- 1Center for Biological Physics and School for Engineering of Matter, Transport, and Energy, Arizona State University, Tempe, AZ, United States
- 2Molecular Foundry, Lawrence Berkeley National Laboratory, Berkeley, CA, United States
- 3Physical Sciences Division, Pacific Northwest National Laboratory, Richland, WA, United States
- 4Department of Materials Engineering, University of British Columbia, Vancouver, BC, Canada
Editorial on the Research Topic
Advanced in situ characterization of biological interfaces and materials
Rapid changes are occurring on this dynamic planet–in 2020 anthropogenic mass was reported to not only match but exceed the mass of natural origins (Elhacham et al., 2020). These indications do not bold well for humanity in the coming generations, as we face 2 degree overall temperature increases over the next 5 years, CO2 is spewing into the atmosphere at the Gigatonne (Gt) rate per year without any signs of mitigation in the foreseeable future, ocean water levels are rising to incredible levels, drinking water availability is disappearing, humanity is heavily reliant on the “drill-and-fill” culture, and two wars are currently being fought in Gaza and Ukraine (Carr et al., 2024). And even with these pressures, humanity continues to eke out impressive scientific and technological achievements in the recent past including mRNA vaccines to counter a global pandemic (Hogan and Pardi, 2022) and the development of CRISPR-Cas9 drugs (Parums, 2024). All these developments are only possible from the incremental methodological improvements taking place presently.
A few take-home messages from the 24th American Conference on Crystal Growth and Epitaxy West Meeting at Fallen Leaf Lake, CA this past June, include a need to address issues of sustainability and a session on “Environmental and Energetic Materials.” These ideas have not fallen off by the side of the road, but must be fully integrated into our own research programs in our own labs on a daily basis. There is an unfavorable evidence that if our society does not mitigate the emission of greenhouse gasses by 2030 significantly, we will lose the opportunity to rein in any impact on these climate effects occurring all around the world (Masson- et al., 2021). Here in this Research Topic, we have gathered some papers of relevance that demonstrate the importance of materials in this discussion on the climate and sustainability on Earth and beyond.
In this Research Topic, current state-of-the-art uses of one of the most abundant minerals CaCO3 on this planet to address climate change is discussed. From utility in carbon capture to the formation of construction materials and seed coats for agricultural applications, CaCO3 still has many “tricks” up its sleeves as a target material for sustainability and climate sensitive applications (Figure 1). Levey et al. examine mechanisms of making CaCO3 stronger through lithification processes. Part of these processes can be better understood by investigating the constituents that lead up to the formation of CaCO3 mineral before nucleation, at the molecular level. Bewernitz et al. and Bewernitz et al. discovered that a measurable liquid condensed phase (LCP) can be formed with a local concentration of bicarbonate ions, leading to questions about the existence of precursor phases that appear before CaCO3 nucleation and crystal growth. These liquid-liquid phase separations have been recently “all the rage” in the fields of molecular and cell biology due to their surprise abundance in cells, but have been initially discovered by materials scientists a few decades before as amorphous materials have been known to materials chemists and physicists for some time (Hyman et al., 2014) (Figure 2).
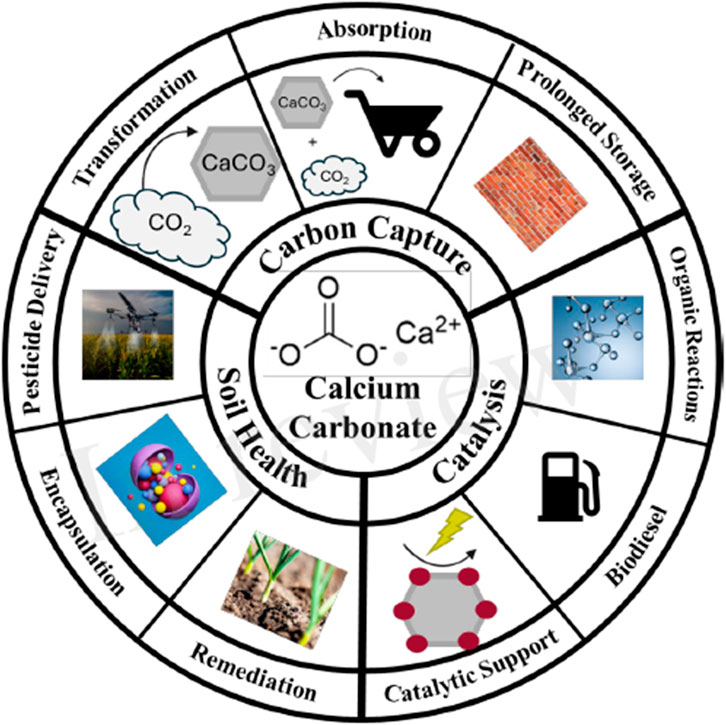
Figure 1. Current state of the art utilization of CaCO3 based materials in industries (Comes et al., 2024).
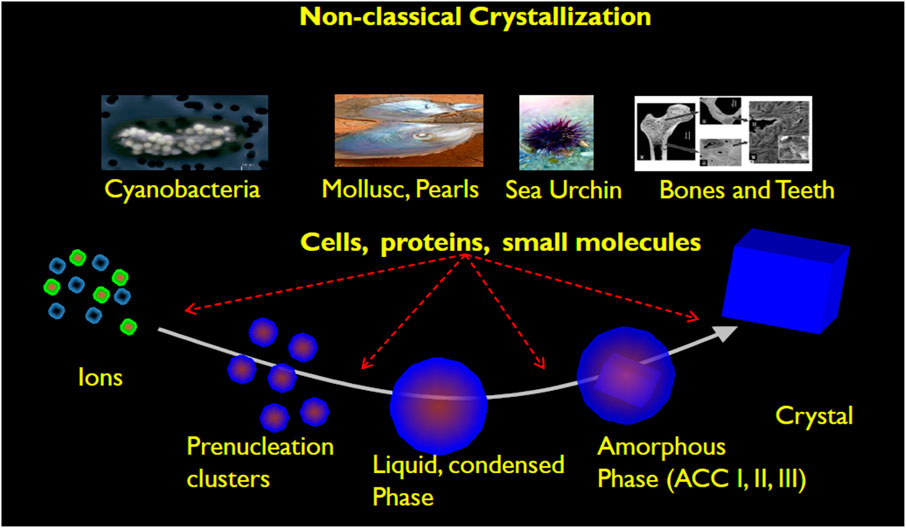
Figure 2. From the various biomineralization models, various precursors and intermediate states exist to intercalate across length-scales to affect pathways of mineralization in Ca-based minerals (Levey et al.; Bewernitz et al.; Bewernitz et al.; Hyman et al., 2014; Seto et al., 2013; Seto et al., 2014; Mergo and Seto, 2020).
All this CaCO3 mineralization brings about the question of whether these materials can be utilized extra-terrestrially as methods for built environments on Lunar and Martian surfaces. Zuo et al. examines the ability to build infrastructure with CaCO3 based bricks made from microbially induced carbonate precipitation (MICP), a variant of enzymatically induced carbonate precipitation (EICP) developed by Edward Kavazanjian and coworkers at the Center of Bio-mediated and Bio-inspired Geotechnics (CBBG), Arizona State University (Almajed et al., 2019). In our own works on CaCO3 mineralization, there will be many more methods developed to manipulate CaCO3 minerals for many applications (Seto et al., 2013; Seto et al., 2014; Mergo and Seto, 2020). As shown in the figure below, there are many interfaces where CaCO3 precursors can interact with to alter mineralization pathways (Figure 2). We only show a few of the numerous applications of CaCO3 utilized, whereby with subsequent methodological developments and improvements these applications will increase exponentially in the following years.
As we witness the next 5 years of advances in science and engineering, many of these new developments will be in the area of in situ methodologies. Many of these improvements will most likely be developed from the materials sciences and materials chemistry communities before spreading into the general scientific community. This was observed for methods like cryo-electron microscopy and micro-electron diffraction techniques which are now widely used in biomedical research (Henderson et al., 1990; Nannega and Gonen, 2019). We envision similar trends in the development of methodology will occur with other in situ methodologies too. Perhaps we will be seeing more in situ (fast scanning) atomic force microscopy, 3D fast force mapping, chemical spectroscopy and microcalorimetry work in the biological sciences in the near future.
Author contributions
JS: Conceptualization, Data curation, Formal Analysis, Funding acquisition, Investigation, Methodology, Project administration, Resources, Supervision, Validation, Visualization, Writing–original draft, Writing–review and editing. BR: Conceptualization, Data curation, Formal Analysis, Funding acquisition, Investigation, Methodology, Project administration, Resources, Software, Supervision, Validation, Visualization, Writing–original draft, Writing–review and editing. JT: Conceptualization, Data curation, Formal Analysis, Funding acquisition, Investigation, Methodology, Project administration, Resources, Software, Supervision, Validation, Visualization, Writing–original draft, Writing–review and editing. RW: Conceptualization, Data curation, Formal Analysis, Funding acquisition, Investigation, Methodology, Project administration, Resources, Software, Supervision, Validation, Visualization, Writing–original draft, Writing–review and editing.
Funding
The author(s) declare that financial support was received for the research, authorship, and/or publication of this article. The authors would like to acknowledge the support of Arizona Biomedical Research Centre New Investigator grant (NIA-RFGA2022-002) awarded to JS.
Acknowledgments
JT also acknowledges support by the US Department of Energy (DOE), Office of Science, Office of Basic Energy Sciences (BES) under an award FWP 80124 at Pacific Northwest National Laboratory (PNNL). PNNL is multi-program national laboratory operated for DOE by Battelle under Contracts No. DE-AC05-76RL01830.
Conflict of interest
The authors declare that the research was conducted in the absence of any commercial or financial relationships that could be construed as a potential conflict of interest.
The author(s) declared that they were an editorial board member of Frontiers, at the time of submission. This had no impact on the peer review process and the final decision.
Publisher’s note
All claims expressed in this article are solely those of the authors and do not necessarily represent those of their affiliated organizations, or those of the publisher, the editors and the reviewers. Any product that may be evaluated in this article, or claim that may be made by its manufacturer, is not guaranteed or endorsed by the publisher.
References
Almajed, A., Tirkolaei, H. K., Kavazanjian, Edward., and Hamdan, N. (2019). Enzyme induced biocemented sand with high strength at low carbonate content. Sci. Rep. 9 (1135), 1–7.
Carr, R., Kotz, M., Pichler, P. P., Weisz, H., Belmin, C., and Wenz, L. (2024). Climate change to exacerbate the burden of water collection on women's welfare globally. Nat. Clim. Chang. 14, 700–706. doi:10.1038/s41558-024-02037-8
Comes, J., Islamovic, E., Lizandara-Pueyo, C., and Seto, J., Improvements in the utilization of calcium carbonate in promoting sustainability and environmental health. Front. Chem., 2024, 12:1472284, doi:10.3389/fchem.2024.1472284
Elhacham, E., Ben-Uri, L., Grozovski, J., Bar-On, Y. M., and Milo, R. (2020). Global human-made mass exceeds all living biomass. Nature 588, 442–444. doi:10.1038/s41586-020-3010-5
Henderson, R., Baldwin, J., Ceska, T., Zemlin, F., Beckmann, E., and Downing, K. (1990). Model for the structure of bacteriorhodopsin based on high-resolution electron cryo-microscopy. J. Mol. Biol. 213 (4), 899–929. doi:10.1016/s0022-2836(05)80271-2
Hogan, M., and Pardi, N. (2022). mRNA vaccines in the COVID-19 pandemic and beyond. Ann. Rev. Med. 73, 17–39. doi:10.1146/annurev-med-042420-112725
Hyman, A., Weber, C., and Juelicher, F. (2014). Liquid-liquid phase separation in biology. Ann. Rev. Cell Dev. Biol. 30, 39–58.
Masson-Delmotte, V., Zhai, P., and Pirani, A. (2021). “IPCC sixth assessment report: the physical science basis,” in Intergovernmental panel on climate change (IPCC). Editor U. Nations (Cambridge, UK: Cambridge University Press).
Mergo, J. S. J., and Seto, J. (2020). On simulating the formation of structured, crystalline systems via non-classical pathways. Front. Mater 7 (75), 1–11. doi:10.3389/fmats.2020.00075
Nannega, B., and Gonen, T. (2019). The cryo-EM method microcrystal electron diffraction (MicroED). Nat. Meth 16, 369–379. doi:10.1038/s41592-019-0395-x
Parums, D., Editorial: First regulatory approvals for CRISPR-cas9 therapeutic gene editing for sickle cell disease and transfusion-dependent β-thalassemia. Med. Sci. Mon., 2024. 30(e944204): p. 1–4. doi:10.12659/msm.944204
Seto, J., Azais, T., and Coelfen, H. (2013). Formation of aragonitic layered structures from kaolinite and amorphous calcium carbonate precursors. Langmuir 29 (24), 7521–7528. doi:10.1021/la400442j
Keywords: Ca-based mineralization, CaCO3, non-classical crystallization, CO2 sequestration and utilization, sustainability challenges
Citation: Seto J, Rad B, Tao J and Wang R (2024) Editorial: Advanced in situ characterization of biological interfaces and materials. Front. Mater. 11:1472269. doi: 10.3389/fmats.2024.1472269
Received: 29 July 2024; Accepted: 21 October 2024;
Published: 07 November 2024.
Edited and reviewed by:
Hafiz M. N. Iqbal, Monterrey Institute of Technology and Higher Education (ITESM), MexicoCopyright © 2024 Seto, Rad, Tao and Wang. This is an open-access article distributed under the terms of the Creative Commons Attribution License (CC BY). The use, distribution or reproduction in other forums is permitted, provided the original author(s) and the copyright owner(s) are credited and that the original publication in this journal is cited, in accordance with accepted academic practice. No use, distribution or reproduction is permitted which does not comply with these terms.
*Correspondence: Jong Seto, am9uZy5zZXRvQGFzdS5lZHU=