- 1School of Mechanics, Civil Engineering and Architecture, Northwestern Polytechnical University, Xi’an, China
- 2CSSC Systems Engineering Research Institute, Beijing, China
- 3School of Architecture and Civil Engineering, Xiamen University, Xiamen, China
- 4QianYuan National Laboratory, Hangzhou, China
This paper examines multiscale theories and numerical methods for interconnect materials in electronic packaging, focusing on the interplay among micro-scale morphology, meso-scale structure, and macro-scale behavior to improve material reliability and performance prediction. It reviews advanced materials, such as sintered silver and lead-free solder, alongside methodologies like Molecular Dynamics (MD) simulations, cohesive modeling, crystal plasticity modeling, and phase-field modeling, to evaluate mechanical and thermal properties across scales and their long-term reliability. At the microscopic scale, MD simulations reveal the influence of atomic arrangements, grain orientations, and dislocation evolution on mechanical behavior. At the mesoscopic scale, phase-field and crystal plasticity models are combined to analyze pore evolution, grain sliding, and stress concentration under thermal cycling. Macroscopically, models like Anand and Unified Creep Plasticity (UCP) describe viscoplasticity, creep, and fatigue life, offering insights into performance under complex conditions. By systematically integrating diverse research methods and theoretical models, this review highlights the applicability of a multiscale coupling framework, providing a comprehensive understanding of the correlations between morphology, structure, and behavior. This framework serves as theoretical guidance for developing innovative packaging solutions and optimizing materials for high-density, low-power electronic devices.
1 Introduction
The rapid advancement of electronics necessitates miniaturization and high-density integration in packaging. Technologies like Wafer-Level Packaging (WLP) (Elshabini et al., 2006), System-in-Package (SiP) (Fontanelli, 2008; Dai, 2016; Wang et al., 2023), and heterogeneous integration (Lee et al., 2020; Lau, 2022) address these needs. Notably, 3D heterogeneous integration bridges chip-level and system-level integration (Choudhury, 2010; Zhang et al., 2022). However, complex materials and micro-scale morphology demand multi-scale investigation into performance and reliability.
As devices shrink, mechanical properties like conductivity and thermal expansion become critical. Traditional materials fall short of modern packaging demands. Research focuses on nanomaterials, sintered silver and copper, and lead-free solder to meet high-density packaging requirements (Paknejad and Mannan, 2017; Zhang et al., 2019; Aamir et al., 2020). Nanoindentation evaluates stress-strain relationships (Long et al., 2021b). While sintered silver offers reliability, it’s costly; thus, sintered copper is explored as a cost-effective alternative (Chen and Siow, 2021). Models study solder deformation and failure over time. Finite Element Analysis (FEA) efficiently assesses performance (Mathew et al., 2021; Gharaibeh and Wilde, 2023). Life prediction models like Coffin-Manson and Basquin are widely used (Tan et al., 2014; Chen et al., 2020; Coffin, 2022; Mathew et al., 2022). These models predict lifespan under cyclic loading, evaluating long-term reliability. Therefore, solder fatigue behavior and failure mechanisms are crucial in macroscopic modeling.
However, macroscopic models cannot fully describe solder properties due to micro- and meso-scale influences. Behaviors like void formation and grain orientation changes affect reliability. Simulations focusing on these scales are key (Lederer et al., 2021; Long et al., 2022), providing insights into porosity effects and guiding design improvements (Ma et al., 2023; Long et al., 2023c). Advances in Molecular Dynamics (MD) and Crystal Plasticity Finite Element Method (CPFEM) are pivotal for understanding these correlations.
This paper explores cross-scale theoretical research on packaging materials, focusing on relationships between micro-scale morphology, meso-scale structure, and macro-scale behavior. It analyzes mechanical behavior and reliability using integrated simulations. MD and Monte Carlo simulations reveal atomic-level details; meso-scale analysis employs crystal plasticity and phase-field methods; macroscopic models like Anand and UCP describe viscoplasticity and creep. Long-term performance evaluation uses SEM and FEM to support life prediction. The proposed multiscale framework spans microscopic to macroscopic scales and transient to long-term behavior, providing a foundation for material development and optimization. It guides simulation and verification of micro-scale morphology and advanced designs, offering new insights and practical guidance for material optimization in electronic packaging.
2 Electronics interconnection materials and its morphologies
In electronic packaging, micro-scale morphology of solders significantly influences performance and stability. SnPb solder and Sn-Ag-Cu (SAC) lead-free solder exhibit unique characteristics. Siviour et al. (2005) observed tin-rich regions appearing dark and lead-rich regions light in SnPb solder, indicating compositional differences. Figure 1A presents a cross-sectional SEM image showing the overall morphology of SnPb solder on copper. Tu and Zheng (2001) noted that a continuous Cu₆Sn₅ layer forms at the SnPb solder interface, thickening over time and developing into columnar grains (Figures 1B, C). Zhang et al. (2007) found that on a copper substrate, the Cu₆Sn₅ layer has a scallop-like shape, while the Cu₃Sn layer is flat. As soldering continues, the intermetallic compound (IMC) layer thickens and forms a complex multilayer structure.
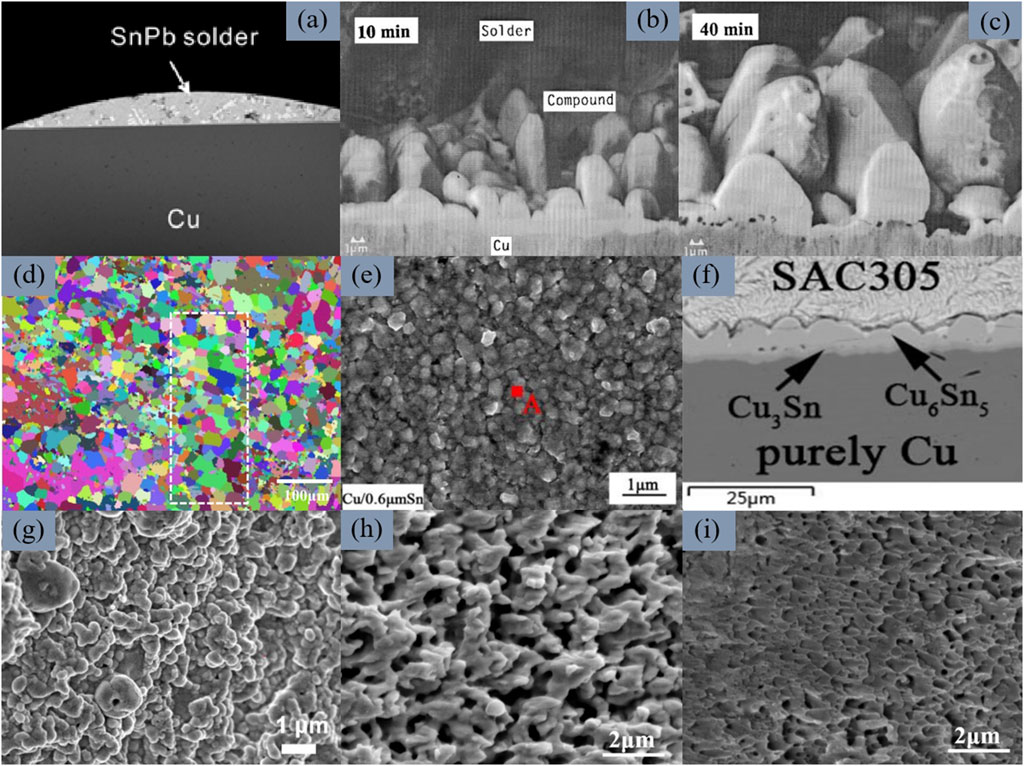
Figure 1. Microstructural evolution of different solders. (A) Cross-sectional SEM image showing the overall morphology of SnPb solder on copper. (B, C) Formation of the Cu₆Sn₅ layer at the SnPb solder interface, which thickens over time and eventually develops into a columnar grain structure. (D) Low-magnification EBSD image of as-cast SAC305 alloy, displaying equiaxed Sn grains with diameters of 20–30 μm and varied orientations. (E) micro-scale morphology of Cu₃Sn grains after 300 min of soldering at 260°C, showing fine equiaxed grains. (F) SEM morphology of the SAC305/Cu interface after aging at 150°C for 360 h (G) SEM micro-scale morphology of sintered silver nanoparticles. (H, I) SEM micro-scale morphology of the fracture surface of the sintered nanosilver layer at 0°C and 75°C, respectively. [Reprinted from Ref (Tu and Zeng, 2001; Zhang et al., 2007; 2018; Gain and Zhang, 2019; Yao et al., 2020; Long et al., 2021b; Ma et al., 2023). with permission from Elsevier].
For SAC lead-free solder, Aamir et al. (2020) observed homogeneously distributed Ag₃Sn and Cu₆Sn₅ IMCs within the β-Sn matrix; adding trace elements like Ti and Fe refines grains and enhances structure. Aamir et al. (2017) noted that thermal aging causes IMC coarsening and structural inhomogeneity, but La doping mitigates these effects. Gain and Zhang (2019) found that SAC305 solder contains uniformly distributed Ag₃Sn and Cu₆Sn₅ IMC particles with Sn matrix grains measuring 20–30 μm. Figure 1D presents the EBSD image of as-cast SAC305 alloy, showing that high temperature and humidity cause significant growth of IMCs and matrix grains, indicating strong environmental influence on micro-scale morphology. Figure 1E shows the micro-scale morphology of Cu₃Sn grains after 300 min of soldering at 260°C, exhibiting fine equiaxed grains. Zhang et al. (2018) indicated that at the interface between Sn3.0Ag0.5Cu solder and Ni-containing Cu substrate, the IMC layer exhibits scallop-shaped Cu₆Sn₅ and planar Cu₃Sn structures. Figure 1F shows SEM morphology of the SAC305/Cu interface after aging at 150°C for 360 h. With Ni content over 5%, a prismatic (Cu,Ni)₆Sn₅ phase forms; IMC grains coarsen further with prolonged aging. Zhao et al. (2019) found that Sn-Cu lead-free solder consists mainly of a β-Sn matrix and Cu₆Sn₅ IMCs; increasing Cu content leads to more abundant and homogeneously distributed Cu₆Sn₅ particles, influencing micro-scale morphology. Yao et al. (2020) demonstrated that Cu₃Sn solder morphology evolves from fine equiaxed grains to coarse columnar grains, forming a mixed structure.
The micro-scale morphology of sintered silver solder has been extensively studied. Long et al. (2021b) demonstrated that adding SiC microparticles enhances density and thermal stability of sintered silver, significantly reducing porosity (Figure 1G illustrates SEM morphology after sintering). K et al. (2023) reported that sintered silver solder composed of 50 nm nanoparticles and 5 μm microparticles exhibits intensified neck growth under high temperatures and shear stress, leading to structural coarsening and reduced porosity (Figures 1H, I). Ma et al. (2017) optimized solder morphology via heterogeneous nucleation, using IMCs as nucleating agents to guide tin crystal growth, mitigating performance degradation and improving fatigue and electromigration resistance.
In summary, solder micro-scale morphology is influenced by material composition, processing techniques, and environmental conditions, directly determining macroscopic performance. Understanding microstructural evolution is crucial for improving mechanical stability and reliability of solder joints, reflecting the close relationship between micro-scale morphology and macroscopic performance.
3 Multi-scale mechanics and their numerical approaches
3.1 Microscopic mechanics models based on MD
Molecular Dynamics (MD) is essential for understanding how tiny structures influence material properties. MD simulations use techniques like the Embedded Atom Method (EAM) to track atom movements by solving basic physics equations. This helps reveal how small-scale features affect how materials perform. In electronic packaging, MD simulations examine how metal grains interact, how grain boundaries change, and how these factors impact strength and heat resistance. Key materials studied include nanoscale silver, nanoscale copper, SAC305 solder, and intermetallic compounds (IMCs). This knowledge supports improving material performance and enhancing packaging processes.
Haris et al. (2021) reviewed the fundamentals of MD simulations, covering their basic principles, methods, and applications. They used MD simulations to uncover atomic-level details of how molecules interact with metal surfaces. These interactions are difficult to observe using other techniques. Luo et al. (2024) employed MD simulations to investigate sintering processes and mechanical property evolution of copper nanomaterials at 500–650 K. They analyzed microstructural changes of nanospheres and nanofilms under varying sintering conditions, establishing quantitative relationships with shear and tensile properties. These transformations significantly influenced mechanical strength and stability, providing theoretical support for developing high-reliability copper-based packaging materials. Alarifi et al. (2013) utilized MD simulations to analyze sintering and surface premelting behavior of silver nanoparticles, revealing how atomic-scale processes like neck formation and grain boundary evolution affect densification and shrinkage. Gu et al. (2022) investigated atomic motion of silver nanoparticles during sintering, elucidating wetting behavior of smaller particles on larger ones, correlating this behavior with structural rigidity and bonding strength.
Hu et al. (2023) explored sintering mechanisms of silver nanoparticles at different temperatures and their impact on mechanical properties. The study revealed roles of neck growth and dislocation evolution in determining material strength and plastic deformation, providing theoretical support for optimizing silver-based packaging materials. Motalab et al. (2019) investigated influence of micro-scale morphology of SAC solder at nanoscale on material properties using MD simulations. They found that formation of IMCs like Ag₃Sn enhances strength and modulus while reducing ductility and thermal conductivity. Ye et al. (2024) employed MD simulations to investigate neck evolution mechanisms of silver nanoparticles on SiC/Cu substrates during sintering, revealing how microscopic changes in neck width influence mechanical properties of the sintered interface. Zhang and Liu (2024) analyzed effects of sintering pressure and temperature on mechanical properties of silver nanomaterials under uniaxial tension, revealing how microscopic energy changes, density, and dislocation evolution regulate yield strength and deformation behavior, providing guidance for optimizing sintering processes.
Although MD simulations have advanced our understanding of microstructures and properties in materials like copper, silver, and SAC solder, most studies focus on specific materials, limiting their broad applicability. Additionally, simulations are not well integrated with experiments, making it hard to predict real-world behavior. High computational demands also restrict large-scale use. In the future, research should explore more materials, improve experimental validation, and optimize multiscale simulation methods to enhance the reliability and efficiency of electronic packaging material design.
3.2 Meso-scale models on crystal plasticity and fracturing
Mesoscopic models—including crystal plasticity, cohesive, and phase-field models—capture aspects of the relationship between meso-scale structure and macroscopic performance. Crystal plasticity focuses on crystallographic orientation and slip mechanisms, while phase-field models reveal the influence of phase transformations and crack propagation through meso-scale evolution.
Xie et al. (2021) applied the Crystal Plasticity Finite Element Method (CPFEM) to simulate mesoscopic deformation mechanisms like slip and dislocation motion in SAC305 solder, linking microstructural features like β-Sn dendritic and eutectic regions with macroscopic mechanical response under thermal aging (Xie et al., 2022). Jiang et al. (2024) used phase-field models to simulate the meso-scale structural changes in sintered silver during thermal aging. They incorporated structures with varying porosity into finite element models to show how pore evolution impacts thermal and mechanical properties. This work provides a multiscale framework for evaluating reliability.
Long et al. (2023a) incorporated crystal plasticity models to account for grain orientation and pore distribution on mesoscopic deformation behavior, revealing how pore structures and grain orientation under thermal cycling induce non-uniform stresses and crack propagation. Su et al. (2021b), Su et al. (2023) developed advanced phase-field models that incorporate micro-scale morphology and account for thermo-elasto-plastic coupling. They explained how porosity affects the growth of large-scale cracks. Their models provide reliable predictions of thermal fatigue behavior in sintered silver and other porous materials.
Xie and Chen (2022) integrated thermal activation mechanisms into their crystal plasticity model to investigate cyclic softening and creep behavior under thermomechanical coupling, showing how grain orientation and temperature affect macroscopic properties. Zhu et al. (2021) employed phase-field models to examine fracture behavior of sintered silver nanoparticles with varying porosities, emphasizing the significant impact of mesoscale structure on properties like elastic modulus and ultimate strength. Long et al. (2023b) used a damage-based CPFEM to simulate plastic deformation and damage evolution in nickel-based polycrystalline alloys under low-cycle fatigue, demonstrating how mesoscopic features influence macroscopic mechanical behavior, effectively bridging the gap between mesoscopic and macroscopic responses.
Although crystal plasticity and phase-field models have advanced the simulation of mesoscale behaviors in materials like SAC305 solder and sintered silver, issues remain. These models are computationally complex, limiting large-scale simulations, and often focus on specific materials and conditions, lacking generality. Parameter calibration heavily relies on experimental data, increasing implementation difficulty. Additionally, some studies do not adequately integrate multiscale methods, affecting predictions of actual performance. In the future, computational efficiency should be optimized, the range of applicable materials expanded, and integration with experimental data strengthened to enhance model practicality and accuracy.
3.3 Macroscopic constitutive models and simulation methods
3.3.1 Macroscopic constitutive models
Macroscopic constitutive models are crucial for understanding the mechanical reliability of chip support materials, serving as a cornerstone in multiscale analysis by describing material responses to applied loads and connecting micro- or meso-scale mechanisms to macroscopic performance. They capture elastic, plastic, and creep behaviors, enabling predictions under various conditions.
Significant progress has been made in viscoplastic constitutive models for solder materials. Anand (1982) introduced strain rate and temperature-dependent constitutive equations, initially for high-strength aluminum, later applied to SnPb and lead-free solders. Building on Anand’s model, Motalab et al. (2014) derived creep responses for SAC305 solder joints, with fitted parameters aligning with experimental trends. Pei and Qu (2005) refined the linear relationship between Anand model parameters and temperature for lead-free solders, improving data fitting accuracy. Long et al. (2017), Long, et al. (2020) used a modified UCP model for SAC305 deformation at low to moderate strain rates and the Johnson-Cook model for high strain rate impacts. These studies highlight the importance of macroscopic constitutive models in enhancing performance predictions.
In materials science, these models are essential in multiscale analysis frameworks. The Anand model, applied by Calabretta et al. (2021), accurately simulates temperature and strain rate effects on mechanical behavior, especially viscoplasticity. However, it heavily relies on experimental data; recharacterization is necessary for new materials or scenarios. Chen et al. (2014) integrated the Ohno-Wang (OW) model with the Anand model in ABAQUS to simulate shear and creep behavior of sintered nanosilver joints, with experimental validation showing the OW model outperforming Anand’s under high-temperature conditions. Gharaibeh and Wilde, 2023 evaluated several parameter sets for the Anand model. They examined how different creep constitutive models affect simulations and highlighted the importance of multiscale analysis in selecting a model. Lederer et al. (2021) developed a constitutive equation encompassing plasticity, creep behavior, and porosity impact on sintered silver joints, implemented in ABAQUS using UMAT and VUMAT subroutines. Long et al. (2023d) stressed the importance of nanoindentation techniques in investigating elastoplastic properties of sintered silver joints, confirming that combining nanoindentation and numerical methods offers comprehensive nanoscale understanding, aiding design and performance optimization (Long et al., 2024).
Although macroscopic constitutive models have improved our understanding of solder materials’ mechanical reliability, they have some limitations. They rely heavily on experimental data, requiring recharacterization for new materials, which is time-consuming. Combining multiple models increases complexity and may reduce accuracy. Many studies do not validate models in different scenarios, limiting their general use. Future research should make models more flexible, simplify integration, and increase validation efforts to enhance their reliability and usefulness in multiscale analyses.
3.3.2 Long-term performance analysis
Long-term performance analysis is vital for predicting material reliability under specific operating conditions, particularly in understanding fatigue and creep behaviors. High temperatures and cyclic loading introduce complex failure mechanisms across micro-, meso-, and macro-scales. Structural characteristics like grain structure, defects, and crack propagation at micro- and meso-scales play pivotal roles.
Chen et al. (2020) demonstrated that sintered silver paste, while exhibiting excellent fatigue resistance at room temperature, deteriorates significantly at 200°C due to increased plastic deformation and accelerated crack propagation (Figure 2A shows SEM image of surface cracks after fatigue testing at 200°C). Zhang et al. (2024) found that meso-scale defects in sintered silver nanoparticles affect macroscopic crack propagation by inducing stress concentration and dislocation emission. Rectangular defects delay failure and enhance toughness by dispersing stress, whereas arc-shaped and triangular defects accelerate crack propagation, leading to faster failure (Figure 2B illustrates the evolution of fatigue cracks in sintered silver chip interconnections simulated by the phase-field method).
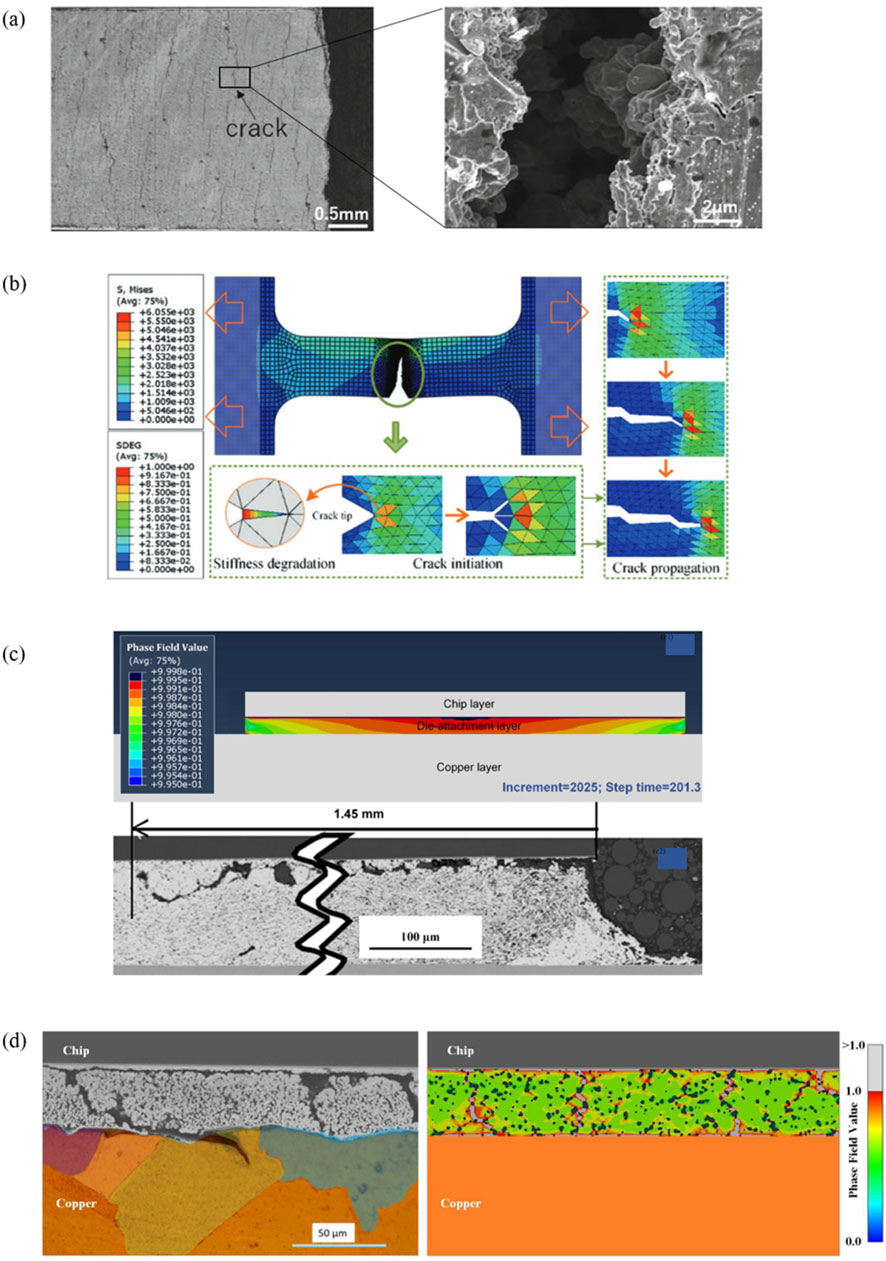
Figure 2. Fatigue Crack Evolution and Macroscopic Crack Growth in Sintered Silver: Phase-Field Simulation and Experimental Analysis. (A) SEM image of surface cracks in a sintered silver specimen after fatigue testing at 200°C. (B) Fatigue crack evolution in sintered silver die-attachment based on phase-field simulation. (C) Macroscopic crack growth in sintered AgNPs specimens. (D) Crack propagation in sintered nano-silver under thermo-elasto-plastic phase-field modeling: comparison between experimental results and numerical simulation. [Reprinted from Ref (Chen et al., 2020; Su et al., 2021b; Su et al., 2021a; Zhang et al., 2024). with permission from Elsevier].
Su et al. (2021a) used finite element and phase-field simulations to investigate the impact of power cycling on sintered silver bumps, revealing that cracks initiate from chip corners and propagate toward the center (Figure 2C). Thermal performance decline becomes more pronounced during long-term degradation (Chen et al., 2022). For SAC305 solder, a novel fatigue damage model based on entropy increase showed a strong correlation between damage parameter evolution and strain rate, providing theoretical support for predicting long-term performance of electronic packaging structures (Long et al., 2023f).
At the macro-scale, cumulative effects of micro- and meso-scale mechanisms manifest as global material failure, such as changes in crack propagation and fracture modes. Multiscale analysis effectively links the evolution of micro-scale morphology and meso-scale structures to macroscopic mechanical performance, providing a reliable foundation for describing material failure under high-temperature conditions. This approach is valuable in electronic packaging and high-temperature applications, offering robust support for design optimization and long-term performance prediction (Long et al., 2021a). Su et al. (2022) further verified the importance of multiscale analysis in failure prediction by analyzing the impact of thermal cycling loads, power density, and switching frequency on crack propagation rate and morphology using a fracture phase-field model.
A study using a thermo-elasto-plastic phase-field model investigated how randomly distributed micro-scale morphology influences the long-term performance of sintered nano-silver materials, focusing on the role of meso-scale pore evolution in crack propagation and network formation (Agyakwa et al., 2020; Su et al., 2021b). Additionally, a new Unified Creep Plasticity (UCP) constitutive model reveals the accumulation and evolution of fatigue damage in viscoplastic materials under varying temperatures and strain rates, offering theoretical insights for material design optimization and reliability enhancement (Long et al., 2023e). Figure 2D shows crack propagation in sintered nano-silver under thermo-elasto-plastic phase-field modeling, comparing experimental results and numerical simulations.
While multiscale analysis effectively connects micro- and meso-scale phenomena to macroscopic performance, it has limitations. The models are often computationally intensive, making large-scale simulations challenging. Additionally, many studies focus on specific materials like sintered silver and SAC305, reducing their general applicability. Experimental validation is sometimes insufficient, which can undermine the reliability of predictions. Future research should aim to streamline models, expand the range of studied materials, and enhance experimental correlations to improve the accuracy and applicability of long-term performance predictions in electronic packaging.
4 Next steps and perspectives
As electronic packaging interconnect materials evolve toward miniaturization, high integration, and multifunctionality, multiscale approaches have become increasingly important. Multiscale modeling will play a crucial role in optimizing design and assessing reliability of packaging materials. By integrating methods like Crystal Plasticity Finite Element Analysis, phase-field modeling, and MD simulations, researchers can more accurately uncover mechanisms driving material evolution. Structural features like grain size, pore distribution, and interfacial properties can be incorporated into macroscopic models, enhancing performance prediction accuracy. These approaches are expected to find broad applications in advanced solder materials, nanomaterials, and heterogeneous packaging systems to meet complex demands of modern packaging.
Advancements in multiscale methods will facilitate a shift in material design from experience-based to theory-driven approaches. Implementing Artificial Intelligence (AI) and Machine Learning (ML) can accelerate extraction and validation of parameters across scales, simplifying simulations and enhancing computational efficiency. This transformation shifts from traditional experimental approaches to systematic, computer-aided performance optimization, improving scientific rigor and efficiency. However, integrating experimental validation with multiscale modeling remains a critical research focus. Experimental data will continue to calibrate and validate models, improving accuracy and reliability, providing deeper insights into how micro-scale and meso-scale mechanisms influence macroscopic performance. In summary, multiscale analysis will continue to play a pivotal role in packaging material research, driving development of high-performance materials and offering theoretical support for high-density, highly reliable electronic products.
5 Conclusion
This review systematically examines advancements in cross-scale modeling of interconnect materials used in electronic packaging, analyzing intrinsic correlations between micro-scale and meso-scale structural features and macroscopic mechanical properties. Conventional macroscopic constitutive models have limitations in capturing mechanical behavior of complex packaging materials, making it challenging to reflect influence of structural evolution on overall performance and failure mechanisms. Through multiscale simulation techniques like MD, CPFEM, and phase-field models, researchers can precisely reveal mechanical responses across scales and conduct in-depth analyses of failure mechanisms in solder and sintered material systems. This paper summarizes strengths of these approaches in describing evolution of defects, grain orientation changes, and pore distribution, exploring potential applications under complex stress conditions.
Cross-scale modeling connects micro- and meso-scale mechanisms with macroscopic performance, enhancing material reliability and design accuracy, especially in long-term performance analysis. This review highlights the profound impact of micro-scale morphology and meso-scale structure on mechanical properties, providing theoretical support for optimizing electronic packaging materials design.
Author contributions
ZeZ: Investigation, Methodology, Visualization, Writing–original draft. FL: Methodology, Writing–review and editing. VF: Methodology, Writing–review and editing. ZoZ: Conceptualization, Supervision, Writing–original draft, Writing–review and editing. SL: Funding acquisition, Methodology, Supervision, Writing–review and editing. RD: Methodology, Writing–review and editing.
Funding
The author(s) declare that financial support was received for the research, authorship, and/or publication of this article. This work was supported by the National Natural Science Foundation of China (Nos. 52475166, 52175148), the Regional Collaboration Project of Shanxi Province (No. 2022104041101122), the Qin Chuang Yuan high-level innovation and entrepreneurship talent project (No. QCYRCXM-2022-306), and the Natural Science Foundation of Chongqing (No. CSTB2022NSCQ-MSX0574).
Conflict of interest
The authors declare that the research was conducted in the absence of any commercial or financial relationships that could be construed as a potential conflict of interest.
Generative AI statement
The author(s) declare that no Generative AI was used in the creation of this manuscript.
Publisher’s note
All claims expressed in this article are solely those of the authors and do not necessarily represent those of their affiliated organizations, or those of the publisher, the editors and the reviewers. Any product that may be evaluated in this article, or claim that may be made by its manufacturer, is not guaranteed or endorsed by the publisher.
References
Aamir, M., Muhammad, R., Ahmed, N., and Waqas, M. (2017). Impact of thermal aging on the intermetallic compound particle size and mechanical properties of lead free solder for green electronics. Microelectron. Reliab. 78, 311–318. doi:10.1016/j.microrel.2017.09.022
Aamir, M., Muhammad, R., Tolouei-Rad, M., Giasin, K., and Silberschmidt, V. V. (2020). A review: microstructure and properties of tin-silver-copper lead-free solder series for the applications of electronics. Solder. and Surf. Mt. Technol. 32, 115–126. doi:10.1108/SSMT-11-2018-0046
Agyakwa, P., Dai, J., Li, J., Mouawad, B., Yang, L., Corfield, M., et al. (2020). Three-dimensional damage morphologies of thermomechanically deformed sintered nanosilver die attachments for power electronics modules. J. Microsc. 277, 140–153. doi:10.1111/jmi.12803
Alarifi, H. A., Atis, M., Özdoğan, C., Hu, A., Yavuz, M., and Zhou, Y. (2013). Molecular dynamics simulation of sintering and surface premelting of silver nanoparticles. Mater. Trans. 54, 884–889. doi:10.2320/matertrans.MD201225
Anand, L. (1982). Constitutive equations for the rate-dependent deformation of metals at elevated temperatures. J. Eng. Mater. Technol. 104, 12–17. doi:10.1115/1.3225028
Calabretta, M., Sitta, A., Oliveri, S. M., and Sequenzia, G. (2021). Power semiconductor devices and packages: solder mechanical characterization and lifetime prediction. IEEE Access 9, 22859–22867. doi:10.1109/ACCESS.2021.3056281
Chen, C., Choe, C., Suetake, A., and Suganuma, K. (2020). “Fatigue and creep properties of sintered Ag paste from room temperature to high temperature,” in TMS 2020 149th annual meeting and exhibition supplemental proceedings (Cham: Springer International Publishing), 707–716. doi:10.1007/978-3-030-36296-6_66
Chen, G., Wang, Y. Y., Li, P., Cui, Y., Shi, S. W., Yang, J., et al. (2022). Constitutive and damage model for the whole-life uniaxial ratcheting behavior of SAC305. Mech. Mater. 171, 104333. doi:10.1016/j.mechmat.2022.104333
Chen, G., Zhang, Z.-S., Mei, Y.-H., Li, X., Yu, D.-J., Wang, L., et al. (2014). Applying viscoplastic constitutive models to predict ratcheting behavior of sintered nanosilver lap-shear joint. Mech. Mater. 72, 61–71. doi:10.1016/j.mechmat.2014.02.001
Chen, T. F., and Siow, K. S. (2021). Comparing the mechanical and thermal-electrical properties of sintered copper (Cu) and sintered silver (Ag) joints. J. Alloys Compd. 866, 158783. doi:10.1016/j.jallcom.2021.158783
Choudhury, D. (2010). “3D integration technologies for emerging microsystems,” in 2010 IEEE MTT-S international microwave symposium, Anaheim, USA, 23-28 May 2010 (IEEE), 1–4. doi:10.1109/MWSYM.2010.5514747
Coffin, L. F. (2022). A study of the effects of cyclic thermal stresses on a ductile metal. Trans. Am. Soc. Mech. Eng. 76, 931–949. doi:10.1115/1.4015020
Dai, W. W.-M. (2016). Historical perspective of system in package (SiP). IEEE Circuits Syst. Mag. 16, 50–61. doi:10.1109/MCAS.2016.2549949
Elshabini, A., Wang, G., and Barlow, F. (2006). “Future trends in electronic packaging,” in Smart Structures and materials 2006: smart electronics, MEMS, BioMEMS, and nanotechnology, (SPIE), 255–262. doi:10.1117/12.668750
Fontanelli, A. (2008). “System-in-Package technology: opportunities and challenges,” in 9th international symposium on quality electronic design (isqed 2008), 589–593. doi:10.1109/ISQED.2008.4479803
Gain, A., and Zhang, L. (2019). Temperature and humidity effects on microstructure and mechanical properties of an environmentally friendly Sn–Ag–Cu material. J. Mater. Sci. 54, 12863–12874. doi:10.1007/s10853-019-03784-2
Gharaibeh, M. A., and Wilde, J. (2023). Numerical evaluation of sintered silver die attachments based on different material parameters and creep constitutive models. IEEE Trans. Components, Packag. Manuf. Technol. 13, 1187–1201. doi:10.1109/TCPMT.2023.3298744
Gu, M., Liu, T., Xiao, X., Li, G., and Liao, W. (2022). Simulation and experimental study of the multisized silver nanoparticles sintering process based on molecular dynamics. Nanomaterials 12, 1030. doi:10.3390/nano12061030
Haris, N. I. N., Sobri, S., Yusof, Y. A., and Kassim, N. K. (2021). An overview of molecular dynamic simulation for corrosion inhibition of ferrous metals. Metals 11, 46. doi:10.3390/met11010046
Hu, Y., Wang, Y., and Yao, Y. (2023). Molecular dynamics on the sintering mechanism and mechanical feature of the silver nanoparticles at different temperatures. Mater. Today Commun. 34, 105292. doi:10.1016/j.mtcomm.2022.105292
Jiang, H., Liang, S., Xu, Y., and Ramachandran, S. (2024). Investigation of effects of porosity and coarsening on thermal and mechanical properties of sintered silver die attachment. IEEE Trans. Electron Devices 71, 5603–5608. doi:10.1109/TED.2024.3421183
Lau, J. H. (2022). Recent advances and trends in advanced packaging. IEEE Trans. Components, Packag. Manuf. Technol. 12, 228–252. doi:10.1109/TCPMT.2022.3144461
Lederer, M., Gökdeniz, Z., Khatibi, G., and Nicolics, J. (2021). Constitutive equations for strain rate and temperature dependent mechanical behaviour of porous Ag-sintered joints in electronic packages. Microelectron. Reliab. 126, 114209. doi:10.1016/j.microrel.2021.114209
Lee, H.-J., Mahajan, R., Sheikh, F., Nagisetty, R., and Deo, M. (2020). “Multi-die integration using advanced packaging technologies,” in 2020 IEEE custom integrated circuits conference (CICC), 1–7. doi:10.1109/CICC48029.2020.9075901
Long, X., Chong, K., Chang, C., and Su, Y. (2023a). “Meso-scale crystal plasticity modeling of sintered silver nanoparticles in typical interconnected structures,” in 2023 24th International Conference on Electronic Packaging Technology (ICEPT) (Shihezi City, China: IEEE), 1–5. doi:10.1109/ICEPT59018.2023.10492057
Long, X., Chong, K., Su, Y., Chang, C., and Zhao, L. (2023b). Meso-scale low-cycle fatigue damage of polycrystalline nickel-based alloy by crystal plasticity finite element method. Int. J. Fatigue 175, 107778. doi:10.1016/j.ijfatigue.2023.107778
Long, X., Chong, K., Su, Y., Du, L., and Zhang, G. (2023c). Connecting the macroscopic and mesoscopic properties of sintered silver nanoparticles by crystal plasticity finite element method. Eng. Fract. Mech. 281, 109137. doi:10.1016/j.engfracmech.2023.109137
Long, X., Dong, R., Su, Y., and Chang, C. (2023d). Critical review of nanoindentation-based numerical methods for evaluating elastoplastic material properties. Coatings 13, 1334. doi:10.3390/coatings13081334
Long, X., Guo, Y., Chang, X., Su, Y., Shi, H., Huang, T., et al. (2021a). “Effect of temperature on the fatigue damage of SAC305 solder,” in 2021 22nd International Conference on Electronic Packaging Technology (ICEPT) (Xiamen, China: IEEE), 1–4. doi:10.1109/ICEPT52650.2021.9568127
Long, X., Guo, Y., Su, Y., Siow, K. S., and Chen, C. (2022). Constitutive, creep, and fatigue behavior of sintered Ag for finite element simulation of mechanical reliability: a critical review. J. Mater Sci. Mater Electron 33, 2293–2309. doi:10.1007/s10854-021-07474-1
Long, X., Guo, Y., Su, Y., Siow, K. S., and Chen, C. (2023e). A new unified creep-plasticity constitutive model coupled with damage for viscoplastic materials subjected to fatigue loading. Fatigue Fract. Eng. Mat. Struct. 46, 1413–1425. doi:10.1111/ffe.13935
Long, X., Guo, Y., Su, Y., Siow, K. S., and Chen, C. (2023f). Unveiling the damage evolution of SAC305 during fatigue by entropy generation. Int. J. Mech. Sci. 244, 108087. doi:10.1016/j.ijmecsci.2022.108087
Long, X., He, X., and Yao, Y. (2017). An improved unified creep-plasticity model for SnAgCu solder under a wide range of strain rates. J. Mater. Sci. 52, 6120–6137. doi:10.1007/s10853-017-0851-x
Long, X., Hu, Y., Su, T., Iyela, P. M., Su, Y., Yan, W., et al. (2024). Thermomechanical constitutive behaviour of 3D printed biomimetic polymer material under high strain rates. Polym. Test. 134, 108439. doi:10.1016/j.polymertesting.2024.108439
Long, X., Jia, Q., Shen, Z., Liu, M., and Guan, C. (2021b). Strain rate shift for constitutive behaviour of sintered silver nanoparticles under nanoindentation. Mech. Mater. 158, 103881. doi:10.1016/j.mechmat.2021.103881
Long, X., Xu, J., Wang, S., Tang, W., and Chang, C. (2020). Understanding the impact response of lead-free solder at high strain rates. Int. J. Mech. Sci. 172, 105416. doi:10.1016/j.ijmecsci.2020.105416
Luo, R., Hu, D., Qian, C., Liu, X., Fan, X., Zhang, G., et al. (2024). Molecular dynamics simulations on mechanical behaviors of sintered nanocopper in power electronics packaging. Microelectron. Reliab. 152, 115284. doi:10.1016/j.microrel.2023.115284
Ma, K., Liu, X., Sun, Y., Song, Y., Feng, Z., Zhou, Y., et al. (2023). A micromechanical analysis to the viscoplastic behavior of sintered silver joints under shear loading. Mater. Basel, Switz. 16, 4472. doi:10.3390/ma16124472
Ma, Z. L., Belyakov, S. A., Sweatman, K., Nishimura, T., Nishimura, T., and Gourlay, C. M. (2017). Harnessing heterogeneous nucleation to control tin orientations in electronic interconnections. Nat. Commun. 8, 1916. doi:10.1038/s41467-017-01727-6
Mathew, A., Dudek, R., Otto, A., Scherf, C., Rzepka, S., Subbiah, N., et al. (2021). “Lifetime modelling of sintered silver interconnected power devices by FEM and experiment,” in 2021 22nd international conference on thermal, mechanical and multi-physics simulation and experiments in microelectronics and microsystems (EuroSimE), 1–9. doi:10.1109/EuroSimE52062.2021.9410877
Mathew, A., Dudek, R., Otto, A., Scherf, C., Rzepka, S., Subhaiah, N., et al. (2022). “Investigation of reliability issues in sintered silver interconnected power devices and its lifetime prediction by FEM and experiment,” in CIPS 2022; 12th international conference on integrated power electronics systems, 1–10. Available at: https://ieeexplore.ieee.org/abstract/document/9862016 (Accessed September 5, 2024).
Motalab, M., Basit, M., Suhling, J. C., Bozack, M. J., and Lall, P. (2014). “Creep test method for determination of Anand parameters for lead free solders and their variation with aging,” in Fourteenth intersociety conference on thermal and thermomechanical phenomena in electronic systems (ITherm), 127–141. doi:10.1109/ITHERM.2014.6892273
Motalab, M., Paul, R., Saha, S., Mojumder, S., Ahmed, T., and Suhling, J. C. (2019). Atomistic analysis of the thermomechanical properties of Sn–Ag–Cu solder materials at the nanoscale with the MEAM potential. J. Mol. Model 25, 59. doi:10.1007/s00894-019-3939-1
Paknejad, S. A., and Mannan, S. H. (2017). Review of silver nanoparticle based die attach materials for high power/temperature applications. Microelectron. Reliab. 70, 1–11. doi:10.1016/j.microrel.2017.01.010
Pei, M., and Qu, J. (2005). “Constitutive modeling of lead-free solders,” in InterPACK2005, (advances in electronic packaging, parts A, B, and C), 1307–1311. doi:10.1115/IPACK2005-73411
Siviour, C. R., Walley, S. M., Proud, W. G., and Field, J. E. (2005). Mechanical properties of SnPb and lead-free solders at high rates of strain. J. Phys. D. Appl. Phys. 38, 4131–4139. doi:10.1088/0022-3727/38/22/018
Su, Y., Fu, G., Liu, C., Liu, C., and Long, X. (2021a). Fatigue crack evolution and effect analysis of Ag sintering die-attachment in SiC power devices under power cycling based on phase-field simulation. Microelectron. Reliab. 126, 114244. doi:10.1016/j.microrel.2021.114244
Su, Y., Fu, G., Liu, C., Zhang, K., Zhao, L., Liu, C., et al. (2021b). Thermo-elasto-plastic phase-field modelling of mechanical behaviours of sintered nano-silver with randomly distributed micro-pores. Comput. Methods Appl. Mech. Eng. 378, 113729. doi:10.1016/j.cma.2021.113729
Su, Y., Tu, B., Tang, R., and Long, X. (2022). “Thermal effects on fracture behaviors of the die-attachment in the SiC power device under power cyclic conditions based on fracture phase-field modeling,” in 2022 23rd International Conference on Electronic Packaging Technology (ICEPT) (Dalian, China: IEEE), 1–4. doi:10.1109/ICEPT56209.2022.9873144
Su, Y., Zhu, J., Long, X., Zhao, L., Chen, C., and Liu, C. (2023). Statistical effects of pore features on mechanical properties and fracture behaviors of heterogeneous random porous materials by phase-field modeling. Int. J. Solids Struct. 264, 112098. doi:10.1016/j.ijsolstr.2022.112098
Tan, Y., Li, X., and Chen, X. (2014). Fatigue and dwell-fatigue behavior of nano-silver sintered lap-shear joint at elevated temperature. Microelectron. Reliab. 54, 648–653. doi:10.1016/j.microrel.2013.12.007
Tu, K. N., and Zeng, K. (2001). Tin–lead (SnPb) solder reaction in flip chip technology. Mater. Sci. Eng. R Rep. 34, 1–58. doi:10.1016/S0927-796X(01)00029-8
Wang, H., Ma, J., Yang, Y., Gong, M., and Wang, Q. (2023). A review of system-in-package technologies: application and reliability of advanced packaging. Micromachines 14, 1149. doi:10.3390/mi14061149
Xie, M., and Chen, G. (2022). A developed crystal plasticity model for viscoplastic mechanical behavior of SAC305 solder under thermomechanical coupled cyclic loading. Int. J. Plasticity 159, 103465. doi:10.1016/j.ijplas.2022.103465
Xie, M., Chen, G., Yu, J., Wu, Y., Liu, X., Yang, J., et al. (2022). A dual-phase crystal plasticity finite-element method for modeling the uniaxial deformation behaviors of thermally aged SAC305 solder. Int. J. Plasticity 155, 103342. doi:10.1016/j.ijplas.2022.103342
Xie, M. W., Chen, G., Yang, J., and Xu, W. L. (2021). Temperature- and rate-dependent deformation behaviors of SAC305 solder using crystal plasticity model. Mech. Mater. 157, 103834. doi:10.1016/j.mechmat.2021.103834
Yao, P., Li, X. Y., Jin, F. Y., and Yang, L. (2020). Morphology transformation on Cu3Sn grains during the formation of full Cu3Sn solder joints in electronic packaging. Solder. and Surf. Mt. Technol. 30, 14–25. doi:10.1108/SSMT-10-2017-0038
Ye, G., Zhang, J., Zhang, P., and Meng, K. (2024). Sintering mechanism between silver nanoparticles and SiC/Cu plates: a molecular dynamics simulation. Powder Technol. 439, 119695. doi:10.1016/j.powtec.2024.119695
Zhang, G., and Liu, Y. (2024). Effect of sintering process on mechanical properties of uniaxial tensile sintered nano-silver component. Phys. B Condens. Matter 673, 415502. doi:10.1016/j.physb.2023.415502
Zhang, L., Sun, G., Li, L., and Zhang, X. (2007). Effect of copper oxide layer on solder wetting temperature under a reduced atmosphere. in 2007 8th International Conference on Electronic Packaging Technology. Shanghai, China, 14-17 August 2007 (IEEE), doi:10.1109/ICEPT.2007.4441423
Zhang, S., Li, Z., Zhou, H., Li, R., Wang, S., Paik, K.-W., et al. (2022). Challenges and recent prospectives of 3D heterogeneous integration. e-Prime - Adv. Electr. Eng. Electron. Energy 2, 100052. doi:10.1016/j.prime.2022.100052
Zhang, S., Xu, X., Lin, T., and He, P. (2019). Recent advances in nano-materials for packaging of electronic devices. J. Mater Sci. Mater Electron 30, 13855–13868. doi:10.1007/s10854-019-01790-3
Zhang, X., Hu, X., Jiang, X., and Li, Y. (2018). Effect of Ni addition to the Cu substrate on the interfacial reaction and IMC growth with Sn3.0Ag0.5Cu solder. Appl. Phys. A 124, 315. doi:10.1007/s00339-018-1736-9
Zhang, Z., Wan, B., Fu, G., Su, Y., Wu, Z., Wang, X., et al. (2024). Impact analysis of microscopic defect types on the macroscopic crack propagation in sintered silver nanoparticles. CMES 139, 441–458. doi:10.32604/cmes.2023.043616
Zhao, M., Zhang, L., Liu, Z.-Q., Xiong, M.-Y., and Sun, L. (2019). Structure and properties of Sn-Cu lead-free solders in electronics packaging. Sci. Technol. Adv. Mater. 20, 421–444. doi:10.1080/14686996.2019.1591168
Zhu, J., Su, Y., Chen, C., Siow, K. S., Tang, R., and Long, X. (2021). “Porosity effect on fracture behavior of sintered silver nanoparticles by phase-field modeling,” in 2021 IEEE 23rd Electronics Packaging Technology Conference (EPTC) (Singapore, Singapore: IEEE), 317–321. doi:10.1109/EPTC53413.2021.9663905
Keywords: multi-scale mechanics, electronics packaging, interconnect materials, finite element analysis, reliability analysis
Citation: Zhou Z, Lang F, Farlim V, Zhang Z, Li S and Dong R (2024) Review on multi-scale mechanics fundamentals and numerical methods for electronics packaging interconnect materials. Front. Mater. 11:1532859. doi: 10.3389/fmats.2024.1532859
Received: 22 November 2024; Accepted: 09 December 2024;
Published: 24 December 2024.
Edited by:
Chuantong Chen, Osaka University, JapanReviewed by:
Nur Izzah Nabilah Haris, Putra Malaysia University, MalaysiaLi Liu, Wuhan University of Technology, China
Kyle He, Central South University, China
Copyright © 2024 Zhou, Lang, Farlim, Zhang, Li and Dong. This is an open-access article distributed under the terms of the Creative Commons Attribution License (CC BY). The use, distribution or reproduction in other forums is permitted, provided the original author(s) and the copyright owner(s) are credited and that the original publication in this journal is cited, in accordance with accepted academic practice. No use, distribution or reproduction is permitted which does not comply with these terms.
*Correspondence: Zhongqing Zhang, YmVja2VldEAxMjYuY29t; Shiyang Li, bHN5YW5nQG53cHUuZWR1LmNu