- 1College of Civil Engineering, Tongji University, Shanghai, China
- 2China West Construction Group Co., Ltd., China State Construction Engineering Corporation Ltd, Chengdu, China
- 3Linyi Lantai Environmental Protection Technology Co., Ltd, Linyi, China
In the pursuit of sustainable construction practices, the utilization of recycled concrete has emerged as a pivotal strategy, distinguished by its commitment to resource conservation and environmental stewardship. Nevertheless, the inherent micro-porosity and micro-cracking within the old mortar of recycled concrete may lead to weak bonding performance at the interfacial transition zone, culminating in diminished strength, reduced density, and elevated water absorption rates compared to conventional concrete, which critically impairs its performance in cold climates subjected to freeze-thaw cycles. Consequently, this paper provides a structured examination of the frost resistance properties of recycled concrete subjected to freeze-thaw cycling. Initially, the study delineates the mechanisms of frost-induced damage in recycled concrete by synthesizing the degradation pathways observed in both conventional and recycled concrete during freeze-thaw exposure. Subsequently, a detailed analysis is conducted to identify the pivotal factors affecting frost resistance, encompassing the proportion and moisture affinity of recycled aggregates, the addition of silica fume and fly ash, the water-to-cement ratio, and the degree of water saturation. In the final segment, the study compiles and reviews the strategies for bolstering the frost resistance of recycled concrete, including the incorporation of air-entraining admixtures, fiber reinforcement, and aggregate modification approaches. The objective of this research is to offer a thorough comprehension of recycled concrete, with a concentration on the mechanisms of frost damage, the critical determinants of frost resistance, and interventions to augment its resilience against freezing conditions. On this basis, the present paper, in conjunction with the characteristics and current research status of recycled concrete, proposes recommendations for the application of recycled concrete in cold regions. This review is anticipated to facilitate researchers in gaining a comprehensive understanding of the freeze-thaw characteristics of recycled concrete and the measures to enhance its frost resistance. Furthermore, it aims to assist engineering and technical personnel in selecting appropriate treatment methods to improve the frost resistance of recycled concrete in cold regions, thereby promoting the practical engineering application of recycled concrete in such areas.
1 Introduction
Concrete is primarily composed of aggregates, binder materials, water, and admixtures. It possesses excellent processing and mechanical properties, which have led to its widespread application in the field of construction. Aggregates are the primary component of concrete, accounting for 60%–80% of the total volume and 70%–85% of the total weight (Muhammad et al., 2024). Traditional concrete heavily relies on the consumption of natural sand and stone, which are non-renewable resources, and this has caused some harm to the natural environment. Previous studies have shown that waste concrete and waste clay bricks constitute the largest volume of construction waste generated during new construction, expansion, reconstruction, and demolition, accounting for 30%–40% of urban waste (Chen et al., 2007). Therefore, utilizing recycled and reprocessed waste materials such as waste concrete (Chandru et al., 2023; Yao et al., 2023), bricks (Chen et al., 2024; Wang et al., 2024), and stone (Shen et al., 2022) aggregates for producing concrete holds great significance for sustainable development.
Frost resistance is a key indicator for assessing its long-term durability in cold environments. However, limitations in disposal technology often result in recycled aggregates having a high amount of attached mortar and microcracks generated during the crushing process. This leads to increased porosity and poorer aggregate quality in recycled concrete (Muhammad et al., 2024; Ren et al., 2024; Tam et al., 2005; Wang et al., 2021; Wang et al., 2014). Furthermore, the interface transition zone (ITZ) in recycled concrete is intricate and less robust compared to that in natural aggregate concrete, making it prone to performance degradation and damage during freeze-thaw cycles (Liu et al., 2019; Sáez Del Bosque et al., 2017; Xiao et al., 2013). As well as, many factors, including the content and water absorption rate of recycled aggregates (Datta et al., 2022; Li T et al., 2024), mineral admixtures (Gunasekara et al., 2020; Li et al., 2012a; Song et al., 2018; Wang et al., 2023a), water-to-cement ratio (Sosa et al., 2021), and water saturation (Luan et al., 2020), can influence its frost resistance. Up to now, scholars have conducted numerous experimental studies and evaluated the frost resistance of these key factors (Guan et al., 2024). Therefore, summarizing the frost damage mechanisms, analyzing its degradation process, and examining how frost resistance indicators change with the number of freeze-thaw cycles can provide valuable insights for researching and applying frost resistance in recycled concrete.
It is crucial to ensure the mechanical performance of recycled concrete in cold environments. Adding air-entraining agents to recycled concrete is an effective method to improve its freeze-thaw resistance (Deng et al., 2021a; Deng et al., 2021b). A large number of uniformly distributed microbubbles are introduced into the concrete by adding an air-entraining agent. These bubbles provide space for the water to expand during freezing, thereby reducing internal stress concentration and the formation of microcracks. Furthermore, the incorporation of fibers can form a three-dimensional network in the concrete, enhancing the crack resistance and spalling resistance of recycled concrete (Guan et al., 2024; Liu et al., 2023; Zhang et al., 2023). Previous studies (Deng et al., 2023; Islam et al., 2022) have shown that the different types of fiber materials have varying effects on improving the frost resistance of recycled concrete. Polypropylene fibers have good flexibility and dispersibility, reducing the formation of microcracks in recycled concrete (Yildizel et al., 2024). However, polypropylene fibers have relatively low strength and modulus, which limits their ability to enhance the frost resistance of concrete. Moreover, steel fibers, with higher strength and toughness, can effectively restrain crack propagation but may increase concrete conductivity. Therefore, selecting the appropriate fiber type based on specific operational conditions is essential. Coating protective agents on recycled aggregates and using chemical adhesives can enhance bond strength between recycled aggregates and fresh concrete, thereby reducing damage during freeze-thaw cycles (Xia et al., 2023; Zheng et al., 2024). However, these methods increase construction costs and require specialized equipment and skills. Therefore, it is necessary to select appropriate modification methods based on engineering conditions to enhance the freeze-thaw resistance of recycled concrete.
This revie summarized the freeze-thaw degradation mechanisms of recycled concrete and discusses the impact of the ITZ on the frost resistance. Subsequently, the key factors, including recycled aggregates, silica fume, water-to-cement ratio, and fly ash affecting the frost resistance are reviewed. Then, this study summarized some improvement techniques (air-entraining, reinforcement fibers, and aggregate modification) to enhance the frost resistance of recycled concrete. This study provides valuable references for improving the durability of recycled concrete structures in cold environments. This will assist researchers and engineering professionals in gaining a comprehensive understanding of the frost resistance of recycled concrete, thereby promoting further in-depth research on the frost resistance of recycled concrete and facilitating its wider application in cold regions.
2 Deterioration mechanisms
2.1 Normal concrete subjected to freeze-thaw cycles
Due to factors such as pore structure and aggregate quality, the material damage after freeze-thaw cycles is extremely complex (Ann et al., 2017; Guo et al., 2022; Kobayashi et al., 2022; Lee et al., 2018; Li Y et al., 2024; Liu and Hansen, 2016). The freeze-thaw damage process does not alter the composition of concrete; thus, its occurrence is primarily due to physical changes (Guo et al., 2022). Currently, the damage mechanisms under freeze-thaw cycles in concrete mainly focused on four representative theories, including the hydraulic pressure theory (Powers, 1945), the osmotic pressure theory (Powers and Helmuth, 1953), the crystallization pressure theory (Scherer, 1999), and the micro-ice crystal model (Setzer, 2001a; Setzer, 2001b). Figure 1 summarizes the principles of these degradation mechanisms, respectively. Among them, the hydraulic and osmotic pressure theories proposed that when water entered the pores of concrete and froze, the volume expansion generated hydrostatic pressure, and the growth of ice crystals led to osmotic pressure, resulting in micro-cracks and damage within the concrete (Gong and Jacobsen, 2019; Wang et al., 2023b). The crystallization pressure theory explains the mechanism of cumulative damage in concrete by detailing the crystallization and pressurization during freezing and the increased water absorption rate during thawing (Liu et al., 2018). The micro-ice crystal theory suggests that the concrete matrix needs to provide additional crystallization pressure to balance the pressure generated by the curvature difference between crystal surfaces and ends (Yan et al., 2014).
2.2 Recycled concrete subjected to freeze-thaw cycles
The internal structure of recycled concrete differs from that of natural concrete, leading to variations in their freeze-thaw damage mechanisms (Luan et al., 2020; Luan et al., 2021). When the moisture content within concrete reaches its critical saturation point, the concrete begins to experience freeze-thaw damage and deterioration. Similarly, the freeze-thaw damage of recycled concrete is also influenced by its sensitivity to internal moisture (Li et al., 2012b; Yang et al., 2024; Yao et al., 2022). Figure 2 illustrates the interface structure inside natural and recycled concrete. Previous studies (Rong et al., 2023; Zhao et al., 2023) have shown that recycled concrete has more complex interface structure, dividing new mortar-mortar interface (ITZ1), old aggregate-new mortar interface (ITZ2), and old mortar-old aggregate interface (ITZ3), respectively. These ITZs exhibit poor performance, resulting increased water absorption and pore structure in recycled concrete (Xiao et al., 2013). Previous studies (Peled et al., 2010; Xiao et al., 2022) have demonstrated that ITZ exhibited characteristics of crystalline looseness and high porosity, especially ITZ1, with its indentation modulus being 10%–20% lower than that of the surrounding mortar, and its width being 15 μm wider than ITZ2 and ITZ3. Therefore, microcracks generated in recycled concrete under freeze-thaw cycles occurred first in weak ITZ and continued to expand, resulting in poor frost resistance (Meng et al., 2024).
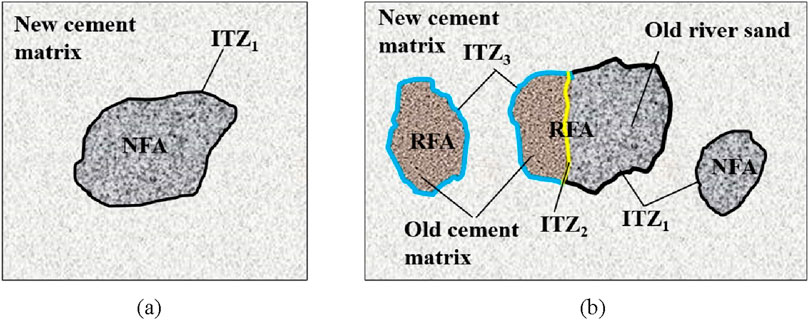
Figure 2. Schematic diagram of interface structure for NC and recycled concrete: (A) NC, (B) recycled concrete (Zhang et al., 2018).
Researchers have focused extensively on studying the influence mechanisms of interface structures in recycled concrete on its frost resistance. Most researchers believe that the high-water absorption caused by poor interface quality in recycled concrete is one of the reasons for freeze-thaw damage (Gao et al., 2018; Qiu et al., 2024; Tuyan et al., 2014; Wang et al., 2023c). This is because in the raw materials, old mortar adheres to the surface of recycled aggregates, leading to higher porosity and the generation of microcracks during the crushing process of recycled aggregates (Alabduljabbar et al., 2024). The interface images were observed using a scanning electron microscope by Liu et al. (2019), demonstrating that there were more weak interfaces, microcracks, and pores in the ITZ of recycled concrete. Zheng et al. (2021) demonstrated that when the water-to-cement ratio was high, there were more microcracks in the ITZ around recycled aggregates. This resulted in increased pore water absorption rate and decreased concrete strength. Lin et al. (2023a) found that in recycled concrete, a higher ratio of old mortar-water cement lead to increased porosity, pore size, crystallization pressure, principal stress, and the lower its frost resistance. In addition, scholars have revealed the freeze-thaw damage mechanism from the perspective of ITZs. Hasholt (2014) found that recycled concrete contained multiple ITZs, generating numerous micro-pores within its interior. During freeze-thaw cycles, smaller pore sizes resulted in faster water absorption rates, increasing the internal osmotic pressure of recycled concrete. Similarly, Xiao et al. (2022) and Zhu et al. (2020) demonstrated that the higher the recycled aggregates content, the higher the porosity and water absorption rate. Luan et al. (2020) found that the critical water saturation was 81%–83% in recycled concrete, which was lower than the critical water saturation range of natural concrete (86%–88%). Therefore, recycled concrete is more prone to freeze-thaw failure than that of natural concrete. In conclusion, scholars generally concur that the high-water absorption rate of recycled aggregates subject the internal small pores in recycled concrete to considerable pressure during the freezing and thawing process. As freeze-thaw cycles increases, the total porosity and micro-cracks between pores increase or expand, establishing channels for the infiltration of external water into the concrete interior. Under high pressure, the weak interface zone of the recycled concrete generates microcracks with the continuous formation and expansion of internal pores, which continuously develop and propagate, ultimately resulting in the loosening of overall internal structures. When the damage accumulates to a certain extent, freeze-thaw damage occurs.
In conclusion, recycled concrete demonstrates a higher incidence of weak ITZs compared to natural concrete, which consequently results in an inferior frost resistance performance. It involved various degradation mechanisms associated with the micro-pore structures in recycled concrete. Therefore, further research is needed to reveal the influence of the ITZ on the mechanism of freeze-thaw damage.
3 Critical influence factors for frost resistance
3.1 Recycled aggregates
3.1.1 Content of recycled aggregates
Generally, the frost resistance of recycled concrete is influenced by the content of recycled aggregates (Bogas et al., 2016; Tran et al., 2022; Wang et al., 2023d; Wang et al., 2023e). Researchers have focused on the recycled fine aggregate (RFA) and conducted corresponding experimental studies. The frost resistance tests were conducted by (Sun and Geng, 2012), and indicated that when the content of RFAs content exceeded 40%, the relative dynamic elastic modulus (RDEM) of the recycled concrete showed a significant decrease, and with the increase in RFAs content, the RDEM decreased more significantly. Bao et al. (2022) examined the frost resistance with varying replacement rates of RFAs. The results showed that the frost resistance was primarily related to the RFAs, showing an initial increase followed by a decrease as the ferronickel slag content increased. When 40% of recycled ferronickel slag fine aggregates were incorporated, the recycled concrete exhibited the best frost resistance, as shown in Figure 3. This is mainly due to the hydration reaction of the active substances in the ferronickel slag, which formed more hydration products and strengthened the ITZ structure within the recycled concrete. Similarly, Saha and Sarker (2020) demonstrated that when ferronickel slag replaced 40% of river sand, the recycled concrete produced more cementitious products, thereby improving its microstructure. The research results of Quan et al. (2022) indicated that the mass loss rates corresponding to molybdenum tailings replacement rates of 0%, 30%, 50%, 70%, and 100% were 0.62%, 0.81%, 0.83%, 0.9%, 1.01%, and 1.11% after 100 freeze-thaw cycles, respectively.
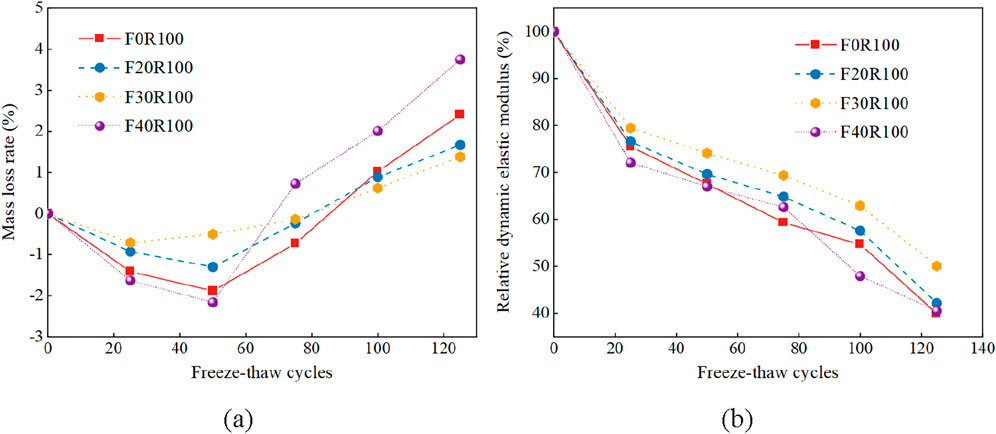
Figure 3. The influence of ferronickel slag content on the mass loss rate and RDEM: (A) mass loss rate, (B) RDEM. Note: F represents nickel-iron slag content; R denotes the recycled coarse aggregate content.
Recycled coarse aggregates (RCAs) exhibited higher porosity and greater absorption capacity compared to RFAs, making them more susceptible to deterioration. For instance, Tuyan et al. (2014) demonstrated that the frost resistance of concrete with crushed limestone recycled aggregates can be significantly decreased. Amorim et al. (2018) observed that the RDEM loss rate of RCA concrete with a 50% content was lower compared to those with 25% and 75% content after 150 freeze-thaw cycles. Additionally, Ji and Wang (2022) found that recycled brick aggregate concrete, with NCA replaced, developed cracks in the ITZ and reduced adhesion between cement mortar and RCAs after 100 freeze-thaw cycles. The substitution of RCAs for NCAs has a negative impact on the frost resistance. Deng et al. (2021) studied the loss rate of flexural strength of RCA concrete after different freeze-thaw cycles and with varying RCA contents. The results showed that the flexural strength loss rate increases with RCA contents, reaching up to 70%, as shown in Figure 4. In conclusion, when using RCAs to replace NCAs, the replacement rate should not exceed 50%.
3.1.2 Porosity and absorption of recycled aggregates
The old mortar in recycled aggregate and microcracks may increase water absorption and porosity, thereby reducing frost resistance. Studies have demonstrated that the water absorption of recycled aggregates are approximately 6–10 times that of natural aggregates (Levy and Helene, 2004). According to (Omary et al., 2016), as the water absorption and porosity increase, the strength and density of recycled aggregates decrease. Consequently, the freeze-thaw resistance and splitting tensile strength of recycled aggregate concrete are notably lower than those of natural aggregate concrete. This is because recycled aggregates retain old mortar and develop micro-cracks. Moreover, RFAs exhibited higher porosity compared to RCAs, likely due to more old cement mortar in RFAs. Xia and Zhao (2024) found that the frost damage in recycled concrete was primarily caused by the defects of recycled aggregates. This is because the old mortar contains numerous tiny and loose pore structures with high-water absorption, resulting in micro-cracks propagation and new micro-cracks generation in the new mortar under frost heave forces. Gokce et al. (2004) found that microcracks in recycled concrete first concentrated on the old mortar adhered to the RCA after absorbing water. Subsequently, these cracks rapidly developed and penetrated the new mortar, ultimately leading to freeze-thaw failure. Literature (Ghorbani et al., 2019; Peceño et al., 2019; Wei et al., 2022) indicated that large-sized RCAs were difficult to disperse uniformly in concrete, and increased the porosity of RCA concrete, reducing the frost resistance. Figure 5 illustrates the development of the ITZ in RCA concrete during freeze-thaw cycles. It can be seen that ITZ became fragmented and porous after 300 freeze-thaw cycles, as shown in Figure 5A. The width of the ITZ increased as microcracks gradually expanded and connected with the pores, forming cross-cracks after 400 freeze-thaw cycles, as shown in Figure 5C. In conclusion, frost resistance can be influenced by factors such as water absorption, porosity, and particle size. Therefore, the next step in research should focus on developing effective modification methods for recycled aggregates to enhance the frost resistance.
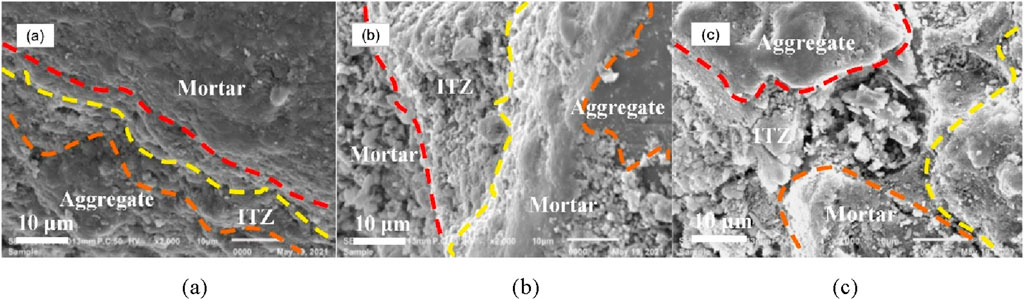
Figure 5. ITZ SEM images of RCA concrete at different freeze-thaw cycles: (A) 0 cycles, (B) 350 cycles, (C) 400 cycles (Wei et al., 2022).
3.2 Mineral admixture
The frost resistance could be improved by incorporating mineral admixtures, including silica fume (Avet et al., 2019; Bu et al., 2022), fly ash (Şimşek et al., 2022; Yu et al., 2021; Yuan et al., 2024), slag (El-Hawary et al., 2021; Lee et al., 2021; Li et al., 2019), and rice husk ash (Ma et al., 2024; Wang et al., 2017; Zhang et al., 2022). On the one hand, it is possible to fill the micro-pores within recycled concrete, increasing its density. On the other hand, the active components of mineral admixtures could promote secondary hydration reactions in recycled concrete, thereby enhancing its frost resistance (Cheng et al., 2018; Mardani-Aghabaglou et al., 2019).
3.2.1 Silica fume
Silica fume (SF) is a finely-grained volcanic ash material. It enhances the durability of concrete by filling internal pores, increasing density, and reducing water absorption. Incorporating the right amount of SF enhances cement hydration and boosts the density of the ITZ in recycled concrete (Oertel et al., 2014; Scrivener et al., 2015). Bai et al. (2023a) investigated the fracture properties of recycled concrete after partially replacing cement with SF. After 125 freeze-thaw cycles, the fracture damage parameters were 0.256 and 0.247 for SF replacement rates of 0% and 9%, respectively. The fracture damage parameter was relatively small when the silica fume content was 9%, indicating a reduction in microcrack density and improved frost resistance after the addition of SF. Wang et al. (2023) found that after 400 freeze-thaw cycles, recycled concrete with 3%, 5%, 8%, 11%, 13%, and 16% SF content showed 16%, 20.0%, 28.0%, 36%, 40%, and 32% increase in splitting tensile strength compared with recycled concrete with 0% SF content, respectively. It can be seen that excessive addition of SF will weaken its effect on improving frost resistance. This is because unhydrated SF particles may cause shrinkage cracks and insert into the hydration products, resulting in a decrease in the bonding strength of the mortar, as shown in Figure 6.
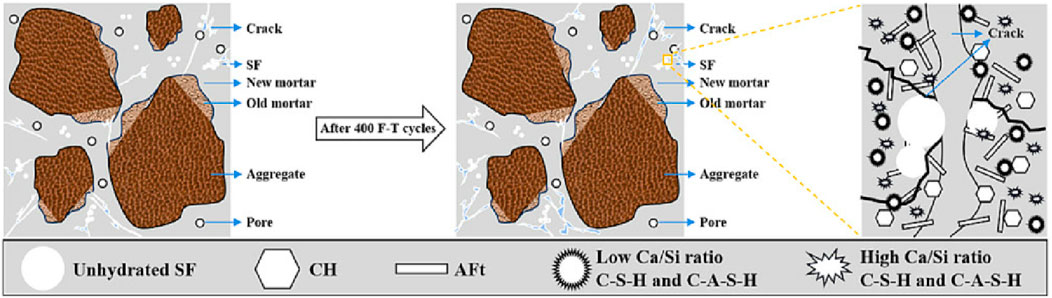
Figure 6. Schematic diagram of the mechanism of excessive silica fume under freeze-thaw cycles (Wang et al., 2023c).
3.2.2 Fly ash
Fly ash is a fine, greyish-white powder rich in silicate and aluminate minerals. Incorporating fly ash into concrete could improve its density (Posi et al., 2016), strength (Lam, 2020), and durability (Corinaldesi, 2012), while also lowering costs and environmental impact. Previous studies have demonstrated that incorporating fly ash into recycled concrete could improve microstructures (Sunayana and Barai, 2021), fill and reduce pores, increase compactness, and delay hydration reactions (Yang et al., 2024), thereby enhancing the frost resistance. Yu et al. (2021) utilized discarded concrete and clay bricks as raw materials, blending them in a 7:3 ratio to produce recycled brick-concrete aggregate, and investigated the frost resistance incorporating both recycled brick-concrete aggregate and fly ash under conditions of freeze-thaw cycling and combined action with sodium chloride. The experimental outcomes indicated that incorporating 15% fly ash content could notably enhance the compressive strength of the recycled concrete, frost resistance, and resistance to chloride-induced synergistic erosion. Nazeer et al. (2024) observed that fly ash would cause a positive effect on the frost resistance. Their findings indicate that using 10% fly ash in recycled concrete leads to a noticeable decrease in the rates of compressive strength loss and mass loss, as depicted in Figure 7. Similarly, LI et al. (2017a) study the influence of fly ash dosage on the frost resistance by substituting cement with fly ash at equal weight percentages of 0%, 20%, and 40%, respectively, to prepare RCA concrete specimens. The tested results demonstrated that the frost resistance was enhanced by incorporating fly ash into RCA concrete. Moreover, the additional pores provided by the RCA in the paste contributed to this enhancement to some extent. Therefore, the utilization of an appropriate amount of fly ash can reduce the loss of RDEM loss and compressive strength in RCA concrete.
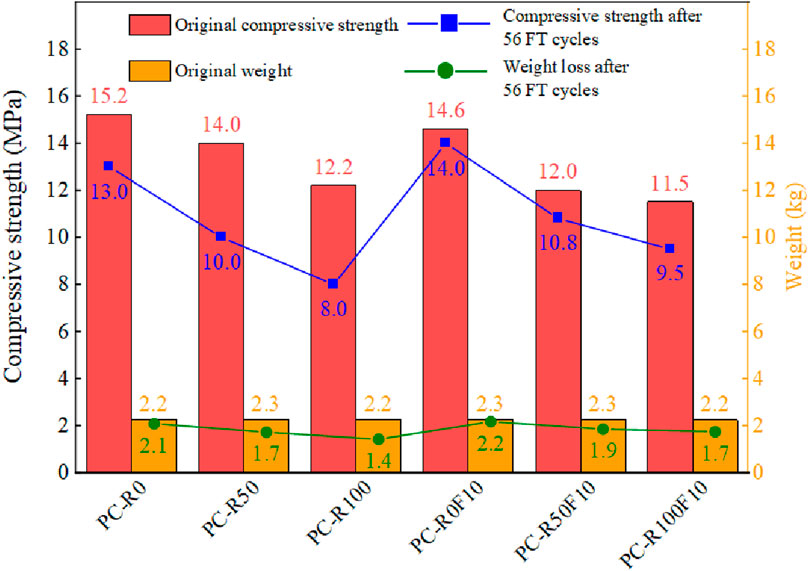
Figure 7. The influence of fly ash on the frost resistance. Note: PC signifies cement, R denotes the recycled aggregate content, and F indicates the fly ash content.
Some scholars argue that adding fly ash will reduce its frost resistance performance of recycled concrete. Bu et al. (2023) studied the microstructure, mechanical properties, flexural toughness, and frost resistance with partial fly ash content, including 0%, 10%, 30%, 50%, and 70% in cement. The findings showed that as fly ash content increased, the mass loss settlement initially decreased and then increased. Conversely, the RDEM loss initially increased and then decreased. Completing 100 freeze-thaw cycles, recycled concrete with a 50% fly ash content exhibited the best mechanical properties, with a significant decrease in mechanical properties at higher or lower replacement rates. Similarly, Cheng et al. (2017) found that the RDEM of recycled concrete specimens without fly ash decreased slowly after 300 freeze-thaw cycles. However, specimens incorporating 10% and 30% fly ash experienced a marked acceleration in RDEM reduction rates after 200 freeze-thaw cycles, whereas those with 50% fly ash showed a pronounced increase in RDEM reduction rates following 150 freeze-thaw cycles, as detailed in Figure 8. Furthermore, Wu et al. (2019) also founded that the incorporation of fly ash resulted a negative impact on the frost resistance.
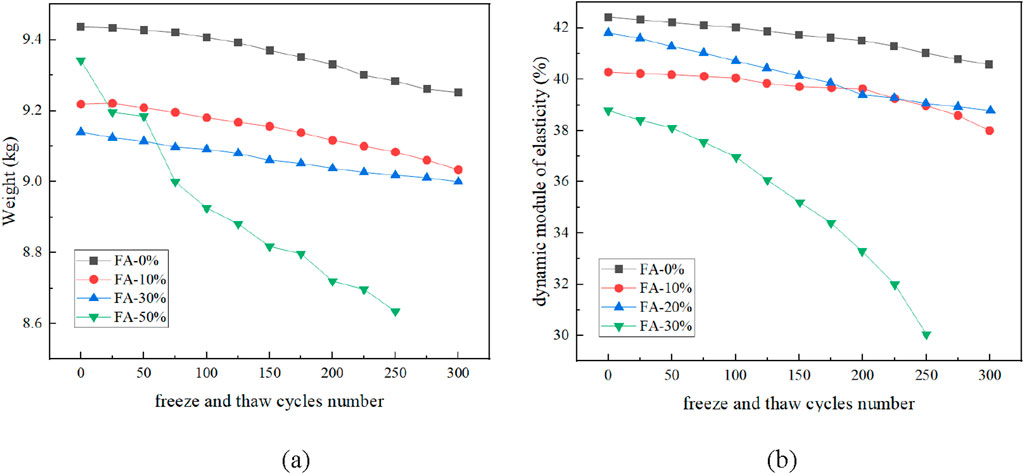
Figure 8. The influence of fly ash content on the frost resistance: (A) effects of freeze-thaw cycles on weight; (B) effects of freeze-thaw cycles on dynamic modulus of elasticity.
In summary, the amount of fly ash incorporation needs to be determined based on specific engineering requirements and the quality of the fly ash. Overusing or underusing fly ash in concrete may negatively impact its performance.
3.2.3 Multiple mineral admixtures
Some researchers have utilized a combination of various mineral admixtures to develop recycled concrete, aiming to explore the effects of composite mineral admixtures on its frost resistance. For instance, Chen et al. (2023) conducted freeze-thaw cycling tests by integrating fly ash (FA) and ground granulated blast furnace slag (GGBS) individually or simultaneously into concrete containing 0%, 35%, and 50% RCAs, replacing part of the cement. The results indicate that the hydration product Ca(OH)₂ effectively activated the pozzolanic reactions between fly ash and ground granulated blast furnace slag. Fly ash and ground granulated blast furnace slag act as catalysts in recycled concrete, accelerating the formation of C-S-H gel and enhancing freeze-thaw resistance. Consequently, while RCAs may reduce the frost resistance, incorporating ground granulated blast furnace slag and fly ash as partial substitutes for cement can help alleviate the adverse effects of RCAs on frost resistance. In particular, using fly ash and ground granulated blast furnace slag together as partial replacements for cement significantly enhances the frost resistance of RCA concrete. The synergistic effect of ground granulated blast furnace slag and fly ash occurs at a ratio of 15% by weight, as detailed in Figure 9. Cheng et al. (2024) selected fly ash, silica fume, and granulated blast furnace slag to prepare five distinct cementitious systems of recycled concrete through various combinations of quantities. The experimental outcomes revealed that a superimposed effect was formed due to the full utilization of the morphological effects and micro-filler effects of silica fume, fly ash, and granulated blast furnace slag within the concrete matrix. This synergistic interaction reduced the porosity of recycled concrete, effectively hindering the free water into its interior, and consequently enhancing the frost resistance. Moreover, mineral admixtures can improve the internal structure of recycled concrete and mitigate the damage caused by pore water pressure expansion. The research results of Guo et al. (2020) indicate that self-compacting concrete blended with fly ash, slag, silica fume, and recycled aggregates showed a strength loss similar to that of normal self-compacting concrete.
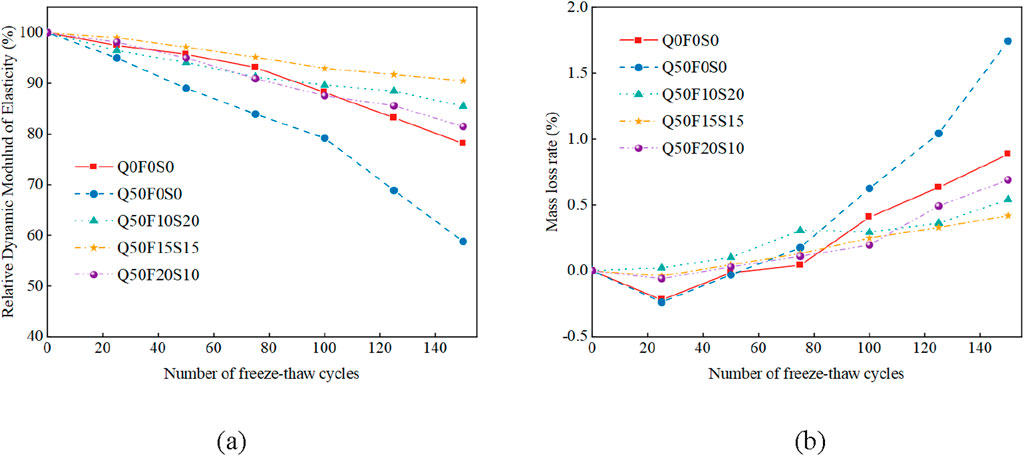
Figure 9. Synergistic effects of FA and GGBS on the mass loss rate and REDM of recycled concrete: (A) RDEM loss rate; (B) Mass loss rate. Note: Q represents the of RCA content; F and S represents FA and GGBS, respectively.
In general, the concurrent use of multiple mineral admixtures in concrete production enhances the performance of recycled concrete more effectively than using single mineral admixtures alone. In other words, the selection of a combination of various mineral admixtures and the determination of appropriate mixing ratios can significantly enhance the frost resistance of recycled concrete.
3.3 Water-to-cement ratio
The water-to-cement ratio is a crucial factor affecting the frost resistance of recycled concrete. Scholars have conducted experimental studies on the frost resistance of recycled concrete under different water-to-cement ratios. Bogas et al. (2016) carried out rapid freeze-thaw tests by partially or entirely replacing natural fine aggregates (NFAs) with RFAs. The results indicated that the frost resistance of recycled concrete primarily depended on the water-to-cement ratio, with lower ratios correlating to better performance. Atasham Ul Haq et al. (2024) utilized recycled brick aggregate to produce recycled concrete specimens with water-to-cement ratios of 0.65, 0.5, and 0.35, with replacement levels of 0%, 25%, 50%, and 100%. The results showed the recycled concrete made with a water-to-cement ratio of 0.65 exhibited a significantly higher mass loss rate than those with ratios of 0.5 and 0.35. Luo et al. (2015) showed that reducing the water-to-cement ratio led to a denser concrete matrix and reduced the mass loss rate.
Increasing the water-to-cement ratio increases the number and volume of capillary pores in concrete, allowing more water to freeze within the cement paste. Therefore, reducing the water-to-cement ratio of recycled concrete is beneficial for improving its frost resistance. This effect is particularly pronounced in specimens with higher water-to-cement ratios and greater contents of recycled aggregates (Tuyan et al., 2014). Yildirim et al. (2015) designed three levels of recycled aggregate saturation (0%, 50%, and 100%) and three water-to-cement ratios (0.7, 0.6, and 0.5), while controlling the proportion of recycled aggregates at different levels, to study the durability of recycled concrete. The tested results indicated that the mass loss rate of all specimens decreased with the decrease in water-to-cement ratio after 300 freeze-thaw cycles. Likewise, Meng et al. (2021) demonstrated that recycled concrete with high RCA content exhibits poor frost resistance. However, by reducing the water-to-cement ratio, the RDEM of the specimens rapidly decreased at the end of the respective experiments. This indicates that reducing the water-to-cement ratio improves the frost resistance of recycled concrete to some extent. The RDEM results for the concrete specimens are presented in Figure 10.
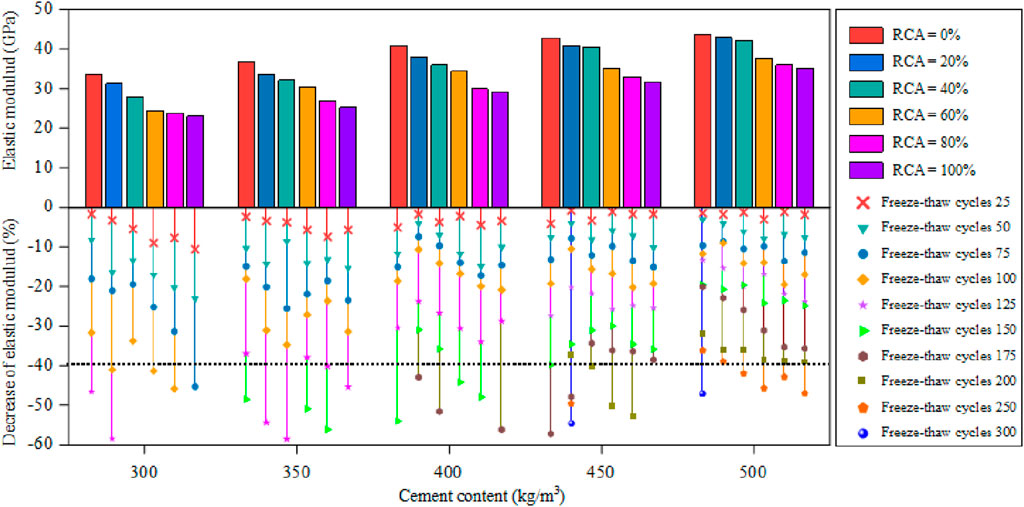
Figure 10. Decrease of elastic modulus under different recycled coarse aggregate and freeze-thaw cycles (Yuan et al., 2023).
3.4 Water saturation of recycled aggregates
The water saturation of recycled aggregates could affect the pore structure, reducing the frost resistance of recycled concrete. As a porous medium, recycled aggregates have a high-water absorption rate (Khoury et al., 2019). Pretreating recycled aggregates with water will result in a certain degree of internal curing effect in recycled concrete. Previous studies (Marchi et al., 2023; Pickel et al., 2017; Yang et al., 2022) have shown that internal curing can reduce water consumption, enhance the degree of hydration in concrete, reduce cracks, and ensure the long-term stability of concrete structures.
Scholars have studied the effect of water saturation of recycled aggregates on the internal curing efficiency of recycled concrete. Deng et al. (2021) indicated that the incorporation of RCAs significantly affected the distribution and quality of different pores. Prior to the commencement of freeze-thaw cycles, due to the higher porosity and water absorption rate of recycled aggregates, pre-wetting provided a certain degree of internal curing for the concrete by supplying water within the aggregate pores. Consequently, recycled aggregate concrete exhibited higher flexural strength compared to natural aggregate concrete. Similarly, de Oliveira and Vazquez (1996) investigated the effect of water saturation on the mechanical properties and durability of recycled concrete. The results showed that recycled concrete made with saturated and dried recycled aggregates exhibited poor frost resistance. Conversely, recycled concrete containing semi-saturated recycled aggregates exhibited better frost resistance, attributed to a denser and stronger interface transition zone. Specifically, the semi-saturated recycled aggregates provided sufficient moisture for internal curing, promoting further hydration of the ITZ and thereby enhancing the frost resistance. Yildirim et al. (2015) found that during the curing process of concrete, water stored in the pores of porous aggregates is gradually released, promoting the hydration of cement. Therefore, achieving an optimal water saturation level for recycled aggregates can improve the frost resistance to some extent. Similarly, El-Hawary and Al-Sulily (2020) have found that recycled concrete specimens containing 50% pre-moistened RCAs exhibit improved frost resistance compared to mixtures without recycled aggregate. In addition, specimens with 50% pre-moistened RCAs and RFAs showed a significant increase in RDEM, indicating that the internal curing provided by pre-moistened recycled aggregates effectively improves the frost resistance. Furthermore, the optimal water saturation of recycled aggregates can still exert their internal curing function in complex environmental conditions, enhancing the frost resistance. For instance, Lei et al. (2018) investigated the effects of mechanical repetitive loading and its interaction with complex environments on the performance of recycled aggregate concrete. The results indicated that pre-saturated recycled aggregates facilitated the hydration of new and old mortar adhered to the recycled aggregates at the ITZ during the curing stage. Figure 11 illustrates variation of RDEM of pre-saturated recycled aggregate concrete with freeze-thaw cycles. In this figure, A represents not recycled aggregate, B represents 50% RCAs and RFAs content, C represents 50% RFAs content, and D represents 50% RCAs content. The numeral “1” represents a water-cement ratio of 0.4, and “75%” indicates that the ambient humidity was 75%. The figure shows that pre-moistened recycled aggregates enhance the frost resistance of concrete, indicating the effectiveness of their internal curing mechanism.
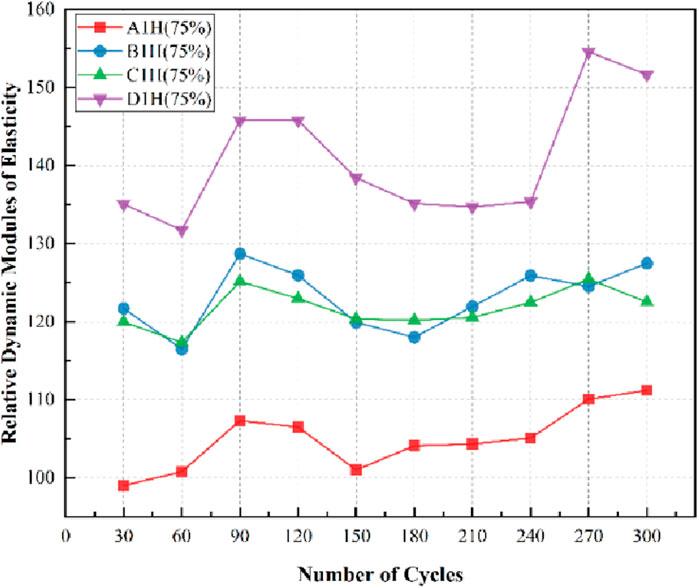
Figure 11. Impact of water saturation of recycled aggregates on frost resistance of recycled concrete.
In summary, recycled aggregates, as a type of porous material with high porosity and strong water absorption capacity, when used at an appropriate water saturation level for preparing fresh mixed concrete, will gradually release moisture during the curing process of the concrete. This moisture release provides a certain level of internal curing within the concrete structure, thereby promoting cement hydration and improving the ITZ of the concrete structure.
4 Improvement techniques for frost resistance of recycled concrete
4.1 Incorporating air-entraining agent
The use of air-entraining admixtures can enhance the workability, internal cohesion, and plasticity of concrete, and reduce segregation and water loss, particularly in terms of frost resistance (Huaishuai et al., 2017; Li and Qin, 2013; Long et al., 2013). Incorporating air-entraining admixtures is considered the most effective method for enhancing concrete’s frost resistance at a fixed water-to-cement ratio. This is because when recycled concrete is mixed with air-entraining admixtures, numerous small, closed spherical air bubbles can be formed within the concrete (Liu et al., 2011). During the freeze-thaw cycle, air bubbles were absorbed and some water was released, alleviating the hydrostatic pressure and osmotic stress on the concrete, thereby reducing the extent of damage caused by ice crystal expansion.
Liu et al. (2016) investigated the frost resistance made with non-aerated and aerated recycled aggregates concrete. The results showed that the strength loss of recycled concrete containing both aerated and non-aerated aggregates was intermediate between that of concrete with only aerated aggregates and that with only non-aerated aggregates. Tarhan and Şahin (2021) investigated the scaling behavior of recycled concrete incorporating different air-entraining admixtures (0.06%, 0.13%, 0.20%) and recycled aggregate content. The results showed that in the absence of any recycled aggregates, the control group with 0.13% air-entraining admixture had the highest scaling rate. Among the mixtures with the same percentage of recycled aggregates, the mixture containing 0.06% air-entraining admixture typically exhibited the least scaling and the weakest signs of deterioration. These results indicate that the pores generated by the air-entraining admixture assist in reducing the pressure buildup caused by the expansion of liquid water and ice crystals. Furthermore, the spherical air void structure formed after incorporating air-entraining admixture is an important reason for the impact on the frost resistance. Deng et al. (2021) found that the addition of air-entraining admixtures had little effect on the mass loss rate of recycled concrete but helped to increase its RDEM. Similarly, Olanike (2014) found that air-entraining admixtures played a significant role in preventing the RDEM of recycled concrete from decreasing after freeze-thaw cycles. In addition, Zhang et al. (2024) found that the mass loss rates of recycled concrete with a 50% recycled aggregate content were 1.31%, 1.18%, and 2.36% after 200 freeze-thaw cycles when 0.01%, 0.03%, and 0.05% air-entraining agents (MR50-A1/A3/A5) were added, as shown in Figure 12. In the figure, R50 denotes recycled concrete with a 50% replacement rate of recycled aggregates, while MR50 refers to the R50 concrete that has been modified by the addition of nano-silica. This indicates that even a small amount of aerated admixture can help reduce the mass loss under salt-based freeze-thaw conditions.
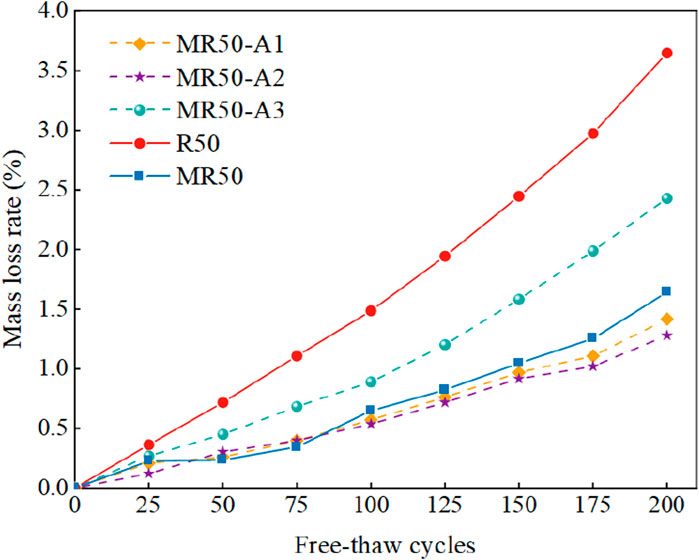
Figure 12. Mass loss rate of recycled concrete with different air-entraining agents after freeze-thaw cycles. Note: R50 signifies a replacement rate of 50% with recycled aggregate; MR50 refers to the R50 concrete that has been modified with nano-silica; MR50-A1 denotes the R50 concrete that has been modified with nano-silica and incorporates 0.01% of an air-entraining agent.
In summary, the incorporation of air-entraining admixtures can significantly enhance the frost resistance. This improvement is mainly seen in the reduction of RDEM loss and the decrease in scaling and mass loss rates.
4.2 Incorporating reinforcing fibers
The incorporation of fiber-reinforced materials into recycled concrete is a common approach to enhance frost resistance. Commonly used fiber-reinforced materials include steel fibers (GAO et al., 2007), polypropylene fibers (Tošić et al., 2022), glass fibers (Bhikshma and Kumar, 2015), carbon fibers (Zaid and Zamir Hashmi, 2021), and basalt fibers (Guo et al., 2023; Kang et al., 2024). Bai et al. (2023b) carried out freeze-thaw cycle tests on recycled concrete with different carbon fiber contents and analyzed the microscopic damage mechanisms. The test results indicated that carbon fibers hindered the entry of external water into the interior of recycled concrete, thus restraining the volume expansion of water during freezing. Furthermore, the presence of carbon fibers effectively resisted the tensile forces generated by concrete expansion and cracking, inhibiting crack formation and reducing the concrete’s mass loss. Zheng et al. (2022) found that the three-dimensional network structure formed by polypropylene fibers within the recycled concrete matrix could withstand some tensile stresses caused by freeze-thaw cycles, limiting the expansion and contraction of recycled concrete and mitigating the damage. Adding 0.10% polypropylene fibers to recycled concrete could simultaneously improve the strength and frost resistance. Yuan et al. (2024) incorporated basalt fibers into recycled concrete to enhance its frost resistance. The experimental results showed that basalt fibers led to the formation of a spatial network structure within the recycled concrete, thereby strengthening the interaction between aggregate particles and between the aggregates and the binding matrix. After 100 freeze-thaw cycles, recycled concrete specimens without basalt fibers (0 kg/m³) showed a significant mass loss, reaching the critical failure threshold. In contrast, specimens with different basalt fiber contents exhibited relatively minor mass loss. References (Gao et al., 2020; Liu et al., 2024) also confirmed the microscopic mechanism of action of fiber-reinforced materials in enhancing the frost resistance. In conclusion, the bridging and filling effects of fibers increase the interaction forces between aggregates and between aggregates and the matrix. On the other hand, they reduce the tensile forces required for the expansion of microcracks within recycled concrete, thereby enhancing the material’s frost resistance. The bridging mechanism of reinforcing fibers on concrete cracks is illustrated in Figure 13.
The effects of different fiber materials on enhancing the frost resistance of recycled concrete are inconsistent. Mahboob et al. (2024) added steel fibers, plastic fibers, and kenaf fibers into recycled concrete and conducted freeze-thaw cycle tests. The results showed that after 300 freeze-thaw cycles, the recycled concrete with fiber-reinforced materials had significantly lower mass loss rates and RDEM losses than traditional recycled concrete. Furthermore, compared to plastic fibers and kenaf fibers, steel fibers exhibited excellent microstructural protection. However, Pehlivanlı et al. (2015) demonstrated that steel fibers have weak bonding with the mortar matrix, limiting their performance. Mastali et al. (2016) found that adding glass fibers to recycled concrete could improve its frost resistance. However, glass fibers are relatively fragile and prone to breakage during incorporation, which leads to uneven distribution within the recycled concrete matrix and suboptimal performance. In contrast, Ramesh and Eswari (2021) found that basalt fibers maintained excellent mechanical properties under freeze-thaw cycles and exhibited better shear and tensile strengths than other fiber-reinforced materials, such as polypropylene. Furthermore, scholars have also studied the impact of incorporating mixed fibers on the frost resistance. For example, Ahmed and Lim (2023) prepared recycled concrete with basalt, polypropylene, and glass fibers, either individually or in combination. The study found that incorporating mixed fibers could significantly enhance the frost resistance. Similarly, Yuan et al. (2023) found that recycled concrete with a controlled proportion of basalt fibers and steel fibers exhibits better frost resistance compared to concrete with only a single type of fiber. The research data is shown in Figure 14. It is not difficult to see from the figure that after 150 freeze-thaw cycles, recycled concrete specimens (S1.5BF3.5/5.5/7.5) with a volume fraction of 1.5% steel fibers and a basalt fiber content of 3.5/55/7.5 kg/m3 exhibit superior compressive strength compared to all single basalt reinforced concrete specimens. This improvement is primarily due to the synergistic use of different fiber types, which reduces fiber aggregation and enhances the frost resistance.
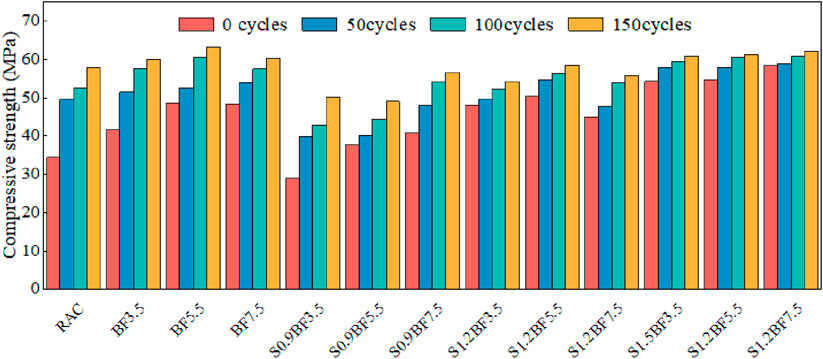
Figure 14. Compressive strength of recycled concrete with different reinforcing fibers after freeze-thaw cycles. Notes: BF denotes basalt fiber, and S represents steel fiber.
In summary, the bridging and filling functions of fibers in recycled concrete help to suppress the tensile stresses generated by freeze-thaw cycles, thereby enhancing the frost resistance. The type and performance of fiber materials are key factors affecting the frost resistance of recycled concrete. Furthermore, compared to incorporating a single type of fiber, the incorporation of multiple types of fibers typically further improves the frost resistance. Therefore, the rational introduction of fibers can effectively enhance the frost resistance of recycled concrete to some extent.
4.3 Aggregate modification
The mortar attached to recycled aggregates limits the promotion and use of recycled concrete. Therefore, modifying recycled aggregates to remove the surface of old mortar or enhance the performance of the ITZ is expected to enhance the frost resistance of recycled concrete. Existing methods for removing old mortar from the recycled aggregates include acid washing (Tanta et al., 2022), mechanical grinding (Lin et al., 2023b), thermal grinding (Kalinowska-Wichrowska et al., 2020), microwave heating (Xiao et al., 2012), and pulsed discharge treatment (Inoue et al., 2009), among others. Wang J et al. (2017) treated recycled concrete aggregates by immersing them in an acetic acid solution, finding that immersion in a 3% acetic acid solution for 24 h could soften the attached mortar, while a concentration of acetic acid above 3% would exert a blocking effect. Tam et al. (2007) treated recycled concrete aggregates with hydrochloric and phosphoric acid, respectively, and the results showed that the water absorption rate of the aggregates decreased by 12%, 9.8%, and 8.3% compared to the untreated state, respectively. However, the use of acid solutions to treat recycled concrete aggregates may damage the structure of natural aggregates containing limestone. Therefore, further optimization of the acid-washing process is required. Pepe et al. (2014) utilized mechanical grinding to treat recycled aggregates, and the results indicated that the water absorption rate was related to the treatment time. Sui and Mueller (2012) heated recycled concrete aggregates at temperatures ranging from 100°C to 600°C and then performed mechanical grinding, finding that treatment at approximately 300°C and sufficient grinding time could grind the apparent density of the recycled aggregates to be close to that of natural coarse aggregates. However, both the mortar attached and the natural aggregate would be worn during the grinding stage. Akbarnezhad et al. (2011) used microwave heating to treat recycled aggregates, and the content of the attached mortar and water absorption rate was reduced by 48% and 33%, respectively, after treatment. With the addition of mechanical friction, the content of the attached mortar and water absorption rate could be further reduced to 85% and 76%, respectively. Pulsed discharge treatment of recycled aggregates is considered one of the most effective treatment methods. Habibzai and Shigeishi (2020) used pulsed discharge to recover natural coarse aggregates and recycled fine aggregates. They discovered that after an appropriate number of discharge treatments, the attached mortar could be completely removed. Inoue et al. (2009) repeatedly discharged recycled concrete, and it was found that the quality of the recycled concrete aggregates increased with the increase in the number of treatments, and the water absorption rate could be reduced from 8% to less than 1%.
By filling the pores within the mortar and the ITZ, it is possible to increase the density of the mortar, reduce surface defects, and thereby enhance the performance of recycled aggregates. Microbially-induced calcium carbonate precipitation can form a protective layer, reducing the erosion caused by the external environment on the aggregate and enhancing the durability of the aggregate. Wang T et al. (2017) reinforced and modified recycled concrete aggregates using spherical bacillus, and the results indicated that the mass of the reinforced aggregates increased by 2.5%, with a maximum reduction in water absorption of 20%. Similarly, Qiu et al. (2014) demonstrated that the organic matter in the calcium carbonate induced by bacteria can reduce the wettability of the aggregate surface, decreasing water absorption in treated recycled aggregates. Coating the recycled aggregate surface with mortar repairs the pores and cracks in both the mortar and the ITZ. Hu et al. (2021) applied a zeolite powder-cement (ZP-C) slurry to the surface of RCAs and immersed them. The results demonstrated that a decrease in water absorption of 19.8% and 16.5% for the treated recycled aggregates, respectively. In addition, Zou et al. (2021) modified recycled aggregates using silane coupling agents. The results showed that after 200 freeze-thaw cycles, the mass loss rate was 54.7% lower than that of the unmodified group, and the RDEM was 26.1% higher, as shown in Figure 15. Kou and Poon (2010) strengthened recycled concrete aggregates by immersing them in different concentrations of polyvinyl alcohol solution, and the results showed that the higher the concentration of the polyvinyl alcohol solution, the higher the density and the lower the water absorption of the recycled concrete aggregates.
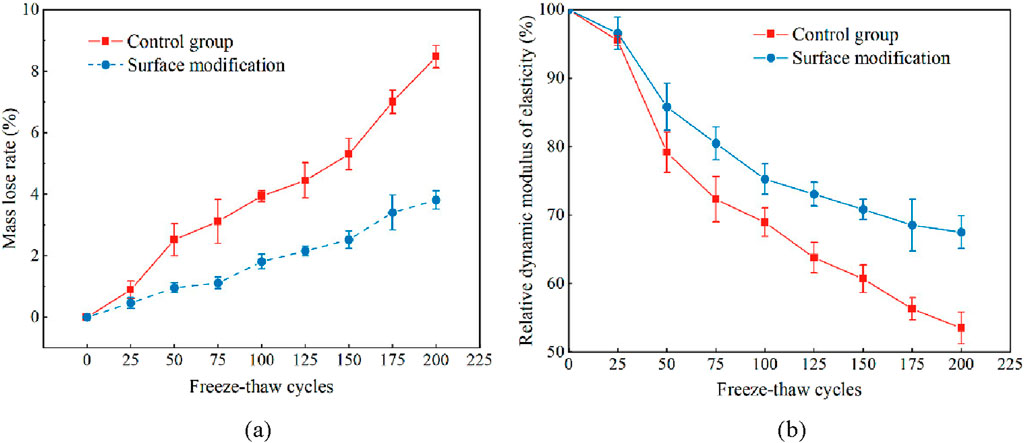
Figure 15. Average index of freeze-thaw durability of recycled concrete: (A) mass loss rate versus freeze-thaw cycles and (B) RDME versus freeze-thaw cycles (Zou et al., 2021).
Accelerated carbonation involves introducing CO2 into recycled concrete aggregates, leading to a chemical reaction with substances such as calcium hydroxide, calcium silicate hydration products, and calcium aluminate hydration products within the attached mortar, resulting in the formation of calcium carbonate precipitates, aluminates, and silicates (Leemann et al., 2023). This process reduces the porosity and water absorption rate, significantly enhancing the frost resistance. Liang et al. (2024) investigated the influence of incorporating CO2 curing on the properties of RAC subjected to freeze-thaw cycles. The results indicated that the CO2 curing method with Ca(OH)2 pre-soaking had the most significant effect on enhancing the frost resistance of recycled concrete. This resulted in a RDEM ranging from 65.27% to 67.71%. Similarly, Li et al. (2017b) investigated the frost resistance of recycled concrete with CO2 curing periods of 0, 3, and 7 days. The experimental results showed that after 300 freeze-thaw cycles, carbonated recycled concrete exhibited a lower mass loss rate compared to non-carbonated recycled concrete. This is because carbonation mitigates the negative effects of high initial porosity and other defects in recycled coarse aggregates during early freeze-thaw cycles and enhances the dispersion of water pressure during later cycles. Moreover, compared to recycled concrete cured for 3 days, recycled concrete cured for 7 days showed a lower mass loss rate and a higher RDEM. It can be observed that extending the CO2 curing time can improve the frost resistance. Peng et al. (2023) found that increasing the CO2-cured recycled aggregates content at the same freeze-thaw cycle number reduces the mass loss rate of recycled concrete. In addition, they found that during the CO2 curing process, the phase transformation occurring inside the material leads to the roughness of the loose ITZ between the matrix and the recycled aggregates, which is the main reason for improving the frost resistance. In summary, carbonation modification relies on the content of carbonizable substances within the attached mortar. However, for recycled concrete aggregates with long service life, the carbonizable substances content in the old mortar and ITZ is extremely low, which limits the efficiency of carbonation modification.
5 Conclusions and remarks
This paper reviews the primary influencing factors on the freeze-thaw resistance of recycled concrete, including the content of recycled aggregate, mineral admixtures, water-to-cement ratio, and water saturation levels, and provides a comprehensive evaluation. Additionally, it briefly summarizes the freeze-thaw damage mechanisms in recycled concrete and outlines several methods for improving its freeze-thaw resistance.
1 The ITZ is a crucial factor affecting the frost resistance of recycled concrete. This is because the ITZ of recycled concrete is complex, and its mechanical properties are poor. Since the internal micropore structure of recycled concrete is influenced by the quality, type, and amount of recycled aggregates, the freeze-thaw damage mechanism in recycled concrete is more complex than that of ordinary concrete, necessitating further investigation.
2 Recycled aggregates are influenced by their production processes, often exhibiting high paste attachment rates on the surface of the aggregates and weak ITZs. This often results in a generally inferior quality of the aggregates. Under freeze-thaw cycling conditions, the frost resistance of recycled concrete typically declines gradually with increasing amounts of recycled aggregates. However, incorporating an appropriate amount of mineral admixtures positively enhances the frost resistance. Extensive testing has shown that a low water-to-cement notably improves frost resistance. Additionally, studies have indicated that an optimal water saturation level of recycled aggregates can provide internal curing effect, further enhancing frost resistance in recycled concrete.
3 Under freeze-thaw actions, the random and isotropic distribution characteristics of fibers play a notable role in the frost resistance of recycled concrete. Additionally, air-entraining agents, commonly used as admixtures, effectively enhance the workability, cohesiveness, and plasticity of concrete while reducing segregation and bleeding. In terms of enhancing the frost resistance of recycled concrete, the impact of air-entraining agents is particularly pronounced. Furthermore, internal modification strategies such as silane treatment and CO2 curing of recycled aggregates also clearly elevate frost resistance, complementing external strengthening measures like fiber additions or admixture use, thus warranting widespread promotion.
4 In general, the inclusion of admixtures and additives significantly affects the frost resistance of recycled concrete. The careful use of these materials can enhance the frost resistance. However, current research lacks a unified quantification for the use of various admixtures and additives, such as silica fume, fly ash, rice husk ash, and air-entraining agents. Moreover, there are no standardized measures for improving the frost resistance of recycled concrete, such as the incorporation of fibers, internal curing, or modification of aggregates, meriting further exploration.
Based on existing research findings, the following recommendations are proposed to facilitate the application and promotion of recycled concrete in cold regions.
1 The incorporation rate of recycled aggregates significantly affects the frost resistance of recycled concrete. Based on current research, it is recommended that the content of recycled fine aggregates should not exceed 40% when replacing natural fine aggregates. When substituting natural coarse aggregates with recycled coarse aggregates, the recommended replacement rate should not exceed 50%.
2 In practical engineering, mineral admixtures are frequently used to replace a portion of the binding materials to achieve the objectives of cost reduction and performance enhancement. The addition of an appropriate amount of mineral admixtures can improve the frost resistance of recycled concrete. It is recommended to consider the impact of the dosage of mineral admixtures on the frost resistance of recycled concrete and determine the optimal amount of mineral admixtures.
3 The water-to-cement ratio is a significant factor influencing the frost resistance of recycled concrete. It is recommended to use a lower water-to-cement ratio in the mix design to achieve better frost resistance in recycled concrete.
4 An appropriate degree of water saturation can play a positive role in enhancing the frost resistance of recycled concrete. It is recommended to determine the optimal water saturation level of recycled aggregates to improve their frost resistance.
5 Air-entraining agents have a favorable effect on improving the frost resistance of recycled concrete. It is recommended to select an appropriate dosage to ensure that recycled concrete has good frost resistance. Moreover, an appropriate fiber content can also contribute to a significant improvement in its frost resistance.
6 The old mortar on the surface of recycled aggregates significantly influences the frost resistance of recycled concrete. It is recommended to choose an appropriate method to remove the old mortar from the surface of recycled aggregates or strengthen the recycled aggregates.
Author contributions
QM: Data curation, Formal Analysis, Investigation, Writing–original draft. ZD: Conceptualization, Funding acquisition, Supervision, Writing–review and editing. JW: Project administration, Validation, Writing–review and editing. GY: Formal Analysis, Writing–review and editing. XL: Conceptualization, Validation, Writing–review and editing.
Funding
The author(s) declare that no financial support was received for the research, authorship, and/or publication of this article.
Conflict of interest
Authors JW and XL were employed by China State Construction Engineering Corporation Ltd.
Author GY was employed by Linyi Lantai Environmental Protection Technology Co., Ltd.
The remaining author declares that the research was conducted in the absence of any commercial or financial relationships that could be construed as a potential conflict of interest.
Publisher’s note
All claims expressed in this article are solely those of the authors and do not necessarily represent those of their affiliated organizations, or those of the publisher, the editors and the reviewers. Any product that may be evaluated in this article, or claim that may be made by its manufacturer, is not guaranteed or endorsed by the publisher.
References
Ahmed, W., and Lim, C. W. (2023). Multicriteria performance assessment of sustainable recycled concrete produced via hybrid usage of basalt, polypropylene and glass fiber. Constr. Build. Mater 397, 132462. doi:10.1016/j.conbuildmat.2023.132462
Akbarnezhad, A., Ong, K. C. G., Zhang, M. H., Tam, C. T., and Foo, T. W. J. (2011). Microwave-assisted beneficiation of recycled concrete aggregates. Constr. Build. Mater 25, 3469–3479. doi:10.1016/j.conbuildmat.2011.03.038
Alabduljabbar, H., Farooq, F., Alyami, M., and Hammad, A. W. (2024). Assessment of the split tensile strength of fiber reinforced recycled aggregate concrete using interpretable approaches with graphical user interface. Mater Today Commun. 38, 108009. doi:10.1016/j.mtcomm.2023.108009
Amorim, N. S., Silva, G., and Ribeiro, D. V. (2018). Effects of the incorporation of recycled aggregate in the durability of the concrete submitted to freeze-thaw cycles. Constr. Build. Mater 161, 723–730. doi:10.1016/j.conbuildmat.2017.12.076
Ann, K. Y., Kim, M. J., Hwang, J. P., Cho, C., and Kim, K. H. (2017). Chloride transport in OPC concrete subjected to the freeze and thaw damage. Adv. Mater Sci. Eng. 2017, 8212856. doi:10.1155/2017/8212856
Atasham Ul Haq, M., Xia, P., Khan, S., Tahir, M., Hassam, M., Gong, F., et al. (2024). Characterizations and quantification of freeze-thaw behaviors of recycled brick aggregate concrete. J. Build. Eng. 86, 108821. doi:10.1016/j.jobe.2024.108821
Avet, F., Sofia, L., and Scrivener, K. (2019). Concrete performance of limestone calcined clay cement (LC3) compared with conventional cements. Adv. Civ. Eng. Mater 8, 275–286. doi:10.1520/ACEM20190052
Bai, W., Song, Z., Yuan, C., Guan, J., Xie, C., Huang, H., et al. (2023a). Study on mechanical properties and damage mechanism of recycled concrete containing silica fume in freeze–thaw environment. Constr. Build. Mater 375, 130872. doi:10.1016/j.conbuildmat.2023.130872
Bai, W., Wang, X., Yuan, C., Guan, J., Cao, K., and Xie, C. (2023b). Study on dynamic mechanical properties and meso-damage mechanism of carbon fibers recycled aggregate concrete under freeze-thaw environment. J. Build. Eng. 79, 107768. doi:10.1016/j.jobe.2023.107768
Bao, J. W., Zheng, R., Yu, Z. H., Zhang, P., Song, Q., Xu, J. G., et al. (2022). Freeze-thaw resistance of recycled aggregate concrete incorporating ferronickel slag as fine aggregate. Constr. Build. Mater 356. doi:10.1016/j.conbuildmat.2022.129178
Bhikshma, V., and Kumar, K. P. (2015). Study on flexural behavior of recycled aggregate concrete beams using glass fibers.
Bogas, J. A., de Brito, J., and Ramos, D. (2016). Freeze–thaw resistance of concrete produced with fine recycled concrete aggregates. J. Clean. Prod. 115, 294–306. doi:10.1016/j.jclepro.2015.12.065
Bu, C., Liu, L., Lu, X., Zhu, D., Sun, Y., Yu, L., et al. (2022). The durability of recycled fine aggregate concrete: a review. doi:10.3390/ma15031110ER
Bu, N., Liu, R., Fang, W., Zhao, H., and Bai, R. (2023). Study on mechanical properties and frost resistance of recycled fly ash concrete for construction. J. Funct. Mater. 54. doi:10.3969/j.issn.1001-9731.2023.05.030
Chandru, U., Bahurudeen, A., and Senthilkumar, R. (2023). Systematic comparison of different recycled fine aggregates from construction and demolition wastes in OPC concrete and PPC concrete. J. Build. Eng. 75, 106768. doi:10.1016/j.jobe.2023.106768
Chen, C., Lu, C., Lu, C., Wei, S., Guo, Z., Zhou, Q., et al. (2023). Synergetic effect of fly ash and ground-granulated blast slag on improving the chloride permeability and freeze–thaw resistance of recycled aggregate concrete. Constr. Build. Mater 365, 130015. doi:10.1016/j.conbuildmat.2022.130015
Chen, J. S., Huang, C. C., Chu, P. Y., and Lin, K. Y. (2007). Engineering characterization of recycled asphalt concrete and aged bitumen mixed recycling agent. J. Mater Sci. 42, 9867–9876. doi:10.1007/s10853-007-1713-8
Chen, X., Guo, Y., Gong, T., Zhang, L., Zhang, H., Qin, M., et al. (2024). Study on preparation and performance of brick-concrete recycled sand powder autoclaved aerated concrete. J. Build. Eng. 91, 109671. doi:10.1016/j.jobe.2024.109671
Cheng, S., Shui, Z., Yu, R., Zhang, X., and Zhu, S. (2018). Durability and environment evaluation of an eco-friendly cement-based material incorporating recycled chromium containing slag. J. Clean. Prod. 185, 23–31. doi:10.1016/j.jclepro.2018.03.048
Cheng, X., Pinghua, Z., and Xinjie, W. (2024). Effects of mineral admixtures on frost resistance and renewability of recycled aggregate concrete. Bull. Chin. Ceram. Soc. 43, 956–964. doi:10.16552/j.cnki.issn1001-1625.2024.03.007
Cheng, Y., Shang, X., and Zhang, Y. (2017). Experimental research on durability of recycled aggregate concrete under freeze-thaw cycles. J. Phys. Conf. Ser. 870, 12018. doi:10.1088/1742-6596/870/1/012018
Corinaldesi, V. (2012). Mechanical performance and durability of fly ash recycled aggregate concretes. Adv. Mater. Res. 538-541, 1568–1572. doi:10.4028/www.scientific.net/AMR.538-541.1568
Datta, S. D., Sobuz, M. H. R., Akid, A. S. M., and Islam, S. (2022). Influence of coarse aggregate size and content on the properties of recycled aggregate concrete using non-destructive testing methods. J. Build. Eng. 61, 105249. doi:10.1016/j.jobe.2022.105249
Deng, Q., Zhang, R., Liu, C., Duan, Z., and Xiao, J. (2023). Influence of fiber properties on abrasion resistance of recycled aggregate concrete: length, volume fraction, and types of fibers. Constr. Build. Mater 362, 129750. doi:10.1016/j.conbuildmat.2022.129750
Deng, X., Gao, X., Wang, R., Gao, M., Yan, X., Cao, W., et al. (2021a). Investigation of microstructural damage in air-entrained recycled concrete under a freeze–thaw environment. Constr. Build. Mater 268, 121219. doi:10.1016/j.conbuildmat.2020.121219
Deng, X. H., Liu, Y. Y., and Wang, R. (2021b). Investigating freeze-proof durability of air-entrained C30 recycled coarse aggregate concrete. Arch. Civ. Eng. 67, 507–524. doi:10.24425/ace.2021.137182
de Oliveira, M. B., and Vazquez, E. (1996). The influence of retained moisture in aggregates from recycling on the properties of new hardened concrete. Waste Manag. 16, 113–117. doi:10.1016/S0956-053X(96)00033-5
El-Hawary, M., and Al-Sulily, A. (2020). Internal curing of recycled aggregates concrete. J. Clean. Prod. 275, 122911. doi:10.1016/j.jclepro.2020.122911
El-Hawary, M., Al-Yaqout, A., and Elsayed, K. (2021). Freezing and thawing cycles: effect on recycled aggregate concrete including slag. Int. J. Sustain Eng. 14, 800–808. doi:10.1080/19397038.2021.1886374
Gao, D., Zhang, L., Zhao, J., and You, P. (2020). Durability of steel fibre-reinforced recycled coarse aggregate concrete. Constr. Build. Mater 232, 117119. doi:10.1016/j.conbuildmat.2019.117119
Gao, Q., Ma, Z., Xiao, J., and Li, F. (2018). Effects of imposed damage on the capillary water absorption of recycled aggregate concrete. Adv. Mater Sci. Eng. 2018, 2890931. doi:10.1155/2018/2890931
Gao, D., Lou, Z., and Wang, Z. (2007). Experimental research on the compressive strength of steel fiber recycled concrete. J. Zhengzhou Univ. 28, 5–10. doi:10.1360/yc-007-0745
Ghorbani, S., Sharifi, S., Ghorbani, S., Tam, V. W., de Brito, J., and Kurda, R. (2019). Effect of crushed concrete waste’s maximum size as partial replacement of natural coarse aggregate on the mechanical and durability properties of concrete. Resour. Conservation Recycl. 149, 664–673. doi:10.1016/j.resconrec.2019.06.030
Gokce, A., Nagataki, S., Saeki, T., and Hisada, M. (2004). Freezing and thawing resistance of air-entrained concrete incorporating recycled coarse aggregate: the role of air content in demolished concrete. Cem. Concr. Res. 34, 799–806. doi:10.1016/j.cemconres.2003.09.014
Gong, F., and Jacobsen, S. (2019). Modeling of water transport in highly saturated concrete with wet surface during freeze/thaw. Cem. Concr. Res. 115, 294–307. doi:10.1016/j.cemconres.2018.08.013
Guan, T., Zhang, L., Zheng, Y., Guan, J., Zhang, Y., and Zhang, Y. (2024). Frost resistance and damage evolution model of basalt fiber-reinforced recycled concrete based on recycled coarse aggregate strengthening and vibration mixing processes. J. Build. Eng. 91, 109627. doi:10.1016/j.jobe.2024.109627
Gunasekara, C., Zhou, Z., Law, D. W., Sofi, M., Setunge, S., and Mendis, P. (2020). Microstructure and strength development of quaternary blend high-volume fly ash concrete. J. Mater Sci. 55, 6441–6456. doi:10.1007/s10853-020-04473-1
Guo, J., Sun, W., Xu, Y., Lin, W., and Jing, W. (2022). Damage Mech. Model. Concr. Freeze–Thaw Cycles a Rev., doi:10.3390/buildings12091317
Guo, Y., Liu, Y., Wang, W., Wang, K., Zhang, Y., and Hou, J. (2023). Effect of basalt fiber on uniaxial compression-related constitutive relation and compressive toughness of recycled aggregate concrete. doi:10.3390/ma16051849
Guo, Z., Jiang, T., Zhang, J., Kong, X., Chen, C., and Lehman, D. E. (2020). Mechanical and durability properties of sustainable self-compacting concrete with recycled concrete aggregate and fly ash, slag and silica fume. Constr. Build. Mater 231, 117115. doi:10.1016/j.conbuildmat.2019.117115
Habibzai, R., and Shigeishi, M. (2020). Quality evaluation of pulsed power recycled sand by electrical resistivity method. Int. J. GEOMATE 19, 66–75. doi:10.21660/2020.75.84833
Hamman, A., Ismail, M., and Roushdy, M. (2021) Incorporation of perlite and recycled aggregates for internal concrete curing. Int. Conf. Transp. Dev., 225–234. doi:10.1061/9780784483541.021
Hasholt, M. T. (2014). Air void structure and frost resistance: a challenge to Powers’ spacing factor. Mater Struct. 47, 911–923. doi:10.1617/s11527-013-0102-9
Hu, H., He, Z., Fan, K., Shibro, T., Liu, B., and Shi, J. (2021). Properties enhancement of recycled coarse aggregates by pre-coating/pre-soaking with zeolite powder/calcium hydroxide. Constr. Build. Mater 286, 122888. doi:10.1016/j.conbuildmat.2021.122888
Huaishuai, S., Zhiheng, W., Peng, Z., Tiejun, Z., Guoxi, F., and Guosheng, R. (2017). Bond behavior of steel bar in air-entrained RCAC in fresh water and sea water after fast freeze-thaw cycles. Cold Reg. Sci. Technol. 135, 90–96. doi:10.1016/j.coldregions.2016.11.005
Inoue, S., Araki, J., Aoki, T., Maeda, S., Iizasa, S., Takaki, M., et al. (2009). Coarse aggregate recycling by pulsed discharge inside of concrete. Acta Phys. Pol. A 115, 1107–1109. doi:10.12693/APhysPolA.115.1107
Islam, M. J., Islam, K., Shahjalal, M., Khatun, E., Islam, S., and Razzaque, A. B. (2022). Influence of different types of fibers on the mechanical properties of recycled waste aggregate concrete. Constr. Build. Mater 337, 127577. doi:10.1016/j.conbuildmat.2022.127577
Ji, Y., and Wang, D. (2022). Durability of recycled aggregate concrete in cold regions. Case Stud. Constr. Mater 17, e01475. doi:10.1016/j.cscm.2022.e01475
Kalinowska-Wichrowska, K., Pawluczuk, E., and Bołtryk, M. (2020). Waste-free technology for recycling concrete rubble. Constr. Build. Mater 234, 117407. doi:10.1016/j.conbuildmat.2019.117407
Kang, J., Chen, X., Yu, Z., and Wang, L. (2024). Study on the fatigue life and toughness of recycled aggregate concrete based on basalt fiber. Mater Today Commun. 40, 109397. doi:10.1016/j.mtcomm.2024.109397
Khoury, E., Cazacliu, B., and Remond, S. (2019). Control of effective water in recycled aggregate concrete using power curves of the mixer. Mater Today Commun. 21, 100721. doi:10.1016/j.mtcomm.2019.100721
Kobayashi, K., Miura, S., Oshima, E., and Yun, H. (2022). Mechanisms of high frost scaling resistance of SHCC. Constr. Build. Mater 324, 126300. doi:10.1016/j.conbuildmat.2021.126300
Kou, S., and Poon, C. (2010). Properties of concrete prepared with PVA-impregnated recycled concrete aggregates. Cem. Concr. Compos. 32, 649–654. doi:10.1016/j.cemconcomp.2010.05.003
Lam, N. N. (2020). Influence of fly ash and recycled AAC waste for replacement of natural sand in manufacture of autoclaved aerated concrete. IOP Conf. Ser. Earth Environ. Sci. 505, 12001. doi:10.1088/1755-1315/505/1/012001
Lee, S., Jang, S. Y., Kim, C. Y., Ahn, E. J., Kim, S. P., Gwon, S., et al. (2018). Effects of redispersible polymer powder on mechanical and durability properties of preplaced aggregate concrete with recycled railway ballast. Int. J. Concr. Struct. Mater 12, 69. doi:10.1186/s40069-018-0304-1
Lee, S., Park, S., Kim, D., and Kang, J. (2021). Effect of freeze–thaw cycles on the performance of concrete containing water-cooled and air-cooled slag. doi:10.3390/app11167291ER
Leemann, A., Münch, B., and Wyrzykowski, M. (2023). CO2 absorption of recycled concrete aggregates in natural conditions. Mater Today Commun. 36, 106569. doi:10.1016/j.mtcomm.2023.106569
Lei, B., Li, W., Tang, Z., Tam, V. W. Y., and Sun, Z. (2018). Durability of recycled aggregate concrete under coupling mechanical loading and freeze-thaw cycle in salt-solution. Constr. Build. Mater 163, 840–849. doi:10.1016/j.conbuildmat.2017.12.194
Levy, S. M., and Helene, P. (2004). Durability of recycled aggregates concrete: a safe way to sustainable development. Cem. Concr. Res. 34, 1975–1980. doi:10.1016/j.cemconres.2004.02.009
Li, J., and Qin, C. L. (2013). Strengths investigation of air entrained recycled fine aggregate mortar. Appl. Mech. Mater. 253-255, 432–435. doi:10.4028/www.scientific.net/AMM.253-255.432
Li, T., Nogueira, R., de Brito, J., and Liu, J. (2024). A simple method to address the high water absorption of recycled aggregates in cementitious mixes. Constr. Build. Mater 411, 134404. doi:10.1016/j.conbuildmat.2023.134404
Li, W., Lu, K., and Walz, J. Y. (2012a). Effects of added kaolinite on the strength and porosity of freeze-cast kaolinite–silica nanocomposites. J. Mater Sci. 47, 6882–6890. doi:10.1007/s10853-012-6631-8
Li, W., Pour-Ghaz, M., Castro, J., and Weiss, J. (2012b). Water absorption and critical degree of saturation relating to freeze-thaw damage in concrete pavement joints. J. Mater Civ. Eng. 24, 299–307. doi:10.1061/(ASCE)MT.1943-5533.0000383
Li, Y., Jin, K., Lin, H., Shen, J., Shi, J., and Fan, M. (2024). Analysis and prediction of freeze-thaw resistance of concrete based on machine learning. Mater Today Commun. 39, 108946. doi:10.1016/j.mtcomm.2024.108946
Li, Y., Wang, R. J., Li, S. Y., and Zhao, Y. (2017b). Assessment of the freeze-thaw resistance of concrete incorporating carbonated coarse recycled concrete aggregates. J. Ceram. Soc. Jpn. 125, 837–845. doi:10.2109/jcersj2.17111
Li, Z., Liu, L., Yan, S., Zhang, M., Xia, J., and Xie, Y. (2019). Effect of freeze-thaw cycles on mechanical and porosity properties of recycled construction waste mixtures. Constr. Build. Mater 210, 347–363. doi:10.1016/j.conbuildmat.2019.03.184
Liang, C., Wang, S., Cai, Z., Yin, Y., Gao, Y., Guo, M., et al. (2024). Effects of CO2 curing methods on frost resistance and mechanical properties of recycled aggregate concrete. Case Stud. Constr. Mater 20, e02973. doi:10.1016/j.cscm.2024.e02973
Lin, Y., Feng, X., and Zhang, Z. (2023a). Microscopic simulation of thermo-mechanical behaviors in recycled concrete under freeze–thaw action. Constr. Build. Mater 409, 133892. doi:10.1016/j.conbuildmat.2023.133892
Lin, Y., He, T., and Da, Y. (2023b). Influence of self-grinding modification on the properties of recycled coarse aggregate and recycled concrete. Mater. Rev. 37.
Liu, G., Hunger, M., Tošić, N., and de la Fuente, A. (2023). Effect of free and embedded polypropylene fibres recovered from concrete recycling on the properties of new concrete. Constr. Build. Mater 409, 134145. doi:10.1016/j.conbuildmat.2023.134145
Liu, J., Shang, Y., Miao, C., and Ran, Q. (2011). Effects of air entraining manners of polycarboxylate-type water reducer on the properties of concrete. J. Build. Mater. 14, 528–531. doi:10.3969/j.issn.1007-9629.2011.04.018
Liu, K., Yan, J., Hu, Q., Sun, Y., and Zou, C. (2016). Effects of parent concrete and mixing method on the resistance to freezing and thawing of air-entrained recycled aggregate concrete. Constr. Build. Mater 106, 264–273. doi:10.1016/j.conbuildmat.2015.12.074
Liu, L., Qin, S., and Wang, X. (2018). Poro-elastic–plastic model for cement-based materials subjected to freeze–thaw cycles. Constr. Build. Mater 184, 87–99. doi:10.1016/j.conbuildmat.2018.06.197
Liu, R., Xiao, H., Liu, J., Guo, S., and Pei, Y. (2019). Improving the microstructure of ITZ and reducing the permeability of concrete with various water/cement ratios using nano-silica. J. Mater Sci. 54, 444–456. doi:10.1007/s10853-018-2872-5
Liu, Z., and Hansen, W. (2016). A geometrical model for void saturation in air-entrained concrete under continuous water exposure. Constr. Build. Mater 124, 475–484. doi:10.1016/j.conbuildmat.2016.07.113
Li, Y., Wang, R., and Zhao, Y. (2017a). Effect of coupled deterioration by freeze-thaw cycle and carbonation on concrete produced with coarse recycled concrete aggregates. J. Ceram. Soc. Jpn. 125, 36–45. doi:10.2109/jcersj2.16183
Long, T., Dong, S. H., Cui, S. M., and Wang, Q. (2013). Compressive behaviour of recycle concrete cubes after freeze-thaw cycles. Adv. Mater. Res. 671-674, 1865–1868. doi:10.4028/www.scientific.net/AMR.671-674.1865
Luan, H., Wu, J., and Pan, J. (2020). Saline water absorption behavior and critical saturation degree of recycled aggregate concrete during freeze-thaw cycles. Constr. Build. Mater 258, 119640. doi:10.1016/j.conbuildmat.2020.119640
Luan, H., Wu, J., and Pan, J. (2021). Freeze-thaw durability of recycled aggregate concrete: an overview. J. Wuhan Univ. Technology-Mater. Sci. Ed. 36, 58–69. doi:10.1007/s11595-021-2378-x
Luo, X. W., Yao, H. L., and Lu, Z. (2015). Production of recycled concrete and its material properties. Mater. Res. Innovations 19, S1–S147. doi:10.1179/1432891715Z.0000000001392
Ma, W., Lv, B., Wang, Y., Huang, L., Yan, L., and Kasal, B. (2024). Freeze-thaw, chloride penetration and carbonation resistance of natural and recycled aggregate concrete containing rice husk ash as replacement of cement. J. Build. Eng. 86, 108889. doi:10.1016/j.jobe.2024.108889
Mahboob, A., Hassanshahi, O., Safi, M., and Majid, T. A. (2024). Experimental investigation of eco-friendly fiber-reinforced concrete using recycled and natural fibers, integrated with recycled aggregates. Adv. Compos Mater, 1–30. doi:10.1080/09243046.2024.2322799
Marchi, T., Garcia Diaz, E., Salgues, M., Souche, J. C., and Devillers, P. (2023). Internal curing capacity of recycled coarse aggregates incorporated in concretes with low water/cement ratios. Constr. Build. Mater 409, 133893. doi:10.1016/j.conbuildmat.2023.133893
Mardani-Aghabaglou, A., Yüksel, C., Beglarigale, A., and Ramyar, K. (2019). Improving the mechanical and durability performance of recycled concrete aggregate-bearing mortar mixtures by using binary and ternary cementitious systems. Constr. Build. Mater 196, 295–306. doi:10.1016/j.conbuildmat.2018.11.124
Mastali, M., Dalvand, A., and Sattarifard, A. R. (2016). The impact resistance and mechanical properties of reinforced self-compacting concrete with recycled glass fibre reinforced polymers. J. Clean. Prod. 124, 312–324. doi:10.1016/j.jclepro.2016.02.148
Meng, D., Wu, X., Quan, H., and Zhu, C. (2021). A strength-based mix design method for recycled aggregate concrete and consequent durability performance. Constr. Build. Mater 281, 122616. doi:10.1016/j.conbuildmat.2021.122616
Meng, T., Lai, Z., Yang, X., Dai, D., Jia, Y., and Yu, H. (2024). An approach to effectively improve the properties of recycled concrete aggregate and recycled brick aggregate by micro-nano particle reconstruction. Constr. Build. Mater 421, 135669. doi:10.1016/j.conbuildmat.2024.135669
Muhammad, F., Harun, M., Ahmed, A., Kabir, N., Khalid, H. R., and Hanif, A. (2024). Influence of bonded mortar on recycled aggregate concrete properties: a review. Constr. Build. Mater 432, 136564. doi:10.1016/j.conbuildmat.2024.136564
Nazeer, M., Kapoor, K., and Singh, S. P. (2024). Impact of recycled concrete aggregates on the strength and durability properties of pervious concrete. Mag. Concr. Res. 76, 272–291. doi:10.1680/jmacr.23.00089
Oertel, T., Hutter, F., Helbig, U., and Sextl, G. (2014). Amorphous silica in ultra-high performance concrete: first hour of hydration. Cem. Concr. Res. 58, 131–142. doi:10.1016/j.cemconres.2014.01.008
Olanike, A. O. (2014). Experimental investigation into the freeze-thaw resistance of concrete using recycled concrete aggregates and admixtures. Ratio (Oxf) 85, 120. doi:10.13189/cea.2014.020404
Omary, S., Ghorbel, E., and Wardeh, G. (2016). Relationships between recycled concrete aggregates characteristics and recycled aggregates concretes properties. Constr. Build. Mater 108, 163–174. doi:10.1016/j.conbuildmat.2016.01.042
Peceño, B., Arenas, C., Alonso-Fariñas, B., and Leiva, C. (2019). Substitution of coarse aggregates with mollusk-shell waste in acoustic-absorbing concrete. J. Mater Civ. Eng. 31, 4019077. doi:10.1061/(ASCE)MT.1943-5533.0002719
Pehlivanlı, Z. O., Uzun, İ., and Demir, İ. (2015). Mechanical and microstructural features of autoclaved aerated concrete reinforced with autoclaved polypropylene, carbon, basalt and glass fiber. Constr. Build. Mater 96, 428–433. doi:10.1016/j.conbuildmat.2015.08.104
Peled, A., Castro, J., and Weiss, J. (2010). Atomic force microscopy examinations of mortar made by using water-filled lightweight aggregate. Transp. Res. Rec. 2141, 92–101. doi:10.3141/2141-16
Peng, X., Shi, F., Yang, J., Yang, Q., Wang, H., and Zhang, J. (2023). Modification of construction waste derived recycled aggregate via CO2 curing to enhance corrosive freeze-thaw durability of concrete. J. Clean. Prod. 405, 137016. doi:10.1016/j.jclepro.2023.137016
Pepe, M., Toledo Filho, R. D., Koenders, E. A. B., and Martinelli, E. (2014). Alternative processing procedures for recycled aggregates in structural concrete. Constr. Build. Mater 69, 124–132. doi:10.1016/j.conbuildmat.2014.06.084
Pickel, D., Tighe, S., and West, J. S. (2017). Assessing benefits of pre-soaked recycled concrete aggregate on variably cured concrete. Constr. Build. Mater 141, 245–252. doi:10.1016/j.conbuildmat.2017.02.140
Posi, P., Thongjapo, P., Thamultree, N., Boontee, P., Kasemsiri, P., and Chindaprasirt, P. (2016). Pressed lightweight fly ash-OPC geopolymer concrete containing recycled lightweight concrete aggregate. Constr. Build. Mater 127, 450–456. doi:10.1016/j.conbuildmat.2016.09.105
Powers, T. C. (1945). Working hypothesis for further studies of frost resistance of concrete. ACI J. Proc. 41. doi:10.14359/8684
Powers, T. C., and Helmuth, R. A. (1953). Theory of volume changes in hardened portland-cement paste during freezing.
Qiu, J., Li, L., Li, L., Luan, X., Guan, X., and Niu, G. (2024). Study on the deterioration characteristics and mechanisms of recycled brick-concrete aggregate concrete under load-freeze-thaw coupling. Constr. Build. Mater 413, 134817. doi:10.1016/j.conbuildmat.2023.134817
Qiu, J., Tng, D. Q. S., and Yang, E. (2014). Surface treatment of recycled concrete aggregates through microbial carbonate precipitation. Constr. Build. Mater 57, 144–150. doi:10.1016/j.conbuildmat.2014.01.085
Quan, X. Y., Wang, S. L., Li, J. T., Luo, J., Liu, K. N., Xu, J., et al. (2022). Utilization of molybdenum tailings as fine aggregate in recycled aggregate concrete. J. Clean. Prod. 372. doi:10.1016/j.jclepro.2022.133649
Ramesh, B., and Eswari, S. (2021). Mechanical behaviour of basalt fibre reinforced concrete: an experimental study. Mater. Today Proc. 43, 2317–2322. doi:10.1016/j.matpr.2021.01.071
Ren, Q., Pacheco, J., de Brito, J., and Hu, J. (2024). Analysis of the influence of the attached mortar’s geometry on the mechanical behaviour of recycled aggregate concrete through mesoscale modelling. Eng. Fract. Mech. 297, 109876. doi:10.1016/j.engfracmech.2024.109876
Rong, X. L., Li, L., Zheng, S. S., Wang, F., Huang, W. Y., Zhang, Y. X., et al. (2023). Freeze‒thaw damage model for concrete considering a nonuniform temperature field. J. Build. Eng. 72, 106747. doi:10.1016/j.jobe.2023.106747
Sáez Del Bosque, I. F., Zhu, W., Howind, T., Matías, A., Sánchez De Rojas, M. I., and Medina, C. (2017). Properties of interfacial transition zones (ITZs) in concrete containing recycled mixed aggregate. Cem. Concr. Compos. 81, 25–34. doi:10.1016/j.cemconcomp.2017.04.011
Saha, A. K., and Sarker, P. K. (2020). Mitigation of the potential alkali-silica reaction of FNS using around FNS as a supplementary binder. Adv. Cem. Res. 32, 537–546. doi:10.1680/jadcr.19.00035
Scherer, G. W. (1999). Crystallization in pores. Cem. Concr. Res. 29, 1347–1358. doi:10.1016/S0008-8846(99)00002-2
Scrivener, K. L., Lothenbach, B., De Belie, N., Gruyaert, E., Skibsted, J., Snellings, R., et al. (2015). TC 238-SCM: hydration and microstructure of concrete with SCMs. Mater Struct. 48, 835–862. doi:10.1617/s11527-015-0527-4
Setzer, M. J. (2001a). Micro-ice-lens formation in porous solid. J. Colloid Interface Sci. 243, 193–201. doi:10.1006/jcis.2001.7828
Setzer, M. J. (2001b). Mechanical stability criterion, triple-phase condition, and pressure differences of matter condensed in a porous matrix. J. Colloid Interface Sci. 235, 170–182. doi:10.1006/jcis.2000.7317
Shen, W., Wu, J., Du, X., Li, Z., Wu, D., Sun, J., et al. (2022). Cleaner production of high-quality manufactured sand and ecological utilization of recycled stone powder in concrete. J. Clean. Prod. 375, 134146. doi:10.1016/j.jclepro.2022.134146
Şimşek, O., Pourghadri Sefidehkhan, H., and Gökçe, H. S. (2022). Performance of fly ash-blended Portland cement concrete developed by using fine or coarse recycled concrete aggregate. Constr. Build. Mater 357, 129431. doi:10.1016/j.conbuildmat.2022.129431
Song, H., Xie, W., Liu, J., Cheng, F., Gasem, K. A. M., and Fan, M. (2018). Effect of surfactants on the properties of a gas-sealing coating modified with fly ash and cement. J. Mater Sci. 53, 15142–15156. doi:10.1007/s10853-018-2679-4
Sosa, M. E., Villagrán Zaccardi, Y. A., and Zega, C. J. (2021). A critical review of the resulting effective water-to-cement ratio of fine recycled aggregate concrete. Constr. Build. Mater 313, 125536. doi:10.1016/j.conbuildmat.2021.125536
Sui, Y., and Mueller, A. (2012). Development of thermo-mechanical treatment for recycling of used concrete. Mater Struct. 45, 1487–1495. doi:10.1617/s11527-012-9852-z
Sun, J., and Geng, J. (2012). Effect of particle size and content of recycled fine aggregate on frost resistance of concrete. J. Build. Mater. 15, 382–385. doi:10.3969/j.issn.1007-9629.2012.03.017
Sunayana, S., and Barai, S. V. (2021). Partially fly ash incorporated recycled coarse aggregate based concrete: microstructure perspectives and critical analysis. Constr. Build. Mater 278, 122322. doi:10.1016/j.conbuildmat.2021.122322
Tam, V. W. Y., Gao, X. F., and Tam, C. M. (2005). Microstructural analysis of recycled aggregate concrete produced from two-stage mixing approach. Cem. Concr. Res. 35, 1195–1203. doi:10.1016/j.cemconres.2004.10.025
Tam, V. W. Y., Tam, C. M., and Le, K. N. (2007). Removal of cement mortar remains from recycled aggregate using pre-soaking approaches. Resour. Conservation Recycl. 50, 82–101. doi:10.1016/j.resconrec.2006.05.012
Tanta, A., Kanoungo, A., Singh, S., and Kanoungo, S. (2022). The effects of surface treatment methods on properties of recycled concrete aggregates. Mater. Today Proc. 50, 1848–1852. doi:10.1016/j.matpr.2021.09.223
Tarhan, Y., and Şahin, R. (2021). Effect of recycled concrete aggregate, air entraining admixture and maximum aggregate particle size on the behavior of concrete under freeze-thaw cycles. J. Green Build. 16, 217–233. doi:10.3992/jgb.16.2.217
Tošić, N., Peralta Martínez, D., Hafez, H., Reynvart, I., Ahmad, M., Liu, G., et al. (2022). Multi-recycling of polypropylene fibre reinforced concrete: influence of recycled aggregate properties on new concrete. Constr. Build. Mater 346, 128458. doi:10.1016/j.conbuildmat.2022.128458
Tran, D. L., Mouret, M., Cassagnabère, F., and Phung, Q. T. (2022). Effects of intrinsic granular porosity and mineral admixtures on durability and transport properties of recycled aggregate concretes. Mater Today Commun. 33, 104709. doi:10.1016/j.mtcomm.2022.104709
Tuyan, M., Mardani-Aghabaglou, A., and Ramyar, K. (2014). Freeze–thaw resistance, mechanical and transport properties of self-consolidating concrete incorporating coarse recycled concrete aggregate. Mater Des. 53, 983–991. doi:10.1016/j.matdes.2013.07.100
Wang, C., Wang, J., Liu, X., Cai, Y., and Zhang, Y. (2023a). Orthogonal experimental design for compressive strength of recycled coarse aggregate concrete with silica fume-slag-fly ash hybrid micro-powders. Constr. Build. Mater 408, 133669. doi:10.1016/j.conbuildmat.2023.133669
Wang, D., Xu, Y., Zheng, Y., and Wu, Y. (2023b). Effect of freeze–thaw cycles on physical and mechanical properties of concrete with different replacement rates of recycled coarse aggregate. Int. J. Pavement Res. Technol. doi:10.1007/s42947-023-00397-6
Wang, H., Zhu, P., Yan, X., Liu, H., Zhu, L., and Wang, X. (2023c). Effect of silica fume on frost resistance and recyclability potential of recycled aggregate concrete under freeze–thaw environment. Constr. Build. Mater 409, 134109. doi:10.1016/j.conbuildmat.2023.134109
Wang, J., Vandevyvere, B., Vanhessche, S., Schoon, J., Boon, N., and De Belie, N. (2017). Microbial carbonate precipitation for the improvement of quality of recycled aggregates. J. Clean. Prod. 156, 355–366. doi:10.1016/j.jclepro.2017.04.051
Wang, T., Xue, Y., Zhou, M., Lv, Y., Chen, Y., Wu, S., et al. (2017). Hydration kinetics, freeze-thaw resistance, leaching behavior of blended cement containing co-combustion ash of sewage sludge and rice husk. Constr. Build. Mater 131, 361–370. doi:10.1016/j.conbuildmat.2016.11.087
Wang, W., Wang, S., Peng, L., Wang, N., Meng, T., Zhao, Y., et al. (2024). Preliminary investigation and life cycle assessment of artificial reefs with recycled brick-concrete aggregates. Constr. Build. Mater 432, 136618. doi:10.1016/j.conbuildmat.2024.136618
Wang, Y., Hu, Z., and Liu, J. (2023d). Freeze-thaw resistance of concrete containing azodicarbonamide expansive agent. Constr. Build. Mater 367, 130335. doi:10.1016/j.conbuildmat.2023.130335
Wang, Y., Liu, J., Zhu, P., Liu, H., Wu, C., and Zhao, J. (2021). Investigation of adhered mortar content on recycled aggregate using image analysis method. J. Mater Civ. Eng. 33, 4021225. doi:10.1061/(ASCE)MT.1943-5533.0003864
Wang, Y., Xie, M., and Zhang, J. (2023e). Mechanical properties and damage model of modified recycled concrete under freeze-thaw cycles. J. Build. Eng. 78, 107680. doi:10.1016/j.jobe.2023.107680
Wang, Z., Zeng, Q., Wang, L., Yao, Y., and Li, K. (2014). Effect of moisture content on freeze–thaw behavior of cement paste by electrical resistance measurements. J. Mater Sci. 49, 4305–4314. doi:10.1007/s10853-014-8126-2
Wei, D., Zhu, P., Yan, X., Liu, H., Chen, C., and Wang, Z. (2022). Potential evaluation of waste recycled aggregate concrete for structural concrete aggregate from freeze-thaw environment. Constr. Build. Mater 321, 126291. doi:10.1016/j.conbuildmat.2021.126291
Wu, Y., Gao, K., and Wu, B. (2019). Influence of fly ash on the compressive strength of concrete containing recycled aggregate. Emerg. Mater Res. 8, 483–491. doi:10.1680/jemmr.18.00013
Xia, G., and Zhao, Y. (2024). Interface parameters of recycled aggregate concrete considering the distribution of old mortar content. Case Stud. Constr. Mater 20, e03262. doi:10.1016/j.cscm.2024.e03262
Xia, P., Yang, L., Wang, S., Gong, F., Cao, W., and Zhao, Y. (2023). Improved freeze-thaw modification of recycled concrete aggregate originally from frost resistive concrete. Cem. Concr. Compos. 144, 105302. doi:10.1016/j.cemconcomp.2023.105302
Xiao, J., Li, W., and Poon, C. (2012). Recent studies on mechanical properties of recycled aggregate concrete in China—a review. Sci. China Technol. Sci. 55, 1463–1480. doi:10.1007/s11431-012-4786-9
Xiao, J., Li, W., Sun, Z., Lange, D. A., and Shah, S. P. (2013). Properties of interfacial transition zones in recycled aggregate concrete tested by nanoindentation. Cem. Concr. Compos. 37, 276–292. doi:10.1016/j.cemconcomp.2013.01.006
Xiao, J., Wu, L., and Fan, Y. (2012). Test on modification of recycled coarse aggregate by microwave heating. Concrete, 55–57. doi:10.3969/j.issn.1002-3550.2012.07.018
Xiao, Q., Wu, Z., Qiu, J., Dong, Z., and Shi, S. (2022). Capillary water absorption performance and damage constitutive model of recycled concrete under freeze–thaw action. Constr. Build. Mater 353, 129120. doi:10.1016/j.conbuildmat.2022.129120
Yan, W. J., Niu, F. J., and Zhang, X. J. (2014). Advances in studies on concrete durability and countermeasures against freezing-thawing effects. Sci. Cold Arid. Reg. 6, 398–408. doi:10.3724/SP.J.1226.2014.00398
Yang, G., Li, Q., Guo, Y., Liu, H., Zheng, S., and Chen, M. (2022). Study on the mechanical properties and durability of recycled aggregate concrete under the internal curing condition. doi:10.3390/ma15175914
Yang, W., Liu, L., Wu, W., Zhang, K., Xiong, X., Li, C., et al. (2024). A review of the mechanical properties and durability of basalt fiber recycled concrete. Constr. Build. Mater 412, 134882. doi:10.1016/j.conbuildmat.2024.134882
Yang, Y., Xu, J., Zhan, B., Gao, P., Yu, Q., Li, R., et al. (2024). Study on hydration characteristics and mechanism of recycled powder-cement binary and multivariate systems. Constr. Build. Mater 420, 135646. doi:10.1016/j.conbuildmat.2024.135646
Yao, T., Tian, Q., Zhang, M., Qi, S., Wang, C., Ruan, M., et al. (2023). Experimental research on the preparation and properties of foamed concrete using recycled waste concrete powder. Constr. Build. Mater 407, 133370. doi:10.1016/j.conbuildmat.2023.133370
Yao, X., Pei, Z., Zheng, H., Guan, Q., Wang, F., Wang, S., et al. (2022). Review of mechanical and temperature properties of fiber reinforced recycled aggregate concrete. doi:10.3390/buildings12081224ER
Yildirim, S. T., Meyer, C., and Herfellner, S. (2015). Effects of internal curing on the strength, drying shrinkage and freeze–thaw resistance of concrete containing recycled concrete aggregates. Constr. Build. Mater 91, 288–296. doi:10.1016/j.conbuildmat.2015.05.045
Yildizel, S. A., Uzun, M., Arslan, M. A., and Ozbakkaloglu, T. (2024). The prediction and evaluation of recycled polypropylene fiber and aggregate incorporated foam concrete using Artificial Neural Networks. Constr. Build. Mater 411, 134646. doi:10.1016/j.conbuildmat.2023.134646
Yu, Y., Wang, J., Wang, N., Wu, C., Zhang, X., Wang, D., et al. (2021). Combined freeze-thaw and chloride attack resistance of concrete made with recycled brick-concrete aggregate. doi:10.3390/ma14237267
Yuan, S., Li, K., Luo, J., Yin, W., Chen, P., Dong, J., et al. (2024). Research on the frost resistance performance of fully recycled pervious concrete reinforced with fly ash and basalt fiber. J. Build. Eng. 86, 108792. doi:10.1016/j.jobe.2024.108792
Yuan, X., Dai, M., Li, M., and Liu, F. (2023). Study of the freeze–thaw resistance for composite fiber recycled concrete with sulphate attack exposure. doi:10.3390/buildings13041037ER
Zaid, O., and Zamir Hashmi, S. R. (2021). Experimental study on mechanical performance of recycled fine aggregate concrete reinforced with discarded carbon fibers. Front. Mater 8.
Zhang, H., Ji, T., Zeng, X., Yang, Z., Lin, X., and Liang, Y. (2018). Mechanical behavior of ultra-high performance concrete (UHPC) using recycled fine aggregate cured under different conditions and the mechanism based on integrated microstructural parameters. Constr. Build. Mater 192, 489–507. doi:10.1016/j.conbuildmat.2018.10.117
Zhang, H., Luo, G., Bao, J., Zhang, P., Lv, H., Li, Y., et al. (2024). Improving the salt frost resistance of recycled aggregate concrete modified by air-entraining agents and nano-silica under sustained compressive loading. Case Stud. Constr. Mater 20, e03170. doi:10.1016/j.cscm.2024.e03170
Zhang, W., Liu, H., and Liu, C. (2022). Impact of rice husk ash on the mechanical characteristics and freeze–thaw resistance of recycled aggregate concrete. doi:10.3390/app122312238ER
Zhang, Y., Zhou, G., and Zheng, Y. (2023). Effect of fiber type on the mechanical properties and durability of hardened concrete. J. Mater Sci. 58, 16063–16088. doi:10.1007/s10853-023-09021-1
Zhao, H., Wang, S., Wang, R., Shen, L., and Wang, Q. (2023). Utilization of raw coal gangue as coarse aggregates in pavement concrete. Constr. Build. Mater 378, 131062. doi:10.1016/j.conbuildmat.2023.131062
Zheng, C., Li, S., Hou, Y., and Jin, B. (2022). Frost resistance of fiber-reinforced self-compacting recycled concrete 61, 711–725. doi:10.1515/rams-2022-0269
Zheng, M., Zhang, L., and Feng, Y. (2024). A review on silane and siloxane materials: enhancing durability of cementitious materials through surface treatments. J. Mater Sci. 59, 10119–10139. doi:10.1007/s10853-024-09771-6
Zheng, Y., Zhuo, J., and Zhang, P. (2021). A review on durability of nano-SiO2 and basalt fiber modified recycled aggregate concrete. Constr. Build. Mater 304, 124659. doi:10.1016/j.conbuildmat.2021.124659
Zhu, P., Hao, Y., Liu, H., Wang, X., and Gu, L. (2020). Durability evaluation of recycled aggregate concrete in a complex environment. J. Clean. Prod. 273, 122569. doi:10.1016/j.jclepro.2020.122569
Keywords: recycled concrete, frost resistance, freeze-thaw cycle, influencing factors, improvement measures
Citation: Ma Q, Duan Z, Wang J, Yin G and Li X (2024) Frost resistance and improvement techniques of recycled concrete: a comprehensive review. Front. Mater. 11:1493191. doi: 10.3389/fmats.2024.1493191
Received: 08 September 2024; Accepted: 30 September 2024;
Published: 28 October 2024.
Edited by:
Yang Zou, Chongqing Jiaotong University, ChinaReviewed by:
Yang Yu, University of New South Wales, AustraliaSalvatore Verre, University of eCampus, Italy
Copyright © 2024 Ma, Duan, Wang, Yin and Li. This is an open-access article distributed under the terms of the Creative Commons Attribution License (CC BY). The use, distribution or reproduction in other forums is permitted, provided the original author(s) and the copyright owner(s) are credited and that the original publication in this journal is cited, in accordance with accepted academic practice. No use, distribution or reproduction is permitted which does not comply with these terms.
*Correspondence: Quan Ma, MTkxMTY3MkB0b25namkuZWR1LmNu