
Recent years have witnessed a deluge of studies focusing on gekkotan adhesion, a remarkable phenomenon whereby geckos can attach to, and move on, smooth, low friction surfaces using series of expanded scales (scansors or lamellae) that possess arrays of hair-like fibers (setae) carrying multiple flattened, triangular-shaped tips (spatulae) (Ruibal and Ernst, 1965; Autumn et al., 2000; Autumn et al., 2002). Intense interest in this was triggered by the discovery of the manner and magnitude of the forces generated by a single seta of the Tokay gecko (Gekko gecko) (Autumn et al., 2000). These revelations served as inspiration for the development of the next generation of smart, reversible synthetic adhesives. The structure and dimensions of the setae examined by Autumn et al. (2000) have become the exemplar for gecko filaments. Attempts to simulate their attributes through fabrication of synthetic fibrils have been guided by these findings.
It is sobering to realize, however, that there are over 1,800 species of extant geckos (Uetz et al., 2020), as well as hundreds of species of anoles (a separate group of lizards that has convergently evolved adhesive toe pads–see below; Poe et al., 2017). Relatively few species, however, have been employed as the source of basic information about setal structure, and those that have generally exhibit structurally intricate setae and morphologically complex configurations of the entire adhesive apparatus (Garner et al., 2020; Russell et al., 2019; Russell and Gamble, 2019). The setal structure taken to be characteristic of these taxa is usually represented by a singular statement of seemingly important dimensions: length, diameter, density and branching pattern. Collectively these oft-repeated properties have led to setal arrays being conceptualized as organized assemblages of fibrils of essentially identical configuration (Garner and Russell, in review).
Work focusing on the form of setae at locations across toe pads, and on the evolutionary origin of adhesively-competent digits in specific lineages of geckos has, however, shed light on (1) the variation of setal structure within individuals (Russell et al., 2007; Johnson and Russell, 2009; Webster et al., 2009; Russell and Johnson, 2014); (2) the manner in which setae may have arisen from non-adhesive filamentous precursors (Russell et al., 2015; Higham et al., 2017); and (3) the way digits exhibiting the first stages of adhesive competence (in that they can support the animal during static clinging and locomotion on vertical, low friction substrata) have been integrated into pre-existing patterns of locomotor mechanics and kinematics (Russell et al., 2015; Higham et al., 2017). More recently, similarly-focused investigation of the structure of setae and the configuration of the adhesive apparatus of anoline lizards have provided insights into which structural and functional attributes are shared with geckos (Garner et al., 2020), thereby enhancing our understanding of which features appear to be of fundamental mechanical importance for the operation of a digit-based adhesive system. We contend that information gleaned from such studies can be of assistance to those seeking to adapt the principles of fibrillar-based adhesion of lizards for technological applications by revealing what is minimally necessary and sufficient for adhesively competent digits to function. Recognition of such attributes should help to simplify the pathway to the development of effective synthetic setae and mechanical mechanisms to operate them (as assessed against the performance of the actual structures being mimicked). As Niewiarowski et al. (2016) note, “no synthetic mimic can yet perform as well as a gecko”.
Transects of Setal Fields
Large numbers of publications dealing with the attributes of the adhesive setae of geckos provide information about their dimensions and morphology (Table 1). Parameters such as setal length, diameter, density, and spatular width and length have been documented. There is, however, considerable inconsistency in such reports (Table 1), this perhaps being attributable to the actual variation expressed between setae, both within and between species (see below). Generalizations about the numbers of terminal branches (hundreds to thousands) that setae bear also abound. Collectively these reports provide little in the way of insight into which of these features are particularly important, or why they should be so. Comparative observations between species (Table 2) intimate that there are species-specific differences in setal structure (Peattie, 2007), with even closely-related species seemingly exhibiting quite different dimensional properties (Table 2—see the data for the three species of Gekko). Questions arise, therefore, as to what this enormous variation might mean and what aspects of it, if any, might be critical for the development of artificial simulacra.
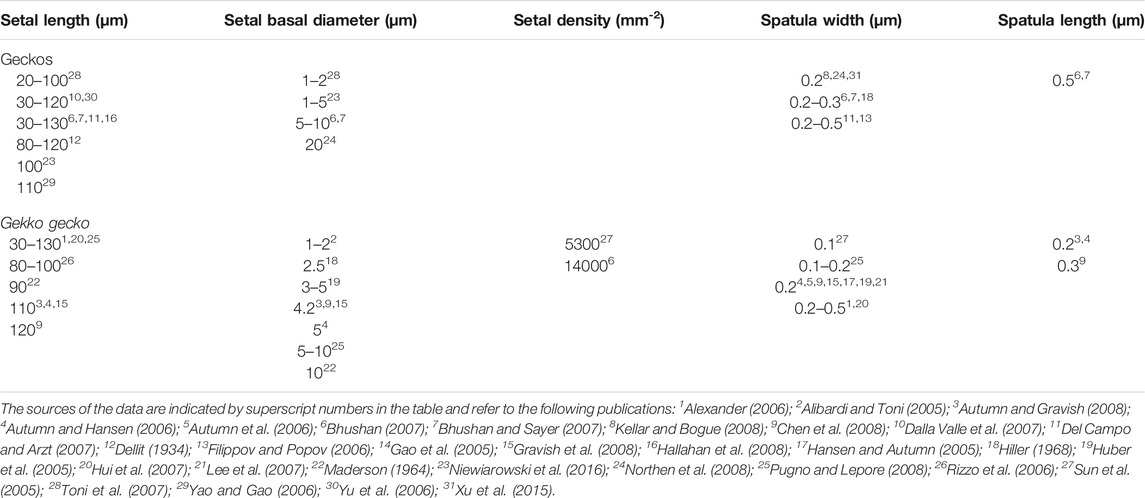
TABLE 1. Data reported for setal dimensions for geckos in general and for Gekko gecko, indicating the variability in the values. For Gekko gecko, which has been used extensively in research focusing on gecko adhesion, the values reported span large ranges but generally are not accompanied by information as to where on the toe pad the measurements were taken from. Apart from a few early investigations, most of the values reported are taken from papers published between 2005 and 2016.
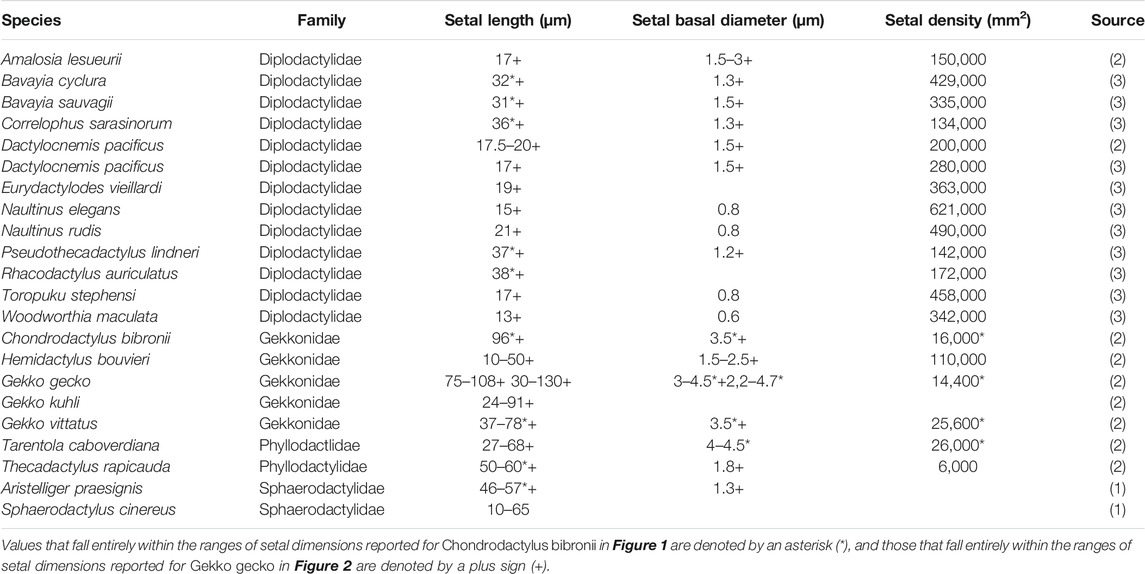
TABLE 2. Data ranges furnished by (1) Ruibal and Ernst (1965), (2) Schleich and Kästle (1986) and (3) Bauer (1998) for setal dimensions of individual species of geckos.
The sources cited in Tables 1 and 2 (apart from Ruibal and Ernst, 1965) do not mention the location on the toe pad from which the exemplar setae were sampled (scansors closer to or farther from the toe tip) and give little or no information about whether setal features vary predictably along the toe pads. Transects along the entire length of toe pads, however, reveal that variation of setal length, basal diameter and stalk density is extensive within individuals of a given species (Figures 1–4), and that this variation is regularized and predictable both along the length of the entire toe pad, as well as locally along the length of each scansor (Russell et al., 2007; Johnson and Russell, 2009; Webster et al., 2009; Russell and Johnson, 2014). There are similarities in the patterns evident in the transect data of the setal fields of Chondrodactylus bibronii (Figure 1) and Gekko gecko (Figures 2–4), with the lengths of the setae decreasing from distal to proximal along the length of each scansor and along the length of the toe pad as a whole, and with the setal stalk diameters (and thus the aspect ratio of the setae) changing in a regularized fashion (Figures 1 and 2). There are also notable differences between C. bibronii and G. gecko with regard to setal and stalk diameters, with the setae of the former increasing in diameter from distal to proximal on each scansor (Figure 1) and those of the latter doing the opposite.
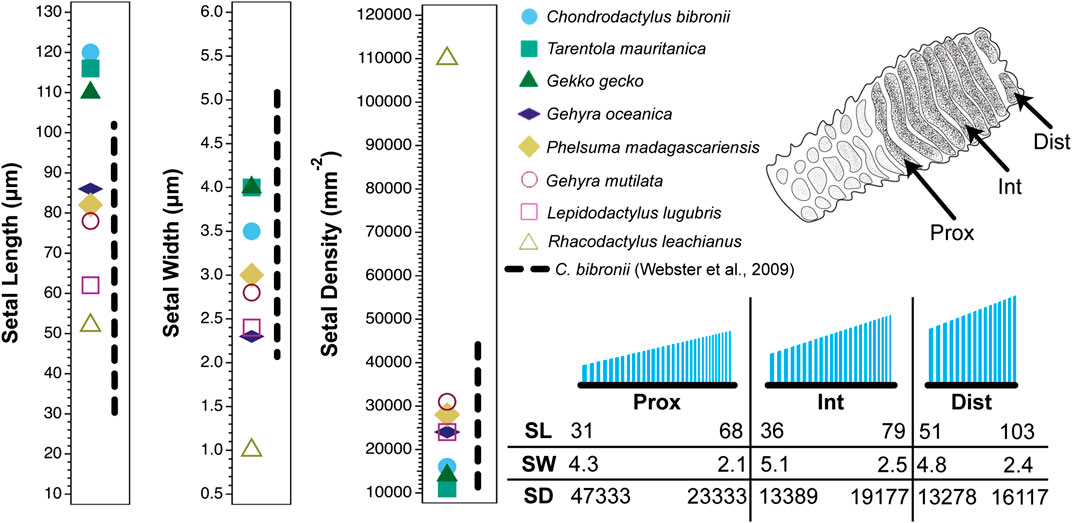
FIGURE 1. Setal dimensions (length, width, and density) recorded for digit IV, left pes of the gekkonid gecko Chondrodactylus bibronii showing variation in relation to location on the toe pad. Data are presented for a proximal (prox), intermediate (int) and distal (dist) scansor (see inset of the ventral view of the digit in the upper right corner of the figure). The different symbols on each graph at the left indicate singular values reported for setal length, width and density for eight gecko species by Peattie (2007) (see legend). The vertical dashed line to the right of the symbols for each graph represents the range of values expressed by Chondrodactylus bibronii as measured by Webster et al. (2009). The lower right inset is a schematic of the setae on the proximal, intermediate and distal scansors. Below this schematic are values of particular setal lengths (SL; µm), widths (SW; µm) and densities (SD; mm−2) that correspond to proximal and distal stations along the proximodistal length of each scansor (distal to the right).
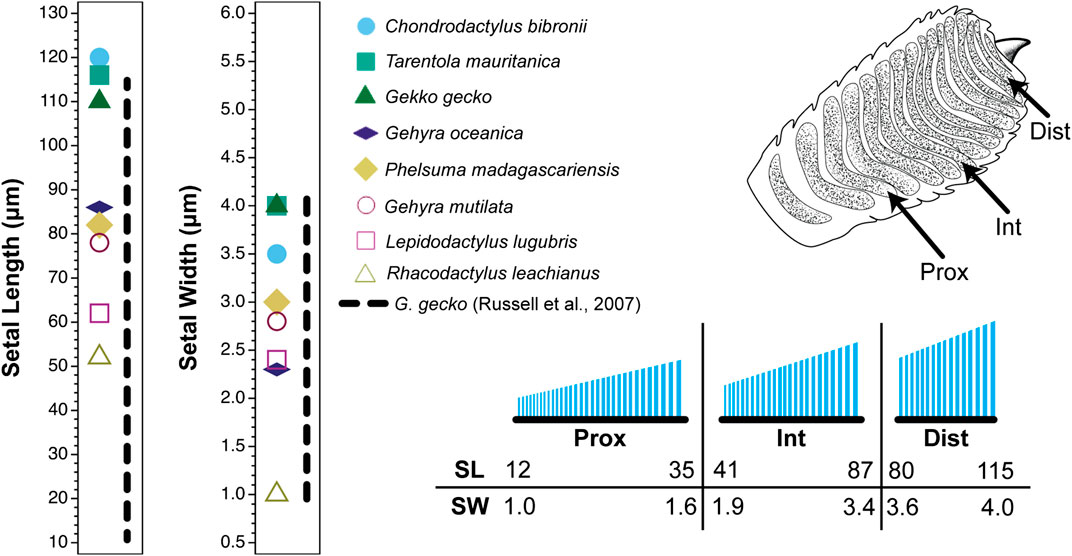
FIGURE 2. Setal dimensions recorded for digit IV, right manus of the gekkonid gecko Gekko gecko showing variation in relation to location on the toe pad. Data are presented for a proximal (prox), intermediate (int) and distal (dist) scansor (see inset of the ventral view of the digit in the upper right corner of the figure). The different symbols on each graph at the left indicate singular values reported for setal length and width for eight gecko species by Peattie (2007) (see legend). The vertical dashed line to the right of the symbols for each graph represents the range of values expressed by Gekko gecko as measured by Russell et al. (2007). The lower right inset is a schematic of the setae on the proximal, intermediate and distal scansors. Below this schematic are values of particular setal lengths (SL; µm) and widths (SW; µm) that correspond to proximal and distal stations along the proximodistal length of each scansor (distal to the right).
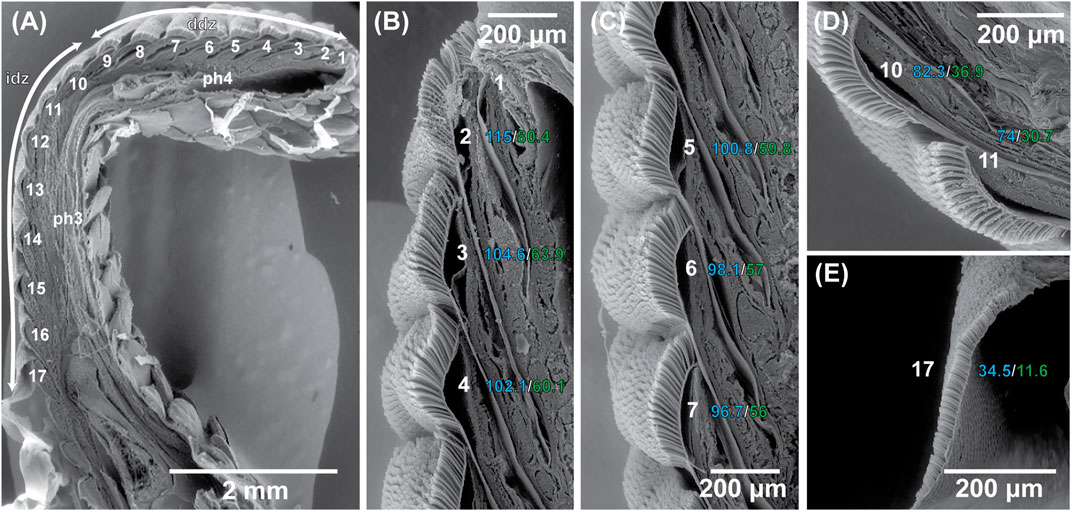
FIGURE 3. Scanning electron microscopic visualization of the sagittally-sectioned fourth digit of the right manus of the Tokay gecko, Gekko gecko. (A) Visualization of the entire toe pad showing the location and relative dimensions of the scansors and lamellae (numbered sequentially 1–17 from distal to proximal). The distal region of the digit (ddz) carries a series of scansors (1–9) that lie beneath the fourth phalanx (ph4). The intermediate region of the digit (idz) carries a series of scansors and lamellae (10–17) that lie beneath phalanx 3 (ph3), the transition from scansor to lamella occurring between plates 13 and 14. Lamellae are distinguished from scansors by not being served by branches of the lateral digital tendon system. Panels (B)–(E) depict enlargements of the individual scansors and lamellae, showing the relative lengths of the plates and the configuration of the fields of setae carried on their outer surfaces. On each plate the lengths of the filaments decrease from a maximum at the distal end to a minimum at the proximal end, and from distal to proximal along the toe pad the lengths of the filaments decrease, although there is overlap of the span of lengths between adjacent plates. For plates 1–7, 10–11, and 17 maximum filament length (in µm) is indicated in blue and minimum filament length (in µm) in green. Data from Russell et al. (2007).
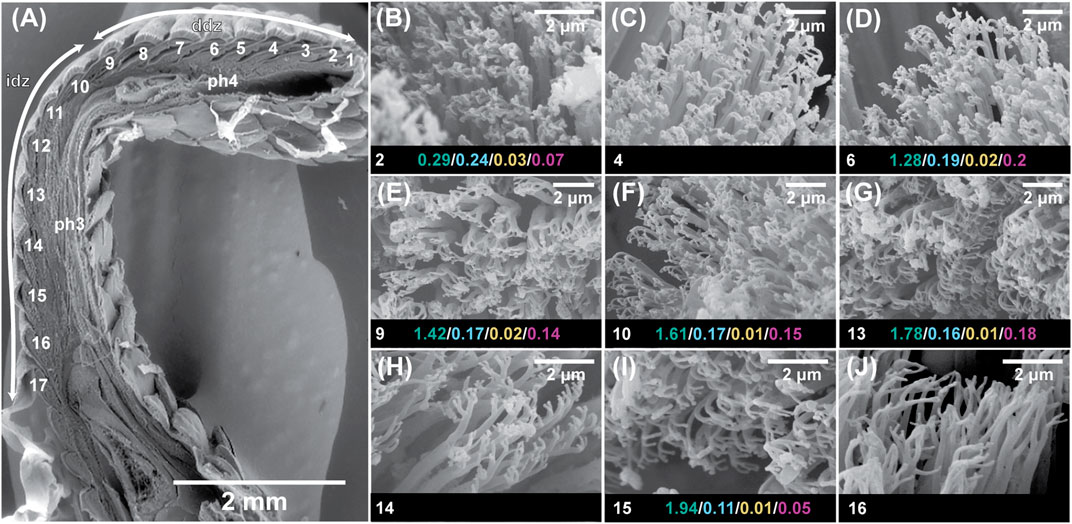
FIGURE 4. Scanning electron microscopic visualization of the sagittally-sectioned fourth digit of the right manus of the Tokay gecko, Gekko gecko. Panel (A) is identical to panel (A) in Figure 3. Panels (B)–(J) depict enlargements of the filaments carried on each plate. For scansors/lamellae 2, 6, 9, 10, 13, and 15 the following data are provided: scansor/lamella surface area (mm2) in green/average setal tip width (µm) in blue/average setal tip area (µm2) in yellow/total setal tip area per scansor/lamella (mm2) in purple. Data from Russell et al. (2007).
The extent of the variance of setal form and stalk density within a single species becomes more poignant when compared to the single seta statements for other species. Variation in setal length, basal diameter and stalk density of Chondrodactylus bibronii (Figure 1) when compared to those parameters reported by Peattie (2007) for eight other species of gecko (encompassing representatives of three families, each of which has independently evolved adhesive toe pads–Russell and Gamble, 2019), reveals that the majority of the variation reportedly encompassed by these eight species (Peattie, 2007) is subsumed within the range of variation displayed by C. bibronii. The same is true (even more so) for the setal length and setal stalk diameter variation shown by Gekko gecko (Figure 2). Further evidence of this overlap of setal dimensions between C. bibronii, G. gecko and other species of gecko is evident from the data compiled by Ruibal and Ernst (1965) and Schleich and Kästle (1986), as presented in Table 2.
Although the clinging performance of whole animals (Irschick et al., 1996) has been correlated with toe pad area, it is evident from transect surveys of the toe pads that setae in different locations differ in aspect ratio (and therefore bending stiffness) (Figures 1 and 2) and the number of spatulate tips that the setae carry (Figure 4) (Russell et al., 2007). For Gekko gecko, the most proximal fibrils recognizable as true setae (with spatulate tips) are carried on scansor/lamella numbers 14 and 15 (Figure 4H,I). These bear relatively few tips (Figure 4H,I) (certainly not the hundreds to thousands typically stated to characterize the setae of this species). They are relatively short and range down to less than 25 µm at the proximal ends of these plates (Figure 3E). Bending stiffness and the number of adhesive contacts likely directly influence adhesive force production, thus we find it probable that setae from various regions of the toe pad differentially contribute to total adhesive force capacity. In addition to variability in dimensions, fibrillar outgrowths on the subdigital surface of gecko vary considerably in form. The 16th subdigital plate (Figure 4J) of Gekko gecko bears filaments that are short, bifid at their distal tips and lack spatulae. Such forms may very well be capable of generating considerable van der Waals interactions, but whether such interactions are capable of supporting whole animal attachment and locomotion is not known.
Beyond the setae themselves, the data summarized in Figure 4 for the Tokay gecko indicate that there are major differences in the surface area of the individual scansors/lamellae that make up the toe pad, with this increasing from distal to proximal. If all setae are idealized as identical structures along the length of the toe pads, this would suggest that those scansors with the greatest surface area contribute a proportionally greater amount of the total adhesive force available to the digit. Average setal tip width and average setal tip area gradually decrease from distal to proximal along the length of the toe pad, however, as does total setal tip area. Together with changes of setal density and setal stalk diameter along the length of the toe pad (Figures 1 and 2), the variation of setal structure along the digital transect is bewilderingly complex. What this means for the potential generation of adhesive force at any station within the toe pad is unknown. It is evident, however, that bringing setae into close enough contact with the locomotor substratum to enable them to generate van der Waals and frictional adhesive attachment will be restricted to certain patches on any given footfall (Russell and Johnson, 2007; Russell and Johnson, 2014) rather than the entirety of the toe pad creating such engagement. It is possible that there is compensatory tradeoff along the length of the toe pad with regard to setal dimensions and configuration such that each localized area has the same potential for adhesive force generation (Russell et al., 2007), but we know nothing about the relative force generation attributes of these different setal configurations.
Such patterns of variation have consequences for the way in which we conceive and design simulacra of setae. Our understanding of the adhesive capacity of individual setae is based upon a single set of observations of filaments taken from an undisclosed part of a digit (Autumn et al., 2000). These observations have neither been repeated for setae with other configurations in the same species, nor for those from other species. There is still much to learn about the mechanical properties of gecko setae and it is unlikely that the single available set of observations (Autumn et al., 2000) suffices to explain the properties of all observed configurations. The current perception of the total clinging ability of a single digit of the Tokay gecko (Gekko gecko), as extrapolated from multiplying the adhesive force of a single (idealized) seta (Autumn et al., 2000) by the stated number of setae carried on such a digit (Sun et al., 2005; Autumn et al., 2006; Tian et al., 2006; Hui et al., 2007) is likely a considerable overestimate. Whole animal observations of clinging force per digit result in performance values lower by an order of magnitude or more (Irschick et al., 1996; Autumn et al., 2000, 2002; Autumn and Peattie, 2002). The discrepancy between these two approaches may reside, to some extent in the variability of the number of spatulate tips per seta along the length of the toe pad (Figure 4), differences in setal density at different stations on the toe pad (Figures 1–3), and the number of setae actually making contact with the surface (which may be reduced based on the surface roughness of the substratum).
There is still much to learn about how individual setae perform and how that performance relates to their structural properties. It is likely that much of this can be achieved through modeling, but the variation must be assessed and appreciated before it can be incorporated into such models. Much of the variation seen (at least within a single species) likely relates to the functioning of the entire setal field and the way in which it is applied to and released from the substratum. It is the entire setal field upon which the animal relies to ensure that, upon each footfall, sufficient contact is made to ensure effective attachment. A deeper understanding of the local regional variation in structure of the setae and their patterning into fields is required for more effective (and purpose-specific) fields of artificial biomimetic fibrils to be developed (Russell et al., 2019). Finding ways of simplifying such surveys of variation is paramount and we here turn to taxa that exhibit, within their ranks, evolutionary transitions from non-adhesively to adhesively competent digits. Such transitions offer the promise of determining what is minimally necessary and sufficient for a functional digital adhesive system to become incorporated into the pre-existing locomotor mechanics of lizards.
Evolutionary Transitions
The gekkotan adhesive system has been both gained and lost on multiple occasions (Gamble et al., 2012; Gamble, 2019). Although geckos are widely known for their possession of adhesive toe pads, somewhere in the region of 40% of the 1800+ living species lack them. Peattie (2008) opined that the discovery of “an extant intermediate” would greatly enhance our understanding of how the gekkotan adhesive system arose. Russell and Gamble (2019) identified a number of candidate taxa for exhibiting such a transition, but only the genus Gonatodes has been studied in detail in this regard (Russell et al., 2015; Higham et al., 2017).
Examination of the setal fields of Gekko (see above), reveals that the simplest of its spatulated setae are relatively short and subdivided into few terminal branches (Figure 4H). We do not know how effective these are in contributing to the total adhesive effectiveness of that species. We do know, however, that the relatively short, sparsely-branched setae of Gontodes humeralis (Russell et al., 2015) are sufficiently developed and numerous to permit support of its own body mass at rest and during steady vertical locomotion on a low-friction substratum (Higham et al., 2017). Equivalent locomotor capabilities are not present in any of its close relatives (Higham et al., 2017). Thus, Gonatodes provides the opportunity, using appropriate phylogenetic comparison and the establishment of evolutionary polarity (Russell et al., 2015), to establish what might be the minimal set of modifications for the emergence of a functioning digital adhesive system that is effective at the whole organism level.
Trends in digit evolution within the genus Gonatodes are depicted in Figure 5, which shows the pertinent digital features of three exemplar species. Gonatodes ocellatus has digits that are elongate and slender with a marked inflection, beneath which reside enlarged friction plates (Figure 5A–C). Gonatodes vittatus (Figure 5F–H) has relatively shorter digits with a less marked inflection, fewer ventral scales, and a flatter proximal portion. Gonatodes humeralis (Figures 5L–N) lacks a marked digital inflection and bears noticeably enlarged scales at the base of the digits. Full details of the morphological features of the digits of these three species are provided by Russell et al. (2015). The salient points of the transition to adhesive competence constitute a correlated suite of small shifts leading to the rather dramatic functional outcome of whole animal adhesive competency (Higham et al., 2017).
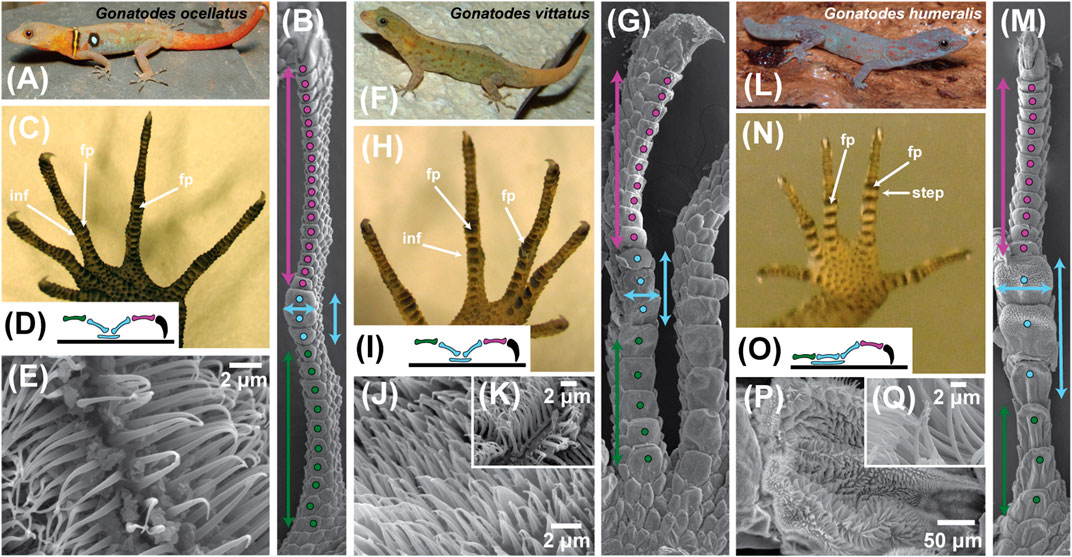
FIGURE 5. Trends in digit form within the sphaerodactylid gekkotan genus Gonatodes. This genus is regarded as lacking subdigital toe pads but exhibits digital adhesive competence in one of its species. Shifts in form and proportion in association with the acquisition of whole animal adhesive competence are illustrated using three exemplar species (A)Gonatodes ocellatus, (F)G. vittatus and (L)G. humeralis. For each species a set of illustrations [panels (A)–(E) for G. ocellatus; (G)–(K) for G. vittatus and (M)–(Q) for G. humeralis) is used to illustrate the trends to whole animal adhesive competence in G. humeralis. Panels (B), (G) and (M) depict scanning electron micrographs of the ventral aspect of digit IV of the left pes (hind foot) of the three species, with features of interest indicated by color-coded symbols. Panels (C), (H) and (N) represent the ventral view of the left pes of the three species and panels (D), (I) and (O) depict a schematic of the lateral view of the skeleton of digit IV of the pes of each species, with the phalanges color coded to match the coding in panels (B), (G) and (M). Panels (E), (J) and (K), and (P) and (Q) are scanning electron micrographs of the filamentous outgrowths of the epidermis beneath the mid-digit inflection point of digit IV of the pes. Coding conventions are as follows: purple dots–scales beneath the distal region of the digit; purple double-headed vertical arrows–proximodistal extent of the distal region of the digit; purple phalanx–penultimate phalanx; blue dots–scales comprising the friction plate region of the digit; blue double-headed vertical arrows–length of the friction plate region; blue double-headed horizontal arrows–width of the friction plate region; blue phalanges–intermediate phalanges; blue ellipsoid–extent of the friction plate region below the intermediate phalanges; green dots–scales beneath the basal region of the digit; green double-headed vertical arrows–proximodistal extent of the basal region of the digit; green phalanx–basal phalanx; curved, black claw–ungual phalanx and claw. The blue and green dotted regions combined constitute the proximal region of the digit. Abbreviations: fp–friction plates; inf–digital inflection; step–step-like transition between the proximal and distal digital regions in G. humeralis.
In the shift from strongly inflected digits, in which friction plates at the midpoint of the digit (Padian and Olsen, 1984; Russell and Bauer, 1990; Peattie, 2008) enhance traction (Figures 5A–C and 6A–D), to the adhesively-competent digits of G. humeralis (Figures 5L–N and 6E,F), the digits become relatively shorter (in relation to overall body size; Figure 5A,C,F,H,L,N). This shift is associated with a change in the relative proportions of the proximal and distal regions of the digits (Figure 5B,G,M) and a greater discrepancy in size between the scales on the underside of the digits in their proximal and distal regions (Figures 5B,G,M and 6A,C,E). Those of the proximal zone become relatively larger (both longer and wider), markedly fewer in number and exhibit greater overlap between successive scales (Figure 5B, 5G, and 5M). The relative enlargement of the subdigital scales is particularly evident for the friction plates (Padian and Olsen, 1984; Russell and Bauer, 1990) (Figures 5B,G,M and 6C,E) that lie beneath the digital inflection (labeled “inf” on Figures 5C,5H and 6C). In G. humeralis (Figure 5M), this enlargement is accompanied by a flattening of the proximal part of the digit such that all of the scales on the underside of the proximal region (Figure 5M) are co-planar and contact the substratum as a continuous strip. The inflection of the digit thus shifts from a “v”-like configuration when viewed in profile (Figure 5D,I) to a step (Figure 5N,O and 6E).
Within the digits, the changes in digital proportions and configuration are accompanied by changes in the arrangement of the phalanges. The basalmost phalanx (Figures 5D,I,O and 6C,E) increases in relative length whereas the penultimate phalanx (Figures 5D,L,O and 6C,E) becomes relatively shorter. The intermediate phalanges (Figures 5D,L,O and 6C,E) retain their relative proportions but become reoriented such that the proximal one becomes linearly aligned with the proximalmost phalanx (Figures 5D,L,O and 6E) and the distal one becomes more vertically-oriented, resulting in the “v”-like configuration of these two phalanges (Figures 5D and 6C) transforming into a step (Figures 5O and 6E). This transition is associated with a proximal extension of the friction plate area (Figures 5D,L,O and 6E) to become more extensive beneath the proximal part of the digit, resulting in the formation of an incipient toe pad (Figures 5M,N and 6E).
In all three exemplar species (Figure 5), the epidermal filaments on the underside of the toes are longest on the friction plates. In G. ocellatus, they are tapered spinules, the longest being about 3.2 µm in length (Figure 5E). In G. vittatus, the longest of these filaments are about 4.0–6.0 µm in length, with bifid tips, the split occurring at a point about two thirds of their height from the base (Figure 5K), appearing much like the filaments on the proximal lamellae of the digits of Gekko gecko (Figure 4J). This bifurcation is thought to be associated with friction enhancement (Lange, 1931; Ruibal and Ernst, 1965; Schleich and Kästle, 1979; Peattie, 2008; Müller and Hildenhagen, 2009; Spinner et al., 2013; Russell et al., 2015). In Gonatodes humeralis, the lengths, diameters, spacing and form of the subdigital spinules are similar to those of G. ocellatus and G. vittatus for the scales on the underside of the distal region of the digit (Russell et al., 2015). On the incipient toe pads, however, the distally-situated epidermal outgrowths range from 10.0 to 15.0 µm in length, their density is relatively low and the spacing relatively great (Russell et al., 2015). These are true setae that are divided terminally and carry spatulate tips of about 0.12 µm wide (Figure 5P,Q). Such setae are similar in form to those on the most proximal scansors of Gekko gecko (Figures 3E and 4H). Unlike the latter, however, the setae on each plate are shorter distally than they are more proximally (13.0 vs 15.1µm), a pattern also observed in Anolis (see below; Garner et al., 2020). The branched, spatula-bearing setae occur only at the distal end of the incipient toe pad plates (Figure 5M) and give way to spinules more proximally. Transition from simple tapered spinules (there is a mixture of unbranched and branched spinules far proximally on such scales) to setae is thus evident within the confines of a single scale.
Although Peattie (2008) suggested that “true adhesive pads require complex morphological elaborations within the toe” to enable operation of an adhesive system, Gonatodes humeralis demonstrates that this is not so, there being no modifications of the digital musculotendinous, circulatory and skeletal systems that are generally considered to be necessary (Peattie, 2008) for the operation of a functional adhesive system in geckos. In terms of their anatomy, the seta-bearing scales of G. humeralis are not scansors (Russell, 2002), but instead are more akin to the basal lamellae found in many geckos. The latter bear elaborate epidermal outgrowths, but are not associated with a lateral digital tendon network (see below). The lamellae of the Tokay gecko (Gekko gecko) carry epidermal outgrowths that range in length from 1.2 µm at their proximal end to over 12.0 µm distally (Russell et al., 2007: fig. 5), the latter being multiply branched and carrying spatulate tips. Thus, Tokay geckos also possess setae that are carried on highly modified scales (lamellae–Figure 3A,E) that lack the characteristics of scansors. Such scales bear a strong structural resemblance to the seta-bearing scales of G. humeralis and serve to support the idea of a transition from friction-enhancing to adhesion-promoting structures prior to the widening of the digits and the acquisition of features that provide the capability for actively controlling the adhesive process via distoproximal digital hyperextension (Russell and Bels, 2001).
When climbing vertical, low friction substrata (Higham et al., 2017), Gonatodes humeralis employs limb and digit kinematics that are essentially unchanged from those of lizards in general (Brinkman, 1980; Russell and Bauer, 2008) (Figure 6A,B). The digits are placed onto the substratum and withdrawn from contact with it such that the distal ends of the digits are the last regions to be withdrawn from it. In Gekko gecko, the opposite occurs, enabled by the specialized musculature of the digits that allows their distal ends to be raised from the substratum while their proximal ends remain in contact (Russell, 2002), a process known as hyperextension. When the digits of Gekko gecko make contact with the substratum, they do so with their proximal ends first. The distal parts, carrying the toe pads, are unfurled after this. Gonatodes humeralis, however, like lizards in general (Figure 6A–D), removes its digits from the locomotor surface by raising its heel first and rising onto the tips of the digits, thereby removing the ventral surface of the digits from contact with the substratum in a proximal to distal sequence (Higham et al., 2017). This results in hyperextension of the digits (as it does in lizards in general), and thus hyperextension is involved with removal of the incipient toe pads from adhesive contact with the substratum (Figure 6E and F). This breaking of adhesive contact is accommodated within a pre-existing pattern of digit kinematics (Figure 6). Adhesive competence of the digits of G. humeralis has been subsumed into an essentially unchanged pattern of digit mechanics. Geckos with incipient toe pads provide insights into the most basic aspects of digit configuration and kinematics compatible with the deployment of an effective adhesive system that can assist locomotion (Figure 6).
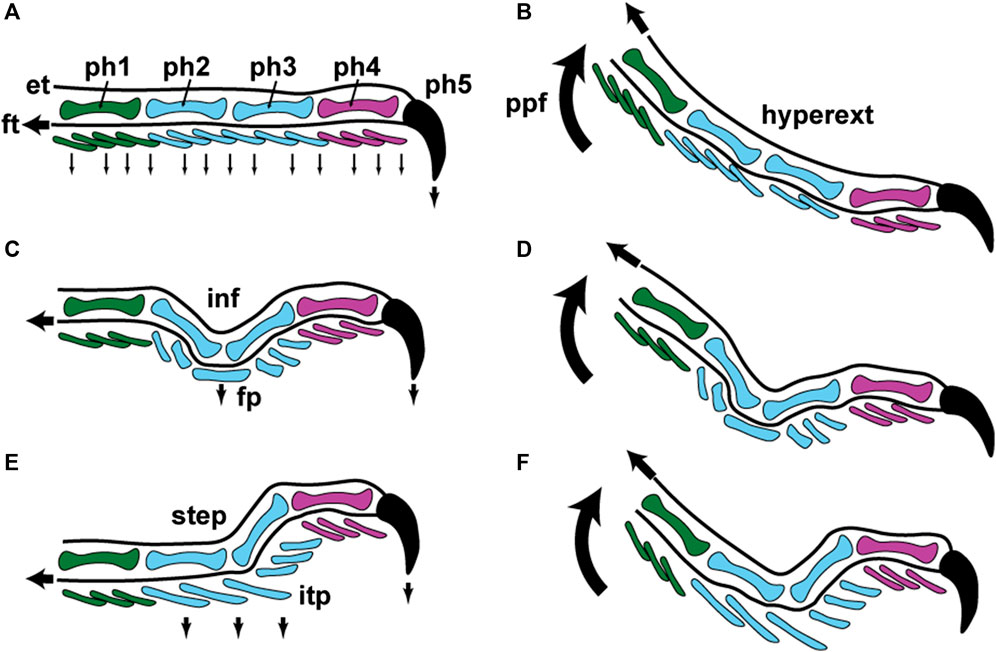
FIGURE 6. Schematic representations of lizard digit kinematics during locomotion and inferences about how incipient toe pads became incorporated into this pattern. Color conventions for the phalanges (ph1–ph5) and ventral scales of the digit are identical to those in Figure 5, panels (B), (D); (G), (I); and (M), (O). (A) and (B) fourth digit of hind foot in lateral view when applied to the substratum (A) and when being raised from contact with it (B). In panel (A) the digit is applied to the substratum with the first four phalanges (ph1–ph4) aligned with each other and the fifth (ph5) and its surrounding claw sheath being driven into the substratum via contraction of the flexor muscles of the digit through the flexor tendons (ft–proximally-pointing black arrow). The digit thus operates as a directional device along its long axis, the phalanges behaving as a series of compression struts with the reaction forces driving the ventral surface of the digit (series of small, ventrally-pointing black arrows) into contact with the substratum. In panel (B) the foot is peeled from the substratum by the heel being raised from the surface via pedal plantar flexion (ppf–curved, upwardly-pointing arrow). Scales are lifted away from the substratum in a proximal to distal sequence and the claw finally being released from contact through contraction of the digital extensor muscles operating through the extensor tendon (et–proximally-pointing black arrow). The digit is bowed as a result of this, becoming hyperextended (hyperext). Panels (C) and (D) depict the same situation for a digit with a mid-digital inflection (inf—as encountered in many climbing lizards and as seen in Gonatodes ocellatus and G. vittatus (Figure 5, panels C, D; and H, I). Because of the inflection only the claw (ph5) and the friction plates (fp) make contact with the surface. The friction plates are driven into contact with the substratum (black, ventrally-directed arrow beneath the friction plates) through tension placed on the flexor tendon (ft) In Gonatodes the ventral surface of the friction plates is clad in elongated filaments that may be bifid at their tips to enhance frictional contact (as in G. vittatus–Figure 5, panel K). Release of the digit (D) occurs via hyperextension, as depicted in panel B. Panels (E) and (F) show application and release for a gecko with incipient toe pads, such as Gonatodes humeralis (Figure 5, panels M, N). The inflection of the digit is modified into a step (step: Figure 5, panel O) and the friction plates are transformed into an incipient toe pad (itp) (Figure 5, panels M, N), the plates of which bear a free distal margin on which spatulate-tipped setae (Figure 5, panel Q) are carried. Digital mechanics relation to attachment and detachment of the incipient toe pad are essentially unchanged from the patterns depicted in panels A–D.
Gonatodes humeralis, unlike other species of Gonatodes, can scale smooth, low friction vertically-oriented substrata (Higham et al., 2017). Although the adhesive forces generated are rather low by gecko standards, they are comparable to those generated by anoles (Figure 7). Given that the setae of G. humeralis are similar in form to the simplest setae of Gekko gecko (Figure 4H), it can be inferred that the latter are also capable of generating adhesive interactions.
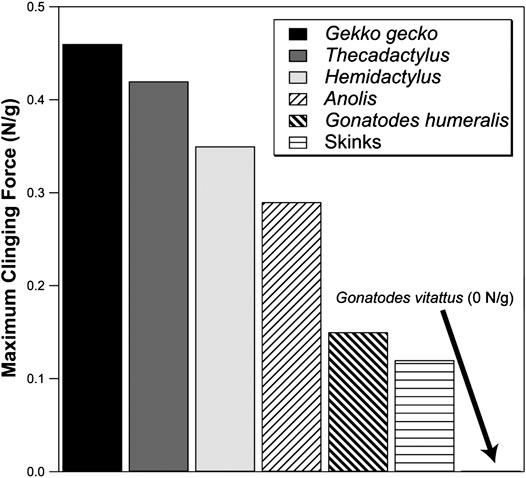
FIGURE 7. Comparison of the maximum adhesive clinging abilities of lizards (recorded as Newtons per gram of body mass). Skinks exhibit very modest output. Anoles exert, at their greatest output, more adhesive force than skinks. Geckos maximally exert adhesive forces well above that of the best-performing anoles (although lesser-performing geckos overlap with the better-performing anoles). Gekko gecko represents the greatest adhesive output yet measured for geckos. In comparison, Gonatodes vittatus generates no adhesive attachment forces whereas G. humeralis performs as well as many anole species and approaches the lower end of the adhesive performance spectrum of geckos with toe pads. Data from Higham et al. (2017) and Irschick et al. (1996).
To this point, we have established the following. (I) That the setae of geckos with structurally complex toe pads, such as Gekko gecko and Chondrodactylus bibronii, exhibit clinal variation in setal dimensions and form along the length of the digit, with the setae located proximally on the toe pad being relatively short and only modestly branched. (II) That similarly built setae are present on the incipient toe pads of geckos such as Gonatodes humeralis, which exhibits little in the way of the “complex morphological adaptations” thought to be required for the operation of an adhesive system (Peattie, 2008). The adhesive capabilities of G. humeralis suggest that the simpler setae occurring more proximally on the digits of Gekko gecko (Figure 4H,I) are also adhesively effective. These observations demonstrate that an operational adhesive system is possible in the absence of either complexly branched setae bearing “100–1,000…spatulae” (Autumn and Hansen, 2006) and complex anatomical modifications promoting attachment and detachment. We now ask (III) whether such basic levels of organization are evident in toe pads of other taxa, turning our attention to the dactyloid iguanian genus Anolis to explore this possibility.
Gecko–Anole Convergence
The evolutionary phenomenon of convergence (the independent acquisition of form and function–Kuhn et al., 2020) potentially provides evidence for determining the most fundamental attributes of complex adaptations (sensuFrazzetta, 1975). Independent but structurally similar “solutions” to challenges imposed by the environment provide insights into the evolutionary responses possible. The adhesive toe pads of geckos and anoles are regarded as convergent adaptations (Russell and Garner, in review), their adhesive toe pads having arisen completely independently. This provides the opportunity to examine which attributes are repeated in the two lineages and thus to attempt to establish what is necessary and sufficient for adhesively competent digits to be incorporated and integrated into the lacertilian pattern of locomotion.
Convergence of the Adhesive Fibrils of Geckos and Anoles
As in geckos, the epidermal outgrowths of the subdigital pads of Anolis (Figure 8A) vary considerably in form along the proximodistal axis of the digit (Figures 3–5) and five morphotypes have been recognized (Peterson and Williams, 1981): (1) true setae, outgrowths 10–30 μm in length with expanded, spatulate tips, (2) seta-prong intermediates, 5–20 μm in length with flattened tips, (3) prongs, 5–20 μm in length terminating in blunt tips with a slight taper, (4) spikes, 5–15 μm in length with straight or recurved, pointed tips, and (5) spines, up to 5 μm in length with recurved, pointed tips. All lamellae (scales bearing true setae) of Anolis with well-developed subdigital pads are thought to display a proximodistal clinal gradation of all 5 morphotypes (from spines to setae) on each lamella, similar to the clinal variation of epidermal outgrowths on the scales of the incipient toe pads of Gonatodes humeralis (Russell et al., 2015). As in gekkotans, it is the true setae and their spatulae that are responsible for the majority of adhesive force capacity in Anolis. In Anolis, however, the setae are unbranched (Ruibal and Ernst, 1965; Williams and Peterson, 1982; Stork, 1983).
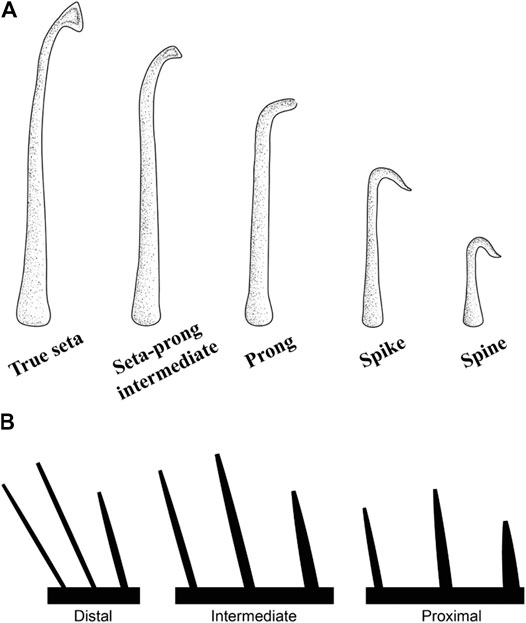
FIGURE 8. (A) Clinal series of the epidermal outgrowths present on the subdigital pad of anoline lizards (Peterson and Williams, 1981). True setae are outgrowths between 10–30 μm in length that carry a single, expanded spatulate tip. Seta-prong intermediates are outgrowths 5–20 μm in length with flattened tips. Prongs terminate in a blunt tip with a slight taper and are between 5–20 μm in length. Spikes possess straight or recurved, pointed tips and are between 5 and 15 μm in length. Spines are outgrowths up to 5 μm in length with pointed recurved tips. (B) Trends of setal morphometrics along subdigital pad regions and lamellar zones of Anolis equestris (Garner et al., 2020). Setal length increases and setal base diameter decreases proximodistally along pad regions. Setal length is maximal in the intermediate zones of lamellae. Setal base diameter decreases proximodistally along lamellar zones. Lamella length decreases proximodistally.
A recent investigation of the morphometrics of the setal arrays of the Cuban knight anole (Anolis equestris), a species similar in size to Gekko gecko, reveals that its setae increase in length and decrease in basal diameter proximodistally along regions (proximal, intermediate, distal) of the subdigital pad (Figure 8B) (Garner et al., 2020). Within a single lamella, however, setal length is greatest in the intermediate region, whereas setal basal diameter decreases proximodistally (Figure 8B), a pattern similar to the dimensions observed on the incipient toe pads of Gonatodes humeralis (see above). Setal density remains relatively consistent along the entire subdigital pad and along regions (proximal, intermediate, distal) of individual lamellae.
The profuse branching noted for the archetypal gecko seta (Autumn et al., 2000) should result in greater adhesive force production compared to an unbranched seta of similar size, such as those of Anolis (Arzt et al., 2003;Peattie and Full, 2007; Murphy et al., 2009; Garner et al., 2020). Thus, if geckos and anoles differed only in the nature of the structural hierarchy of their setae, the setal fields of gekkotans, when scaled against body mass, should be able to induce greater adhesive forces. Gekkotan and anoline setae and setal fields, however, differ not only in setal size and number of spatulae per seta, but also in the number of fibrils present per unit area (Garner et al., 2020). Indeed, when compared to setal density patterns in Chondrodactylus bibronii, those of Anolis equestris are 33.3–78 times as densely packed. Application of the Johnson-Kendall-Roberts (JKR) theory of elastic contact to the assessment of adhesive performance of gekkotan and anoline setal arrays, in conjunction with the comprehensive morphological data obtained for the setal fields of Gekko gecko (Russell et al., 2007) and Anolis equestris (Garner et al., 2020), reveal that adhesive performance of gekkotan and anoline setal arrays should theoretically be similar when taking the differences in the morphology and configuration of their setae and setal fields into consideration. Anoles appear to compensate for the lack of structural hierarchy of their setae by possessing greater setal density compared to geckos (Garner et al., 2020), although structural hierarchy of setae may be important in other aspects of the gekkotan adhesive system (Persson, 2003; Yao and Gao, 2007). Peterson et al. (1982) also note that anoles appear to combat increased adhesive demands related to increases in body size by possessing greater setal densities. Not all geckos, however, are characterized by such densities, and the setae of A. equestris are only 1.75–2.5 times as densely packed as those of Naultinus elegans, the gecko so far examined to exhibit the highest density and shortest setae, and these are also not profusely branched (Bauer, 1998). Table 2 reveals that many diplodactylid geckos have short, slender, densely packed setae, with values greatly exceeding those for gekkonid geckos (the group to which Chondrodactylus and Gekko belong). Very little is known about the adhesive capabilities of diplodactylid geckos, although this is now beginning to be explored (Pillai et al., 2020). This again reveals that there is much more to be learned about the variation in structure and function of gekkotan setae, cautioning against making sweeping generalizations that appear to characterize geckos overall.
Theabove-mentioned calculations of adhesive performance of geckos and anoles, based on setal morphometrics (Garner et al., 2020), are supported by whole animal observations. Ruibal and Ernst (1965) qualitatively observed gecko and anole adhesive locomotion on a vertical raceway and noted no obvious differences in performance. More comprehensive work by Irschick et al. (1996) corroborated this finding and found that static clinging performance of geckos and anoles is not markedly different (Figure 7). The general similarity of setal form and material properties of anoles and geckos indicate the requirement for similar loading conditions (normal load followed by shear load) because measurements of whole animal adhesive performance indicate that their adhesive systems can be engaged in the same manner (Irschick et al., 1996). The means by which setal loading and unloading occurs in anoles, however, differs from that typically attributed to geckos (Russell and Bels, 2001), but is similar to that employed by Gonatodes humeralis (Figure 6E,F), with subdigital pad retraction progressing proximodistally (as opposed to the distoproximal pattern thought to be typical of geckos). This pattern of release of the setae is consistent with setal length increasing proximally along the length of each lamella (Figure 8B), suggesting that clinal variation in setal length is related to the biomechanics of subdigital pad peeling (Johnson and Russell, 2009; Garner et al., 2020), although further empirical work is needed to validate this.
Convergence of the Digital Anatomy of Geckos and Anoles
The anatomical modifications generally associated with the adhesive system in geckos (Peattie, 2008) are only modestly represented by Anolis, being confined to skeletal (phalangeal) and tendinous features (Russell and Gamble, 2019). The digits of Anolis exhibit little in the way of muscular modifications akin to those exhibited by at least some gecko lineages (Russell and Gamble, 2019). Anolis does, however, incorporate a compliance-promoting cushioning system (Figure 9A) into its toe pads, in the form of hypertrophied lacunar cells of the epidermis (Russell, 2016). This cushioning system is unique to Anolis but is analogous and positionally similar to the vascular (Russell, 1981) and adipose tissue (Russell and Bauer, 1988) compliance structures found among geckos.
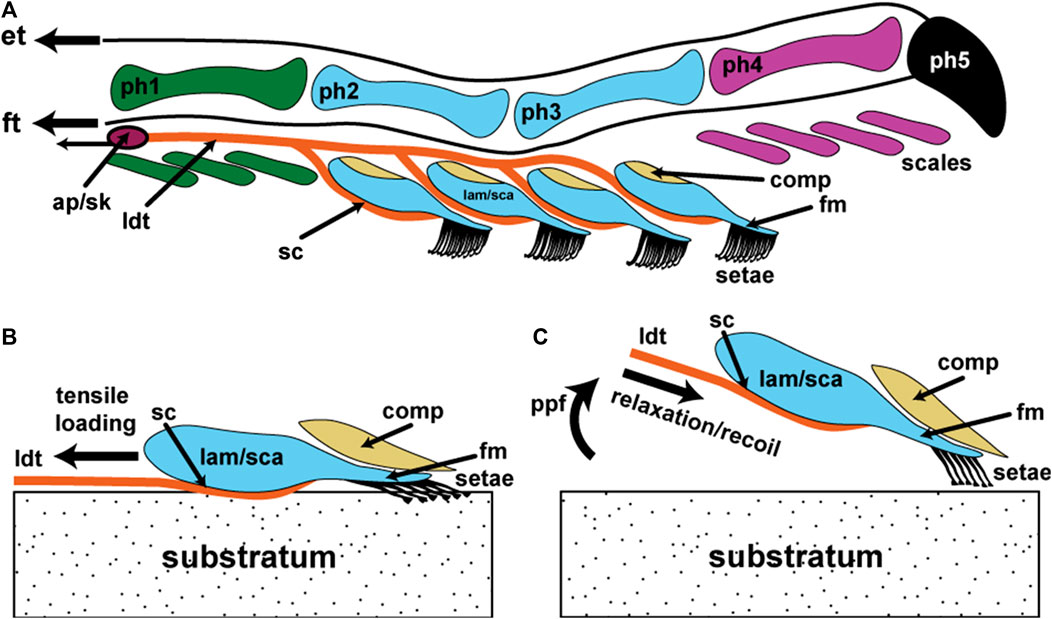
FIGURE 9. Schematic representation of the basic components of the lacertilian digital adhesive system assembled as a model for the operation of this basic mechanism. Information about structure is derived from a combination of anoles and gekkotan taxa exhibiting incipient toe pads. (A) Diagrammatic lateral view of digit IV. Phalanges 1–5 (ph1–ph5) and their associated ventral scales (color coded to match the phalanges) that bear micro-ornamentation that is modified into unbranched setae bearing a single spatulate tip on the free margin of enlarged scales (lamellae/scansors; lam/sca) situated beneath phalanges 2 and 3. The rows of setae on each lamella/scansor increase in length from distal to proximal. Each lamella/scansor has an expanded proximal zone with an extensive dermal and subdermal core. Its ventral integument consists of epidermis and a dermal stratum compactum (sc) dominated by linearly-arranged (parallel with the long axis of the digit) collagen fibers that is continuous with the lateral digital tendons (ldt) lying to either side of the phalanges. This sc/ldt continuum is linked to an aponeurotic network (ap) controlled by muscles in the flexor compartment of the lower limb and anchored onto skeletal elements (sk) that limit the tensile loading placed upon it. Overlying the setal batteries is a flexible compliance system (comp) that, when pressurized during application of the lamellae/scansors to the substratum, assists in aligning the setal batteries into a continuous setal field along the length of the toe pad. Extensor (et) and flexor (ft) tendons, through the actions of their associated muscles, control the claw and flexion and extension of the entire digit. Lamellae/scansors also possess a thin, flexible free margin (fm). (B) Diagrammatic lateral view of a single lamella/scansor with the setae applied to the substratum in the adhesion mode. (C) Diagrammatic lateral view of a single lamella/scansor in the process of being released from adhesive contact with the substratum as a result of digital hyperextension, driven by pedal plantarflexion (ppf).
Modifications of the intermediate phalanges enhance both the pressing of the toe pads onto the substratum during attachment and their hyperextension during release (Figure 6E,F). The toe pads of Anolis are located basally on the digits, ventral to the location of the digital inflection (between phalanges 2 and 3 of digit IV) of its close relatives, in a similar location to those of Gonatodes humeralis (Figures 5M,N and 6E,F). Associated with the toe pads of Anolis are lateral digital tendons similar to those of geckos. These course along the lateral and medial borders of the phalanges of the digit and branch to serve each lamella. As in geckos, the dense, collagen rich connective tissue of the lateral digital tendons is continuous with the stratum compactum of the dermis of the lamellae (the lamellar dermis lacking a stratum laxum). Thus, as in geckos (Russell, 1986), the lateral digital tendon/lamellar dermis complex furnishes a tensile skeleton that provides connectivity between the setae and skeleton at the metapodial-phalangeal joint capsules. This chain reinforces the junction between the integument and the underlying tissues (Russell, 1986) and permits the tensile load imposed on the setae (Peterson et al., 1982) to be channeled to points of resistance deep within the manus and pes, and to be regulated in its magnitude.
Synthesis and the Generation of a Model of the Fundamentals of Form and Function of the Digital Adhesive System of Lizards
Our examination of transects of setal fields constituting the toe pads of geckos, the evolutionary transition to functionally adhesive digits in the naked-toed gecko genus Gonatodes, and the comparison of the structure of gecko and anole adhesive systems has allowed us to deduce what we consider to represent the most basic level of organization and mechanical operation of such a system. Both setal structure, at its most elaborate, and the configuration and operation of the entire adhesive system of the Tokay gecko (Gekko gecko) are complex (Russell, 2002). They permit impressive feats of behavior that are assisted by the deployment of the adhesive apparatus (Autumn 2006), but are not typical of all geckos.
Examination of the variability of setal structure exhibited by several species of geckos (and anoles) across the entirety of their setal fields indicates that setae vary considerably in form and dimensions depending on the particular location on the digit (Russell et al., 2007; Johnson and Russell, 2009; Webster et al., 2009; Russell and Johnson, 2014; Garner et al., 2020). Such data indicate that there is no single morphology of setae for the toe pads of lizards bearing adhesive pads and that these parameters vary regionally, presumably reflecting the functional contribution of particular regions of the toe pads to the totality of the adhesive capabilities of the entire setal fields. A common feature, however, regardless of the particular dimensions of setae at different stations on the toe pad, is that the setae on individual scansors show gradation in length (Figures 1 and 2). In the case of Gekko and Chondrodactylus, increase in length of the setae occurs in a proximal to distal direction (Figures 1 and 2). This has been suggested to be associated with the achievement of simultaneous release of all setae on a scansor as the digit is hyperextended (Johnson and Russell, 2009). In the case of Gonatodes humeralis (Russell et al., 2015) and Anolis equestris (Garner et al., 2020), however, setal length decreases proximodistally. Interestingly, the locomotor kinematics of Gonatodes humeralis and Anolis are essentially unchanged from those typical of lizards in general, the toes being peeled from the substratum proximodistally as the ankle and wrist extend (a motion called plantarflexion) (Russell and Bels, 2001; Higham et al., 2017) (Figure 6B,D,F). Thus, a seta-based adhesive mechanism has been co-opted into a pre-existing pattern of foot and digital mechanics in both G. humeralis and Anolis.
The relatively simple setae, although still branched, at the proximal end of the setal fields of Gekko gecko somewhat resemble those of Gonatodes humeralis (Figure 5M,N). Gonatodes humeralis exhibits the early stages of evolutionary acquisition of adhesively-competent digits (Russell et al., 2015) and is capable of adhesively-assisted locomotion on vertical, smooth, low-friction surfaces (Higham et al., 2017). It lacks any of the major skeletal, muscular, tendinous and compliance modifications evident in the digits of the Tokay gecko (Russell, 2002), but is still able to attach and detach its adhesively-competent digits in a controlled fashion (Higham et al., 2017). The morphological modifications that it does show are related to changes of scale size and number on the underside of the digits, alterations of in phalangeal proportions and orientation, and transformation of some of the epidermal outgrowths on the expanded scales of the incipient toe pads into setae with spatulate tips. There are no evident lateral digital tendons associated with the plates of the incipient toe pads, and it appears that tensile loading is placed upon the setae and lamellae by gravitational loading, potentially restricting the circumstances in which this rudimentary adhesive system can be engaged.
It is interesting to note that all geckos capable of supporting static and dynamic adhesion on vertical, low friction substrata, including Gekko gecko and Gonatodes humeralis, possess setae that exhibit some level of branching. Comparison of both Gekko gecko and Gonatodes humeralis with Anolis reveals a setal structure that is much simpler than that of Gekko gecko or Gonatodes humeralis. Anolis possesses unbranched setae that are relatively short and present in higher density (compared to that of gekkonid geckos–Table 2). Its clinging abilities (Figure 7) are similar to those of many geckos (Irschick et al., 1996), despite the absence of structural hierarchy that purportedly increases the adhesive capacity of a single fiber (Arzt et al., 2003; Peattie and Full, 2007; Murphy et al., 2009; Garner et al., 2020). The higher setal density of Anolis is thought to compensate for the lack of branching in anoline setae (Garner et al., 2020). A number of species of Anolis also appear to respond to the increased adhesive demands of increased in body size by increasing setal density (Peterson et al., 1982). Like G. humeralis, Anolis exhibits proximal to distal hyperextension of its digits (Figure 6) and its setae increase in length from distal to proximal on each lamella (Figure 8B), consistent with the simultaneous release hypothesis proposed by Johnson and Russell (2009). In terms of digital anatomy, Anolis exhibits a simpler manifestation than Gekko, but is more fully elaborated than that of Gonatodes humeralis. Anolis exhibits phalangeal modifications associated with application of the toe pads to the substratum and their hyperextension upon release. Beyond this it also has a lateral digital tendon system and a compliance mechanism associated with enhancing contact of the setae with the substratum.
The foregoing yields information about what is minimally necessary and sufficient for the effective operation of a lacertilian digital filamentous adhesive system. Such information should potentially be useful for simplifying approaches to the development of biomimetic derivatives. From the above-mentioned observations we can conclude that a functional adhesive system, effective at the whole animal level, can exist and operate with relatively short and unbranched or moderately branched setae, and that operational control of such a system requires only minor morphological and behavioral changes compared to lizards in general. Squamate adhesive systems do not need to be morphologically highly divergent from their ancestral non-adhesive precursors. Basic whole animal adhesive competence can be attained with relatively simple morphological and biomechanical underpinnings.
The situation evident in Anolis and gekkotans with incipient toe pads provides the best approximation of the necessary and sufficient components. A model for the basic functioning of a digit-based, filamentous adhesive system is presented in Figure 9. The presence of spatulate-tipped setae arranged in orderly ranks and rows provide the necessary adhesive interface with the substratum. Such setae do not have to be branched to be effective but must be present in sufficient numbers to enable effective support (of the animal’s body mass) when using only a (potentially small) subset of them on surfaces that permit only patchy contact. If a rolling-type pattern of contact and detachment (as embodied in hyperextension of digits, whether proximodistal or distoproximal) is involved, with the filaments carried on a series of plates, then a staggering of filament length, row by row, that is geometrically integrated with the mechanics (angulation) of plate release, is needed to promote simultaneous release and attachment of the fibrils (Figure 9).
Digits are inherently directional devices when used to interact with the substratum during locomotion. As such they undergo tensile loading. Setae are inherently tensile (Williams and Peterson, 1982) and directional (Autumn et al., 2000) structures that require a mechanism of controllable application (Figure 9B) and release (Figure 9C) of tensile loading to exploit available attachment forces. The lateral digital tendon system of anole and gecko digits facilitates this load-bearing function (Figure 9). The lamellae (and scansors) bear setae that are intimately connected to such a mechanism through firm interdigitation of the epidermis with a richly collagenous, longitudinally-oriented fibrous sheet that invests the inner face of the lamella/scansor and that is continuous with the lateral digital tendons (Russell, 1986). The collagen fibers, at rest, exhibit crimp and upon loading it is likely that the crimp is straightened (Figure 9B), the collagenous bundles thereby shifting into their high modulus phase, preventing further extension. We suggest that the magnitude of extension allowed is tuned to the parallel preload required for the setae to be effectively adhesively engaged with the substratum (Autumn et al., 2000) and that this tensile loading is maintained until detachment is initiated. In lizards, we propose that this is accomplished by the lateral digital tendon/stratum compactum mechanism being connected deep in the foot such that constant tension can be maintained by internally regulated locking devices. We suggest that the perpendicular preload (Autumn et al., 2000) required to establish initial setal tip contact is provided by the unfurling of the hyperextended digit (Figure 6B,D,F), and that tension imposed through the lateral digital tendon/stratum compactum system (Figure 9A) places tension on the lamella/scansor and its setae, resulting in the lowering the setal angle and the bringing of the broad face of the spatulate tip(s) into contact with the substratum (Figure 9B). The small amount of movement required for the application of the parallel preload (Autumn et al., 2000) is likely controlled by the very limited extensibility of the lateral digital tendons/stratum compactum. When this tension is released, the lateral digital tendons and the collagenous networks in the lamellae recoil and the crimp in the collagen fibers is restored (Figure 9C). This results in the setae being relieved of their tensile loading, permitting their shafts to increase their angle relative to the surface of the lamella (Figure 9C) and allowing them to approach their critical release angle (Figure 9C). This process occurs as the digit is hyperextended through actions of the extensor muscles and angular changes between the phalanges driven by pedal plantarflexion (Figure 6B,D,F).
Both pad-bearing anoles and geckos exhibit some sort of compliance mechanism to assist with enhancing contact with the substratum (Russell, 1981, 2002, 2017). This may chiefly assist in aligning the setal arrays on individual lamellae/scansors so that the setal field essentially forms one unified structure, with the junctions between the plates being overlain by a continuous cushioning mechanism (Figure 9A,B). It may also assist the setal fields to match more closely with irregularities in the substratum (Russell and Johnson, 2007). Such a compliance mechanism (differently constructed in different lineages) is a common feature of lizards with adhesive toe pads. Compliance of the setal fields with the substratum may also be assisted by the setae being carried on a relatively slender, free distal component of the lamellae/scansors (Figure 9). Indeed, in Anolis, the setae are borne on a very thin free margin that is comprised solely of epidermal tissue. The free margin of geckos is slightly more robust. In both cases, the free margin is overlain by the compliance mechanism.
Conclusion
The last two decades have witnessed a flood of investigations examining the form, function, and properties of the gecko adhesive system, many of them aiming, directly or indirectly, to inform the design and fabrication of the next generation of smart, reversible, fibrillar synthetic adhesives. Most of such applied research, however, has focused on relatively few species of gecko that exhibit complex manifestations of the entire adhesive apparatus, perhaps unnecessarily complicating the biomimetic process. Here we contend that studies examining setal transects of adhesive pad-bearing lizards, evolutionary transitions from non-adhesive to adhesively competent digits, and the convergent evolution of the adhesive apparatuses of geckos and anoles provide a wealth of information from which researchers may gain engineering inspiration. Specifically, these biologically focused studies collectively demonstrate what is minimally structurally necessary for an effective and functional fibrillar adhesive system that is capable of reversibly attaching to vertical, low friction substrata. We urge researchers focused on bio-inspired adhesives to consider the natural variation in both structure and function of the adhesive fibrils and their associated higher order digital anatomy exhibited by lizards with subdigital adhesive pads. Such information is likely to not only increase the available information for successful biomimicry, but also decrease functional disparities between synthetic simulacra and their natural counterparts.
Data Availability Statement
The original contributions presented in the study are included in the article. Further inquiries should be directed to the corresponding author.
Ethics Statement
The animal studies were reviewed and approved by the University of Calgary Animal Research and Education Executive Committee under Protocol Numbers BI2002-013, BI2008-24 for those studies completed by AR and the University of Akron Institutional Animal Care and Use Committee Protocol Number 19-07-13 NGC for those studies completed by AG.
Author Contributions
AR conceived of the approach and wrote the first draft of the paper. AR and AG developed the ideas further and contributed equally to subsequent drafts. AR developed the ideas for the figures and these were refined by AG. AR employed various aspects of his previous research on geckos and anoles to develop the synthesis of ideas and AG provided new insights into anole and gecko adhesion based upon his ongoing research.
Conflict of Interest
The authors declare that the research was conducted in the absence of any commercial or financial relationships that could be construed as a potential conflict of interest.
Acknowledgments
We thank Stas Gorb and Ken Nakano for inviting us to contribute to this Research Topic collection of papers. AR acknowledges financial support from a Natural Science and Engineering Research Council of Canada Discovery Grant (9745–2008).
References
Alexander, R. M. (2006). Introduction to biotribology: animal locomotion. Proc. Inst. Mech. Eng. Part J J. Eng. Tribol. 220, 649–656. doi:10.1243/13506501jet157
Alibardi, L., and Toni, M. (2005). Distribution and characterization of proteins associated with cornification in the epidermis of gecko lizard. Tissue Cell 37, 423–433. doi:10.1016/j.tice.2005.05.005
Arzt, E., Gorb, S., and Spolenak, R. (2003). From micro to nano contacts in biological attachment devices. Proc. Natl. Acad. Sci. U.S.A. 100, 10603–10606. doi:10.1073/pnas.1534701100
Autumn, K., and Gravish, N. (2008). Gecko adhesion: evolutionary nanotechnology. Philos. Trans. R. Soc. Math. Phys. Eng. Sci. 366, 1575–1590. doi:10.1098/rsta.2007.2173
Autumn, K., and Hansen, W. (2006). Ultrahydrophobicity indicates a non-adhesive default state in gecko setae. J. Comp. Physiol. 192, 1205–1212. doi:10.1007/s00359-006-0149-y
Autumn, K., and Peattie, A. M (2002). Mechanisms of adhesion in geckos. Integr. Comp. Biol. 42, 1081–1090. doi:10.1093/icb/42.6.1081
Autumn, K., Liang, Y. A., Hsieh, S. T., Zesch, W., Chan, W. P., Kenny, T. W., et al. (2000). Adhesive force of a single gecko foot-hair. Nature 405, 681–685. doi:10.1038/35015073
Autumn, K., Majidi, C., Groff, R. E., Dittmore, A., and Fearing, R. (2006). Effective elastic modulus of isolated gecko setal arrays. J. Exp. Biol. 209, 3558–3568. doi:10.1242/jeb.02469
Autumn, K., Sitti, M., Liang, Y. A., Peattie, A. M., Hansen, W. R., Sponberg, S., et al. (2002). Evidence for van der Waals adhesion in gecko setae. Proc. Natl. Acad. Sci. U. S. A. 99, 12252–12256. doi:10.1073/pnas.192252799
Bauer, A. M. (1998). Morphology of the adhesive tail tips of carphodactyline geckos (Reptilia: Diplodactylidae). J. Morphol. 235, 41–58.
Bhushan, B. (2007). Adhesion of multi-level hierarchical attachment systems in gecko feet. J. Adhes. Sci. Technol. 21, 1213–1258.
Bhushan, B., and Sayer, R. A. (2007). Gecko feet: natural attachment systems for smart adhesion in applied scanning probe methods VII. Berlin: Springer, 41–76.
Brinkman, D. (1980). Structural correlates of tarsal and metatarsal functioning in Iguana (Lacertilia; Iguanidae) and other lizards. Can. J. Zool. 58, 277–289.
Chen, B., Wu, P. D., and Gao, H. (2008). Hierarchical modelling of attachment and detachment mechanisms of gecko toe adhesion. Proc. R. Soc. -Math. Phys. Eng. Sci. 464, 1639–1652. doi:10.1098/rspa.2007.0350
Dalla Valle, L., Nardi, A., Toffolo, V., Niero, C., Toni, M., and Alibardi, L. (2007). Cloning and characterization of scale beta-keratins in the differentiating epidermis of geckoes show they are glycine-proline-serine-rich proteins with a central motif homologous to avian beta-keratins. Dev. Dynam. 236, 374–388. doi:10.1002/dvdy.21022
Del Campo, A., and Arzt, E. (2007). Design parameters and current fabrication approaches for developing bioinspired dry adhesives. Macromol. Biosci. 7, 118–127. doi:10.1002/mabi.200600214
Dellit, W. (1934). Zur anatomie und physiologie der geckozehe. Jenaische Z. Für Naturwissenschaft. 68, 613–656.
Filippov, A. E., and Popov, V. (2006). To optimal elasticity of adhesives mimicking gecko foot-hairs. Phys. Lett. 358, 309–312. doi:10.1016/j.physleta.2006.05.034
Frazzetta, T. H. (1975). Complex adaptations in evolving populations. Sunderland, MA: Sinauer Associates.
Gamble, T. (2019). Duplications in corneous beta protein genes and the evolution of gecko adhesion. Integr. Comp. Biol. 59, 193–202. doi:10.1093/icb/icz010
Gamble, T., Greenbaum, E., Jackman, T. R., Russell, A. P., and Bauer, A. M. (2012). Repeated origin and loss of adhesive toepads in geckos. PLoS One 7, e39429. doi:10.1371/journal.pone.0039429
Gao, H., Wang, X., Yao, H., Gorb, S., and Arzt, E. (2005). Mechanics of hierarchical adhesion structures of geckos. Mech. Mater. 37, 275–285. doi:10.1016/j.mechmat.2004.03.008
Garner, A. M., and Russell, A. P. (In review). Revisiting the classification of squamate adhesive setae: historical, morphological, and functional perspectives.
Garner, A. M., Wilson, M. C., Wright, C., Russell, A. P., Niewiarowski, P. H., and Dhinojwala, A. (2020). The same but different: the setal arrays of anoles and geckos indicate alternative approaches to achieving similar adhesive effectiveness. J. Anat. doi:10.1111/joa.13377
Gravish, N., Wilkinson, M., and Autumn, K. (2008). Frictional and elastic energy in gecko adhesive detachment. J. R. Soc. Interface 5, 339–348. doi:10.1098/rsif.2007.1077
Hallahan, D. L., Keiper-Hrynko, N. M., Ganzke, T. S., Toni, M., Dalla Valle, L., and Alibardi, L. (2008). Gene expression in analysis of adhesive pads of gecko digits shows they mainly code for significant production of cysteine-rich and glycine-rich beta-keratins. J. Exp. Zool. B. 312, 58–73. doi:10.1098/rsif.2007.1077
Hansen, W. R., and Autumn, K. (2005). Evidence for self-cleaning in gecko setae. Proc. Natl. Acad. Sci. U.S.A. 102, 385–389. doi:10.1073/pnas.0408304102
Higham, T. E., Gamble, T., and Russell, A. P. (2017). On the origin of frictional adhesion in geckos: small morphological changes lead to a major biomechanical transition in the genus Gonatodes. Biol. J. Linn. Soc. 120, 503–517. doi:10.1111/bij.12897
Hiller, U. (1968). Untersuchungen zum Feinbau und zur Funktion der Haftborsten von Reptilien. Z. Morphol. Tiere. 62, 307–362.
Huber, G., Gorb, S. N., Spolenak, R., and Arzt, E. (2005). Resolving the nanoscale adhesion of individual gecko spatulae by atomic force microscopy. Biol. Lett. 1, 2–4. doi:10.1098/rsbl.2004.0254
Hui, C.-Y., Jagota, A., Shen, L., Rajan, A., Glassmaker, N., and Tang, T. (2007). Design of bio-inspired fibrillar interfaces for contact and adhesion—theory and experiments. J. Adhes. Sci. Technol. 21, 1259–1280. doi:10.1163/156856107782328362
Irschick, D. J., Austin, C. C., Petren, K., Fisher, R. N., Losos, J. B., and Ellers, O. (1996). A comparative analysis of clinging ability among pad‐bearing lizards. Biol. J. Linn. Soc. 59, 21–35.
Johnson, M. K., and Russell, A. P. (2009). Configuration of the setal fields of Rhoptropus (Gekkota: gekkonidae): functional, evolutionary, ecological and phylogenetic implications of observed pattern. J. Anat. 214, 937–955. doi:10.1111/j.1469-7580.2009.01075.x
Kellar, E., and Bogue, R. (2008). Biomimetic adhesives: a review of recent developments. Assemb. Autom. 28, 282–288. doi:10.1108/01445150810904422
Kuhn, A., Skipwith, P., and Overcast, I. (2020). Digest: an emerging model system for understanding ecomorphological convergence. Evolution. 74, 696–697. doi:10.1111/evo.13935
Lange, B. (1931). “Integument der Sauropsiden,” in Hanbuch der vergleichenden Anatomie der Wirbeltiere. Editors L. Bolk, E. Göppert, E. Kallius, and W. Lubosch (Berlin: Urban and Schwarzenberg).
Lee, H., Lee, B. P., and Messersmith, P. B. (2007). A reversible wet/dry adhesive inspired by mussels and geckos. Nature 448, 338–341. doi:10.1038/nature05968
Maderson, P. F. A. (1964). Keratinized epidermal derivatives as an aid to climbing in gekkonid lizards. Nature 203, 780–781.
Müller, R., and Hildenhagen, T. (2009). Untersuchungen zu Subdigital-und Subkaudalstrukturen bei Chamäleons (Sauria: chamaeleonidae). Sauria. 31, 41–54. doi:10.1515/9783110928242.11
Murphy, M. P., Kim, S., and Sitti, M. (2009). Enhanced adhesion by gecko-inspired hierarchical fibrillar adhesives. ACS Appl. Mater. Interfaces 1, 849–855. doi:10.1021/am8002439
Niewiarowski, P. H., Stark, A. Y., and Dhinojwala, A. (2016). Sticking to the story: outstanding challenges in gecko-inspired adhesives. J. Exp. Biol. 219, 912–919. doi:10.1242/jeb.080085
Northen, M. T., Greiner, C., Arzt, E., and Turner, K. L. (2008). A Gecko‐inspired reversible adhesive. Adv. Mater. 20, 3905–3909. doi:10.1063/pt.5.028438
Padian, K., and Olsen, P. E. (1984). Footprints of the Komodo monitor and the trackways of fossil reptiles. Copeia. 14, 662–671.
Peattie, A. M., and Full, R. J. (2007). Phylogenetic analysis of the scaling of wet and dry biological fibrillar adhesives. Proc. Natl. Acad. Sci. Unit. States Am. 104, 18595–18600. doi:10.1002/ar.20706
Peattie, A. M. (2008). Subdigital setae of narrow-toed geckos, including a Eublepharid (Aeluroscalabotes felinus). Anat. Rec. 291, 869–875. doi:10.1073/pnas.0707591104
Persson, B. N. J. (2003). On the mechanism of adhesion in biological systems. J. Chem. Phys. 118, 7614–7621. doi:10.1063/1.1562192
Peterson, J. A., Benson, J. A., Ngai, M., Morin, J., and Ow, C. (1982). Scaling in tensile “skeletons”: structures with scale-independent length dimensions. Science 217, 1267–1270.
Peterson, J. A., and Williams, E. E. (1981). A case history in retrograde evolution: the onca lineage in anoline lizards: II. Subdigital fine structure. Bull. Mus. Comp. Zool. 149, 215–268.
Pillai, R., Nordberg, E., Reidel, J., and Schwarzkopf, L. (2020). Nonlinear variation in clinging performance with surface roughness in geckos. Ecol. Evol. 10, 2597–2607. doi:10.1002/ece3.6090
Poe, S., Nieto-Montes de Oca, A., Torres-Carvajal, O., De Queiroz, K., Velasco, J. A., Truett, B., et al. (2017). A phylogenetic, biogeographic, and taxonomic study of all extant species of Anolis (Squamata; Iguanidae). Syst. Biol. 66, 663–697. doi:10.1093/sysbio/syx02
Pugno, N. M., and Lepore, E. (2008). Observation of optimal gecko’s adhesion on nanorough surfaces. Biosystems 94, 218–222. doi:10.5353/th_b4552928
Rizzo, N. W., Gardner, K. H., Walls, D. J., Keiper-Hrynko, N. M., Ganzke, T. S., and Hallahan, D. L. (2006). Characterization of the structure and composition of gecko adhesive setae. J. R. Soc. Interface 3, 441–451. doi:10.1154/1.2754446
Ruibal, R., and Ernst, V. (1965). The structure of the digital setae of lizards. J. Morphol. 117, 271–293. doi:10.1002/jmor.1051170302
Russell, A. P., Baskerville, J., Gamble, T., and Higham, T. E. (2015). The evolution of digit form in Gonatodes (Gekkota: sphaerodactylidae) and its bearing on the transition from frictional to adhesive contact in gekkotans. J. Morphol. 276, 1311–1332. doi:10.1002/jmor.20420
Russell, A. P., and Bauer, A. M. (1990). Digit I in pad-bearing gekkonine geckos: alternate designs and the potential constraints of phalangeal number. Mem. Qld. Mus. 29, 453–472.
Russell, A. P., and Bauer, A. M. (1988). Paraphalangeal elements of gekkonid lizards - a comparative survey. J. Morphol. 197, 221–240. doi:10.1002/jmor.1051970208
Russell, A. P., and Bauer, A. M. (2008). “The appendicular locomotor apparatus of Sphenodon and normal-limbed Squamates,” in Biology of the Reptilia. Editors C. Gans, A. S. Gaunt, and K. Adler (Ithaca, New York: Society for the Study of Amphibians and Reptiles), 1–466.
Russell, A. P., and Bels, V. (2001). Digital hyperextension in Anolis sagrei. Herpetologica. 57, 58–65. doi:10.7717/peerj.1874/fig-1
Russell, A. P. (1981). Descriptive and functional anatomy of the digital vascular system of the tokay, Gekko gecko. J. Morphol. 169, 293–323.
Russell, A. P., and Gamble, T. (2019). Evolution of the gekkotan adhesive system: does digit anatomy point to one or more origins?. Integr. Comp. Biol. 59, 131–147. doi:10.1093/icb/icz006
Russell, A. P., and Garner, A. M. (In review). “Solutions to a Sticky Problem: convergence of the adhesive systems of geckos and anoles (Reptilia: Squamata),” in Convergent Evolution. Editors V. Bels, and P. Legreneur. (New York, NY: ISTE).
Russell, A. P. (2002). Integrative functional morphology of the gekkotan adhesive system (Reptilia: Gekkota). Integr. Comp. Biol. 42, 1154–1163. doi:10.1093/icb/42.6.1154
Russell, A. P., and Johnson, M. K. (2014). Between a rock and a soft place: microtopography of the locomotor substrate and the morphology of the setal fields of Namibian day geckos (Gekkota: gekkonidae: Rhoptropus). Acta Zool. 95, 299–318. doi:10.1111/azo.12028
Russell, A. P., Johnson, M. K., and Delannoy, S. M. (2007). Insights from studies of gecko-inspired adhesion and their impact on our understanding of the evolution of the gekkotan adhesive system. J. Adhes. Sci. Technol. 21, 1119–1143. doi:10.1163/156856107782328371
Russell, A. P., and Johnson, M. K. (2007). Real-world challenges to, and capabilities of, the gekkotan adhesive system: contrasting the rough and the smooth. Can. J. Zool. 85, 1228–1238. doi:10.1139/Z07-103
Russell, A. P., Stark, A. Y., and Higham, T. E. (2019). The integrative biology of gecko adhesion: historical review, current understanding, and grand challenges. Integr. Comp. Biol. 59, 101–116. doi:10.1093/icb/icz032
Russell, A. P. (1986). The morphological basis of weight-bearing in the scansors of the tokay gecko (Reptilia: sauria). Can. J. Zool. 64, 948–955.
Russell, A. P. (2016). The structure of anoline (Reptilia: Dactyloidae: Anolis) toe pads in relation to substratum conformity. Acta Zool. 14, 2–10. doi:10.1111/azo.12180
Russell, A. P. (2017). The structure of anoline (Reptilia: Dactyloidae: Anolis) toe pads in relation to substratum conformity. Acta Zool. 98, 300–309. doi:10.1111/azo.12180
Schleich, H. H., and Kästle, W. (1979). Hautstrukturen als kletteranpassungen bei Chamaeleo and Cophotis (Reptilia: sauria: Chamaeleonidae, Agamidae). Salamandra 16, 95–100.
Schleich, H. H., and Kästle, W. (1986). Ultrastrukturen an Gecko-Zehen (reptilia: sauria: gekkonidae). Amphib.-Reptil. 7, 141–166.
Spinner, M., Westhoff, G., and Gorb, S. N. (2013). Subdigital and subcaudal microornamentation in chamaeleonidae: a comparative study. J. Morphol. 274, 713–723. doi:10.1002/jmor.20137
Stork, N. E. (1983). A comparison of the adhesive setae on the feet of lizards and arthropods. J. Nat. Hist. 17, 829–835.
Sun, W., Neuzil, P., Kustandi, T. S., Oh, S., and Samper, V. D. (2005). The nature of the gecko lizard adhesive force. Biophys. J. 89, L14–L17. doi:10.1529/biophysj.105.065268
Tian, Y., Pesika, N., Zeng, H., Rosenberg, K., Zhao, B., McGuiggan, P., et al. (2006). Adhesion and friction in gecko toe attachment and detachment. Proc. Natl. Acad. Sci. U. S. A. 103, 19320–19325. doi:10.1073/pnas.0608841103
Toni, M., Dalla Valle, L., and Alibardi, L. (2007). The epidermis of scales in gecko lizards contains multiple forms of beta-keratins including basic glycine-proline-serine-rich proteins. J. Proteome Res. 6, 1792–1805. doi:10.1021/pr060626+
Uetz, P., Slavenko, A., Meiri, S., and Heinicke, M. (2020). Gecko diversity: a history of global discovery. Isr. J. Ecol. Evol. 1, 1–9. doi:10.1163/22244662-bja10003
Webster, N. B., Johnson, M. K., and Russell, A. P. (2009). Ontogenetic scaling of scansorial surface area and setal dimensions of Chondrodactylus bibronii (Gekkota: gekkonidae): testing predictions derived from cross‐species comparisons of gekkotans. Acta Zool. 90, 18–29. doi:10.1111/j.1463-6395.2008.00324.x
Williams, E. E., and Peterson, J. A. (1982). Convergent and alternative designs in the digital adhesive pads of scincid lizards. Science 215, 1509–1511.
Xu, Q., Wan, Y., Hu, T. S., Liu, T. X., Tao, D., Niewiarowski, P. H., et al. (2015). Robust self-cleaning and micromanipulation capabilities of gecko spatulae and their bio-mimics. Nat. Commun. 6, 8949. doi:10.1038/ncomms9949
Yao, H., and Gao, H. (2007). Bio-inspired mechanics of bottom-up designed hierarchical materials: robust and releasable adhesion systems of gecko. Bull. Pol. Acad. Sci. Tech. Sci. 55, 141–150. doi:10.1163/156856107782328326
Yao, H., and Gao, H. (2006). Mechanics of robust and releasable adhesion in biology: bottom–up designed hierarchical structures of gecko. J. Mech. Phys. Solid. 54, 1120–1146. doi:10.1016/j.jmps.2006.01.002
Keywords: Anolis, biomimetics, convergent evolution, fibrillar adhesion, Gekkota, synthetic adhesives
Citation: Russell AP and Garner AM (2021) Setal Field Transects, Evolutionary Transitions and Gecko–Anole Convergence Provide Insights Into the Fundamentals of Form and Function of the Digital Adhesive System of Lizards. Front. Mech. Eng 6:621741. doi: 10.3389/fmech.2020.621741
Received: 26 October 2020; Accepted: 15 December 2020;
Published: 27 January 2021.
Edited by:
Stanislav N. Gorb, University of Kiel, GermanyReviewed by:
Luciano Afferrante, Politecnico di Bari, ItalyYoshitaka Nakanishi, Kumamoto University, Japan
Copyright © 2021 Russell and Garner. This is an open-access article distributed under the terms of the Creative Commons Attribution License (CC BY). The use, distribution or reproduction in other forums is permitted, provided the original author(s) and the copyright owner(s) are credited and that the original publication in this journal is cited, in accordance with accepted academic practice. No use, distribution or reproduction is permitted which does not comply with these terms.
*Correspondence: Anthony P. Russell, YXJ1c3NlbGxAdWNhbGdhcnkuY2E=