- 1Department of Prosthetic Dental Sciences, College of Dentistry, King Saud University, Riyadh, Saudi Arabia
- 2Dental Internship Training Program, College of Dentistry, King Saud University, Riyadh, Saudi Arabia
Pre-etching ceramic restorations in dental laboratories is preferred by numerous dentists due to the potential health concerns associated with handling hydrofluoric acid (HF) if not managed correctly. This study aimed to evaluate the effect of different surface treatments on the shear bond strength (SBS) between lithium disilicate ceramics and self-adhesive resin cement. For this study, a total of 60 IPS e. max CAD (Ivoclar Vivadent) lithium disilicate specimens were prepared. Specimens were randomly divided into six groups, with 10 specimens per each. Different surface treatments were applied (1: Control group no surface treatment (C); 2: 10% hydrofluoric with silane coupling agent (HFS); 3: 10% hydrofluoric acid only (HF); 4: Sandblasting with 50 μm aluminum-oxide powder (AO); 5: Sandblasting with 30 μm silica-coated aluminum-oxide powder (SC), and 6: Er,Cr:YSGG laser (L). SBS tests were performed, and data analyzed using one-way ANOVA and Tukey’s multiple comparisons. The difference between the mean average of SBS was statistically significant among all different surface treatments (p ≤ 0.05) except for the L group with a (p = 1.00). The highest mean value was observed in the HFS followed by the HF group. Whereas the L group showed no statistical significance in comparison with the C group (p > 0.05). Hydrofluoric acid etching in combination with a silane coupling agent was the most effective surface treatment for bonding lithium disilicate ceramics with resin cement. In contrast, laser irradiation was the least effective among all other groups.
1 Introduction
Many novel dental ceramic materials that are both aesthetically appealing and extremely durable have recently been discovered. There are several high-strength ceramic systems with different therapeutic indications that are available, including zirconium ox-ide, lithium disilicate, infiltrating alumina, and densely sintered aluminum oxide ceramics (Bajraktarova-Valjakova et al., 2018). Lithium disilicate glass ceramics, a new generation of heat-pressure ceramics, offer greater bending and fracture resistance than the preceding generation of ceramics reinforced by leucite (Phark and Duarte, 2022). In general, lithium disilicate is thought of as an acid etchable ceramic made up of 60%–65% crystals of lithium oxide (Li2O) embedded in a matrix of silica glass [Zarone et al., 2023].
The cementation process has a major influence on how long ceramic restorations last. A number of clinical studies have suggested that clinical failure could result from the restoration’s insufficient luting performance (Sinha, 2023). Adhesive and non-adhesive cementing techniques are the two varieties. The procedure of adhesive cementation strengthens the bond between the restorative material and substrate by combining adhesive chemical bonding with micromechanical interlocking (Abad-Coronel et al., 2019). Conventional (non-adhesive) cementation fills the area between the restoration and the original tooth with a luting solution and relies only on macro and micromechanical retention (Ghodsi et al., 2021). The indications for each type of cementation are determined by the composition of the ceramic, the available forms for preparation, retention, and resistance, as well as by the field control during cementation. Recent developments in adhesive dentistry have led to numerous studies examining various ceramic restorative surface treatments in an effort to increase bond strength. Like silica coating, sandblasting, and acid etching (Perdigão et al., 2021).
The clinical service outcome of lithium disilicate is significantly influenced by the resin cement and ceramic bond. Robust and strong resin bonding increases marginal adaptation, strengthens fracture resistance, increases retention, and reduces bacterial micro leakage (Simasetha et al., 2022). The cement-ceramic bond can be created by surface wettability, chemical bonding to a silica-based lithium disilicate surface, and micromechanical retention. To achieve micromechanical retention, the surface can be prepared by abrasion with airborne particles or by etching with hydrofluoric acid. Airborne particle abrasion is not recommended since it significantly reduces flexural strength and does not give lithium disilicate a satisfactory bond strength (Blatz et al., 2018).
Hydrofluoric acid etching dissolves the glass phase from the matrix, produces micro-porosity, and increases surface areas. The cement-ceramic surfaces can be chemically coupled by means of a silane coupling agent. The silane coupling agent is a bifunctional chemical that enables covalent connections with hydroxyl (OH) groups on the lithium disilicate surface to facilitate adhesion. One functional group can react with the inorganic lithium disilicate surface, and the other methacrylate group can react with an organic resin matrix (Ramakrishnaiah et al., 2016). Silane and Si-OH combine to generate a condensation reaction bond on a ceramic surface. Methyl methacrylate’s double bonds hold the adhesive to the surface. Applying silane before using resin cement to lute will strengthen the bond by enhancing infiltration into the surface flaws of the etched lithium disilicate. Furthermore, the resin cement has a major impact on the binding to high crystal-line content ceramics. Adhesion between lithium disilicate and resin cement is the outcome of a physico-chemical interaction over the resin-ceramic contact (Fidalgo-Pereira et al., 2023).
The best method to improve the adhesion between resin cement and ceramic surfaces is still up for debate, despite a number of research comparing those various surface treatments (Abad-Coronel et al., 2019; Ghodsi et al., 2021; Perdigão et al., 2021; Simasetha et al., 2022; Blatz et al., 2018; Ramakrishnaiah et al., 2016; Fidalgo-Pereira et al., 2023). Thus, this study’s objective was to assess and compare the effect of different surface treatments including 10% hydrofluoric acid with silane coupling agent, 10% hydrofluoric acid only, Sandblasting with 50 μm Al₂O₃,30 μm silica-coated Al₂O₃ and Er,Cr:YSGG laser on the shear bond strength (SBS) between lithium disilicate and self-adhesive resin cement (RelyXU200). In addition, the surface topography of the surfaces before and after debonding of the tested specimens were also evaluated by using a scanning electron microscope (SEM).
2 Materials and methods
The study was carried out in the Department of Prosthodontics at King Saud University’s College of Dentistry and College of Dentistry Research Center (CDRC) in Riyadh, Saudi Arabia. The CDRC at King Saud University in Riyadh (CDRC # lR 0,466) provided ethical approval.
2.1 Specimen fabrication and grouping
Two groups of sixty samples each were formed from a total of 120 lithium disilicate (IPS e. max CAD, Ivoclar Vivadent, Liechtenstein) square shaped samples that were manufactured in the laboratory in accordance with the manufacturer’s instructions (Figure 1). Groups (A) and (B) have different dimensions (10 mm × 10 mm × 2 mm) and (5 mm × 5 mm × 2 mm), respectively. Group (A) samples were all embedded in a powder and liquid mixture of self-curing polymer, poly-methylmethacrylate (Major Ortho, Major Prodotti Dentari, Moncalieri, Italy), and then mounted in cylindrical molds made of polyvinyl chloride that had dimensions of 10 mm in diameter and 15 mm in height to accommodate the testing apparatus (Figures 1, 2). Group (B) samples were not embedded and cemented on the samples of group (A). Group (B) specimens were randomly divided into six groups with 10 specimens per each group (n = 10). Different surface treatment methods were applied on the specimens of Group (B).
• Group (1): Control group without any surface treatment (C).
• Group (2): 10% hydrofluoric acid with silane coupling agent (HFS).
• Group (3): 10% hydrofluoric acid (HF).
• Group (4): Sandblasting with 50 μm Al₂O₃ (AO).
• Group (5): Coating with 30 μm silica-coated Al₂O₃ (SC).
• Group (6): Er,Cr:YSGG laser (erbium, chromium:yttrium, scandi-um, gallium, garnet) (L) [Figures 1, 2].
2.2 Surface treatment of the specimens
All the materials and manufacturers of the present study are listed and described in Table 1. Each specimen was polished using aluminum oxide sandpaper and cleansed for 10 min with an ultrasonic cleaner to remove any abrasive particles and then air-dried. Subsequently, the specimens were divided into six groups for different surface treatments. In group (1) control group, no surface treatment was applied. Group (2) was treated with 10% hydrofluoric acid (Condac Porcelana, FGM, Joinville, SC, Brazil) for 20 s (s), rinsed for 20 s, and dried. Then silane coupling agent (Silane Primer, Pentron, United States) was applied, air dried, and light cured for 30 s. Group (3) was treated with 10% hydrofluoric acid (Condac Porcelana, FGM, Joinville, SC, Brazil) for 20 s, rinsed for the 20 s, and dried. In group (4) sandblasting of the specimens were carried out using 50 μm Al₂O₃ (Aluminium oxide 50 μm, Shera, Germany) for 15 s under atmospheric pressure of 2.8 atm at 10 mm distance. Following sandblasting the specimens were cleansed in the ultrasonic cleaner for 60 s and dried. Group (5) specimens were coated using 30 μm silica-coated Al₂O₃ (CoJet™ Sand, 3 M ESPE, Neuss, Germany) for 15 s under atmospheric pressure of 2.8 atm at 10 mm distance. Group (6) specimens were treated with Er,Cr:YSGG laser, water-cooled laser (Waterlase iPlus, Biolase, Irvine, California, United States) applied at the surface at 1 W, 10 Hz for 40 s at 10 mm distance.
2.3 Observing the treated surfaces under SEM
After treatment, one specimen from each group was analyzed under a scanning electron microscope (SEM) with ×300 magnification to show the various surface morphologies (Figure 3) to assess the impact of surface treatment.
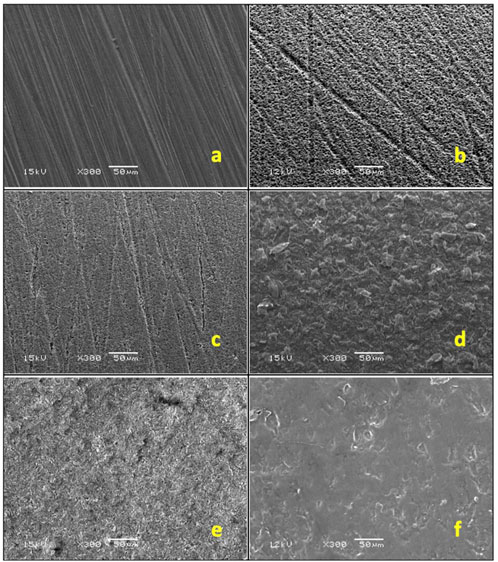
Figure 3. SEM images: (A) control, (B) hydrofluoric acid with silane, (C) hydrofluoric acid, (D) Al₂O₃ sandblasting, (E) silica-coated Al₂O₃ sandblasting (F) Er: YAG laser.
2.4 Profilometry analysis for surface roughness
Additionally, the surface roughness (Sa) measurements were performed on representative specimens from each treatment group using a 3-D profilometer (Contour-GT-X®, 3D Optical Microscope, Bruker Nano Surfaces Division, San Jose, CA, United States) after the surface treatments. Minimum three readings were recorded for each specimen and the mean of the three readings was considered as the final reading for the test group.
2.5 Cementation of the specimens with self-adhesive resin cement
Self-adhesive resin cement (RelyX U200 Automix, 3 M ESPE, St. Paul, MN, United States) was used to cement each specimen. Using a dental surveyor, a platform weighing 3 kg was created to ensure that the load applied during cementation was uniform (Figure 4). As directed by the manufacturer, resin cement was put on the specimens and allowed to set for 20 seconds, with a light-emitting diode (LED) (Bluephase G2, Ivoclar Vivadent, Schaan, Liechtenstein) for 40 s on each side. An explorer was used to remove extra cement.
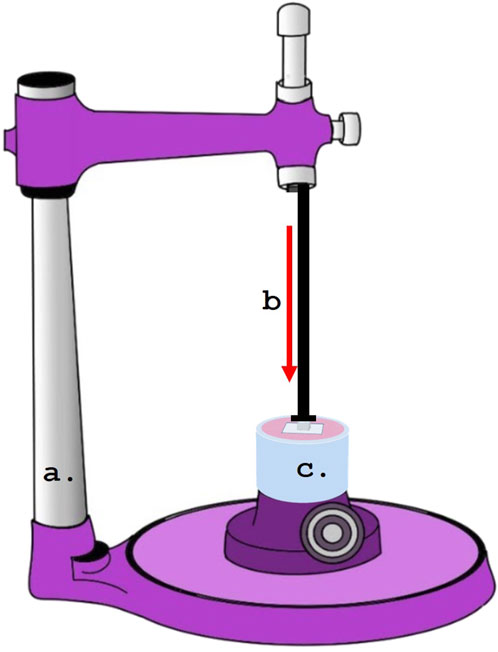
Figure 4. Dental surveyor used for the cementation of specimens under standardized loading. (A) Dental Surveyor.; (B) Load application during cementation.; (C) Specimens.
2.6 Aging and thermocycling of specimens
To replicate the conditions of the oral cavity, all groups were submerged for 24 h at 37°C in distilled water following cementation. The specimens were then aged by being placed in a thermocycling machine (Huber, SD-Mechatronik-Thermocycler, Germany) for 6,000 rotations, with a rest time of 30 s and a transition time of 5 s, at temperatures ranging from 5°C to 55°C.
2.7 Testing the shear bond strength (SBS) and the type of bonding failure
A universal testing apparatus (Instron, model 8,500 Plus; Dynamic Testing System; Instron Corp.) was used to measure the SBS for each specimen. A knife-edge piston was positioned at the interface parallel to the ceramic surface of Group-B specimens and driven at a crosshead speed of 1 mm/min while the ceramic block was loaded vertically into the metal testing device (Figures 5, 6). The SBS value for each specimen was reported in MPa. To ascertain each specimen’s failure mode, the surfaces of all specimens were visually inspected and subjected to a digital microscope examination (HIROX, KH-7700, Digital microscope system, Tokyo, Japan) at a ×50 magnification following debonding. Additionally, a single specimen from per group was studied with an ×300 SEM magnification (Figure 6). Three categories were used to group the failure modes: adhesive failure, cohesive failure, and combined failure.
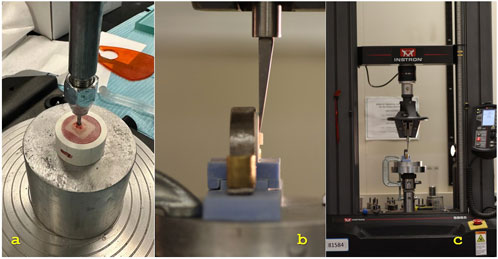
Figure 5. (A) Cementation of specimen under the load.; (B) Close up view under the universal testing machine.; (C) Placement of the specimen in the universal testing machine.
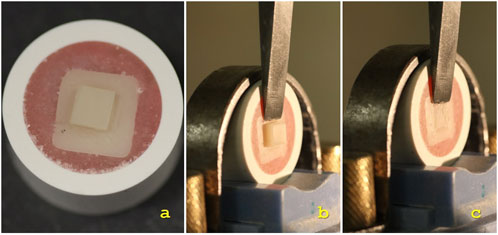
Figure 6. (A) Prepared specimen.; (B) Specimen in the universal testing machine before debonding.; (C) Specimen in the universal testing machine after debonding.
2.8 Statistical analysis
SPSS Version 23 was used to examine the data that had been gathered (SPSS Inc., Chicago, IL, United States). The statistical examination compared SBS mean values with 95% confidence intervals for all samples from all labs using one-way analysis of variance (ANOVA) and Tukey’s post hoc testing for multiple comparisons. A significant threshold of p ≤ 0.05 was established.
3 Results
This study demonstrated that the various surface treatments done to lithium disilicate ceramic had a significant effect on the SBS when using self-adhesive resin cement. The 10% hydrofluoric acid with silane group had the highest mean SBS value (40.48 ± 13.32 MPa), followed by the 10% hydrofluoric acid only group (33.96 ± 11.58 MPa). Nevertheless, there was no discernible statistical difference between the two groups (p > 0.05). The laser group recorded 1.68 ± .37 MPa and the control group recorded 0.94 ± 0.22 MPa, respectively, with no statistically significant difference between the two groups (p > 0.05). The mean values of the Al₂O₃ sandblasting and silica-coated Al₂O₃ coating groups were 23.23 ± 7.15 MPa and 6.70 ± 2 MPa, respectively, with no statistically significant differences (p > 0.05). Nevertheless, both were remarkably higher than the control and laser groups but lower than HFS and HF groups (Table 2).
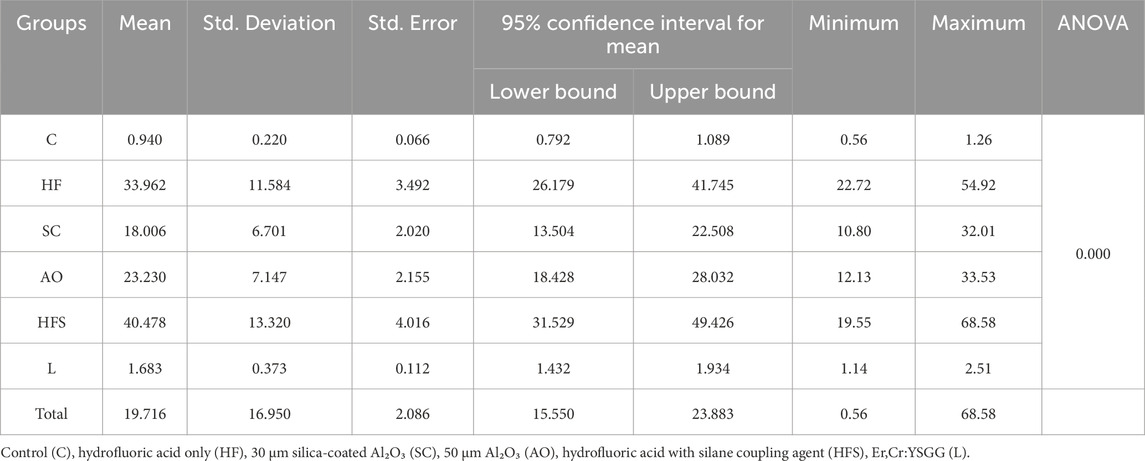
Table 2. Mean and standard deviation of shear bond strength values (MPa) for the six experimental groups.
The most successful surface treatment was 10% hydrofluoric acid with silane, with a mean value noticeably higher than all other groups (p < 0.05) and little difference with the group treated with 10% hydrofluoric acid alone. The laser group, on the other hand, had the least impact on SBS, with a mean value that was considerably lower than that of any other group (p < 0.05) save for the control group. With a mean value significantly higher than the laser and control groups (p < 0.05), but lower than the 10% hydrofluoric acid with saline and 10% hydrofluoric acid groups (p < 0.05), both sandblasting groups demonstrated improvement in SBS. Nonetheless, unimportant in relation to one another (p > 0.05) (Table 3).
At the ceramic-cement interface, adhesive and mixed failure modes were seen in most specimens (Table 4). Compared to the other groups, groups HFS, HF, and AO displayed higher levels of cement-retained on the surface. Some samples of the SC group displayed more than half of the cement retained. Only two samples with retained cement were displayed in the control group, and none at all in the L group. Adhesive failures and combined cement and lithium disilicate failures accounted for the majority of bond failures (Figure 7).
A highly smooth surface was visible in the control group (Figure 3A). Hydrofluoric acid-treated groups generated uniformly retentive, elongated linear crystals (Figures 3B, C). A similar irregularity pattern was produced by airborne abrasion using 30 μm silica particles and 50 μm Al2O3 particles (Figures 3D, E). For the test group treated with Er,Cr:YSGG laser, three distinct pilot samples underwent SEM evaluation after being treated with varying powers (1, 2, and 3 W) (Figure 8). A power of 1 W was utilized since surfaces exposed to 2 W and 3 W radiation-induced greater melting than retentive impact. In contrast to the other groups, less retentive regions were discovered under laser irradiation (Figure 3F).
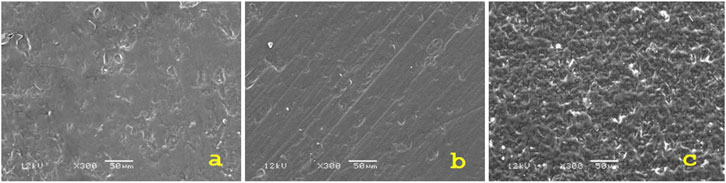
Figure 8. SEM images under ×300 magnification of various samples that treated using different power (A) 1W, (B) 2W, (C) 3 W.
Table 5 displays the Sa values for each of the test groups. The AO group had the highest Sa values (Sa = 4,527.44), followed by the SC group (Sa = 1,054.48) as expected because particles of both these materials were blasted over the ceramic specimens in both groups. The L group, however, had the lowest Sa value (Sa = 257.32), revealing that the laser application had a smoothing impact on the lithium disilicate ceramics' surface.
4 Discussion
This study aimed to evaluate the effects of different surface treatments on the self-adhesive resin cement and lithium disilicate ceramic SBS. In this investigation, test specimens with the same dimensions and forms were used to standardize the cementation process and achieve consistency. To find out if the SBS of these surface-treated specimens and resin cement improved, the test specimens' surfaces were subjected to various surface treatments. Using universal testing equipment from Instron, the SBS values of the cemented lithium disilicate specimens were determined in megapascals (MPa). Moreover, a scanning electron microscope (SEM) was used to scan the surface topography of the surfaces both before and after the tested specimens' debonding. Numerous scholars have noted that the method used in this protocol to measure the SBS using universal testing equipment produces reliable results and is one of the most frequently recommended methods (Jayasheel et al., 2017). Measurements of quantitative bond strength have demonstrated the superiority and dependability of universal testing apparatus (El Mourad, 2018; Ismail et al., 2021; Sirisha et al., 2014). The SBS values in MPa continue to be a helpful general bond strength guideline because they are a practical and understandable value that allows the comparison of the SBS values of the tested specimens with different surface treatments and cemented with resin cement, as well as the comparison of the current findings with other studies and standards (Jungbauer et al., 2022). Additional useful information on the SBS values of the materials under test was obtained by using SEM to evaluate the surfaces of the tested specimens.
In the current study, the SBS between resin cement and lithium disilicate dental restorative material with different surface treatments was examined. Lithium disilicate ceramic has grown a lot in popularity and is utilized a lot, particularly in circumstances that are quite attractive. It can be applied in a range of clinical settings and has shown to be an extremely durable substance. Understanding the intricacy of this material’s structure is crucial to be able to use it confidently as a ceramist, technician, or clinician. The physical qualities of this material are greatly influenced by its microstructure, which can be altered by various surface treatments (Zarone et al., 2019). This can change the material’s bond strength with resin cement which is the cement of choice when using glass ceramics (Zarone et al., 2016). The goal of the current study was to evaluate and examine how various surface treatments affected the lithium disilicate bond strength with resin cement. The study’s findings demonstrated that all surface treatments—except laser irradiation—improved the self-adhesive resin to lithium disilicate ceramic’s SBS, therefore, the null hypothesis stating that various surface treatments have no effect on the shear bond strength between lithium disilicate and resin was thus disproved.
The clinical performance, success, and longevity of the ceramic restorations are significantly influenced by the cementation technique and adhesive cement type employed (Vargas et al., 2011; Chirca et al., 2021). When entire ceramic crowns were bonded with resin cement, their fracture resistance rose (Jurado et al., 2023). This was because the resin filled in the imperfections on the restoration’s inside surface, halting the spread of cracks (Fathy et al., 2022; Tyor et al., 2023). Moreover, resin cement is superior to other forms of cement on the market in terms of its mechanical, physical, and aesthetic qualities (Muhammed et al., 2023). To offer acceptable surface qualities, several procedures such as acid etching, laser irradiation, diamond rotary tools, airborne particle abrasion with silica or aluminum oxide, and combinations of any of these are commonly utilized (Bajraktarova-Valjakova et al., 2018; Berk et al., 2008). Because the microstructure has a major impact on the bond strength, it is imperative to choose the right surface treatment for the type of ceramic (Limpuangthip et al., 2023).
The surfaces treated with 10% hydrofluoric acid and silane coupling agent had the highest SBS values in the current study, with a mean value of (40.478 Mpa). This was in line with an earlier investigation by Lyann et al. (2018), who demonstrated similar SBS values of (40.6 ± 6.3 Mpa) when using acid etching with hydrofluoric acid and silane coupling agent. Hydrofluoric acid etching and silanization, in which the silica particles react with the hydrofluoric acid, make up the micromechanical and chemical bonding mechanism of silica-based ceramics (Yavuz and Eraslan, 2016). Acid etching increases the free energy microporosity and surface area, which lowers the contact angle and enhances the wettability of resin cement and lithium disilicate (Levartovsky et al., 2021). Little, randomly oriented, interconnecting, densely packed, needle-like lithium disilicate crystals make up the architecture of lithium disilicate, to which even smaller secondary lithium orthophosphate crystals are added. By dissolving and eliminating the glassy matrix including silica and silicates, HF etching creates a porous surface. Increased surface micromechanical retention is provided by the secondary crystal phase (Li et al., 2019). HF, in particular, has the potential to break down the glassy matrix of the ceramic, leaving the lithium disilicate surface with an uneven microstructure. This impact increased the surface area for micromechanical bonding and interlocking, which increased the bond strength (Ersu et al., 2009; Fischer et al., 2008).
Airborne abrasion with Al2O3 is another effective method that is often used on oxide ceramics (Fidalgo-Pereira et al., 2023). In order to improve the surface area by producing more micromechanical cuts for the cement interlocking, several manufacturers proposed utilizing airborne particle abrasion to increase surface roughness (Fischer et al., 2008). As shown by SEM Figure 2, increasing the powder size from 30 μm to 50 µm has enhanced the surface roughness while maintaining a constant duration and pressure. However, excessive roughness on ceramic surfaces might lead to weak interfacial interactions instead of strong ones in certain cases (De Jager et al., 2000; Erdemir et al., 2014).
Consistent with earlier studies (Fischer et al., 2008; Hummel and Kern, 2004; Sadan et al., 2003), the Al2O3 sandblasting group in our study showed significantly higher SBS values (23.23 ± 7.15 MPa) than the control group. However, the current study found no statistically significant differences between 30 μm silica particles and 50 μm Al2O3. In the present investigation, the use of advanced surface pretreatments techniques beyond the commonly used standard surface treatments were studied, including Er,Cr:YSGG laser irradiation to evaluate its effect on SBS. It is expected that silica-based ceramics will lose their glass phase when exposed to laser light, resulting in a rough surface (Yavuz et al., 2017). However, the current results showed that Er,Cr:YSGG laser irradiation had no effect on the bonding strength with resin cement when compared to the control samples. Similar findings were reached by Foxton et al. (2011) when they examined the effect of the Er:YAG laser on the SBS of resin cement and alumina ceramics. Additionally, similar SBS values of (3.88 ± 1.94 MPa) using laser-irradiation with a power of 1.5 were reported in another investigation by Kursoglu et al. (2013).
The mode of failure in the current study correlated to the shear bond strength values, in other words, it was predominantly cohesive failure in the groups that displayed higher shear bond strength values such as the HFS, HF and AO groups, and as the shear bond strength decreased such as in the group treated with laser, the adhesive failure mode was predominating. It is reasonable to explain such correlation to the fact that the stronger the bond between the cement and the ceramic surface, the more cement portions retain on the ceramic surface. Understanding the bonding mechanisms with the different surface treatment modalities used in the current investigation may be partially aided by the results of the Sa measurements conducted using a profilometer. The maximum Sa values were shown by airborne-particle abrasion with 50 μm Al₂O₃ particles. This was also supported by the SEM pictures, which showed rough and uneven surfaces at varying depths and heights. In contrast to the control, laser-treated, and silica-coated groups, these morphological alterations produced interlocking retentive structures in the lithium disilicate surface, resulting in a micromechanical bond that increased the SBS. The SBS of the AO samples was lower than that of the hydrofluoric acid with and without silane, despite the fact that they seemed the roughest of all the examined groups. This result was consistent with previous studies that showed the strongest bonds were created by hydrofluoric acid treatment followed by silane application (Abdulkader et al., 2021; Ayad et al., 2008; Şişmanoğlu et al., 2020). When combined with silane, the roughness of the ceramics produced by hydrofluoric acid etching resulted in an efficient production of siloxane linkages upon mixing with the acid solution, according to Ayad et al. (Ayad et al., 2008). In terms of shear bond strength, this chemical link was superior than the micromechanical characteristics of the acid-etched HFS and HF groups, which were visible in SEM images as grooves and pores. However, the lowest Sa values were obtained after laser treatment. SEM examination validated this conclusion by demonstrating that laser treatment created smooth surface topography devoid of retentive micromechanical features, which may account for the low SBS values that did not differ significantly from the control groups.
The present investigation had certain limitations. Although standard protocols were followed during sample fabrication, specimen’s surface characteristics may have been affected during manufacturing process, besides, handling of the materials during production might have an impact on the outcome. Moreover, the study only used one type of self-adhesive resin cement; therefore, greater investigation into other types of cements is strongly advised in order to better understand the long-term behavior of SBS. Additionally, as this study was conducted in vitro, it was unable to account for all the factors that might be present in the oral media, such as fatigue and pH changes. Considering the aforementioned constraints, it is imperative to carefully evaluate the current study’s findings.
5 Conclusion
Based on the findings of the study, it can be concluded that.
• Using 10% hydrofluoric acid with silane coupling agent effectively improved the shear bond strength between lithium disilicate ceramics and self-adhesive resin cement.
• There was no statistical significance between using hydrofluoric acid with and without silane coupling agent.
• Using 30 μm silica particles and 50 μm Al2O3 showed lower shear bond strength values than 10% hydrofluoric acid between lithium disilicate ceramics and self-adhesive resin cement.
• No significant distinctions between 30 μm silica particles and 50 μm Al2O3 were found.
• Er,Cr:YSGG laser was the least effective in enhancing the shear bond strength.
• The combination of chemical bonding and physical bonding (micromechanical interlocking) is responsible for the long-term bonding durability.
Author contributions
GA: Conceptualization, Data curation, Formal Analysis, Funding acquisition, Resources, Supervision, Validation, Writing–original draft, Writing–review and& editing. RA: Data curation, Investigation, Methodology, Writing–original draft. BA: Data curation, Investigation, Methodology, Writing–original draft. FA: Data curation, Investigation, Methodology, Writing–original draft. AB: Data curation, Funding acquisition, Investigation, Methodology, Writing–original draft. SH: Conceptualization, Formal Analysis, Software, Supervision, Writing–original draft, Writing–review and editing.
Funding
The author(s) declare that financial support was received for the research, authorship, and/or publication of this article. The research was funded by Researchers Supporting Project number (RSPD 2024R950), King Saud University, Riyadh, Saudi Arabia.
Conflict of interest
The authors declare that the research was conducted in the absence of any commercial or financial relationships that could be construed as a potential conflict of interest.
Publisher’s note
All claims expressed in this article are solely those of the authors and do not necessarily represent those of their affiliated organizations, or those of the publisher, the editors and the reviewers. Any product that may be evaluated in this article, or claim that may be made by its manufacturer, is not guaranteed or endorsed by the publisher.
References
Abad-Coronel, C., Naranjo, B., and Valdiviezo, P. (2019). Adhesive systems used in indirect restorations cementation: review of the literature. Dent. J. (Basel) 7 (3), 71. doi:10.3390/dj7030071
Abdulkader, K. F., Elnaggar, G. A., and Kheiralla, L. S. (2021). Shear bond strength of cemented zirconia-reinforced lithium silicate ceramics (Celtra Duo) with two surface treatments (in vitro study). J. Adhesion Sci. Technol. 35 (1), 35–51. doi:10.1080/01694243.2020.1785082
Ayad, M. F., Fahmy, N. Z., and Rosenstiel, S. F. (2008). Effect of surface treatment on roughness and bond strength of a heat-pressed ceramic. J. Prosthet. Dent. 99 (2), 123–130. doi:10.1016/s0022-3913(08)60028-1
Bajraktarova-Valjakova, E., Korunoska-Stevkovska, V., Kapusevska, B., Gigovski, N., Bajraktarova-Misevska, C., and Grozdanov, A. (2018). Contemporary dental ceramic materials, A review: chemical composition, physical and mechanical properties, indications for use. Open Access Maced. J. Med. Sci. 6 (9), 1742–1755. doi:10.3889/oamjms.2018.378
Berk, N., Başaran, G., and Ozer, T. (2008). Comparison of sandblasting, laser irradiation, and conventional acid etching for orthodontic bonding of molar tubes. Eur. J. Orthod. 30 (2), 183–189. doi:10.1093/ejo/cjm103
Blatz, M. B., Vonderheide, M., and Conejo, J. (2018). The effect of resin bonding on long-term success of high-strength ceramics. J. Dent. Res. 97 (2), 132–139. doi:10.1177/0022034517729134
Chirca, O., Biclesanu, C., Florescu, A., Stoia, D. I., Pangica, A. M., Burcea, A., et al. (2021). Adhesive-ceramic interface behavior in dental restorations. FEM study and SEM investigation. Mater. (Basel) 14 (17), 5048. doi:10.3390/ma14175048
De Jager, N., Feilzer, A. J., and Davidson, C. L. (2000). The influence of surface roughness on porcelain strength. Dent. Mater. 16 (6), 381–388. doi:10.1016/s0109-5641(00)00030-0
El Mourad, A. M. (2018). Assessment of bonding effectiveness of adhesive materials to tooth structure using bond strength test methods: a review of literature. Open Dent. J. 12, 664–678. doi:10.2174/1745017901814010664
Erdemir, U., Sancakli, H. S., Sancakli, E., Eren, M. M., Ozel, S., Yucel, T., et al. (2014). Shear bond strength of a new self-adhering flowable composite resin for lithium disilicate-reinforced CAD/CAM ceramic material. J. Adv. Prosthodont. 6 (6), 434. doi:10.4047/jap.2014.6.6.434
Ersu, B., Yuzugullu, B., Yazici, A. R., and Canay, S. (2009). Surface roughness and bond strengths of glass-infiltrated alumina-ceramics prepared using various surface treatments. J. Dent. 37 (11), 848–856. doi:10.1016/j.jdent.2009.06.017
Fathy, H., Hamama, H. H., El-Wassefy, N., and Mahmoud, S. H. (2022). Clinical performance of resin-matrix ceramic partial coverage restorations: a systematic review. Clin. Oral Investig. 26 (5), 3807–3822. doi:10.1007/s00784-022-04449-2
Fidalgo-Pereira, R., Torres, O., Carvalho, Ó., Silva, F. S., Catarino, S. O., Özcan, M., et al. (2023). A scoping review on the polymerization of resin-matrix cements used in restorative dentistry. Mater. (Basel) 16 (4), 1560. doi:10.3390/ma16041560
Fischer, J., Grohmann, P., and Stawarczyk, B. (2008). Effect of zirconia surface treatments on the shear strength of zirconia/veneering ceramic composites. Dent. Mater. J. 27 (3), 448–454. doi:10.4012/dmj.27.448
Foxton, R. M., Cavalcanti, A. N., Nakajima, M., Pilecki, P., Sherriff, M., Melo, L., et al. (2011). Durability of resin cement bond to aluminium oxide and zirconia ceramics after air abrasion and laser treatment. J. Prosthodont. Implant, Esthetic Reconstr. Dent. 20 (2), 84–92. doi:10.1111/j.1532-849x.2010.00678.x
Ghodsi, S., Arzani, S., Shekarian, M., and Aghamohseni, M. (2021). Cement selection criteria for full coverage restorations: a comprehensive review of literature. J. Clin. Exp. Dent. 13 (11), e1154–e1161. doi:10.4317/jced.58671
Hummel, M., and Kern, M. (2004). Durability of the resin bond strength to the alumina ceramic Procera. Dent. Mater. 20 (5), 498–508. doi:10.1016/j.dental.2003.10.014
Ismail, A. M., Bourauel, C., ElBanna, A., and Salah Eldin, T. (2021). Micro versus macro shear bond strength testing of dentin-composite interface using chisel and wireloop loading techniques. Dent. J. (Basel). 9 (12), 140. doi:10.3390/dj9120140
Jayasheel, A., Niranjan, N., Pamidi, H., and Suryakanth, M. B. (2017). Comparative evaluation of shear bond strength of universal dental adhesives -an in vitro study. J. Clin. Exp. Dent. 9 (7), e892–e896. doi:10.4317/jced.53816
Jungbauer, R., Proff, P., Edelhoff, D., and Stawarczyk, B. (2022). Impact of different pretreatments and attachment materials on shear bond strength between monolithic zirconia restorations and metal brackets. Sci. Rep. 12, 8514. doi:10.1038/s41598-022-12542-5
Jurado, C. A., Bora, P. V., Azpiazu-Flores, F. X., Cho, S. H., and Afrashtehfar, K. I. (2023). Effect of resin cement selection on fracture resistance of chairside CAD-CAM lithium disilicate crowns containing virgilite: a comparative in vitro study. J. Prosthet. Dent. (23), S0022–S3913. doi:10.1016/j.prosdent.2023.08.019
Kursoglu, P., Motro, P. F., and Yurdaguven, H. (2013). Shear bond strength of resin cement to an acid etched and a laser irradiated ceramic surface. J. Adv. Prosthodont. 5 (2), 98. doi:10.4047/jap.2013.5.2.98
Levartovsky, S., Bohbot, H., Shem-Tov, K., Brosh, T., and Pilo, R. (2021). Effect of different surface treatments of lithium disilicate on the adhesive properties of resin cements. Mater. (Basel) 14 (12), 3302. doi:10.3390/ma14123302
Li, R., Ma, S. Q., Zang, C. C., Zhang, W. Y., Liu, Z. H., Sun, Y. C., et al. (2019). Enhanced bonding strength between lithium disilicate ceramics and resin cement by multiple surface treatments after thermal cycling. PLoS One 14 (7), e0220466. doi:10.1371/journal.pone.0220466
Limpuangthip, N., Surintanasarn, A., and Vitavaspan, P. (2023). Mechanical and chemical surface treatment enhances bond strength between zirconia and orthodontic brackets: an in vitro study. BDJ Open 9, 53. doi:10.1038/s41405-023-00180-6
Lung, C. Y., and Matinlinna, J. P. (2012). Aspects of silane coupling agents and surface conditioning in dentistry: an overview. Dent. Mater. 28 (5), 467–477. doi:10.1016/j.dental.2012.02.009
Lyann, S. K., Takagaki, T., Nikaido, T., Uo, M., Ikeda, M., Sadr, A., et al. (2018). Effect of different surface treatments on the tensile bond strength to lithium disilicate glass ceramics. J. Adhesive Dent. 20 (3), 261–268. doi:10.3290/j.jad.a40632
Muhammed, H. A., Mahmoud, E. M., Fahmy, A. E., and Nasr, D. M. (2023). The effect of sandblasting versus acid etching on the surface roughness and biaxial flexural strength of CAD/CAM resin-matrix ceramics (in vitro study). BMC Oral Health 23, 169. doi:10.1186/s12903-023-02883-6
Perdigão, J., Araujo, E., Ramos, R. Q., Gomes, G., and Pizzolotto, L. (2021). Adhesive dentistry: current concepts and clinical considerations. J. Esthet. Restor. Dent. 33 (1), 51–68. doi:10.1111/jerd.12692
Phark, J. H., and Duarte, S. (2022). Microstructural considerations for novel lithium disilicate glass ceramics: a review. J. Esthet. Restor. Dent. 34 (1), 92–103. doi:10.1111/jerd.12864
Ramakrishnaiah, R., Alkheraif, A. A., Divakar, D. D., Matinlinna, J. P., and Vallittu, P. K. (2016). The effect of hydrofluoric acid etching duration on the surface micromorphology, roughness, and wettability of dental ceramics. Int. J. Mol. Sci. 17 (6), 822. doi:10.3390/ijms17060822
Sadan, A., Blatz, M. B., and Soignet, D. (2003). Influence of silanization on early bond strength to sandblasted densely sintered alumina. Quintessence Int. 34 (3), 172–176.
Simasetha, S., Klaisiri, A., Sriamporn, T., Sappayatosok, K., and Thamrongananskul, N. (2022). Surface treatment effect on shear bond strength between lithium disilicate glass-ceramic and resin cement. Eur. J. Dent. 16 (2), 373–380. doi:10.1055/s-0041-1735908
Sinha, I. (2023). Adhesive cementation of ceramic restorations: a comprehensive review. INNOSC Theranostics Pharmacol. Sci. 6, 28–34. doi:10.36922/itps.197
Sirisha, K., Rambabu, T., Ravishankar, Y., and Ravikumar, P. (2014). Validity of bond strength tests: a critical review-Part II. J. Conserv. Dent. 17 (5), 420–426. doi:10.4103/0972-0707.139823
Şişmanoğlu, S., Gürcan, A. T., Yıldırım-Bilmez, Z., Turunç-Oğuzman, R., and Gümüştaş, B. (2020). Effect of surface treatments and universal adhesive application on the microshear bond strength of CAD/CAM materials. J. Adv. Prosthodont. 12 (1), 22. doi:10.4047/jap.2020.12.1.22
Tyor, S., Al-Zordk, W., and Sakrana, A. A. (2023). Fracture resistance of monolithic translucent zirconia crown bonded with different self-adhesive resin cement: influence of MDP-containing zirconia primer after aging. BMC Oral Health 23 (1), 636. doi:10.1186/s12903-023-03365-5
Vargas, M. A., Bergeron, C., and Diaz-Arnold, A. (2011). Cementing all-ceramic restorations: recommendations for success. J. Am. Dent. Assoc. 142 (Suppl. 2), 20S–4S. doi:10.14219/jada.archive.2011.0339
Yavuz, T., and Eraslan, O. (2016). The effect of silane applied to glass ceramics on surface structure and bonding strength at different temperatures. J. Adv. Prosthodont 8 (2), 75–84. doi:10.4047/jap.2016.8.2.75
Yavuz, T., Özyılmaz, Ö. Y., Dilber, E., Tobi, E. S., and Kiliç, H. Ş. (2017). Effect of different surface treatments on porcelain-resin bond strength. J. Prosthodont. 26 (5), 446–454. doi:10.1111/jopr.12387
Zarone, F., Di Mauro, M. I., Ausiello, P., Ruggiero, G., and Sorrentino, R. (2019). Current status on lithium disilicate and zirconia: a narrative review. BMC Oral Health 19 (1), 134. doi:10.1186/s12903-019-0838-x
Keywords: dental ceramics, lithium disilicate, laser treatment, airborne particle abrasion, silica coating, cement bonding
Citation: Alrabeah G, Alhamid RF, Alamer BA, Alrajhi FN, Binrayes A and Habib SR (2024) Impact of various surface treatments on the shear bond strength between lithium disilicate ceramics and resin cement. Front. Mater. 11:1496749. doi: 10.3389/fmats.2024.1496749
Received: 15 September 2024; Accepted: 15 November 2024;
Published: 28 November 2024.
Edited by:
Ken Lee, National Aeronautics and Space Administration (NASA), United StatesReviewed by:
Rafael Mello Trommer, National Institute of Metrology, BrazilEvangelia Vouvoudi, Aristotle University of Thessaloniki, Greece
Copyright © 2024 Alrabeah, Alhamid, Alamer, Alrajhi, Binrayes and Habib. This is an open-access article distributed under the terms of the Creative Commons Attribution License (CC BY). The use, distribution or reproduction in other forums is permitted, provided the original author(s) and the copyright owner(s) are credited and that the original publication in this journal is cited, in accordance with accepted academic practice. No use, distribution or reproduction is permitted which does not comply with these terms.
*Correspondence: Syed Rashid Habib, c3loYWJpYkBrc3UuZWR1LnNh