- Department of Biosystems Engineering, Chungbuk National University, Cheongju, South Korea
Of several higher alcohols such as pentanol, hexanol, octanol, decanol, dodecanol, phytol, and fusel oil, pentanol isomers have recently received remarkable attention as alternative fuels for diesel engines because they emit less greenhouse gases and harmful pollutants. Because of great potential as a blending component and physicochemical properties of pentanol, binary blends such as diesel/pentanol and biodiesel/pentanol and ternary blends such as diesel/biodiesel/pentanol were mainly studied in the conventional diesel engine. Quaternary blends of diesel, biodiesel, straight vegetable oil, and pentanol were also investigated. Very few information related to the application of pentanol to advanced compression ignition (CI) engine is available in the literature. The diesel/pentanol blends coupled with exhaust gas recirculation (EGR) technology could simultaneously reduce NOx and soot emissions from CI engine. Further, diesel/pentanol blends generally produced higher CO and hydrocarbon (HC) emissions than did diesel fuel. However, CO and HC emissions were significantly reduced by mixing the cetane improver with the blends. Up to 45–50% n-pentanol/diesel blends can be safely used in diesel engines without any engine modification or any additive. However, in terms of kinematic viscosity, lubricity, and oxidation stability of diesel/pentanol blends, the use of pentanol should be limited to concentrations below 10%. Generally, in the studies of biodiesel/pentanol blends in CI engines, the reduction of CO, HC, NOx, and smoke emissions was observed compared to neat biodiesel. For ternary blends, diesel/biodiesel/pentanol blends were mainly investigated by the researchers. The emission characteristics in terms of CO, HC, NOx, and smoke opacity showed the different trends according to the pentanol proportion in the ternary blends, particularly <10% or more than 10%. To increase the biofuels in the CI engine, more studies for the quaternary blends of pentanol are required.
Introduction
Generally, alcohols such as methanol, ethanol, propanol, and butanol are the popular alcohols which could be used for automotive fuels (Yusri et al., 2017; Awad et al., 2018; No, 2019). Investigations related to the application of propanols (n-propanol and isopropanol) to internal combustion engines are rare and have been mainly restricted to spark ignition (SI) engines. Although high volatility, low viscosity, and high oxygen content of the above short-chain alcohols could improve spray combustion and emissions, several disadvantages such as high latent heat of vaporization, low heating value, and low cetane number (CN) lead to the lower proportion applications in compression ignition (CI) engines. Further, lower alcohols exhibit several problems such as phase separation, cold start problem (ethanol), stability issues, storage problems, and corrosion (methanol) when blended with diesel (Sridhar et al., 2018). Even in the case of diesel/biodiesel/ethanol ternary blends, the proportion of ethanol in the blend should be kept as low as possible and NOx emission from burning this ternary blend was not desirable (Shahir et al., 2015).
Therefore, the utilization of higher alcohol or long-chain alcohols blended with diesel fuel has recently received remarkable attention as alternative fuels for diesel engines. The term “higher alcohol” usually refers to the series of straight-chain alcohols containing four or more carbon atoms, that is, butanol (C4H9OH), pentanol (C5H11OH), hexanol (C6H13OH), octanol (C8H17OH), decanol (C10H23OH), dodecanol (C12H25OH), phytol (C20H41OH), and fusel oil (No, 2019). Of several higher alcohols, pentanol isomers have recently received remarkable attention as alternative fuels for diesel engines because they emit less greenhouse gases and harmful pollutants.
Although propanol (C3H7OH) cannot be treated as a higher alcohol, propanol was also included in the review by Rajesh Kumar and Saravanan (2016a) on the application of higher-alcohol biofuels in diesel engines as it is used as a solvent and a blending component with diesel fuels. Butanol use in diesel engines was mainly discussed, and pentanol including other higher alcohols in diesel engines was briefly introduced. The feasibility of utilizing butanol, pentanol, and octanol as fuels for internal combustion engines had been assessed by Sarathy (2016). However, only two engine studies had been introduced with n-pentanol as a diesel fuel substitute for CI engines.
The comprehensive review conducted by Babu et al. (2017) concluded that it is possible to replace diesel up to 40–45% through blending with butanol and pentanol, respectively, for use in CI engines without any engine modifications. In addition, it is also possible to use neat butanol or pentanol under high pressure in CI engines for achieving advanced CI engines.
Further, the effects of various alcohols as an additive in biodiesel/diesel blends on performance, combustion, and emission characteristics of diesel engine were reviewed by Zaharin et al. (2017). The presence of oxygen in alcohol fuel promotes a more complete combustion, in turn resulting in an improvement of thermal efficiency with the reduction of CO, HC, and particulate matter (PM) emissions. According to a recent review on the effect of alcohols on PM emissions from CI engines by Verma et al. (2019), the lower PM number concentration was obtained for n-pentanol blended fuels compared to diesel and biodiesel. In addition, n-pentanol blended fuels showed a longer ignition delay due to the lower CN and viscosity of n-pentanol.
It is clear from the existing reviews discussed in the above that the discussions related to the recent application of pentanol isomers for alternative fuels to CI engine are not enough in the literature. The objective of this study is, therefore, to provide a comprehensive review of the literature on the utilization of pentanol isomers in conventional and advanced CI engines. It should be noted that the contents in this study are the extension of the pentanol application to CI engines which is included in a book written by the author (No, 2019).
Physicochemical Properties of Pentanol
The pentanol (amyl alcohol) isomers include eight alcohols with the formula C5H12O as shown in Figure 1. The primary pentanol isomers include pentan-1-ol (n-pentanol), 3-methylbutan-1-ol (isopentanol), and 2-methylbutan-1-ol (2-methylbutanol). In addition, pentan-2-ol, tert-amyl alcohol (2-methyl-2-butanol), and pentan-3-ol belong to secondary and tertiary pentanols, respectively. Isopentanol is also known as isoamyl alcohol, isopentyl alcohol, isobutyl carbinol, and 3-methyl-1-butanol. In addition, alcohols with desirable properties as advanced SI engine fuels include 2-pentanol and 2-methyl-1-butanol (McCormick et al., 2017).
Of several pentanol isomers, the physicochemical properties of pentanol isomers which are mainly considered as fuel are shown in Table 1. Due to its preferable physical and chemical properties, n-pentanol is one of the higher alcohols with five carbons in its structure and has big potential as a blending agent with diesel fuel or biodiesel because of its high energy density, high CN, better blend stability, and less hygroscopic nature compared with other widely studied lower alcohols such as methanol, ethanol, and butanol (Imdadul et al., 2016a). As can be seen in Table 1, some properties of n-pentanol such as density, viscosity, latent heat of vaporization, and lower heating value are closer to diesel. The kinematic viscosity of pentanol/diesel blends measured at 40°C is sharply reduced with a small pentanol concentration (<20%), but it is slightly increased with an increase in pentanol concentration of more than 20% (Lapuerta et al., 2012). Recently, Cano-Gomez and Iglesias-Silva (2019) proposed a new correlation for the prediction of kinematic viscosity as a function of the volume fraction of biodiesel/pentanol blends from 283.15 to 343.15 K at atmospheric pressure as follows.
where ν is the kinematic viscosity of the mixture in mm2/s; ϕ is the volume fraction; ξ, Ω, and, ω are adjustable temperature parameters; and T is the temperature in K. The parameters a, b, c, d, e, and f are found from the experimental data given in the article of Cano-Gomez and Iglesias-Silva (2019).
Oxidation stability of biodiesel/diesel blends with pentanol addition (10 and 15%) was investigated by Peer et al. (2017). They concluded that pentanol addition of only up to 10% volume to Calophyllum inophyllum biodiesel/diesel blends increased the oxidation stability, but above which it decreased the storage stability.
It is vital to test the blends of pentanol and diesel for their capacity to hold water to avoid phase separation. Recently, it is found that the water concentration added into the blends gradually decreases with an increase in temperature (Jin et al., 2019).
Fundamental Combustion Study of Pentanol
The discussion for the fundamental combustion study of pentanol will be limited to the chemical kinetics of pentanol combustion herein. The chemical kinetic model can be divided into the detailed and reduced (skeletal) kinetic mechanisms. A complete discussion on the fundamental combustion and chemistry of alcohols may be found in the comprehensive review of Sarathy et al. (2014), but the studies related to pentanol were not fully discussed.
Detailed Chemical Kinetic Mechanism
The several different detailed chemical kinetic mechanisms for 1-pentanol were developed by Togbe et al. (2011), Heufer et al. (2012, 2013), Kohler et al. (2015), Wang et al. (2015), Nativel et al. (2016), and Pelucchi et al. (2017). For isopentanol, Dayma et al. (2011), Tsujimura et al. (2011), Sarathy et al. (2012, 2013), Lucassen et al. (2013), Park et al. (2015a), and Nativel et al. (2016) had suggested the detailed chemical kinetic mechanisms. Further, the detailed mechanism for 2-methyl-1-butanol was proposed by Tang et al. (2013), Serinyel et al. (2014), Park et al. (2015a,b), and Lucassen et al. (2015). In addition, Li et al. (2013) and Kohler et al. (2015) had developed the detailed mechanism for 2- and 3-pentanols.
Reduced Chemical Kinetic Mechanism
In addition to the detailed chemical kinetic mechanisms discussed in the above section, seven reduced chemical kinetic mechanisms were developed for pentanol isomers and blends as shown in Table 2. Two models for n-pentanol were recently proposed by Chang et al. (2018) and Katoch et al. (2019), and two mechanisms for isopentanol were proposed by Sarathy et al. (2013) and Park et al. (2015a). One combined reduced chemical kinetic mechanism was suggested by Liu et al. (2016) for the oxidation of primary reference fuel/C1-C5 alcohol mixtures. Further, two models for blends were developed by Huang et al. (2018) for diesel/pentanol blends and Ma et al. (2018) for diesel/biodiesel/n-pentanol.
The chemical kinetic oxidation models for pentanol isomers are summarized in Table 2. It can be found that 14 detailed kinetic mechanisms for pentanol isomers were suggested. In addition, five reduced kinetic mechanisms for pentanol isomers, one reduced mechanism for binary blend of diesel/pentanol, and another one for tertiary blend of pentanol were developed. A comparative study of the detailed mechanism for each pentanol isomers is required. Only a brief description of the model developed after 2010 is summarized herein, and readers can refer to No (2019) for details.
Utilization of Pentanol in Conventional CI Engine
The application of pentanol in conventional CI engine can be classified into four different categories: neat pentanol and binary, ternary, and quaternary blends. For the expression of blends, diesel, biodiesel, and pentanol are denoted by D, B, and P, respectively, where DxxBxxPxx refers to a blend of xx vol% diesel, xx vol% biodiesel, and xx vol% pentanol.
Neat Pentanol
For the neat pentanol in the single-cylinder engine experiment by Li et al. (2015a,b), ultralow emissions of NOx and smoke could be obtained with exhaust gas recirculation (EGR) under an engine speed of 1,600 rpm and indicated mean effective pressure of 0.6 MPa and with maintained high thermal efficiency. The higher indicated thermal efficiency and lower indicated specific fuel consumption were obtained for neat pentanol as injection timing was retarded. However, the introduction of neat pentanol in diesel engine resulted in higher CO and HC emissions, which should be solved by after-treatment methods such as oxidizing catalysts. Recently, pentanol was selected by Rajak and Verma (2019) in the numerical simulation of the diesel engine for comparative analysis of engine characteristics for diesel, vegetable oil, animal fats, waste cooking oil (WCO), and alcohols. The commercial software named Diesel-RK was used for their study.
Binary Blends
The binary blends for pentanol can be divided into diesel/pentanol blends, biodiesel/pentanol blends, kerosene/pentanol blends, and straight vegetable oil (SVO)/pentanol blends.
Diesel/Pentanol Blends
Spray characteristics
In the spray experiments of pentanol addition to diesel in different ratios (D100P0, D80P20, and D60P40), the results obtained by Ma et al. (2016) showed that diesel/n-pentanol blends produce a shorter spray tip penetration and a wider spray angle as compared to neat diesel under non-evaporating conditions. The effect of ambient density on spray penetration was negligible, while spray penetration increased with increase in ambient temperature. Increase in ambient density resulted in remarkable increase in spray angles of all fuels tested, while spray angles were unaffected by ambient temperature except D60P40. Even though they obtained a fairly good agreement between the empirical correlation and the measured data for liquid-phase penetration, the data for vapor-phase penetration were not discussed. Recently, Pan et al. (2019) also found that for n-pentanol/diesel blends (D75P25 and D50P50), the spray angle increased, while spray penetration and spray area decreased compared to neat diesel fuel. However, Li et al. (2019) found the opposite trend for spray area, although spray penetration and spray angle for pentanol/diesel blends (D90P10, D80P20, and D60P40) showed the same trend with Ma et al. (2016) and Pan et al. (2019). The correlation for the prediction of liquid length in the diesel/pentanol blends (D80P20 and D60B40) was newly suggested by Liu et al. (2019).
Performance characteristics
In the study of n-pentanol/diesel blends with fractions of up to 25% in CI engines, the addition of pentanol to diesel could improve brake thermal efficiency (BTE), while a slight decrease of power output was observed by Campos-Fernandez et al. (2012). They continued the performance tests of a diesel engine without any modification run by 1-pentanol/diesel fuel blends (Campos-Fernandez et al., 2013). Their results showed insignificant engine power, BTE, and brake-specific fuel consumption (BSFC) variations when the engine was fueled with the majority of the blends. Among the blends of 10, 15, 20, and 25% 1-pentanol with diesel, they recommended the D75P25 blend as a diesel fuel substitute if long-term diesel engine tests provide satisfactory results. As expected, several studies reported that pentanol addition in the diesel/pentanol blends causes BSFC to increase due to their low calorific value while BTE remained unaffected with increase in pentanol proportion in the blends (Wei et al., 2013, 2014; Rajesh Kumar and Saravanan, 2015; Yilmaz and Atmanli, 2017b). However, at the same loads, BSFC increased with increasing pentanol concentration, while BTE followed an opposite tread (Pan et al., 2019). Further, Sridhar et al. (2018) observed a slight reduction of BTE for diesel/1-pentanol blend (D80P20) compared to diesel fuel at all engine loads.
At all engine loads, a higher BSFC than that of diesel was obtained for pentanol/diesel blends, and BTE increased with an increase in engine load for all the fuel blends (Yilmaz and Atmanli, 2017b; Pan et al., 2019). However, compared to diesel fuel, BSFC increased and BTE decreased in their subsequent study of different engines, loads, and speeds (Atmanli and Yilmaz, 2018). Exhaust gas temperature of pentanol/diesel blends of up to 35% increased with respect to the load and pentanol addition for all the blends (Yilmaz and Atmanli, 2017b).
Combustion and emission characteristics
Wei et al. (2013, 2014) and Ma et al. (2017a) confirmed that in the experimental results for three pentanol/diesel blends (D90P10, D80P20, D70P30, and D60P40), the addition of n-pentanol leads to longer ignition delay and increases the peak heat release rate in the premixed combustion phase. Same with the results of Wei et al. (2014), the findings of Rajesh Kumar and Saravanan (2015) for D90P10, D80P20, D70P30, and D55P45 confirmed that n-pentanol blends with diesel showed large amounts of premixed combustion, which resulted in the rapid rise of in-cylinder pressures and heat release rates. In addition, n-pentanol addition results in the increase of HC and CO emissions for pentanol in the blended fuel at all loads (Wei et al., 2013, 2014) and at low engine loads but results in a decrease at high engine loads (Rajesh Kumar and Saravanan, 2015). According to Rajesh Kumar and Saravanan (2015), HC and CO emissions also increased with increasing EGR rates (0–30%). Further, a slight increase of NOx emissions and remarkable decrease of the particulate mass and number concentrations were obtained with increase in pentanol addition (Wei et al., 2013, 2014). However, a significant decrease of NOx emissions was observed by Atmanli and Yilmaz (2018) for D95P5, D75P25, and D65P35.
According to Rajesh Kumar et al. (2016a), D60P40 produced the lowest CO, HC, and NOx emissions but the highest smoke emissions when compared to 40% n-propanol/diesel and 40% n-butanol/diesel blends as shown in Table 3. NOx and smoke could be simultaneously reduced using the combination of n-pentanol/diesel blends, late injection by 2 crank angle degree (CAD) and moderate EGR rates of 30% (Rajesh Kumar et al., 2016b). However, it can be found from Table 3 that 10% pentanol/diesel blend emitted the highest CO, NOx, and soot but the lowest HC emission when compared to 10% n-propanol/diesel and 10% n-butanol/diesel blends (Yilmaz et al., 2016).
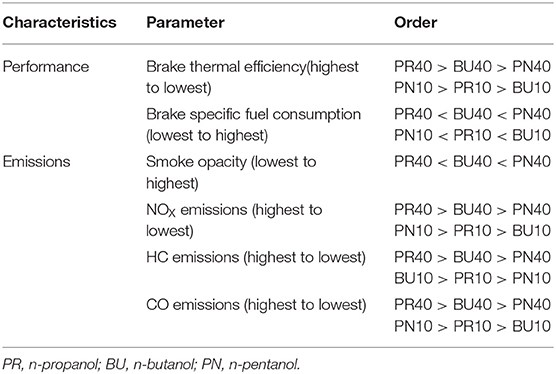
Table 3. Comparative evaluation of diesel engine characteristics for three alcohols/diesel blends (Rajesh Kumar et al., 2016a; Yilmaz et al., 2017).
n-Pentanol/diesel blends except 5% pentanol produced higher CO emissions than did diesel, while higher HC emissions than that of diesel were obtained for all pentanol blends up to 35%. This can be attributed to higher latent heat of evaporation of n-pentanol (Yilmaz and Atmanli, 2017b). Presence of 5 and 35% pentanol in n-pentanol/diesel blends exhibited lower NOx emissions than did diesel, while 10, 20, and 25% pentanol blends produced higher NOx emission than did diesel (Yilmaz and Atmanli, 2017b). However, the subsequent study reported that the addition of pentanol to diesel exhibited a remarkable impact on the reduction of NOx emissions for 10 and 20% pentanol/diesel blends (Yilmaz et al., 2017). Further, Atmanli and Yilmaz (2018) found that the 5, 25, and 35% addition of 1-pentanol to diesel also showed a significant decrease of NOx emissions in engines, loads, and speeds different from previous ones (Yilmaz and Atmanli, 2017b). However, Sridhar et al. (2018) for D80P20 and Prabhu and Ramanan (2018) for D85P15, D75P25, and D65P35 recently reported the reduction of CO, HC, and NOx emissions simultaneously and also a decrease in fuel consumption, which increases in BTE. Meanwhile, Zhang et al. (2018) found for D80P20 that the performance and emission characteristics in terms of NOx, soot, CO, and HC were close to those of the diesel fuel in the six-cylinder, turbocharged, intercooled, heavy-duty common rail direction injection (CRDI) diesel engine.
Compared to diesel fuel, the blended fuel (10 and 20%) of pentanol by volume with ultra-low sulfur diesel can effectively reduce the PM and elemental carbon emissions and exhibits a slight decrease in organic carbon emissions but a significant increase in water-soluble organic carbon emissions (Zhang et al., 2016). Additionally, with the same proportion of butanol or pentanol in the blended fuels, the pentanol/diesel blend shows lower potential to inhibit elemental carbon emissions and solid and volatile particle number emissions than that of the butanol/diesel blend. Four fuels containing 30% by volume of isobutanol, n-pentanol, n-hexanol, and n-octanol in ultra-low sulfur diesel were selected by Rajesh Kumar et al. (2016c) for a comparative study on combustion and emissions of higher-alcohol/diesel blends in CI engine. The results are shown in Table 4.
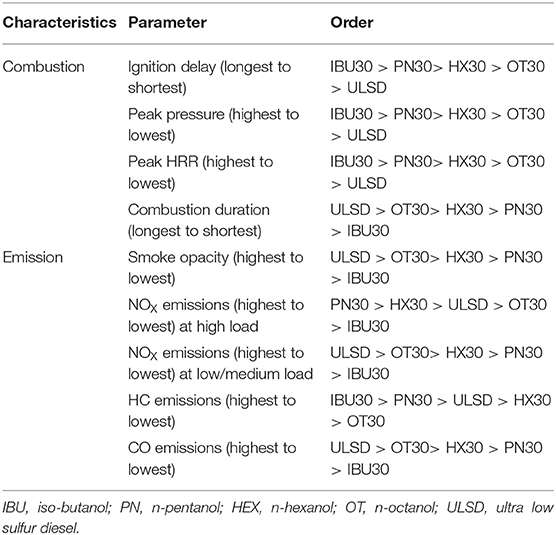
Table 4. Comparative summary of diesel engine characteristics for four higher alcohols/diesel blends (Rajesh Kumar et al., 2016d).
It was concluded by Rajesh Kumar and Saravanan (2015) that 45% n-pentanol/diesel blends can be used in CI engines without any modifications and without causing any visible damage to the engine parts subject to long-term durability tests. The pentanol/diesel blends coupled with EGR technology could simultaneously reduce NOx and soot emissions from the CI engine. Further, when a CN improver, 2-ethylhexyl nitrate (EHN), was added to the D50P50 blend, ignition delay was shortened, BTE increased, and HC and CO emissions were remarkably decreased (Pan et al., 2018). For D50P50, as the engine load increased, CO, HC, and NOx emissions decreased, while soot emission increased (Pan et al., 2019). Therefore, utilization of n-pentanol/diesel blend combined with EGR and EHN resulted in the reduction of HC, CO, NOx, and soot emission simultaneously and a slight decrease of BTE.
At the same EGR rate and injection timing, D70P30 showed a noticeable reduction in NOx and smoke emissions in a single-cylinder diesel engine (de Poures and Sathiyagnanam, 2017). However, CO, HC, and soot emissions except NOx emission were increased as the EGR rate was increased from 10 to 30%. Further, when the injection timing was advanced, all other emission concentrations except NOx decreased.
For D95P5, D90P10, and D80P20, at 25% engine load, the in-cylinder pressure and maximum heat release rate of all blends tested were reduced (Abdullah et al., 2018). CO emissions increased as the engine load and pentanol percentage increased and were higher than those of diesel fuel. However, HC emissions decreased as the engine load increased but increased as the pentanol proportion increased. CO2 emissions were slightly reduced for all fuel blends at different engine loads compared to diesel fuel. NOx emissions increased for all fuel blends tested. At low and high engine loads, there was no remarkable difference in NOx emissions in all fuel blends compared to diesel fuel.
Further, in the experiments of D95P5, D90P10, D80P20, D70P30, and D60P40 conducted on a two-cylinder CRDI diesel engine running at a constant speed of 2,000 rpm for lower and higher loads, Radheshyam et al. (2020) found that at up to 30% of n-pentanol addition, the engine was operated without modification, while the 40% n-pentanol blend showed poor performance compared to neat diesel. With an increase in the concentration of pentanol in the blends, HC and CO emissions were increased, while NOx emissions were decreased. They recommended that D95P5 can be a better choice of alternative fuels, because it has slightly improved BTE, NOx, and CO emissions.
For isopentanol application to diesel engine (D70P30 and D70B30 by mass basis), Yoshimoto et al. (2018) found that the smoke and NOx emissions for isopentanol blend were lower than those for the n-pentanol blends. In addition, regardless of boost pressure and EGR rate, lower smoke emissions for the butanol isomer blends were obtained compared to those obtained for the pentanol isomers blends.
Biodiesel/Pentanol Blends
Spray characteristics
As one of the macroscopic spray characteristics, Ma et al. (2017b) showed that the maximum liquid-phase penetration of n-pentanol/WCO biodiesel blends decreases with an increase in ambient temperature and declines sharply with an increase in n-pentanol concentration. Among various prediction models of maximum liquid-phase penetration (No, 2008), the empirical correlation suggested by Higgins was adopted to model the experimental data. It is noteworthy that the selected model gives a closer fit to the measured data for pentanol/ biodiesel blends, rather than data for neat biodiesel, because the selected model was inherently for arbitrary fuel blends. Meanwhile, the spray penetration decreased, while the spray angle increased as the proportion of pentanol in biodiesel/pentanol blends increased (Li et al., 2018). It should be noted that as an indicator of atomization and evaporation, they suggested the ratio between liquid core area and spray area. The 60% biodiesel/40% pentanol blends showed spray penetration, spray angle, and ratio between the liquid core area and spray area that are similar to those of diesel. Recently, Liu et al. (2019) newly proposed the correlations of liquid length and ignition delay time for biodiesel/pentanol blends (B80P20 and B60P40).
Performance characteristics
According to the engine test results by Yilmaz et al. (2016, 2017), BSFC and exhaust gas temperature increased with the addition of n-pentanol to diesel (D90P10 and D80P20) and biodiesel (B90P10 and B80P20), while the BTE decreased for D90P10 and D80P20. In contrast, B90P10 and B80P20 showed an increase in BTE. Similar results were reported by Devarajan et al. (2017c) who demonstrated the effect of adding pentanol to cashew nut shell oil biodiesel on performance of diesel engine. Zhang and Balasubramanian (2016) and Yilmaz et al. (2016) also found that 10 and 20% pentanol/WCO biodiesel blends resulted in an improvement in BTE and a maximum of 2% increase in the BSFC. These findings are in agreement with the recent results by Mahalingam et al. (2018) for 10 and 20% pentanol/Mahua oil methyl ester (MOME) blends and by Rajasekar (2016) for the Jatropha oil biodiesel/pentanol (B70B30) blend. However, in a single-cylinder water-cooled diesel engine fueled with Karanja oil biodiesel blends along with pentanol addition of 10 and 20% by volume (Jeyakumar and Narayanasamy, 2019), the BTE increased and BSFC decreased with binary blends.
Combustion and emission characteristics
In the study of n-pentanol/WCO biodiesel blends with pentanol fractions of up to 30% in CI engines (Zhu et al., 2016; Yang et al., 2017), in-cylinder pressure and heat release rate of n-pentanol/biodiesel blends increased continuously with an increase in n-pentanol. In addition, the addition of pentanol in biodiesel resulted in the delayed start of combustion and shortened combustion duration. Similar results were recently obtained by Nanthagopal et al. (2018a) for 10, 20, and 30% concentrations of pentanol with C. inophyllum methyl ester (CIME). However, the increase in pentanol proportion (40, 50, and 60% concentrations by volume) in the blend with CIME reduces the in-cylinder pressure (Nanthagopal et al., 2018b). Furthermore, the addition of pentanol with CIME resulted in lower cylinder pressure and heat release rate than did diesel and neat CIME at all engine loads. The ignition delay increased with the pentanol/CIME blends compared to diesel and neat CIME.
According to Ma et al. (2017b), the ignition delay times of n-pentanol/diesel blends decrease with an increase in n-pentanol concentration for most experimental conditions, while neat biodiesel shows a similar or even shorter ignition delay than that shown by n-pentanol blends in low oxygen concentrations and low-temperature conditions. CO and HC emissions of n-pentanol/WCO biodiesel blends are higher than those of diesel and biodiesel, particularly at low engine loads (Zhu et al., 2016). Similarly, Rajasekar (2016) found for Jatropha biodiesel/pentanol blends (B70P30) that smoke opacity and NOx emissions decreased while CO and HC emissions increased. Further, J70P30 resulted in lower CO, NOx, and PM emissions than J70BU30 (butanol) for all loads, while J70P30 produced higher HC emissions than J70BU30 for all loads.
However, Devarajan et al. (2017a,b,c) by adding 10 and 20% pentanol to cashew nut shell biodiesel demonstrated that reductions in CO, HC, NOx, and smoke were observed when compared with neat biodiesel, while the blends were inferior than diesel at all conditions. In addition, the 10% pentanol/biodiesel blend had lower HC emissions than WCO biodiesel for all engine loads, while the 20% pentanol/biodiesel blend had lower HC emissions at lower loads and higher HC emissions at higher loads (Yilmaz et al., 2017). Similar results were obtained by Mahalingam et al. (2018) for 10 and 20% pentanol/MOME. However, at 20% EGR rate, CO and HC emissions increased when compared to 0% EGR rate. However, Sridhar et al. (2018) reported that 1-pentanol/WCO biodiesel blends decreased the emissions of NOx, HC, and CO simultaneously compared to neat biodiesel, with a slight penalty of BTE. It was also observed by Radhakrishnan et al. (2017) that for palm oil biodiesel/pentanol blends (B90P10 and B80P20), CO, HC, NOx, and smoke emissions were reduced compared to neat palm oil biodiesel.
Lower NOx emissions than those of WCO methyl ester were obtained for 10% pentanol/biodiesel blends (Yilmaz et al., 2016), while NOx emissions of the 30% pentanol/biodiesel blend is higher than those of other fuels (Zhu et al., 2016). However, Yilmaz et al. (2017) found out that NOx emissions increased in the case of 10 and 20% pentanol/WCO biodiesel blends. They analyzed that two factors, namely, the oxygen content in the pentanol and higher latent heat of vaporization, may contribute to NOx formation in oxygen-rich regions inside the cylinder. According to the results from an indirect-injection, four-cylinder diesel engine generator (Yilmaz et al., 2016), the 10% n-pentanol/WCO biodiesel blend produced the highest CO and NOx emissions but lowest HC emissions, compared to 10% propanol/WCO biodiesel and 10% n-butanol/WCO biodiesel blends. With the addition of pentanol in biodiesel and the reduction of the particle mass and number concentrations, particularly particles in the nucleation mode (Zhu et al., 2016), n-pentanol/biodiesel blends exhibited a slower soot formation rate and a faster soot oxidation rate than those of neat biodiesel (Ma et al., 2017b).
In the study of the effect of blending n-butanol and n-pentanol with WCO biodiesel at 10 and 20% by volume on engine performance and particulate emissions in a CI engine (Zhang and Balasubramanian, 2016), n-pentanol/biodiesel blends showed a reduction of the particulate mass and elemental carbon emissions, with butanol being more effective than pentanol. Both blended fuels also exhibited a lower emission of total particle-phase polycyclic aromatic HCs and also a lower carcinogenic potential. However, the proportion of particulate-bound organic carbon and water-soluble organic carbon was increased for both blended fuels. The number of particles of pentanol/biodiesel blends was less than that of neat biodiesel in all size groups due to the longer ignition delay time, lower viscosity and boiling point, and higher oxygen content of pentanol. The addition of pentanol leads to a reduction of elemental carbon emissions and an increase of organic carbon at low and medium loads. In addition, the 30% pentanol/biodiesel blend gives lower total particle-phase polycyclic aromatic HC emissions and also a lower benzo[a]pyrene equivalent (BaPeq) compared to neat biodiesel under the all engine loads (Yang et al., 2017). The addition of 10% pentanol to biodiesel was recommended by Zhu et al. (2016) as a suitable replacement ratio for diesel engines. Recently, Nanthagopal et al. (2018a) for 10, 20, and 30% pentanol/CIME and Nanthagopal et al. (2018b) for 40, 50, and 60% pentanol/CIME found that the CO, HC, and soot emissions are higher compared to those for neat CIME and lower than those for diesel fuel, while NOx emissions are higher than those for diesel fuel and lower than those for neat CIME for all engine loads. The addition of n-pentanol of more than 40% to diesel showed a negative effect in terms of performance and combustion, and thus, among all blends, B90P10 in the case of CIME was found to show better performance and emission characteristics (Ashok et al., 2019).
NOx and smoke emissions were reduced for pentanol and MOME blends compared to neat MOME, but they were decreased more at the 20% EGR rate compared to the 0% EGR rate (Mahalingam et al., 2018).
Recently, in the experiments performed by Jeyakumar and Narayanasamy (2019) in a single-cylinder water-cooled diesel engine fueled with Karanja oil biodiesel blends along with pentanol addition of 10 and 20% by volume, reductions of CO, HC, NOx, and smoke emissions were observed.
Kerosene/Pentanol Blends
Unlike with the diesel/pentanol blends, the higher levels of pentanol addition to kerosene increase the viscosity and oxygen content of the kerosene/pentanol blends. Two blended fuels, K80P20 (80% kerosene and 20% pentanol) and K60P40, and diesel and aviation kerosene (RP-3) were tested in an aircraft CI engine by Chen et al. (2017a,b). The indicated thermal efficiency of K60P40 was highest among all fuels tested. The indicated specific fuel consumption values of K80P20 and K60P20 were demonstrated to be lower than that of baseline diesel fuel under all the injection timings tested. Pentanol addition prolonged the ignition delay of kerosene/pentanol blends and reduced the combustion duration compared to the baseline diesel. Generally, advancing the injection timing reduced the ignition delay but increased the total combustion duration. Compared to kerosene, the soot and CO emissions of kerosene/pentanol blends decreased at high loads with a slight difference in terms of NOx and HC emissions. However, at low loads, pentanol addition increased the CO and HC emissions.
SVO/Pentanol Blends
The use of alcohols as blends in proper proportion with SVO provides significant improvement in the kinematic viscosity and flash point of SVO. Neem oil and its blends of 5, 10, 15, and 20 vol% with ethanol, 1-propanol, 1-butanol, and 1-pentanol, respectively, were evaluated by Sivalakshmi and Balusamy (2011) and compared with diesel operation. BTE was slightly higher with an increase in alcohol content in neem oil/alcohol blends compared to neat neem oil. Instead of BSFC, brake-specific energy consumption, which is a proper parameter for comparing the engine performance of fuels having different heating values and density, was adopted, and there was a decreasing trend in brake-specific energy consumption with an increase in alcohol content compared to neat neem oil. The CO and HC emissions with neem oil/alcohol blends were observed to be lower with respect to that of pure neem oil at higher loads, while they were higher at low loads. Compared to neat neem oil, neem oil/alcohol blends showed significantly reduced smoke intensity and very slightly reduced NOx emissions. In summary, the use of pentanol as blends in proper proportion with SVO such as neem oil provides improved performance and emission characteristics.
The experimental studies related to several pentanol-added binary blends in CI engines are summarized in Tables 5, 6. Fuel type, engine type, compression ratio, displacement, and operating conditions can be found in these tables.
Ternary Blends
Four different kinds of ternary blends can be found in the literature, that is, diesel/biodiesel/pentanol, diesel/SVO/pentanol, biodiesel/SVO/pentanol, and diesel/alcohol/pentanol blends.
Diesel/Biodiesel/Pentanol Blends
Instead of binary blends such as diesel/pentanol (D70P30) and diesel/biodiesel (D70B30) blends, Li et al. (2015b) recommended diesel/biodiesel/pentanol blends (D40B30P30) for a fuel design approach in order to improve the performance, combustion, and emission characteristics in a single-cylinder-mode CI engine.
In the comparative study of the effect of ternary blends of diesel, WCO biodiesel, and n-propanol, n-butanol, or 1-pentanol on performance and emissions of a CI engine (Atmanli, 2016b), three different 20% alcohols were mixed with 40% diesel and 40% biodiesel blend. Lower BSFC and higher BTE and exhaust gas temperature were obtained for the D40B40P20 blend relative to the D50B50 blend.
The addition of n-pentanol to high concentrations of WCO biodiesel (85 and 90%)/diesel (5%) blends leads to an increase of cylinder gas pressure and ignition delay and a decrease of combustion duration (Babu and Anand, 2017). The lower CN of n-pentanol prolonged the ignition delay time, and the higher ignition delay allows more combustible mixture being prepared with the delay period, which results in a higher cylinder gas pressure. Higher brake-specific energy consumption and lower BTE were obtained for B90D5P5 and B85D5P10 compared with neat diesel.
Similar with the results of Atmanli (2016b), in the case of diesel/polanga seed biodiesel/pentanol blends (D80B10P10, D70B15P15, and D60B20P20), increased BTE, higher brake power, and reduced BSFC in contrast to diesel and D80B20 blend were obtained (Imdadul et al., 2016a). However, Yilmaz and Atmanli (2017a) for WCO methyl ester and Yesilyurt et al. (2018) for yellow mustard oil methyl ester reported that ternary blends of diesel/biodiesel/1-pentanol (5, 10, and 20%) showed decreased BTE, increased BSFC, and increased exhaust gas temperature.
For combustion and emission characteristics, at no loads, ternary blends (D70B20P10, D60B20P20, and D50B20P30) produced lower NOx emissions than did neat diesel and neat biodiesel fuel (Clayson, 2016). However, as the load increased, ternary blends showed the increase of NOx emissions. Further, in terms of NOx emissions, the D50B20P30 blend stayed consistently below the diesel fuel. At all engine loads, CO and HC emissions for ternary blends were higher than those for neat diesel, neat biodiesel, and D80B20 blend. However, Atmanli (2016b) reported reduced HC and NOx but increased CO for D40B40P20 compared to D50B50. However, no smoke emissions data were presented. It is interesting to note that as opposed to pentanol, the ternary blends of 10% ethanol and methanol produced lower CO emissions compared to the diesel/biodiesel blend (Alptekin et al., 2015; Yasin et al., 2015).
The ternary blends (D70B20P10, D65B20P15, and D60B20P20) showed higher NO emissions and reduced smoke intensity compared to binary blends (D80B20). The increase in pentanol content in ternary blends considerably increased NO emissions and decreased CO and HC emissions (Imdadul et al., 2016a). In addition, CO and HC emissions of ternary blends remarkably reduced compared with those of the diesel and biodiesel blends (Imdadul et al., 2016b). Similarly, Babu and Anand (2017) also found that B90D5P5 and B85D5P10 showed lower emissions of CO, HC, and smoke than did neat diesel and biodiesel, whereas NO emissions for B90D5P5 and B85D5P10 were higher than those for neat biodiesel, while lower than those for diesel. Among biodiesel/diesel/n-pentanol and biodiesel/diesel/n-hexanol (B90D5P5, B85D5P10, B90D5H5, and B85D5H10), the pentanol is more effective than hexanol in terms of CO, HC, NOx, and smoke emissions.
On the contrary, for D75B20P5, D70B20P10, and D60B20P20, addition of pentanol to diesel/biodiesel blends increased CO and HC emissions (Yilmaz and Atmanli, 2017a). However, for 5 and 10% pentanol-blended fuels (D75B20P5 and B70B20P10), Yesilyurt et al. (2018) found that n-pentanol could be an effective additive to decrease NOx, smoke opacity, and CO emissions for the binary blends of diesel and biodiesel.
According to Imdadul et al. (2016c, 2017), although CN improvers such as EHN and di-tertiary-butyl peroxide when added with pentanol-treated palm oil biodiesel blends at a proportion of 1,000 and 2,000 ppm have the potential of decreasing NO and smoke emissions compared with biodiesel and ternary alcohol blends, the level of NO emission was still higher than that in diesel. In addition, CO and HC increased slightly with cetane improver, but not more than those of diesel. They had obtained CN of the blends by calculating the saponification number (SN) and iodine value (IV) of the fuels using the following equations.
where Ai is the proportion of each component, D is the number of double bonds, and MWi is the molecular weight of each component.
n-Pentanol was introduced by Ramakrishnan et al. (2018a as an additive to CIME/diesel blends at 10, 15, and 20% by volume. NO and CO2 emissions increased with an increase in pentanol addition in ternary blends compared with CIME20 and neat diesel fuel. CO and HC emissions were reduced with an increase in pentanol percentage in the ternary blends compared with CIME20 and neat diesel. They suggested that 20% pentanol addition with the CIME/diesel blend is suitable for improved performance and combustion characteristics of a diesel engine without any engine modifications. Similarly, the use of n-pentanol (10%) as a fuel additive significantly reduced the smoke opacity for diesel/tamarind seed methyl ester blend (D80B20P10) in addition to the decrease of CO and HC emissions, while the specific fuel consumption and NOx emissions were marginally increased (Dhana Raju et al., 2018).
It was confirmed by Imdadul et al. (2016b) and Yesilyurt et al. (2018) that n-pentanol added ternary blends revealed better performance and emission results than 1-butanol blends. Similar results were reported by Ghadikolaei et al. (2018) with diesel, WCO biodiesel, and lower alcohols (methanol and ethanol) and higher alcohols (2-propanol, n-butanol, and n-pentanol) ternary blends, which were blended with various blending mass percentages to obtain blended fuels with constant fuel oxygen, nearly constant carbon and hydrogen contents, and almost constant lower heating values. They found that all the blended fuels with alcohol can reduce both NOx and PM emissions with a higher PM reduction effect. They also observed that diesel, biodiesel, and methanol ternary blends exhibited the best engine performance and had the lowest emissions compared to all the other tested fuels.
Furthermore, based on the response surface methodology, it was noticed that ternary blend-fueled engine exhibited better performance and emission at a compression ratio of 17.5, P20CI20D60 blend, and 2.5 brake mean effective pressure load conditions (Ramakrishnan et al., 2018b). However, Appavu et al. (2019a) reported that the addition of pentanol to diesel/Jatropha biodiesel blends (D60B20P20) resulted in reduction of CO and NOx emissions and smoke opacity, simultaneously. However, the data for HC emission were not reported.
Titanium oxide was recently introduced by Manigandan et al. (2019) as an additive to improve the combustion, performance, and emission characteristics of diesel engines fueled with diesel, corn oil methyl ester, and pentanol blends. Remarkable reductions were found on CO, HC, and smoke emissions with the use of biodiesel compared to diesel. In addition, more reductions in NOx and particulate emission were obtained with D50B25P20 with 5% TiO2 at 300 ppm.
Diesel/SVO/Pentanol Blends
Transesterification of SVO to biodiesel is time-consuming, complex, and expensive. However, mixing alcohols with SVO is found to be a cheaper, practical, and easier method than transesterification to reduce the viscosity of vegetable oils, with no need for engine modification or complex chemical reactions (Dhanasekaran et al., 2017). However, there are many hurdles to using alcohol/SVO blends, particularly lower alcohols, in diesel engines, such as lower CNs, limited solubility in diesel, phase separation in lower temperature, and water tolerance in alcohol/SVO blends. Therefore, the effect of EHN addition as a cetane improver on fuel properties and the performance and emission characteristics of diesel engine fueled with diesel/SVO/n-pentanol microemulsion blends were investigated by Ileri (2016) for sunflower oil and Atmanli (2016a) for hazelnut oil. BSFC values were decreased due to EHN addition, but no differences in brake power and brake mean effective pressure values were observed with or without EHN. With the addition of EHN to D70S20P10, CO emissions increased, while HC and NOx emissions decreased.
With an objective to substitute diesel fuel by 50% with up to 45% WCO (restaurant yellow grease) and up to 20% n-pentanol, three ternary blends (D50WCO45P5, D50WCO40P10, and D50WCO30P20) were selected by Ravikumar and Saravanan (2017) and Dhanasekaran et al. (2017) to investigate the performance, combustion, and emissions of a stationary CI engine. Addition of n-pentanol showed viscosity reduction of up to 45% and CN and density comparable to those of diesel. Addition of n-pentanol to D50WCO50 exhibited improved BSFC for all ternary blends, and BTE for D50WCO30P20 blends was comparable to that of diesel due to improved atomization. Addition of n-pentanol leads to slightly longer ignition delays due to the decrease in CN of the ternary blends. The peak pressure and heat release rate increased with increase in pentanol content in D50WCO50 due to the improved combustion process as a result of increased oxygen content in the blends. HC emissions increased and CO emissions remained unchanged with increase in n-pentanol in the blends. NOx emissions increased with increasing n-pentanol content in D50WCO50 but remained lower than those in diesel, while smoke opacity reduced with an increase in n-pentanol proportion.
A comparative study of ternary blends of three alcohols (n-propanol, n-butanol, and n-pentanol) with WCO and diesel for performance and emission in a single-cylinder CI engine using response surface methodology was carried out by Krishnamoorthy et al. (2018). They concluded that pentanol was a suitable alcohol because the ternary blend D50WCO30P20 injected at 23 CAD before top dead center (bTDC) at 15% EGR showed the highest desirability.
To improve the performance and reduce NOx and smoke emissions simultaneously from a CI engine fueled with SVO/diesel blends, 5 and 10% of 1-pentanol were added by Mathan Raj et al. (2018) with 20 and 40% SVO blends. A considerable improvement in performance was obtained with the addition of 1-pentanol in Simarouba glauca oil and diesel blends (SVO20 and SVO40 blends). Higher CO, HC, NOx, and smoke emissions associated with SVO20 and SVO40 blends were reduced with 1-pentanol addition.
Biodiesel/SVO/Pentanol Blends
The effect of methanol and pentanol addition (M20 and P20) with Karanja oil methyl ester and SVO (orange oil) blend and activated carbon-based after-treatment system in a single-cylinder CI engine was compared by Subramanian et al. (2017). They observed that the addition of methanol compared to pentanol is more effective in biodiesel/SVO blend in terms of the simultaneous reduction of NO, smoke, and CO2 emissions. However, with an after-treatment system, an increase in HC and CO emissions and decreased thermal efficiency were noticed due to backpressure effects.
Diesel/Alcohol/Pentanol Blends
As an effective additive for methanol/diesel blends, n-pentanol is known to have many advantages over biodiesel, such as higher oxygen, lower viscosity and surface tension, and higher volatility. Therefore, the effect of D70M15P15 and D70M10P20 on combustion and emission characteristics of a CRDI diesel engine was recently examined by Chen et al. (2019). The ternary blends showed longer ignition delays, higher peak heat release rate, shorter combustion duration, and higher peak combustion temperature than did diesel at low and partial loads. They found that D70M15P15 has the lowest soot and ultrafine particle emissions and that a suitable delay of injection timing can reduce the soot and NOx emissions simultaneously compared to diesel, particularly at medium and high loads.
Pentanol is also known as a strong candidate to improve the blending stability of hydrous ethanol and diesel. Pentanol addition to hydrous ethanol/diesel blend (P10E10D80) showed lower peak cylinder pressure (Nour et al., 2019). Ignition delay and combustion duration also increased for the ternary blend. In addition, smoke, NOx, CO, and CO2 emissions were reduced compared with those of D100.
Table 7 shows the summary of experimental studies on the utilization of several pentanol-added ternary and quaternary blends in CI engines. This table includes fuel type, engine type, compression ratio, displacement, and operating conditions.
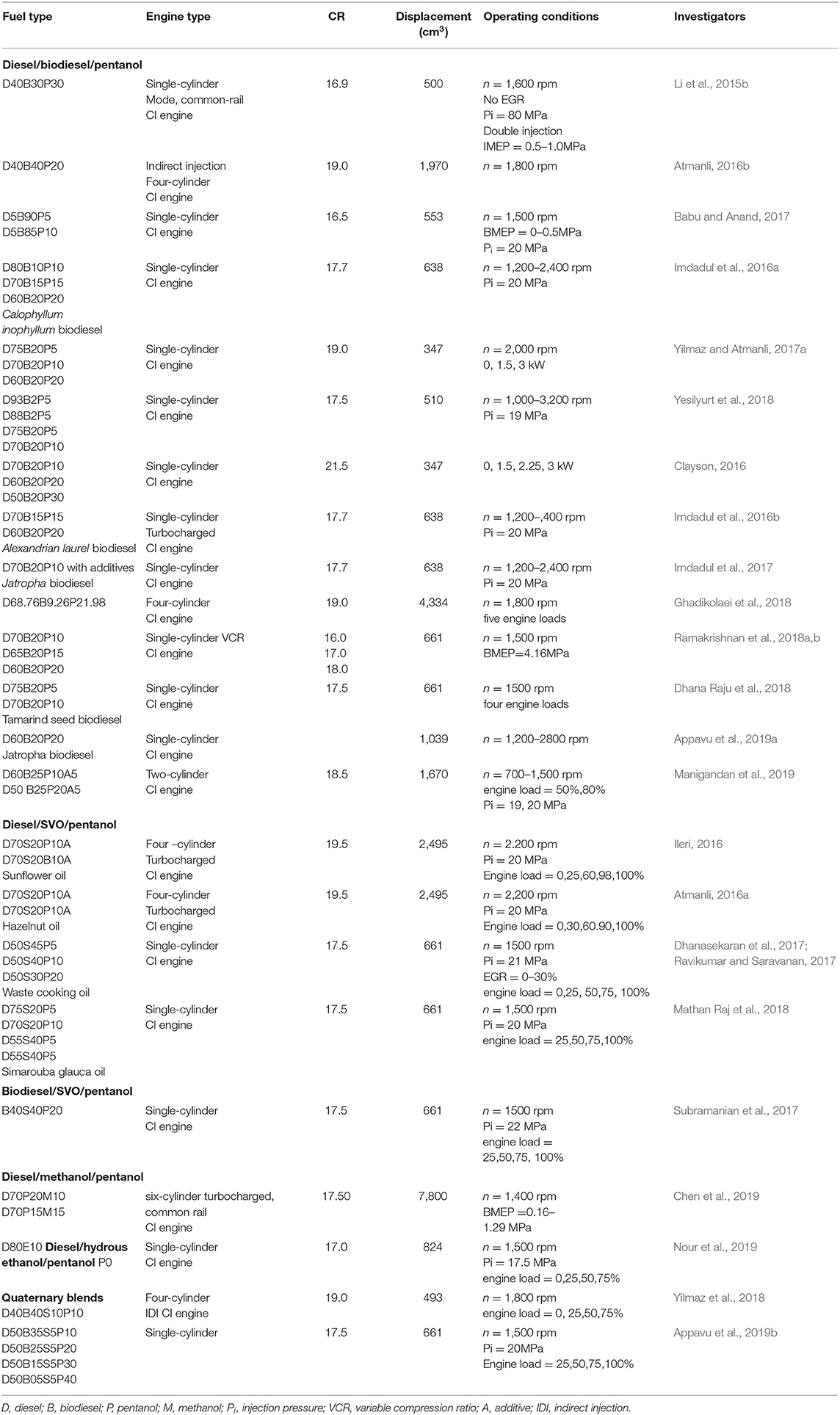
Table 7. Summary of experimental studies related to pentanol added ternary and quaternary blends in CI engine.
Quaternary Blends
In order to establish 20% biofuel in CI engines by 2020 in the European Union, a quaternary blend of diesel (D), biodiesel (B: WCO methyl ester), SVO (soybean oil), and pentanol (D40B40S10P10) was tested by Yilmaz et al. (2018) in an indirect-injection, four-cylinder diesel engine generator. The addition of pentanol to ternary blends increased CO and HC emissions due to lower CN and higher latent heat of evaporation. NOx emissions of the quaternary blends containing pentanol were lower than those of D50B50 blends. However, no smoke emissions data are presented.
Recently, apart from the biodiesel produced from edible oils such as soybean oil, inedible Jatropha oil and Jatropha biodiesel were introduced by Appavu et al. (2019b) to quaternary blends such as D50B35S5P10, D50B25S5P20, D50B15S5P30, and D50B5S5P40. All the quaternary blends showed high BSFC in comparison with neat diesel fuel. Accordingly, BTE of all quaternary blends was higher than that of neat biodiesel. D50B5S5P40 had maximum peak pressure with increased heat release rate. As the concentration of pentanol was increased, CO, HC, and smoke emissions were decreased, whereas NOx emissions were increased. They recommend that 40% pentanol addition in diesel/biodiesel/SVO can be a suitable fuel blend for improving performance and minimizing emissions with marginal compromise in NOx emissions.
Utilization of Pentanol in Advanced CI Engines
Very few information related to the application of pentanol to advanced CI engine is available in the literature.
The combustion characteristics of neat isopentanol in a six-cylinder diesel engine with homogeneous charge compression ignition (HCCI) combustion mode under wide ranges of speed were investigated by Yang et al. (2010). Using isopentanol, they showed that no low-temperature heat release was found in ethanol-fueled HCCI (No, 2019) even at very low engine speeds or with considerable intake boost. All data were acquired with satisfying clean (ISNOx <0.1 g/kWh, no soot), efficient (combustion efficiency >96%), stable (COVIMEP <2%), and non-knocking (RI <5 MW/m2) combustion. They concluded that isopentanol can be a good alternative fuel in its neat form or as a blend with gasoline in an HCCI engine.
As one type of advanced CI combustion schemes, the partially premixed combustion (PPC) mode fueled with alcohols such as methanol, ethanol, and butanol has been widely studied (No, 2019). According to Rajesh Kumar and Saravanan (2016b), the combination of late injection timing of 21° CA bTDC, a low EGR of 30%, and a pentanol/diesel blend (D60P40) can obtain a PPC mode and reduce NOx and smoke emissions simultaneously with a slight decrease in performance. D60P40 presented a shorter ignition delay, lower peak pressure, and lower premixed heat release rate than did the butanol/diesel blend (D60Bu40). It should be noted that CO emissions remained low, but HC emissions increased at all EGR rates (10, 20, and 30%). To mitigate NOx and PM emissions simultaneously, dimethyl ether (DME) and n-pentanol were blended with diesel, and their effect on emission characteristics of a CI engine under low-temperature combustion mode was recently studied by Raza et al. (2019). Although PM mass and PM number concentrations as well as NOx emissions decreased for binary (D85DME15 and D65P35) and ternary blends (D60DME20P20), CO and THC emissions were higher compared to those of neat diesel.
To summarize, the physicochemical properties of pentanol are favorable to achieve advanced CI engines, and higher reactivity at lower temperatures is necessary for engine operation, such as HCCI, PPC, reaction controlled compression ignition (RCCI), and dual fuel combustion. However, advanced CI engines fueled with pentanol or blends have not been studied extensively.
Discussion and Summary
Among several C5 alcohols, n-pentanol and isopentanol have been mainly investigated as a blending component for CI engines. More research on the utilization of neat pentanol in CI engines is required because several properties such as density, viscosity, and boiling point of pentanol as shown in Table 1 are closer to those of diesel fuel.
Many studies demonstrated that an increase in pentanol content in the binary blends causes the ignition delay to increase due to their lower CN. The peak of in-cylinder pressure and heat release rate increased with increase in pentanol proportion because of enhanced premixed combustion phase caused by the prolonged ignition delay.
According to the optimization study with an objective to minimize NOx, smoke, and BSFC with maximum BTE, diesel/n-pentanol blends injected 24 CAD bTDC with 10% EGR showed the best performance and emission characteristics. However, in the comparative study of advanced biofuels such as dimethyl carbonate (DMC), isobutanol, and n-pentanol, isobutanol/diesel blends injected at 22 CAD bTDC with no EGR were found to be optimum. Generally, the cetane number, oxygen content, and latent heat of evaporation affect the combustion positively in terms of NOx reduction. CO emissions tend to increase because of the lack of oxygen or rich fuel/air ratio. Moreover, larger fuel droplets and ignition delay due to higher density and viscosity cause slower evaporation and higher CO emissions. HC emissions can increase due to higher density and viscosity of fuels which create fuel-rich regions inside the cylinder.
The diesel/pentanol blends generally produced higher CO and HC emissions than did diesel fuel. However, the diesel/pentanol blends coupled with EGR technology and a cetane improver could simultaneously reduce HC, CO, NOx, and soot emissions from CI engines.
Up to 45–50% diesel/n-pentanol blends can be safely used in diesel engines without any engine modification or any additive. However, in terms of kinematic viscosity, lubricity, and oxidation stability of diesel/pentanol blends, the use of pentanol should be limited to concentrations below 10%. Therefore, the use of additives or fatty acid esters would be required for the increase of pentanol proportion. As a result, ternary blends such as diesel/biodiesel/pentanol and diesel/SVO/pentanol were investigated widely by many researchers. Generally, The BTE increased and BSFC decreased with biodiesel/pentanol binary blends, and reduced CO, HC, NOx, and smoke emissions were obtained.
Among four different kinds of ternary blends, diesel/biodiesel/pentanol blends were mainly investigated by researchers. The emission characteristics in terms of CO, HC, NOx, and smoke opacity showed the different trends according to the pentanol percentage in the ternary blends, particularly <10% and more than 10%. Generally, the increase in pentanol content in ternary blends considerably increased NO emission and decreased CO and HC emissions. In addition, CO and HC emissions of ternary blends remarkably reduced compared with the diesel and biodiesel blends. The ternary blends of n-pentanol, biodiesel, and diesel showed better performance and emission characteristics than did the ternary blends of n-butanol, biodiesel, and diesel. In addition, pentanol revealed less exhaust emissions than n-butanol when blended with diesel/biodiesel blends. More researches for the quaternary blends of pentanol are required to use more biofuels in future CI engines.
Author Contributions
The author confirms being the sole contributor of this work and has approved it for publication.
Conflict of Interest
The author declares that the research was conducted in the absence of any commercial or financial relationships that could be construed as a potential conflict of interest.
Abbreviations
BSFC, brake-specific fuel consumption; bTDC, before top dead center; BTE, brake thermal efficiency; CAD, crank angle degree; CI, compression ignition; CIME, Calophyllum inophyllum methyl ester; CN, cetane number; COV, coefficient of variance; CRDI, common rail direct injection; EGR, exhaust gas recirculation; EHN, 2-ethylhexyl nitrate; HCCI, homogeneous charge compression ignition; IC, internal combustion; MOME, Mahua oil methyl ester; PPC, partially premixed combustion; RCCI, reaction controlled compression ignition; RI, ringing intensity; SI, spark ignition; SVO, straight vegetable oil; WCO, waste cooking oil.
References
Abdullah, Z., Suhaimi, H., and Ada, A. (2018). Effect of pentanol-diesel fuel blends on thermo-physical properties, combustion characteristics, engine performance and emissions of a diesel engine. Int. J. Automot. Mech. Eng. 15, 5435–5450. doi: 10.15282/ijame.15.3.2018.3.0418
Alptekin, E., Canakci, M., Ozsezen, A. N., Turkcan, A., and Sanli, H. (2015). Using waste animal fat based biodiesels-bioethanol-diesel fuel blends in a DI diesel engine. Fuel 157, 245–254. doi: 10.1016/j.fuel.2015.04.067
Appavu, P., Madhavan, V. R., Venu, H., and Jayaraman, J. (2019a). A novel alternative fuel mixture (diesel-biodiesel-pentanol) for existing unmodified DI diesel engine: performance and emission characteristics. T. Can. Soc. Mech. Eng. 44, 1–9. doi: 10.1139/tcsme-2019-0049
Appavu, P., Ramanan, V., and Venu, H. (2019b). Quaternary blends of diesel/diodiesel/vegetable oil/pentanol as potential alternative feedstock for existing unmodified biodiesel engine: performances, combustion and emission characteristics. Energy 186:115856. doi: 10.1016/j.energy.2019.115856
Ashok, B., Jeevanantham, A. K., Nanthagopal, K., Saravanan, B., Senthil Kumar, M., and Johny, A., et al. (2019) An experimental analysis on the effect of n-pentanol Calophyllum Inophyllum biodiesel binary blends in CI engine characteristics. Energy 173, 290–305. doi: 10.1016/j.energy.2019.02.092.
Atmanli, A. (2016a). Effects of a cetane improver on fuel properties and engine characteristics of a diesel engine fueled with the blends of diesel, hazelnut oil and higher carbon alcohol. Fuel 172, 209–217. doi: 10.1016/j.fuel.2016.01.013
Atmanli, A. (2016b). Comparative analyses of diesel-waste oil biodiesel and propanol, n-butanol or 1-pentanol blends in a diesel engine. Fuel 176, 209–215. doi: 10.1016/j.fuel.2016.02.076
Atmanli, A., and Yilmaz, N. (2018). A comparative analysis of n-butanol/diesel and 1-pentanol/diesel blends in a compression ignition engine. Fuel 234, 161–169. doi: 10.1016/j.fuel.2018.07.015
Awad, O. I., Mamat, R., Ali, O. M., Sidik, N. A. C., Yusaf, T., Kadirgama, K., et al. (2018). Alcohol and ether as alternative fuels in spark ignition engine: a review. Renew. Sustain. Energy Rev. 82, 2586–2605. doi: 10.1016/j.rser.2017.09.074
Babu, D., and Anand, D. (2017). Effect of biodiesel-diesel-n-pentanol and biodiesel-diesel-n-hexanol blends on diesel engine emission and combustion characteristics. Energy 133, 761–776. doi: 10.1016/j.energy.2017.05.103
Babu, V., Murthy, M., and Rao, A. P. (2017). Butanol and pentanol: the promising biofuels for CI engines- a review. Renew. Sustain. Energy Rev. 78, 1068–1088. doi: 10.1016/j.rser.2017.05.038
Campos-Fernandez, J., Arnal, J. M., Gomez, J., and Dorado, M. P. (2012). A comparison of performance of higher alcohols/diesel fuel blends in a diesel engine. Appl. Energy 95, 267–275. doi: 10.1016/j.apenergy.2012.02.051
Campos-Fernandez, J., Arnal, J. M., Gomez, J., Lacalle, N., and Dorado, M. P. (2013). Performance tests of a diesel engine fueled with pentanol/diesel fuel blends. Fuel 107, 866–872. doi: 10.1016/j.fuel.2013.01.066
Cano-Gomez, J. J., and Iglesias-Silva, G. A. (2019). A new correlation for the prediction of kinematic viscosities of biodiesel + higher alcohols blends at atmospheric pressure. Fuel 237, 1254–1261. doi: 10.1016/j.fuel.2018.10.038
Chang, Y., Jia, M., Niu, B., Zu, Z., Liu, Z., Li, Y., et al. (2018). Construction of a skeletal oxidation mechanism of n-pentanol by integrating decoupling methodology, genetic algorithm, and uncertainty quantification. Combust. Flame 194, 15–27. doi: 10.1016/j.combustflame.2018.04.012
Chen, H., Su, X., He, J., and Xie, B. (2019). Investigation on combustion and emission characteristics of a common rail diesel engine fueled with diesel/n-pentanol/methanol blends. Energy 167, 297–311. doi: 10.1016/j.energy.2018.10.199
Chen, L., Ding, S., Liu, H., Lu, Y., Li, Y., and Roskilly, A. P. (2017a). Comparative study of combustion and emissions of kerosene(RP-3), kerosene-pentanol blends and diesel in a compression ignition engine. Appl. Energy 203, 91–100. doi: 10.1016/j.apenergy.2017.06.036
Chen, L., Raza, M., and Xiao, J. (2017b). Combustion analysis of an aviation compression ignition engine burning pentanol-kerosene blends under different injection timings. Energy Fuels 31, 9429–9437. doi: 10.1021/acs.energyfuels.7b00813
Clayson, R. A. (2016). Emissions and performance characteristics of a compression ignition engine fueled with diesel-biodiesel-pentanol blends (MS. thesis). Mechanical Engineering Dept, New Mexico Institute of Mining and Technology, Socorro, NM, Unites States.
Dayma, G., Togbe, C., and Dagaut, P. (2011). Experimental and detailed kinetic modeling study of isoamyl alcohol(isopentanol) oxidation in a jet-stirred reactor at elevated pressure. Energy Fuels 25, 4896–4898. doi: 10.1021/ef2012112
de Poures, M. V., and Sathiyagnanam, A. P. (2017). Effect of n-pentanol addition on performance and emission characteristics of a single cylinder DI diesel engine under the influence of different EGR and injection timings. Int. J. Eng. Trends Tech. 54, 203–208. doi: 10.14445/22315381/IJETT-V54P229
Devarajan, Y., Jayabal, R. K., Ragupathy, D., and Venu, H. (2017a). Emission analysis on second generation biodiesel. Front. Environ. Sci. Eng. 11:3. doi: 10.1007/s11783-017-0891-0
Devarajan, Y., Munuswamy, D. B., and Nagappan, B. (2017b). Emissions analysis on diesel engine fueled with cashew nut shell biodiesel and pentanol blends. Environ. Sci. Pollut. Res. 24, 13136–13141. doi: 10.1007/s11356-017-8915-7
Devarajan, Y., Nagappan, B. K., and Munuswamy, D. B. (2017c). Performance and emissions analysis on diesel engine fueled with cashew nut shell biodiesel and pentanol blends. Korean J. Chem. Eng. 34, 1021–1026. doi: 10.1007/s11814-016-0364-3
Dhana Raju, V., Kishore, P. S., and Yamini, K. (2018). Experimental studies on four stroke diesel engine fueled with tamarind seed oil as potential alternative fuel for sustainable green environment. Eur. J. Sustain. Dev. 2:10. doi: 10.20897/ejosdr/78489
Dhanasekaran, R., Krishnamoorthy, V., Rana, D., Saravanan, S., Nagendran, A., and Kumar, B. R. (2017). A sustainable and eco-friendly fueling approach for direct-injection diesel engines using restaurant yellow grease and n-pentanol in blends with diesel fuel. Fuel 193, 419–431. doi: 10.1016/j.fuel.2016.12.030
Ghadikolaei, M. A., Cheung, C. S., and Yung, K.-F. (2018). Study of combustion, performance and emissions of diesel engine fueled with diesel/biodiesel/alcohol blends having the same oxygen concentration. Energy 157, 258–269. doi: 10.1016/j.energy.2018.05.164
Heufer, K. A., Bugler, J., and Curran, H. J. (2013). A comparison of longer alkane and alcohol ignition including new experimental results for ne-pentanol and n-hexanol. Proc. Combust. Inst. 34, 511–518. doi: 10.1016/j.proci.2012.05.103
Heufer, K. A., Sarathy, S. M., Curran, H. J., Davis, A. C., Westbrook, C. K., and Pitz, W. J. (2012). Detailed kinetic modeling study of n-pentanol oxidation. Energy Fuels 26, 6678–6685. doi: 10.1021/ef3012596
Huang, H., Lv, D., Zhu, J., Chen, Y., Zhu, Z., Pan, M., et al. (2018). Development and validation of a new reduced diesel-n-pentanol mechanism for diesel engine applications. Energy Fuels 32, 9934–9948. doi: 10.1021/acs.energyfuels.8b02083
Ileri, E. (2016). Experimental study of 2-ethylhexyl nitrate effects on engine performance and exhaust emissions of a diesel engine fueled with n-butanol or 1-pentanol diesel-sunflower oil blends. Energy Convers. Manage. 118, 320–330. doi: 10.1016/j.enconman.2016.04.015
Imdadul, H. K., Masjuki, H. H., Kalam, M. A., Zulkifli, N. W. M., Alabdulkarem, A., Kamruzzaman, M., et al. (2016b). A comparative study of C4 and C5 alcohol treated diesel-biodiesel blends in terms of diesel engine performance and exhaust emission. Fuel 179, 281–288. doi: 10.1016/j.fuel.2016.04.003
Imdadul, H. K., Masjuki, H. H., Kalam, M. A., Zulkifli, N. W. M., Alabdulkarem, A., Rashed, M. M., et al. (2016a). Higher alcohol-biodiesel-diesel blends: an approach for improving the performance, emission, and combustion of a light-duty diesel engine. Energy Convers. Manage. 111, 174–185. doi: 10.1016/j.enconman.2015.12.066
Imdadul, H. K., Masjuki, H. H., Kalam, M. A., Zulkifli, N. W. M., Alabdulkarem, A., Rashed, M. M., et al. (2016c). Influence of ignition improver additive on ternary (diesel-biodiesel-higher alcohol) blends thermal stability and diesel engine performance. Energy Convers. Manage. 123, 252–264. doi: 10.1016/j.enconman.2016.06.040
Imdadul, H. K., Rashed, M. M., Shahin, M. M., Masjuki, H. H., Kalam, M. A., Kamruzzaman, M., et al. (2017). Quality improvement of biodiesel blends using different promising fuel additives to reduce fuel consumption and NO emission from CI engine. Energy Convers. Manage. 138, 327–337. doi: 10.1016/j.enconman.2017.01.077
Jeyakumar, N., and Narayanasamy, B. (2019). Effect of pentanol-biodiesel blends on performance and emission characteristics of diesel engine. Int. J. Ambient Energy. doi: 10.1080/01430750.2019.1568910
Jin, C., Pang, X., Zhang, X., Wu, S., Ma, M., Xiang, Y., et al. (2019). Effects of C3-C5 alcohols on solubility of alcohols/diesel blends. Fuel 236, 65–74. doi: 10.1016/j.fuel.2018.08.129
Katoch, A., Alfazazi, A., Sarathy, S. M., Chauhan, A., Kumar, R., and Kumar, S. (2019). Measurement of laminar burning velocity of n-pentanol + air mixtures at elevated temperatures and a skeletal kinetic model. Fuel 237, 10–17. doi: 10.1016/j.fuel.2018.09.145
Kohler, M., Kathrotia, T., Obwald, P., Fischer-Tammer, M. L., Moshammer, K., and Riedel, U. (2015). 1-,2- and 3-pentanol combustion in laminar hydrogen flames - a comparative experimental and modeling study. Combust. Flame 162, 3197–3209. doi: 10.1016/j.combustflame.2015.05.007
Krishnamoorthy, V., Dhanasekaran, R., Rana, D., Saravanan, S., and Rajesh Kumar, B. (2018). A comparative assessment of ternary blends of three bio-alcohols with waste cooking oil and diesel for optimum emissions and performance in a CI engine using response surface methodology. Energy Convers. Manage. 156, 337–357. doi: 10.1016/j.enconman.2017.10.087
Lapuerta, M., Garcia-Contreras, R., Campos-Fernandez, K., and Dorado, M. P. (2012). Stability, lubricity, viscosity and cold-flow properties of alcohol-diesel blends. Energy Fuels 24, 4497–4502. doi: 10.1021/ef100498u
Li, F., Fu, W., Yi, B., Song, L., Liu, T., Wang, X., et al. (2018). Comparison of macroscopic spray characteristics between biodiesel-pentanol blends and diesel. Exp. Thermal Fluid Sci. 98, 523–533. doi: 10.1016/j.expthermflusci.2018.07.003
Li, F., Yi, B., Fu, W., Song, L., Liu, T., Hu, H., et al. (2019). Experimental study on spray characteristics of long-chain alcohol-diesel fuels in a constant volume chamber. J. Energy Inst. 92, 94–107. doi: 10.1016/j.joei.2017.11.002
Li, L., Wang, J., Wang, Z., and Liu, H. (2015a). Combustion and emissions of compression ignition in a direct injection diesel engine fueled with pentanol. Energy 80, 575–581. doi: 10.1016/j.energy.2014.12.013
Li, L., Wang, J., Wang, Z., and Xiao, J. (2015b). Combustion and emission characteristics of diesel engine fueled with diesel/biodiesel/pentanol fuel blends. Fuel 156, 211–218. doi: 10.1016/j.fuel.2015.04.048
Li, Q., Hu, E., Zhang, X., Cheng, Y., and Huang, Z. (2013c). Laminar flame speeds and flame instabilities of pentanol isomer-air mixtures at elevated temperatures and pressures. Energy Fuels 27, 1141–1150. doi: 10.1021/ef301901c
Liu, X., Wang, H., Zheng, Z., Liu, J., Reitz, R. D., and Yao, M. (2016). Development of a combined reduced primary reference fuel-alcohols (methanol /ethanol /propanols/butanols/n-pentanol) mechanism for engine applications. Energy 114, 542–558. doi: 10.1016/j.energy.2016.08.001
Liu, Y., Yuan, Z., Ma, Y., Fu, J., Huang, R., and Liu, J. (2019). Analysis of spray combustion characteristics of diesel, biodiesel and their n-pentanol blends based on a one-dimensional semi-phenomenological model. Appl. Energy 238, 996–1009. doi: 10.1016/j.apenergy.2019.01.176
Lucassen, A., Park, S., Hansen, N., and Sarathy, S. M. (2015). Combustion chemistry of alcohols: experimental and modeled structure of a premixed 2-methylbutanol flame. Proc. Combust. Inst. 35, 813–820. doi: 10.1016/j.proci.2014.05.008
Lucassen, A., Warkentin, J., Hansen, N., Park, S., and Sarathy, S. M. (2013). “Detailed analysis of iso-pentanol combustion chemistry in flames,” in 8th US National Combustion Meeting May 19-22-2013 paper #070LT-007 (Salt Lake city, UT).
Ma, Y., Huang, R., Fu, J., Huang, S., and Liu, J. (2018). Development of a diesel/biodiesel/alcohol(up to n-pentanol) combined mechanism based on reaction pathways analysis methodology. Appl. Energy 225, 835–847. doi: 10.1016/j.apenergy.2018.05.065
Ma, Y., Huang, R., Huang, S., Zhang, Y., Xu, S., and Wang, Z. (2017b). Experimental investigation on the effect of n-pentanol blending on spray, ignition and combustion characteristics of waste cooking oil biodiesel. Energy Convers. Manage. 148, 440–455. doi: 10.1016/j.enconman.2017.06.027
Ma, Y., Huang, S., Huang, R., Zhang, Y., and Xu, S. (2016). Spray and evaporation characteristics of n-pentanol-diesel blends in a constant volume chamber. Energy Convers. Manage. 130, 240–251. doi: 10.1016/j.enconman.2016.10.063
Ma, Y., Huang, S., Huang, R., Zhang, Y., and Xu, S. (2017a). Ignition and combustion characteristics of n-pentanol-diesel blends in a constant volume chamber. Appl. Energy 185, 519–530. doi: 10.1016/j.apenergy.2016.11.002
Mahalingam, A., Munuswamy, D. B., Devarajan, Y., and Radhakrishnan, S. (2018). Emission and performance analysis on the effect of exhaust gas recirculation in alcohol-biodiesel aspirated research diesel engine. Environ. Sci. Pollut. Res. Int. 25, 12641–12647. doi: 10.1007/s11356-018-1522-4
Manigandan, S., Gunasekar, P., Devipriya, J., and Nithya, S. (2019). Emission and injectgion characteristics of corn biodiesel blends in diesel engine. Fuel 235, 723–735. doi: 10.1016/j.fuel.2018.08.071
Mathan Raj, V., Subramanian, L. R. G., Thiyagarajan, S., and Geo, E. V. (2018). Effects of minor addition of aliphatic (1-pentanol) and aromatic (benzyl alcohol) alcohols in Simarouba Glauca-diesel blend fueled CI engine. Fuel 234, 934–943. doi: 10.1016/j.fuel.2018.07.122
McCormick, R. L., Fioroni, G., Fouts, L., Christensen, E., Yanowitz, J., Polikarpov, E., et al. (2017). Selection criteria and screening of potential biomass-derived streams as fuel blendstocks for advanced spark-ignition engines, SAE Int. J. Fuels. Lubr. 10. doi: 10.4271/2017-01-0868
Nanthagopal, K., Ashok, B., Saravanan, B., Korah, S. M., and Chandra, S. (2018a). Effect of next generation higher alcohols and Calophyllum inophyllum methyl ester blends in diesel engine. J. Clean. Prod. 180, 50–63. doi: 10.1016/j.jclepro.2018.01.167
Nanthagopal, K., Ashok, B., Saravanan, B., Patel, D., Sudarshan, B., and Ramasamy, R. A. (2018b). An assessment on the effects of 1-pentanol and 1-butanol as additives with Calophyllum Inophyllum biodiesel. Energy Convers. Manage. 158, 70–80. doi: 10.1016/j.enconman.2017.12.048
Nativel, D., Pelucchi, M., Frassoldati, A., Comandini, A., Cuoci, A., Ranzei, E., et al. (2016). Laminar flame speeds of pentanol isomers: an experimental and modeling study. Combust. Flame 166, 1–18. doi: 10.1016/j.combustflame.2015.11.012
No, S. Y. (2008). Prediction of maximum liquid-phase penetration in diesel spray-a review. ILASS-Korea J. 13, 117–125. Available online at: www.jchps.com
No, S. Y. (2019). Application of Liquid Biofuels to Internal Combustion Engines. Springer Nature. Singpore Pte Ltd. doi: 10.1007/978-981-13-6737-3
Nour, M., Attia, A. M. A., and Nada, S. A. (2019). Improvement of CI engine combustion and performance running on ternary blends of higher alcohol (pentanol and octanol)/hydrous ethanol/diesel. Fuel 251, 10–22. doi: 10.1016/j.fuel.2019.04.026
Pan, M., Huang, R., Liao, J., Jia, G., Zhou, X., Huang, H., et al. (2019). Experimental study of the spray, combustion, and emission performance of a diesel engine with high n-pentanol blending ratios. Energy Convers. Manage. 194, 1–10. doi: 10.1016/j.enconman.2019.04.054
Pan, M., Huang, R., Liao, J., Ouyang, T., Zheng, Z., Lv, D., et al. (2018). Effect of EGR dilution on combustion, performance and emission characteristics of a diesel engine fueled with n-pentanol and e-ethylhexyl nitrate additive. Energy Convers. Manage. 176, 246–255. doi: 10.1016/j.enconman.2018.09.035
Park, S., Chung, S. H., Lu, T., and Sarathy, S. M. (2015a). Combustion characteristics of C5 alcohols and a skeletal mechanism for homogeneous charge compression ignition combustion simulation. Energy Fuels 29,7584–7594. doi: 10.1021/acs.energyfuels.5b01392
Park, S., Manna, O., Khaled, F., Bougacha, R., Manour, M. S., Farooq, A., et al. (2015b). A comprehensive experimental and modeling study of 2-methylbutanol combustion. Combust. Flame 162, 2166–2176. doi: 10.1016/j.combustflame.2015.01.014
Peer, M. S., Kasimani, R., Rajamohan, S., and Ramakrishnan., P. (2017). Experimental evaluation on oxidation stability of biodiesel/diesel blends with alcohol addition by rancimat instrument and FTIR spectroscopy. J. Mech. Sci. Tech. 31, 455–463. doi: 10.1007/s12206-016-1248-5
Pelucchi, M., Bissoli, M., Rizzo, C., Zhang, Y., Somers, K. P., Frassoldati, A., et al. (2017). A kinetic modelling study of alcohols operating regimes in a HCCI engine. SAE Int. J. Eng. 10. doi: 10.4271/2017-24-0077
Prabhu, A., and Ramanan, M. V. (2018). Emission and performance analysis of pentanol-diesel blends in unmodified diesel engine. Int. J. Ambient Energy. 41, 699–702. doi: 10.1080/01430750.2018.1490356
Radhakrishnan, S., Devarajan, Y., Mahlingam, A., and Nagappan, B. (2017). Emissions analysis on diesel engine fueled with palm oil biodiesel and pentanol blends. J. Oil Palm Res. 29, 380–386. doi: 10.21894/jopr.2017.2903.11
Radheshyam, Santhosh, K., Kumar, G. N. (2020). Effect of 1-pentanol addition and EGR on the combustion performance and emission characteristics of a CRDI diesel engine. Renew. Energy 145, 925–936. doi: 10.1016/j.renene.2019.06.043
Rajak, U., and Verma, T. N. (2019). A comparative analysis of engine characteristics from various biodiesels: numerical study. Energy Convers. Manage. 180, 904–923. doi: 10.1016/j.enconman.2018.11.044
Rajasekar, V. (2016). Experimental investigations to study the effect of butanol and pentanol addition in a jatropha oil methyl ester fueled compression ignition engine. J. Chem. Pharm. Sci. 9, 665–668. Available online at: www.jchps.com
Rajesh Kumar, B., Muthukkumar, T., Krishnamoorthy, V., and Saravanan, S. (2016a). A comparative evaluation and optimization of performance and emission characteristics of a DI diesel engine fueled with n-propanol/diesel, n-butanol/diesel and n-pentanol/diesel blends using response surface methodology. RSC Adv. 6, 61869–61890. doi: 10.1039/C6RA11643D
Rajesh Kumar, B., and Saravanan, S. (2016b). Effects of iso-butanol/diesel and n-pentanol/diesel blends on performance and emission of a DI diesel engine under premixed LTC (low temperature combustion) mode. Fuel 170,49–59. doi: 10.1016/j.fuel.2015.12.029
Rajesh Kumar, B., Saravanan, S., Rana, D., and Nagendran, A. (2016b). Use of some advanced biofuels for overcoming smoke/NOx trade-off in a light-duty DI diesel engine. Renew. Energy 96, 687–699. doi: 10.1016/j.renene.2016.05.029
Rajesh Kumar, B., Saravanan, S., Rana, D., and Nagendran, A. (2016c). A comparative analysis on combustion and emissions of some next generation high-alcohol/diesel blends in a direct-injection diesel engine. Energy Convers. Manage. 119, 246–256. doi: 10.1016/j.enconman.2016.04.053
Rajesh Kumar, B., Saravanan, S., Rana, D., and Nagendran, A. (2016d). Combined effect of injection timing and exhaust gas recirculation (EGR) on performance and emissions of a DI diesel engine fueled with next-generation advanced biofuel-diesel blends using response surface methodology. Energy Convers. Manage. 123, 470–486. doi: 10.1016/j.enconman.2016.06.064
Rajesh Kumar, B. R., and Saravanan, S. (2015). Effect of exhaust gas recirculation (EGR) on performance and emissions of a constant speed DI diesel engine fueled with pentanol/diesel blends. Fuel 160, 217–226. doi: 10.1016/j.fuel.2015.07.089
Rajesh Kumar, B. R., and Saravanan, S. (2016a). Use of higher alcohol biofuels in diesel engines: a review. Renew. Sustain. Energy Rev. 60, 84–115. doi: 10.1016/j.rser.2016.01.085
Ramakrishnan, P., Kasimani, R., and Peer, M. S. (2018a). Optimization in the performance and emission parameters of a DI diesel engine fueled with pentanol added Calophyllum inophyllum/diesel blends using response surface methodology. Environ. Sci. Pollut. Res. 25, 29115–29128. doi: 10.1007/s11356-018-2867-4
Ramakrishnan, P., Kasimani, R., Peer, M. S., and Rajamohan, S. (2018b). Assessment of n-pentanol/Calophyllum inophyllum/diesel blends on the performance, emission, and combustion characteristics of a constant-speed variable compression ratio direct injection diesel engine. Environ. Sci. Pollut. Res. 25, 13731–13744. doi: 10.1007/s11356-018-1566-5
Ravikumar, J., and Saravanan, S. (2017). Performance and emission analysis on blends of diesel, restaurant yellow grease and n-pentanol in direct-injection diesel engine. Environ. Sci. Pollut. Res. 24, 5381–5390. doi: 10.1007/s11356-016-8298-1
Raza, M., Chen, L., Ruiz, R., and Chu, H. (2019). Influence of pentanol and dimethyl ether blending with diesel on the combustion performance and emission characteristics in a compression ignition engine under low temperature combustion mode. J. Energy Inst. 92, 1658–1669. doi: 10.1016/j.joei.2019.01.008
Sarathy, S. M. (2016). “Fuel class higher alcohols chap.2,” in Biofuels from Lignocellulosic Biomass: Innovations beyond Bioethanol, First, ed M.Boot (Wiley-VCH Verlag GmbH & CO. KGaA), 29–57. doi: 10.1002/9783527685318.ch2
Sarathy, S. M., Obwald, P., Hansen, N., and Kohse-Hoinghaus, K. (2014). Alcohol combustion chemistry. Prog. Energy Combust. Sci. 44, 40–102. doi: 10.1016/j.pecs.2014.04.003
Sarathy, S. M., Park, S. W., Weber, B. W., Wang, W., Veloo, P. S., Davis, A. C., et al. (2013). A comprehensive experimental and modeling study of iso-pentanol combustion. Combust. Flame 160, 2712–2728. doi: 10.1016/j.combustflame.2013.06.022
Sarathy, S. M., Vranckx, S., Yasunaga, K., Mehl, M., OBwald, P., Metcalfe, W. K., et al. (2012). A comprehensive chemical kinetic combustion model for the four butanol iosmers. Combust. Flame 159, 2028–2055. doi: 10.1016/j.combustflame.2011.12.017
Serinyel, Z., Togbe, C., Dayma, G., and Dagaut, P. (2014). An experimental and modeling study of 2-methyl-1-butanol oxidation in a jet-stirred reactor. Combust. Flame 161, 3003–3013. doi: 10.1016/j.combustflame.2014.06.004
Shahir, S. A., Masjuki, H. H., Kalam, M. A., Imran, A., and Ashraful, A. M. (2015). Performance and emission assessment of diesel-biodiesel-ethanol/bioethanol blend as a fuel in diesel engines: a review. Renew. Sustain. Energy Rev. 48, 62–78. doi: 10.1016/j.rser.2015.03.049
Sivalakshmi, S., and Balusamy, T. (2011). Performance and emission characteristics of a diesel engine fueled by neem oil blended with alcohols. Int. J. Ambient Energy 32, 170–178. doi: 10.1080/01430750.2011.625717
Sridhar, R., Jeevahan, J., and Chandrasekaran, M. (2018). Effect of the addition of 1-pentanol on engine performance and emission characteristics of diesel and biodiesel fueled single cylinder diesel engine. Int. J. Ambient Energy. 41, 58–63. doi: 10.1080/01430750.2018.1443505
Subramanian, T., Varuvel, E. G., Martin, L. J., and Beddhannan, N. (2017). Effect of lower and higher alcohol fuel synergies in biofuel blends and exhaust treatment system on emission from CI engine. Environ. Sci. Pollut. Res. 24, 25103–25113. doi: 10.1007/s11356-017-0214-9
Tang, C., Wei, L., Man, X., Zhang, J., Huang, Z., and Law, C. K. (2013). High temperature ignition delay times of C5 primary alcohols. Combust. Flame 160, 520–529. doi: 10.1016/j.combustflame.2012.11.018
Togbe, C., Halter, F., Foucher, F., Mounaim-Rousselle, C., and Dagaut, P. (2011). Experimental and detailed kinetic modeling study of 1-pentanol oxidation in a JSR and combustion in a bomb. Proc. Combust. Inst. 33, 367–374. doi: 10.1016/j.proci.2010.05.003
Tsujimura, T., Pitz, W. J., Yang, Y., and Dec, J. E. (2011). Detailed kinetic modeling of HCCI combustion with isopentanol. SAE Int. J. Fuels. Lubr. 4. doi: 10.4271/2011-24-0023
Verma, P., Stevanovic, S., Zare, A., Dwivedi, G., Van, T. C., Davidson, M., et al. (2019). An overview of the influence of biodiesel, alcohols, and various oxygenated additives on the particulate matter emissions from diesel engines. Energies 12:1987. doi: 10.3390/en12101987
Wang, G., Yuan, W., Li, Y., Zhao, L., and Qi, F. (2015). Experimental and kinetic modeling study of n-pentanol pyrolysis and combustion. Combust. Flame 162, 3277–3287. doi: 10.1016/j.combustflame.2015.05.017
Wei, L., Cheung, C. S., and Huang, Z. (2013). Experimental study on performance and emissions of diesel/n-pentanol blends on a DI diesel engine,” in 9th Asia-Pacific Conference on Combustion (Gyeongju: Gyeongju Hilton).
Wei, L., Cheung, C. S., and Huang, Z. (2014). Effect of n-pentanol addition on the combustion, performance and emission characteristics of a direct-injection diesel engine. Energy 70,172–180. doi: 10.1016/j.energy.2014.03.106
Yang, K., Wei, L., Cheung, C. S., Tang, C., and Huang, Z. (2017). The effect of pentanol addition on the particulate emission characteristics of a biodiesel operated diesel engine. Fuel 209, 132–140. doi: 10.1016/j.fuel.2017.07.093
Yang, Y., Dec, J., Dronniou, N., and Simmons, B. (2010). Characteristics of isopentanol as a fuel for HCCI engines. SAE Int. J. Fuels. Lubr. 3, 725–741.
Yasin, M. H. N., Mamat, R., Yusop, A. F., Aziz, A., and Najafi, G. (2015). Comparative study on biodiesel methanol diesel low proportion blends operating with a diesel engine. Energy Procedia. 75, 10–16. doi: 10.1016/j.egypro.2015.07.128
Yesilyurt, M. K., Eryilmaz, T., and Arslan, M. (2018). A comparative analysis of the engine performance, exhaust emissions and combustion behaviors of a compression ignition engine fueled with biodiesel/diesel/1-butanol (C4 alcohol) and biodiesel/diesel/n-pentanol (C5 alcohol) fuel blends. Energy 165, 1332–1351. doi: 10.1016/j.energy.2018.10.100
Yilmaz, N., and Atmanli, A. (2017a). Experimental assessment of a diesel engine fueled with diesel-biodiesel-1-pentanol blends. Fuel 191, 190–197. doi: 10.1016/j.fuel.2016.11.065
Yilmaz, N., and Atmanli, A. (2017b). Experimental evaluation of a diesel engine running on the blends of diesel and pentanol as a next generation higher alcohol. Fuel 210, 75–82. doi: 10.1016/j.fuel.2017.08.051
Yilmaz, N., Atmanli, A., and Trujillo, M. (2017). Influence of 1-pentanol additive on the performance of a diesel engine fueled with waste oil methyl ester and diesel fuel. Fuel 207, 461–469. doi: 10.1016/j.fuel.2017.06.093
Yilmaz, N., Atmanli, A., and Vigil, F. M. (2018). Quaternary blends of diesel, biodiesel, higher alcohols and vegetable oil in a compression ignition engine. Fuel 212, 462–469. doi: 10.1016/j.fuel.2017.10.050
Yilmaz, N., Ileri, E., and Atmanli, A. (2016). Performance of biodiesel/higher alcohols blends in a diesel engine. Int. J. Energy Res.40, 1134–1143. doi: 10.1002/er.3513
Yoshimoto, Y., Tserenochir, E., Kinoshita, E., and Otaka, T. (2018). Combustion improvements by c4/c5 bio-alcohol isomer blended fuels combined with supercharging and EGR in a diesel engine. Int. J. Mech. Mechatro. Eng. 12, 961–969. doi: 10.1299/jsmemecj.2018.J0710401
Yusri, I. M., Mamat, R., Najafi, G., Razman, A., Awad, O. I., and Azmi, W. H., et al. (2017). Alcohol based automotive fuels from first four alcohol family in compression and spark ignition engine: a review on engine performance and exhaust emissions. Renew. Sustain. Energy Rev. 77, 169–181. doi: 10.1016/j.rser.2017.03.080
Zaharin, M. S. M., Abdullah, N. R., Najafi, G., Sharudin, H., and Yusaf, T. (2017). Effects of physicochemical properties of biodiesel fuel blends with alcohol on diesel engine performance and exhaust emissions: a review. Renew. Sustain. Energy Rev. 79, 475–493. doi: 10.1016/j.rser.2017.05.035
Zhang, Q., Hu, X., Li, Z., and Liu, B. (2018). Combustion and emission characteristics of diesel engines using diesel, dmf/diesel, and n-pentanol/diesel fuel blends. J. Energy Eng. 144:04018030. doi: 10.1061/(ASCE)EY.1943-7897.0000549
Zhang, Z.-H., and Balasubramanian, R. (2016). Investigation of particulate emission characteristics of a diesel engine fueled with higher alcohols/biodiesel blends. Appl Energy 16, 71–80. doi: 10.1016/j.apenergy.2015.10.173
Zhang, Z.-H., Chua, S.-M., and Balasubramanian, R. (2016). Comparative evaluation of the effect of butanol-diesel and pentanol-diesel blends on carbonaceous particulate composition and particle number emissions from a diesel engine. Fuel 176, 40–47. doi: 10.1016/j.fuel.2016.02.061
Keywords: pentanol, diesel engine, kinetic mechanism, binary, ternary and quaternary blends, combustion, exhaust emission
Citation: No S-Y (2020) Utilization of Pentanol as Biofuels in Compression Ignition Engines. Front. Mech. Eng. 6:15. doi: 10.3389/fmech.2020.00015
Received: 16 October 2019; Accepted: 06 March 2020;
Published: 22 April 2020.
Edited by:
Alpaslan Atmanli, National Defense University, TurkeyReviewed by:
Shiyou Yang, Ford Motor Company, United StatesIM Rizwanul Fattah, University of Technology Sydney, Australia
Dipak Rana, University of Ottawa, Canada
Copyright © 2020 No. This is an open-access article distributed under the terms of the Creative Commons Attribution License (CC BY). The use, distribution or reproduction in other forums is permitted, provided the original author(s) and the copyright owner(s) are credited and that the original publication in this journal is cited, in accordance with accepted academic practice. No use, distribution or reproduction is permitted which does not comply with these terms.
*Correspondence: Soo-Young No, sooyoung@cbnu.ac.kr