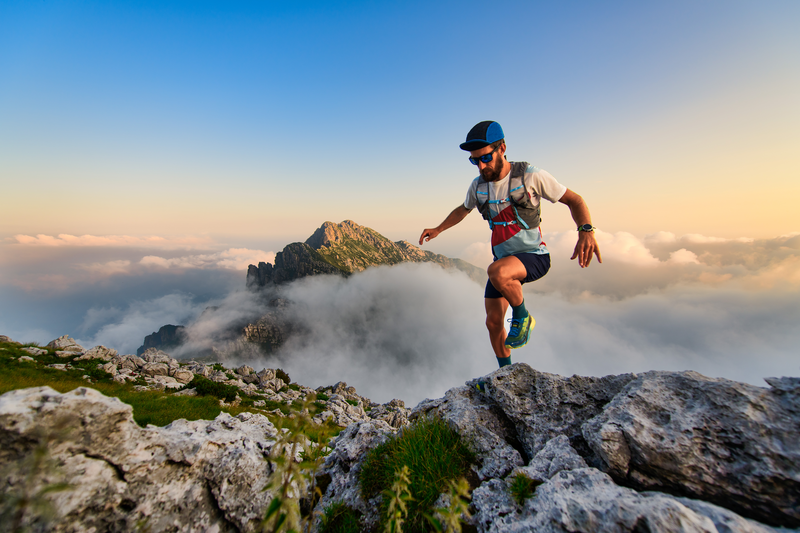
94% of researchers rate our articles as excellent or good
Learn more about the work of our research integrity team to safeguard the quality of each article we publish.
Find out more
REVIEW article
Front. Mater. , 26 February 2024
Sec. Biomaterials and Bio-Inspired Materials
Volume 11 - 2024 | https://doi.org/10.3389/fmats.2024.1382014
Non-renewable nature and continuously increased consumptions of bitumen is a serious challenge for the development of infrastructure, especially for the constructions and maintenances of asphalt pavements. To cope with this situation, some sustainable strategies are needed. Extensive efforts have been made to improve the resilience, sustainability and circularity of asphalt pavements. Among them, the utilisation of bio-based materials is one of the most promising measures. This paper systemically reviewed the state-of-the-art knowledge in the development of bio-bitumen. Wood-based oils, waste cooking oils and manure-based bio-bitumen were selected as the review objectives. It was revealed that all bio-based oils currently being used are more suitable for serving as additives like softeners, rejuvenators, or modifiers rather than as alternative of binders. One of the most promising utilisations of bio-oils is as rejuvenators, potentially antioxidants as well. Incorporating polymers with bio-oils is another feasible practice to improve the performance of bio-bitumen. Causation should be taken when producing bio-based bitumen since compatibility might have some kind of influence on the performance and this issue should be addressed carefully.
Bitumen, a by-product of petroleum refining, has attracted attention since its inception. Its applications have gradually expanded with the development of people’s understanding and technological progress. To date, asphalt has become a crucial raw material in industries such as road construction, building, roof waterproof. A significant amount of bitumen produced globally each year is consumed in the construction and maintenance of roads, confirming its dominant role in bitumen consumption within the construction industry. However, the non-renewable nature of bitumen, combined with the decreasing global reserves of petroleum and the complex external factors, has led to consistently high bitumen prices. This current situation has created a substantial impact on the road construction industry, where the demand for asphalt pavement construction and maintenance is expanding while bitumen consumption is on the rise. The intensifying contradiction between supply and demand has made it imperative to seek alternatives to petroleum bitumen that are green, renewable, have a stable supply, and are cost-effective.
In recent decades, bio-oil has garnered widespread attention from researchers due to its advantages such as abundant feedstock, strong renewability, and low cost. Bio-oil is a liquid product derived from biomass (such as wood, agricultural waste, animal manure, and waste cooking oil) through processes like gasification pyrolysis, hydrothermal liquefaction (Suwarto et al., 2023a), plasma electrolytic liquefaction (Hu et al., 2022), and other methods. The primary elemental composition of bio-oil and petroleum bitumen includes carbon (C), hydrogen (H), oxygen (O), and nitrogen (N). Additionally, both possess certain bonding and viscoelastic properties. Scholars generally consider bio-oil as a potential organic binder material for road surfaces, with the possibility of partially or entirely replacing petroleum bitumen.
Among various biomass sources, wood-based biomass is abundant, with various types of timber, discarded wood products, sawdust, etc., serving as reliable sources for pyrolysis oil. These materials can be locally sourced. Scholars have studied the physicochemical properties of various wood-based bio-oils and explored their application as additives in bitumen performance modification, rejuvenation of aged bitumen, and improvement of bitumen ageing resistance. Some research achievements have been made in this regard.
However, challenges such as high content of lightweight and volatile components, poor high-temperature deformation resistance, and high sensitivity to ageing limit the current application effectiveness. The substitution rate of bio oil for petroleum bitumen remains limited, and overcoming numerous technical barriers is necessary before achieving complete replacement of petroleum bitumen.
Wood-based bio-oil is produced by chemically treating wood-based biomass (logs, sawdust, and by-products of various wood processing) to depolymerise organic polymers like cellulose, hemicellulose, and lignin. The resulting intermediate products undergo a series of physical processes (evaporation, condensation, etc.) and chemical changes (polymerization, esterification, etc.) to form a dark, non-Newtonian liquid with a certain viscosity. Currently, the main methods for preparing bio-oil include gasification pyrolysis, hydrothermal liquefaction and plasma electrolytic liquefaction (Ding et al., 2021). Due to the high similarity between gasification pyrolysis systems and existing petroleum refining systems, gasification pyrolysis has become a significant means of biomass oil preparation.
Based on the residence time of the gas generated during pyrolysis, gasification pyrolysis can be classified into regular thermal cracking, fast thermal cracking, flash thermal cracking, catalytic thermal cracking, and mixed thermal cracking. Since fast thermal cracking yields a higher bio-oil production rate and can meet the substantial demand for binding materials in road infrastructure construction and maintenance, the predominant biomass pyrolysis method currently used in road engineering is fast thermal cracking (Suwarto et al., 2023b).
Fast pyrolysis refers to a technique in which dried biomass is heated in an oxygen-free environment at an extremely high heating rate (above 1,000°C·s-1) to temperatures ranging from 400°C to 550°C. The process involves rapid cracking with an extremely short gas residence time (usually less than 2 s). This method typically offers advantages such as mild reaction conditions (temperature, pressure, etc.), high liquid yield (around 70%), short processing time, strong adaptability, quick response, and ease of transportation and storage of the resulting products.
Due to wood being one of the easily accessible renewable natural resources, current research on bio-bitumen often emphasises wood as a crucial feedstock for preparing bio-oil through pyrolysis. Documented wood sources for road-grade bio-oil in the literature include pine wood (Mohammad et al., 2013; Stankovikj et al., 2016; He et al., 2019; Tahir et al., 2020; Park et al., 2022), cypress (Zhou et al., 2020), willow wood (He et al., 2019), poplar wood (Li et al., 2021), Michigan wood (Yang et al., 2017), oak wood (Raouf and Williams, 2010), fir (Pahlavan et al., 2022), birch (Pahlavan et al., 2022), Japanese cedar (Yang and Suciptan, 2016), and some unspecified types of wood and sawdust (Yang et al., 2013; Yang et al., 2014; Bao et al., 2020; Zhou et al., 2021; Hu et al., 2023).
Similar to petroleum asphalt, wood-based bio-oil is primarily composed of the elements C (carbon), H (hydrogen), O (oxygen), and N (nitrogen). The specific elemental composition may vary depending on the source of the biomass, but generally, the sum of C, H, and O exceeds 95% of the total bio-oil composition. Numerous studies indicate that, in comparison to petroleum bitumen, wood-based bio-oil typically exhibits higher oxygen content, lower carbon content, and a lower H/C ratio (saturation). Scholars widely consider the elevated oxygen content as one of the key reasons for the susceptibility of bio-oil to ageing (Omairey et al., 2024).
In reality, the major components of wood, namely, cellulose, hemicellulose, and lignin, undergo pyrolysis or depolymerisation reactions to form fragments that serve as the main molecular sources of wood-based bio-oil. The oxygen elements present in these precursor compounds persist in various product compounds after pyrolysis, contributing to the high oxygen content in bio-oil. Additionally, the abundance of ether bonds, carbonyl groups, and aromatic hydrocarbons in cellulose, hemicellulose, and lignin is a significant factor contributing to the lower H/C ratio in bio-oil (Mohan et al., 2006).
Bio-oil obtained from the pyrolysis of wood materials is a complex mixture of water, soluble organic compounds, and insoluble organic compounds. Based on the characteristic functional groups of these compounds, they can be roughly categorised into acids, alcohols, aldehydes, esters, ketones, phenols, guaiacols, eugenol, sugars, furans, alkenes, aromatics, nitrogen compounds, and other low-concentration oxygenated compounds. These compounds exhibit a wide range of molecular weights, contributing to the diversity of bio-oil composition (Stankovikj and Garcia-Perez, 2017). According to incomplete statistics, there are over 400 identified types of compounds in bio-oil, and due to current technological limitations, this figure does not represent the complete spectrum of compounds present in bio-oil. In summary, determining the complete chemical composition of bio-oil is challenging (Stankovikj et al., 2016).
In addition to the diverse array of organic compounds, water is also a significant component of wood-based bio-oil, with its content typically ranging from 20 to 40 wt% (Oasmaa et al., 2010). As is well known, moisture can have a series of detrimental effects on the performance of asphalt and asphalt mixtures. Therefore, untreated bio-oil with high water content cannot be blended with petroleum asphalt to produce bio-asphalt, and it cannot directly replace petroleum asphalt. Moreover, bio-oil with high water and acidic content tends to have poor storage stability (Xu et al., 2022). As a result, researchers have conducted extensive studies on water separation from bio-oil.
Research indicates that water tends to extract light components and polar substances from bio-oil. These polar substances typically include carboxylic acids, sugars, ketones, alcohols, and ethers, with small amounts of phenolic compounds also present. The reduction of light components leads to an increase in the viscosity of bio-oil. Additionally, the removal of certain carboxylic acids, such as larixic acid, butanoic acid, 2-propenyl ester, and butanoic acid anhydride, results in low acidity in the treated oil (Yoosuk et al., 2014).
Based on the different boiling points of various components in bio-oil, Capunitan et al. (Capunitan and Capareda, 2013)conducted distillation on bio-oil derived from corn stover. By controlling the distillation temperature range, they successfully concentrated water and light components from bio-oil into low-boiling fractions. In addition to effectively separating water from bio-oil, distillation can concentrate most of the acidic substances in bio-oil into intermediate boiling fractions, which is beneficial for reducing acidity in light and heavy fractions. Raouf et al. (Raouf and Williams, 2010)heated oak pyrolysis oil at 110°C for 2 h to reduce water and light component content in bio-oil. Yang et al. (Yang and You, 2015)found that untreated waste wood bio-oil had a high water content of 15%–30%, but after dehydrating bio-oil at 110°C, the water content was effectively reduced to 5%–8%. It is important to note that during distillation, the temperature needs to be controlled, especially for bio-oils with high contents of compounds such as aldehydes, phenols, and furfural, as these components are prone to thermal coking, which is a significant factor leading to low total recovery rates during distillation (Capunitan and Capareda, 2013).
Vacuum distillation is an effective method to avoid severe ageing of bio-oil due to high-temperature heating. It allows the evaporation of high-boiling substances at lower temperatures compared to atmospheric pressure distillation. However, faster evacuation of vapor during reduced pressure conditions may lead to a higher loss of some compounds, resulting in a lower yield for vacuum distillation (Capunitan and Capareda, 2013).
Researchers typically refer to bio-oil that has undergone technical measures to remove water as “bio binder” (treated bio-oil). After separating water from bio-oil through technical measures, the treated bio-oil differs significantly from the original product bio-oil. The most notable change is the significant reduction in oxygen content and a significant increase in carbon content (Capunitan and Capareda, 2013), as a significant portion of the oxygen in bio-oil exists in the form of water. Despite the successful removal of water from bio-oil, bio binder still has a complex compound composition. Therefore, researchers often adopt a four-component analysis method similar to petroleum bitumen to simplify the analysis, dividing bio binder into saturated fraction, aromatic fraction, resin, and asphaltene. Compared to petroleum bitumen, wood-based bio binder usually contains only trace amounts of saturated fractions, and some studies even suggest that bio binder may not contain any saturated fractions (Kabir et al., 2020). This characteristic is closely related to the lower H/C ratio of bio binder. In addition to the low asphaltene content, the asphaltene content in bio binder is usually lower than that in petroleum bitumen, but its resin fraction and aromatic fraction content are typically higher. These significant differences in composition are among the important factors causing the rheological differences between bio-oil and petroleum bitumen.
Bio-binder possesses a chemical composition and rheological characteristics similar to petroleum bitumen, making it reasonable to explore the feasibility of completely replacing petroleum bitumen with bio-binder. If a suitable wood-based bio-binder can be found to replace petroleum bitumen as the main binding material for flexible road surfaces, it could revolutionize sustainable road infrastructure. Therefore, many researchers have conducted studies on the feasibility of directly replacing petroleum bitumen with bio-binder.
The properties of binders are crucial for the performance of mixtures and road structures. To ensure good serviceability of asphalt roads, without premature failure of the binder, various fundamental or innovative performance indicators have been proposed by road construction workers from various perspectives. These performance indicators limit the possibility of premature failure of petroleum bitumen in terms of high temperature, low temperature, fatigue, ageing, and other aspects. Since research on bio-binder in the field of road construction materials started relatively late, studies on the road performance of bio-binder have mostly relied on well-established research equipment and methods used for petroleum bitumen.
Although there are few examples of using wood-based bio-binder directly as a binding material in road engineering, numerous indoor studies have revealed various shortcomings of wood-based bio-binder as a binding material for flexible road surfaces. It is reasonable to conclude that the performance of bio-binder, especially pure bio-binder, is not sufficient to completely replace petroleum bitumen.
Wood-based bio-binder typically has lower viscosity, and the temperature range of its viscous behaviours may be lower than that of bitumen by about 30°C–40°C (Raouf et al., 2010). Additionally, bio-binder’s viscosity exhibits higher temperature sensitivity and shear rate sensitivity, which may lead to shear thinning (Raouf and Williams, 2010). Studies have found that even with reasonable upgrades to Oak wood-based bio-binder, its dynamic shear modulus, rutting factor, and elastic recovery rate are relatively low, and the High Temperature Performance Grade of the virgin bio-binder is less than 40°C. To address the poor high-temperature performance of bio-binder, researchers have adopted methods from the modification of petroleum bitumen and blended common modifiers such as SBS, rubber particles (Peralta et al., 2012), and a terpolymer (POLIMUL) (Espinosa et al., 2021a) with bio-binder to enhance its high-temperature stability. Research indicates that a higher content of light components in bio-binder is favourable for the full swelling and uniform distribution of modifiers such as SBS and rubber particles. Adding appropriate modifiers can effectively improve the high-temperature performance grade of bio-binder. Therefore, the use of modifiers may be one of the important technologies for achieving the complete replacement of petroleum asphalt with bio-binder in the future.
It is worth noting that Espinosa et al. reported a field study that compared the structural evaluation (FWD) and roughness test (IRI) of regular petroleum-based asphalt pavement (HMA) and bio-binder flexible pavement (BM) during the 3-year operation period after construction (Espinosa et al., 2021a). The test results showed that the performance of BM was comparable to that of HMA. This study effectively demonstrated the possibility of using appropriate modifiers to enhance the potential for bio-binder to replace petroleum bitumen.
During pavement construction and operation, binders undergo continuous ageing. Therefore, in addition to the performance of newly produced bio-binder, the changes in performance after ageing (such as low-temperature performance and fatigue characteristics) are also decisive factors in determining whether it can replace petroleum bitumen. Espinosa et al. (Espinosa et al., 2021b)compared the master curves of dynamic shear modulus for pine wood resin bio-binder and AC 30/45 petroleum bitumen before and after short-term aging (RTFOT). The results showed that whether at lower frequencies (higher temperatures) or higher frequencies (lower temperatures), the increase in dynamic shear modulus after ageing was higher for bio-binder than for petroleum bitumen. Moreover, the dynamic shear modulus of aged bio-binder was higher than that of aged petroleum bitumen (unaged bio-binder had a lower dynamic shear modulus than unaged petroleum bitumen). Multiple stress creep recovery test results also indicated that aged bio-binder had lower unrecoverable creep compliance and higher elastic recovery rate (Espinosa et al., 2021a). These changes suggest that bio-binder is more “brittle and stiff” after ageing, and its elastic characteristics become more pronounced. Although ageing to some extent improves its high-temperature performance, it has a more serious adverse effect on the fatigue resistance of bio-binder (Camargo et al., 2018).
The susceptibility of bio-binder/bio-oil to ageing is closely related to the higher content of light components in its material composition (Castro-Alonso et al., 2023). It was also found that bio-binder contains a significant amount of volatile light components, with volatile components reaching 40 wt% at 290°C. GC-MS testing showed that the molecular weight of the volatile components was below 250 g mol⁻1, and the volatile residues exhibited apparent brittleness (Hu et al., 2024). The higher content of light components in bio-binder results in a significantly lower number-average molecular weight (Mn), weight-average molecular weight (Mw), and z-average molecular weight (Mz) compared to petroleum bitumen. In addition, researchers generally believe that the higher oxygen content in bio-binder is another important reason for its susceptibility to aging. However, beyond this, few researchers have systematically studied the changes in functional groups, components, and colloidal structures of bio-binder during aging. As a result, there is currently no comprehensive and clear understanding of bitumen ageing at multiple scales.
Although bio-binder can be comparable to or even surpass petroleum bitumen in certain properties, there are currently few bio-binders that meet the requirements of the evaluation system for petroleum bitumen, making them satisfactory substitutes for petroleum bitumen. Therefore, the most common application of wood-based bio-binder in road engineering is as a modifier, partially replacing petroleum bitumen. The mixture of bio-oil and petroleum bitumen is commonly referred to as bio-bitumen.
Similar to polymer-modified petroleum bitumen, the good compatibility between bio-oil and petroleum bitumen (forming a homogeneous and stable system) is the foundation for bio-bitumen to exhibit qualified road performance. Yang et al. used the Automatic Flocculation Titration (AFT) method to evaluate the molecular compatibility of Michigan wood-based bio-binder and petroleum bitumen from the perspective of miscibility (Yang et al., 2017). The test results indicated that in bio-bitumen with 2% bio-oil content, bio-oil and petroleum bitumen were stable and compatible. However, it is speculated that with an increase in bio-oil content, the compatibility between bio-oil and petroleum bitumen will decrease. Unfortunately, there are no tests for bio-bitumen with higher bio-oil content.
Yang et al. employed the Standard Practice for Determining the Separation Tendency of Polymer from Polymer Modified Asphalt (ASTM D7131) to characterise the potential separation of bio-binder and petroleum bitumen within bio-bitumen by the difference in rutting factors between the upper and lower parts of the bio-bitumen specimen (Yang and Suciptan, 2016). The experimental results showed that when the bio-binder content in bio-bitumen reached 50%, there was a significant difference in rutting factors between the upper and lower parts of the specimen, indicating reduced compatibility. Zhang et al. (Castro-Alonso et al., 2023) analysed the thermal storage stability of bio-bitumen with different high bio-binder contents (10%, 15%, 20%, 25%, and 30%) from the perspectives of softening point, Brookfield viscosity, and rheological properties. The test results showed that compared to the base bitumen, bio-bitumen had lower thermal storage stability. There were significant differences in softening points, Brookfield viscosity, and rutting factors between the upper and lower parts of the specimen after thermal storage, indicating poor thermal storage stability. Moreover, these differences increased noticeably with higher bio-binder content and longer storage time.
In summary, there is limited research on the compatibility between petroleum bitumen and bio-binder. The judgment of “good compatibility” based on elemental composition, compound composition, and rheological characteristics lacks practical validation. Existing studies have confirmed that when the bio-binder content is relatively high in bio-bitumen, their compatibility is poor. Therefore, establishing multi-scale characterisation methods for the compatibility between petroleum bitumen and bio-oil and proposing technical measures to improve their compatibility are crucial for increasing the substitution rate of bio-oil for petroleum bitumen.
After blending, whether chemical reactions occur between bio-oil and petroleum bitumen, how the colloidal structure of bio-bitumen changes compared to that of petroleum bitumen, and how the colloidal structure of bio-binder changes compared to bio-oil are key to understanding the changes in the performance of bio-bitumen. Some researchers have explored whether chemical reactions occur after blending bio-oil with petroleum bitumen. Zhang et al. conducted a comparative analysis of the Fourier-transform infrared absorption spectra of petroleum bitumen, bio-bitumen, and bio-binder (Zhang et al., 2020). They found changes in absorption peaks corresponding to certain functional groups, such as a significant weakening or disappearance of the absorption peaks of phenolic compounds at 1,115 cm⁻1 and 1,270 cm⁻1, and varying degrees of weakening at 1,018 cm⁻1 and 3,374 cm⁻1. However, attributing these changes in functional groups solely to chemical reactions is difficult to determine, as changes in the concentration and volatility of relevant compounds (unavoidable during high-temperature blending) can also cause the observed changes in functional groups. Ingrassia et al. found that the addition of bio-oil altered the shape of the Fourier-transform infrared absorption spectra corresponding to sulfone groups, which might render sulfone unsuitable as an ageing characterisation indicator for bio-bitumen (Ingrassia et al., 2020). Considering possible physical factors, there is no apparent change in functional groups after blending bio-binder with petroleum bitumen, and these findings serve as strong evidence against chemical reactions occurring.
Therefore, researchers tend to attribute the performance changes after blending to changes in the colloidal structure/composition of binders. This is because bio-binder has a small content of saturated fractions and asphaltene compared to petroleum bitumen. Zhang et al. found that bio-bitumen with 15 wt% bio-oil content had significantly increased resin and aromatic fractions compared to the corresponding petroleum bitumen (PG 64–22). The saturated fraction and asphaltene decreased by 7.5% and 7.7%, respectively. Ingrassia et al. found that, compared to petroleum bitumen, bio-oil had a higher resin content, while other component contents were lower, and even the saturated fraction was absent. Through comparative analysis of the experimentally measured component composition and the mathematically calculated (estimated) component composition, the authors concluded that there was no chemical reaction between bio-oil and petroleum bitumen (Ingrassia et al., 2020).
The above research results show clear material dependencies, particularly regarding the characteristics of the selected petroleum bitumen. Therefore, adopting the performance grading of petroleum bitumen as a reference for bio-binder may be a more universally applicable research method. However, there are some obstacles to directly applying existing petroleum bitumen performance grading methods and equipment. Taking the SHARP program’s proposed high-temperature performance grading of petroleum bitumen as an example, when determining the high-temperature grade of bio-binder, the rutting factor after the Rolling Thin Film Oven Test (RTFOT) is one of the evaluation criteria. However, the current standard specifies an RTFOT test temperature of 163°C and a test time of 80 min. Numerous studies indicate that the upper temperature limit for heating bio-binder is typically between 120°C and 140°C, and the heating time does not exceed 30 min. Processing bio-binder under RTFOT test conditions would lead to severe ageing of the binder. Additionally, RTFOT specimens undergo significant carbonisation after undergoing Pressure Aging Vessel (PAV) aging tests, making it impossible to continue preparing samples. Clearly, the current high-temperature grading standards for petroleum bitumen are not suitable for direct application to the high-temperature performance grading of bio-binder.
Despite the significant ageing susceptibility of bio-binders due to higher volatile component content and elevated oxygen levels, research indicates that the oxidative ageing degree of bio-bitumen is noticeably lower compared to petroleum bitumen. Specifically, after ageing, bio-bitumen exhibits lower aging indices than petroleum bitumen, as calculated using parameters such as carbonyl index and activation energy. The ratio of polar to non-polar components is also lower in bio-bitumen. This suggests that, despite the intense ageing of bio-binders due to their higher volatile component content, they have a substantial inhibitory effect on the oxidative ageing process of petroleum bitumen. Therefore, studying the crucial role of specific phenolic compounds in the oxidative ageing process of bio-binder is beneficial for advancing the engineering application of bio-binder as an antioxidant for bio-bitumen.
Phenolic compounds have been confirmed to possess antioxidant properties by capturing free radicals generated during the oxidation process of petroleum bitumen, thereby deactivating the free radicals and terminating the chain reactions that lead to oxidation. Additionally, phenolic compounds moderate the aggregation effects during the ageing process, caused by an increase in intermolecular forces due to oxidation. Given that wood-based bio-binders contain a significant number of phenolic compounds, investigating the types of phenolic compounds present in bio-oil and their antioxidant effects under different oxidation conditions is a trending research direction.
Apart from phenolic compounds, carbonaceous particles in bio-oil can adsorb ultraviolet light and act as scavengers for some of the free radicals formed during asphalt ageing, effectively slowing down the photo-oxidative ageing process of bio-asphalt (Hosseinnezhad et al., 2020).
In addition to the specific reaction processes reported in the aforementioned studies, researchers have proposed more macroscopic mechanisms for the antioxidant properties of bio-bitumen. Mousavi et al. suggested that highly reactive components in bio-binder, such as α-tocopherol, can act as sacrificial agents to protect critical components in petroleum bitumen from oxidation (Mousavi et al., 2016). Less-reactive molecular species can serve as a protective shell for the easily oxidizable molecules in petroleum bitumen. Furthermore, bio-binder constituents are considerably less polarisable than bitumen molecules, indicating a lower propensity for these chemical species to develop new polar functionalities in the presence of oxidative agents.
It is essential to note that bitumen ageing mechanisms involve not only oxidation but also chain scission, aromatisation, carbonation, aggregation, and the loss of volatile components. Therefore, when characterising the anti-ageing effects of bio-oil in bio-bitumen using different indicators, the same bio-oil may exhibit significant differences in effects. Additionally, during the ageing process of bio-bitumen, the loss of light components due to volatilisation still occurs, and this phenomenon becomes more pronounced when the specimen is in film form or when the bio-binder content is higher (Ingrassia and Canestrari, 2022).
Vegetable oils are obtained through physical or chemical processes from various plants. Generally, there are two types of vegetable oils: fresh vegetable oil and waste vegetable oil. Actually, both fresh vegetable oil and waste vegetable oil are capable in modification of petroleum asphalt and rejuvenation of aged petroleum asphalt, the brief application principle is shown in Figure 1.
FIGURE 1. The application of vegetable oil-based asphalt (Conglin et al., 2023).
The primary components of vegetable oils consist of various fatty acids (FAs) and triglycerides of glycerol, with major fatty acids including palmitic acid, stearic acid, oleic acid, linoleic acid, and linolenic acid (Cong et al., 2019). The distinction between different vegetable oils lies in the composition of fatty acid complexes, and variations in fatty acid composition significantly influence the physicochemical properties of the oil, potentially influencing its behaviours in bitumen (Giakoumis, 2018; Kolawole et al., 2021).
Researchers have extensively investigated the applications of soybean oil and its derivatives in bitumen and asphalt mixtures (Portugal et al., 2018; Maxwell et al., 2021; Rezvan and Alireza, 2021). Soybean oil and its derivatives have been used in some ongoing experimental road sections, and their long-term performance under real environmental and traffic conditions has been evaluated (Ali et al., 2022). Sunflower seed oil can serve as an alternative to soybean oil, expanding the options for the use of vegetable oils in road engineering applications. High oleic vegetable oils, characterized by higher oleic acid content, demonstrate superior oxidative stability compared to traditional vegetable oils. It has been reported that the purity level of oleic acid in vegetable oils can influence the chemical, thermal, and rheological properties of vegetable oil-based polymers, such as glass transition temperature, viscosity, and thermal resistance (Houlei et al., 2021).
However, using fresh vegetable oil as a pavement binder is considered wasteful from any perspective, especially when considering that many regions globally are still grappling with hunger issues. Waste vegetable oil (WVO) is typically a by-product resulting from the frying or heating of fresh plant oil at high temperatures. It is non-edible, exhibiting a dark brown colour and a viscosity higher than that of fresh oil (Ramadhansyah et al., 2020). The compositional variations among WVOs are highly correlated with the source of WVO, the processing method employed, and the type of fried food. Due to the processes of frying and heating, hydrogenation and oxidation degradation occur in vegetable oils, transforming the fatty acid (FA) profile from saturated fatty acids (SFAs) and polyunsaturated fatty acids (PUFAs) to SFAs and monounsaturated fatty acids (MUFAs) (Omojola et al., 2019). Simultaneously, polyunsaturated fatty acids decompose into other compounds such as small molecules, e-aldehydes, trans-fatty acids, epoxy compounds, and others (Zixiang et al., 2020). Substantial differences in physicochemical properties exist between pure and waste oils, particularly in terms of viscosity, iodine value, and peroxide value.
Commonly used traditional bitumen rejuvenators are extracted from heavy oil to supplement the light components of aged asphalt. However, rejuvenators based on heavy oil tend to volatilize at high temperatures, significantly impacting the efficiency of rejuvenation. The primary components of fresh and waste vegetable oils are triglycerides, comprising unsaturated fatty acids, akin to the light oil fraction of bitumen, and exhibiting good temperature resistance (Ji et al., 2016). Due to their environmental friendliness, biodegradability, and cost-effectiveness, they can be classified as bio-rejuvenators (Hınıslıoğlu and Ağar, 2004), making them advantageous alternatives to traditional rejuvenators. Consequently, numerous scholars have conducted extensive research to explore the potential of using vegetable oils as bio-rejuvenators to restore the performance of aged asphalt binders.
The rejuvenation effect on bitumen primarily focuses on restoring rheological performance and improving low-temperature performance. Ji et al. (Ji et al., 2016), for instance, utilised two waste restaurant vegetable oils as substitutes for heavy oil and one commercially available rejuvenator in different proportions (based on asphalt weight) for the rejuvenation of aged asphalt extracted from reclaimed asphalt pavement (RAP) materials. Rheological properties of asphalt binders were evaluated using dynamic shear rheometer (DSR) and rotational viscosity (RV) tests. The low-temperature performance of recovered asphalt binders was assessed through bending beam rheometer (BBR) tests. Indoor experiments and analysis of variance (ANOVA) results indicated that vegetable oil rejuvenators effectively reduce the viscosity and stiffness of aged asphalt, benefiting both fatigue performance and low-temperature crack resistance.
Zhi et al. studied the microstructure of aged asphalt with varying waste vegetable oil content and rejuvenation time, found that the addition of waste vegetable oil effectively supplements the content of saturates and aromatics, inhibiting the oxidation of saturates and aromatics into asphaltene and resin (Zhi et al., 2021). The optimal rejuvenation time was 60 min, with a rejuvenator content of 7.0%. Zhang et al. (Zhang et al., 2018) evaluated the rejuvenation effect of waste soybean oil with different viscosities, characterised the basic performance of regenerated asphalt using three major indicators, and then assessed the impact of waste cooking oil (WCO) viscosity on the rheological properties of rejuvenated bitumen through rotational viscosity, rutting parameters, complex modulus and phase angle master curves, and creep recovery rate. Experimental results indicated that the three major indicators and rheological performance of aged asphalt can be recovered to a certain extent, but there is still a gap between the basic performance of WCO-rejuvenated bitumen and fresh bitumen. Both excessively high and low viscosity values of WCO adversely affect the performance recovery of rejuvenated bitumen. This study contributes to making appropriate choices for bio-rejuvenators in asphalt applications and promotes the practical application of bio-regenerators.
Zheng et al. investigated the potential reparative role of sunflower seed oil in asphalt materials using a dynamic shear rheometer (DSR) to evaluate the performance of asphalt binders (Zheng, 2019). The resulting master curve indicated that the addition of 5% sunflower seed oil could restore approximately 30% of the modulus loss in aged bitumen. Thus, the test results robustly demonstrate the potential of sunflower seed oil to fully restore the rheological performance and healing capacity of bitumen. The aforementioned studies collectively demonstrate that the type, content, and rejuvenation time of vegetable oils play crucial roles in recovering the performance of aged bitumen under different levels of rejuvenation.
On a microscopic level, aging leads to the oxidation of abundant benzyl and sulfuric compounds in bitumen, transforming them into carbonyl functional groups and sulfoxide functional groups. Regarding the apparent morphology of bitumen, the honeycomb structure of asphalt binder tends to disappear after ageing. It has been reported that the rejuvenated binder with the use of plant oil does not chemically interact with asphalt compounds; instead, asphalt compounds only supplement the light components of saturated and aromatic fractions. However, the addition of plant oil can inhibit the oxidation of benzyl and sulphur compounds, forming a transition from light to heavy components, effectively slowing down the aging of the binder (Zhi et al., 2021). Simultaneously, with the assistance of bio-rejuvenators, the honeycomb structure can be restored and uniformly distributed. Compared to traditional rejuvenators, bio-rejuvenators are more stable and less susceptible to high-temperature volatilisation, significantly enhancing the efficiency of rejuvenation (Suo et al., 2021).
It is worth noting that the penetration effect of WCO rejuvenator on aged asphalt significantly influences its regeneration effectiveness. Existing research has shown that when WCO is used as a rejuvenator to regenerate aged asphalt, a diffusion area without a distinct interface, as shown in Figure 2, is formed. Investigating the physicochemical properties of binder at different positions within this area is an important means of understanding the regeneration mechanism of WCO rejuvenators. Xiao used techniques such as interface image threshold analysis and tracer analysis, comprehensively studied the diffusion mechanism between bio-rejuvenators and aged bitumen at the microscopic level, establishing both microscopic and macroscopic relationships for a more comprehensive and in-depth understanding of plant oil-rejuvenated bitumen (Xiao et al., 2020).
FIGURE 2. Diagrammatic sketch for GC–MS analysis (Xiao et al., 2020).
Traditional bitumen struggles to meet the performance requirements of asphalt pavements under high traffic volume and extreme weather conditions. Therefore, polymers and additives are employed to enhance the performance of base bitumen, aiming to achieve better rheological properties and extend the pavement’s service life (Seidel and Haddock, 2014). Plant oil is considered an environmentally friendly alternative to traditional modifiers and has been investigated in bitumen modification.
The use of plant oil in asphalt modification can be categorised into three types: direct modification of base bitumen (plant oil directly added to base bitumen for modification), composite modification of bitumen with other materials (plant oil added to base bitumen, along with the addition of other materials such as plastics and rock bitumen (Somé et al., 2016), or waste plastics), and activation modification of other bitumen additives (plant oil used to activate bitumen additives to enhance their modification effects in bitumen).
Due to the similarity in chemical composition, plant oil and bitumen exhibit excellent compatibility (Ahmed and Hossain, 2020). Fatty acids in plant oil with a linear alkane structure can reduce the viscosity of base bitumen, improving its resistance to low-temperature cracking (Cao et al., 2019). However, the addition of light molecules can physically melt the bitumen and result in an imbalance between bitumen components, potentially negatively impacting the high-temperature rutting resistance of bitumen (Ma et al., 2021). Simultaneously, the use of plant oil can effectively lower the polymer content in hard petroleum bitumen by supplementing light components. Adding WVO to the petroleum asphalt system significantly alters the composition of the asphalt colloidal system due to the introduction of a large amount of light components, resulting in noticeable differences in the high-temperature performance between bio-asphalt and matrix petroleum asphalt.
To balance the high-temperature and low-temperature performance of plant oil-modified bitumen, some researchers have studied composite bitumen with plant oil and other polymers or additives. Lv et al. (Lv et al., 2021) conducted a comprehensive study on five types of rock bitumen bio-bitumen with different amounts, using tests such as temperature sweep (TS), multiple stress creep recovery (MSCR), bending beam rheometer (BBR), Fourier-transform infrared reflection (FTIR), and scanning electron microscopy (SEM) from macroscopic and microscopic perspectives. The study indicated that the incorporation of bio-oil reduces the high-temperature performance of bitumen while improving its low-temperature performance. The modification process of bio-oil on bitumen is physical process, and, except for waste oil and plant oil, there is no apparent correlation between the rheological properties and infrared spectra of other bio-oils. Joni through multiple laboratory tests, investigated the physical properties of matrix bitumen and modified bitumen (Joni et al., 2020),. The results showed that waste plant oil-modified bitumen has better resistance to thermal cracking and lower rutting resistance, making it more suitable for use in moderately cold regions. Aldagari mixed PET particles treated with waste plant oil into bitumen using a high shear mixer and aged them in the laboratory using rolling thin film oven (RTFO) and pressure aging vessel (PAV). Dynamic shear rheometer (DSR) and Fourier-transform infrared (FTIR) spectroscopy captured the rheological and chemical changes during ageing (Aldagari et al., 2021). The results indicated that the oil-treated PET-modified binder lost 15.6% of its healing capacity after long-term aging, while the pure binder lost nearly 66%. As indicated by lower aging index values, oil-treated PET has been proven to effectively reduce aging effects.
On one hand, the use of plant oil in composite bitumen modification can supplement light components and adjust the composition of bitumen, similar to its functioning in neat bitumen or aged bitumen (Gökalp and Uz, 2019; Joni et al., 2020; Tarar et al., 2020; Lv et al., 2021; Ma et al., 2021). Additionally, plant oil can activate other modifiers, enhancing the performance and compatibility of modified bitumen (Aldagari et al., 2021). Studies have shown that waste polymers (such as polyvinyl chloride (PVC), low-density polyethylene (LDPE), polystyrene (PS), polyethylene (PE), rubber crumbs (Li et al., 2022), etc.) can absorb light components in bitumen, expanding to form a network structure after modification. The formation of a network structure improves the viscoelasticity and high-temperature performance of base bitumen while increasing bitumen’s creep stiffness, making it more prone to low-temperature cracking. However, their compatibility with the base binder varies due to different materials (Appiah et al., 2017; Xu et al., 2021). These initial studies demonstrate promising prospects for the direct use of plant oil in bitumen or plant oil as a pre-treatment material for other waste additives, contributing to resource conservation, recycling, and sustainable development in road construction. However, further research is needed to delve into the technical optimisation of using plant oil in modified binders, to fully understand the diffusion pathways and interface interactions of the materials.
Additionally, sunflower seed oil (SO) can serve as an anti-ageing modifier in asphalt binders. Tarar et al. reported that the addition of sunflower seed oil can enhance the fatigue resistance and ageing durability of asphalt binders (Tarar et al., 2020). The presence of α-tocopherol in sunflower seed oil is believed to undergo carboxylic acid and bitumen anhydride esterification. The formation of esters can retard ageing and inhibit the volatilisation of light components in asphalt binders. Ricinoleic acid, a major component of castor oil, contains natural hydroxyl groups, theoretically allowing it to react with carboxylic acids and anhydrides in bitumen (Yamamoto et al., 2020). However, research on castor oil-modified bitumen and the use of castor oil as an anti-ageing modifier for bitumen are still lacking. Further investigation is needed to elucidate the anti-ageing mechanisms of other plant oils in bitumen modification.
Bio bitumen has economic, social, and environmental benefits. The use of bio bitumen can save the consumption of non-renewable resources and has good environmental benefits. The use of swine manure and other materials for bitumen modification is in line with this direction.
In order to utilize this material, scholars have studied its preparation method. Wang et al. proposed a method for preparing swine manure bio-oil (Wang et al., 2021). As shown in Figure 3, swine manure particles were subjected to dehydration and crushing treatment quickly passed through the fluidised bed reactor from bottom to top under the drive of nitrogen. The swine manure particles rapidly exchanged heat with the preheated quartz stone in the reactor in an oxygen-free environment. The swine manure particles underwent a rapid pyrolysis reaction during the rapid temperature rise process.
FIGURE 3. Production process of swine manure bio-oil (Wang et al., 2021).
After developing this modifier, it is necessary to test its effectiveness, among which the basic performance testing of bitumen and asphalt mixtures is important.
Wang et al. evaluated the high and low temperature performance of modified bitumen made by different processes through MSCR and BBR test (Wang et al., 2021). The results showed that the addition of swine manure bio-oil to petroleum bitumen is beneficial to the workability, but cause negative effect on its high temperature performance, and fatigue performance.
Although manure-based bio-oil has adverse effects on the high-temperature performance of bitumen, it has an enhancing effect on the flexibility of bitumen. Oldham et al. examined the performance and workability of bitumen designed with and without a specified percentage of a bio-binder produced from swine manure and Recycled Bitumen Shingle (RAS) (Oldham et al., 2015). It was found that the ductility and fracture energy of the RAS modified bitumen binder was improved significantly when bio-binder was introduced. Mogawer et al. added a biological modifier made from swine manure to the RAP material (Mogawer et al., 2016). The data shows that the bio-modified binder improved the fatigue performance and cracking resistance of the 40% RAP binder, without negatively affecting its moisture sensitivity/rutting characteristics. Zhou et al. used DSR, X-ray diffraction, and high-pressure liquid chromatograph to measure the chemical composition, structure, and pyrolysis yield of biochar and bio-oil prepared from waste wood and swine manure (Zhou et al., 2021). Research has observed that biochar can significantly alter the basic properties of petroleum bitumen, including softening point, ductility, viscosity, and complex modulus. Most importantly, compared to untreated bio-oil, the performance of upgraded manure-based bio-oil is overall enhanced, increasing the potential for partial or complete substitution of petroleum asphalt.
It was also found that swine manure-based bio-oil have good resistance and repair effects on bitumen ageing, which may be a key aspect of the application of swine manure modifiers. Fini et al. studied the effects of the introduction of four different bio-binders made from swine manure, mango grass particles, corn straw, and sawdust particles on the rheological and chemical properties of the selected bitumen binder (PG64-22) before and after oxidation ageing through DSR, RV, and FTIR test (Fini et al., 2017). The results showed that the bio binder with swine manure is less susceptible to ageing compared to plant-based bio-oils. Karnati er al. Introduced a sustainable and economic bio-binder made from hydrothermal liquefaction of swine manure for surface functionalisation of silica nanoparticles (SNPs) (Karnati et al., 2021). The bio-binder functionalized SNPs (BB-SNPs) were characterised by scanning electron microscope (SEM), dynamic light scattering (DLS), Fourier-transform infrared spectroscopy (FTIR), and inverse gas chromatography (IGC), and BB-SNPs demonstrated superior capability to delay ageing of bitumen while being economically viable. Oldham et al. also characterised the aged bitumen before and after rejuvenation using rheology and atomic force microscopy (AFM). (Oldham et al., 2018). The results showed that the addition of bio-oil derived from swine manure referred to as bio-rejuvenator (BR) significantly restored the performance of bitumen in this study. Pahlavan et al. also studied the co-liquefying high-protein algae with high-lipid swine manure to form bio-oils containing high concentrations of nitrogen rich condensed aromatic hydrocarbons (Pahlavan et al., 2020). Through DSR test, this bio-oil can restore the original chemical equilibrium and molecular conformation of aged bitumen binder, enabling the rejuvenation of aged bitumen (Xu et al., 2024).
In summary, swine manure modified bitumen is usually used in conjunction with other modifiers for the rejuvenation process of aged bitumen. Its addition will enhance the low-temperature performance and ductility of bitumen. However, in the case of inaccurate dosage, there is a certain degree of damage to the high-temperature performance and fatigue performance of bitumen.
This paper systematically reviewed the state-of-the-art knowledge in the application of bio-binders for asphalt pavements. The feasibility and challenges of using bio-based additives have been analysed. Specifically, wood-based bio-oils, waste cooking oils, manure-based bio-oils were investigated as the representatives of the most promising bio-oils being used nowadays or the modification and replacement of petroleum bitumen. Based on the analysis, the following conclusions could be drawn.
(1) All bio-based oils currently being used are more suitable for serving as additives like softeners, rejuvenators, or modifiers rather than as alternative of binders. Existing research revealed that using bio-oils as additives are feasible, however, current technologies are not capable of replacing petroleum bitumen by bio-oils.
(2) One of the most promising utilisations of bio-oils is as rejuvenators. It was reported that bio-oils can effectively soften the aged bitumen, reduced the stiffness while increase the flexibility, thereby improving the low-temperature performance and fatigue resistance of bitumen while keeping high-temperature performance reasonably efficient.
(3) Bio-oils are potentially to be used as antioxidants. It was evidently reported that bitumen modified with bio-oils has improved ageing resistance. Therefore, bio-oils may play a dual role in the modification of bitumen.
(4) Bio-oil and polymer composites are other options to improve all temperature range performance of bitumen, especially in rejuvenation of aged binders. Incorporating polymers can avoid the unfavorable reduction in high-temperature related issues. The main concern is the compatibility between different components.
YZ: Conceptualization, Project administration, Writing–original draft. PD: Formal Analysis, Methodology, Writing–original draft. LZ: Investigation, Writing–original draft. XL: Writing–original draft. XC: Project administration, Supervision, Writing–review and editing. HZ: Writing–original draft.
The author(s) declare financial support was received for the research, authorship, and/or publication of this article. This work was finically supported by Lanzhou Highway Development Centre, Fundamental Research Funds for the Central Universities, CHD under grant number of [300102213204].
Author HZ was employed by China State Construction Silk Road Investment Group Co, Ltd.
The remaining authors declare that the research was conducted in the absence of any commercial or financial relationships that could be construed as a potential conflict of interest.
All claims expressed in this article are solely those of the authors and do not necessarily represent those of their affiliated organizations, or those of the publisher, the editors and the reviewers. Any product that may be evaluated in this article, or claim that may be made by its manufacturer, is not guaranteed or endorsed by the publisher.
Ahmed, R. B., and Hossain, K. (2020). Waste cooking oil as an asphalt rejuvenator: a state-of-the-art review. Constr. Build. Mater. 230, 116985. doi:10.1016/j.conbuildmat.2019.116985
Aldagari, S., Kabir, S. F., and Fini, E. H. (2021). Investigating aging properties of bitumen modified with polyethylene-terephthalate waste plastic. Resour. Conservation Recycl. 173, 105687. doi:10.1016/j.resconrec.2021.105687
Ali, A., Christopher, W. R., Nacú, H., Williams, R. C., Hohmann, A. D., Hernández, N., et al. (2022). Performance evaluation of bioengineered recycled asphalt materials. Transp. Res. Rec. 2676, 464–475. doi:10.1177/03611981211036365
Appiah, J. K., Berko-Boateng, V. N., and Tagbor, T. A. (2017). Use of waste plastic materials for road construction in Ghana. Case Stud. Constr. Mater. 6, 1–7. doi:10.1016/j.cscm.2016.11.001
Bao, D. X., Yu, Y. Y., and Zhao, Q. M. (2020). Evaluation of the chemical composition and rheological properties of bio-asphalt from different biomass sources. Road Mater. Pavement Des. 21 (7), 1829–1843. doi:10.1080/14680629.2019.1568287
Camargo, I. G. D., Bernucci, L. L. B., and Vasconcelos, K. L. (2018). “Aging characterization of biobinder produced from renewable sources,” in RILEM technical committee (TC) 252 international symposium on chemo-mechanics of bituminous materials (CMB) (Braunschweig, GERMANY: Spinger), 9–14.
Cao, Z., Chen, M., Liu, Z., He, B., Yu, J., and Xue, L. (2019). Effect of different rejuvenators on the rheological properties of aged sbs modified bitumen in long term aging. Constr. Build. Mater. 215, 709–717. doi:10.1016/j.conbuildmat.2019.04.257
Capunitan, J. A., and Capareda, S. C. (2013). Characterization and separation of corn stover bio-oil by fractional distillation. Fuel 112, 60–73. doi:10.1016/j.fuel.2013.04.079
Castro-Alonso, M. J., Espinosa, L. V., Marcelino, P. R. F., Savasini, K. V., Dos Santos, J. C., Moraes, R., et al. (2023). Physicochemical and aging characterisation of bio-binders from pine wood resin for paving applications. Road Mater. Pavement Des. 24, 229–244. doi:10.1080/14680629.2023.2180306
Cong, Y., Zhang, W., Liu, C., and Huang, F. (2019). Composition and oil-water interfacial tension studies in different vegetable oils. Food Biophys. 15 (2), 229–239. doi:10.1007/s11483-019-09617-8
Conglin, C., Jinbo, L., Tao, M., Yang, Z., Linhao, G., and Xiang, C. (2023). Applications of vegetable oils and their derivatives as Bio-Additives for use in asphalt binders: a review. Constr. Build. Mater. 383, 131312. doi:10.1016/j.conbuildmat.2023.131312
Ding, Y. J., Shan, B. L., Cao, X. J., Liu, Y. G., Huang, M. X., and Tang, B. M. (2021). Development of bio oil and bio asphalt by hydrothermal liquefaction using lignocellulose. J. Clean. Prod. 288, 125586. doi:10.1016/j.jclepro.2020.125586
Espinosa, L. V., Gadler, F., Mota, R. V., Guatimosim, F. V., Camargo, I., Vasconcelos, K., et al. (2021a). Multi-scale study of bio-binder mixtures as surface layer: laboratory evaluation and field application and monitoring. Constr. Build. Mater. 287, 122982. doi:10.1016/j.conbuildmat.2021.122982
Espinosa, L. V., Gadler, F., Mota, R. V., Vasconcelos, K., and Bernucci, L. L. B. (2021b). Comparison of the rheological and the thermal behaviour of a neat asphalt binder and a wood-based binder for pavement surface layer. Road Mater. Pavement Des. 22, S702–S717. doi:10.1080/14680629.2021.1911834
Fini, E. H., Hosseinnezhad, S., Oldham, D. J., Chailleux, E., and Gaudefroy, V. (2017). Source dependency of rheological and surface characteristics of bio-modified asphalts. Road Mater. Pavement Des. 18 (2), 408–424. doi:10.1080/14680629.2016.1163281
Giakoumis, E. G. (2018). Analysis of 22 vegetable oils’ physico-chemical properties and fatty acid composition on a statistical basis, and correlation with the degree of unsaturation. Renew. Energy 126, 403–419. doi:10.1016/j.renene.2018.03.057
Gökalp, İ., and Uz, V. E. (2019). Utilizing of waste vegetable cooking oil in bitumen: zero tolerance aging approach. Constr. Build. Mater. 227, 116695. doi:10.1016/j.conbuildmat.2019.116695
He, M., Tu, C., Cao, D. W., and Chen, Y. J. (2019). Comparative analysis of bio-binder properties derived from different sources. Int. J. Pavement Eng. 20 (7), 792–800. doi:10.1080/10298436.2017.1347434
Hınıslıoğlu, S., and Ağar, E. (2004). Use of waste high density polyethylene as bitumen modifier in asphalt concrete mix. Mater. Lett. 58 (3-4), 267–271. doi:10.1016/s0167-577x(03)00458-0
Hosseinnezhad, S., Hung, A. M., Mousavi, M., Sharma, B. K., and Fini, E. (2020). Resistance mechanisms of biomodified binders against ultraviolet exposure. Acs Sustain. Chem. Eng. 8 (6), 2390–2398. doi:10.1021/acssuschemeng.9b05490
Houlei, G., Christopher, H., Qing, L., Xungai, W., Wang, X., and Long, R. L. (2021). Effect of oleic purity on the chemical structure, thermal and rheological properties of bio-based polymers derived from high oleic cottonseed oil via RAFT polymerization. Industrial Crops Prod. 171, 113882. doi:10.1016/j.indcrop.2021.113882
Hu, Y., Si, W., Kang, X., Xue, Y., Wang, H., Parry, T., et al. (2022). State of the art: multiscale evaluation of bitumen ageing behaviour. Fuel 326, 125045. doi:10.1016/j.fuel.2022.125045
Hu, Y., Wang, H., Zhou, L., and Airey, G. D. (2024). “Towards an enhanced understanding of the functional groups within bitumen during ageing processes,” in Advances in functional pavements (London: CRC Press), 83–87.
Hu, Y., Xia, W., Xue, Y., Zhao, P., Wen, X., Si, W., et al. (2023). Evaluating the ageing degrees of bitumen by rheological and chemical indices. Road Mater. Pavement Des. 24 (1), 19–36. doi:10.1080/14680629.2023.2180289
Ingrassia, L. P., and Canestrari, F. (2022). VECD analysis to investigate the performance of long-term aged bio-asphalt mixtures compared to conventional asphalt mixtures. Road Mater. Pavement Des. 23 (12), 2697–2712. doi:10.1080/14680629.2021.1991839
Ingrassia, L. P., Lu, X. H., Ferrotti, G., and Canestrari, F. (2020). Chemical, morphological and rheological characterization of bitumen partially replaced with wood bio-oil: towards more sustainable materials in road pavements. J. Traffic Transp. Engineering-English Ed. 7 (2), 192–204. doi:10.1016/j.jtte.2019.04.003
Ji, J., Yao, H., Suo, Z., You, Z., Li, H., Xu, S., et al. (2016). Effectiveness of vegetable oils as rejuvenators for aged asphalt binders. J. Mater. Civ. Eng. 29 (3), D4016003. doi:10.1061/(asce)mt.1943-5533.0001769
Joni, H. H., Al-Rubaee, R. H., and Al-zerkani, M. A. (2020). Characteristics of asphalt binder modified with waste vegetable oil and waste plastics. IOP Conf. Ser. Mater. Sci. Eng. 737, 012126. doi:10.1088/1757-899x/737/1/012126
Kabir, S. F., Mousavi, M., and Fini, E. H. (2020). Selective adsorption of bio-oils' molecules onto rubber surface and its effects on stability of rubberized asphalt. J. Clean. Prod. 252, 119856. doi:10.1016/j.jclepro.2019.119856
Karnati, S. R., Oldham, D., Fini, E. H., and Zhang, L. F. (2021). Surface functionalization of silica nanoparticles with swine manure-derived bio-binder to enhance bitumen performance in road pavement. Constr. Build. Mater. 266, 121000. doi:10.1016/j.conbuildmat.2020.121000
Kolawole, S., George, R., and Liqun, Z. (2021). Molecular dynamics simulation on vegetable oil modified model asphalt. Constr. Build. Mater. 270, 121687. doi:10.1016/j.conbuildmat.2020.121687
Li, C., Rajib, A., Sarker, M., Liu, R. H., Fini, E. H., and Cai, J. M. (2021). Balancing the aromatic and ketone content of bio-oils as rejuvenators to enhance their efficacy in restoring properties of aged bitumen. Acs Sustain. Chem. Eng. 9 (20), 6912–6922. doi:10.1021/acssuschemeng.0c09131
Li, J., Xiao, X., Chen, Z., Xiao, F., and Amirkhanian, S. N. (2022). Internal de-crosslinking of scrap tire crumb rubber to improve compatibility of rubberized asphalt. Sustain. Mater. Technol. 32, e00417. doi:10.1016/j.susmat.2022.e00417
Lv, S., Liu, J., Peng, X., and Jiang, M. (2021). Laboratory experiments of various bio-asphalt on rheological and microscopic properties. J. Clean. Prod. 320, 128770. doi:10.1016/j.jclepro.2021.128770
Ma, J., Sun, G., Sun, D., Hu, M., and Lu, T. (2021). Chemo-rheological characterization of the effect of Iran rock asphalt on the performance of waste bio-oil modified asphalts. Transp. Res. Rec. 2675 (10), 1324–1338. doi:10.1177/03611981211015989
Maxwell, S., Ali, A., Christopher, W. R., and Eric, C. (2021). Statistical analysis factor screening of soybean oil and polymer modified asphalt blending using master batching technique. Constr. Build. Mater. 313, 125435. doi:10.1016/j.conbuildmat.2021.125435
Mogawer, W. S., Fini, E. H., Austerman, A. J., Booshehrian, A., and Zada, B. (2016). Performance characteristics of high reclaimed asphalt pavement containing bio-modifier. Road. Mater. Pavement Des. 17 (3), 753–767. doi:10.1080/14680629.2015.1096820
Mohammad, L. N., Elseifi, M. A., Cooper, S. B., Challa, H., and Naidoo, P. (2013). Laboratory evaluation of asphalt mixtures that contain biobinder technologies. Transp. Res. Rec. 2371 (2371), 58–65. doi:10.3141/2371-07
Mohan, D., Pittman, C. U., and Steele, P. H. (2006). Pyrolysis of wood/biomass for bio-oil: a critical review. Energy and Fuels 20 (3), 848–889. doi:10.1021/ef0502397
Mousavi, M., Pahlavan, F., Oldham, D., Hosseinnezhad, S., and Fini, E. H. (2016). Multiscale investigation of oxidative aging in biomodified asphalt binder. J. Phys. Chem. C 120 (31), 17224–17233. doi:10.1021/acs.jpcc.6b05004
Oasmaa, A., Solantausta, Y., Arpiainen, V., Kuoppala, E., and Sipilä, K. (2010). Fast pyrolysis bio-oils from wood and agricultural residues. Energy and Fuels 24 (2), 1380–1388. doi:10.1021/ef901107f
Oldham, D., Hung, A., Parast, M. M., and Fini, E. H. (2018). Investigating bitumen rejuvenation mechanisms using a coupled rheometry-morphology characterization approach. Constr. Build. Mater. 159, 37–45. doi:10.1016/j.conbuildmat.2017.10.113
Oldham, D. J., Fini, E. H., and Chailleux, E. (2015). Application of a bio-binder as a rejuvenator for wet processed asphalt shingles in pavement construction. Constr. Build. Mater. 86, 75–84. doi:10.1016/j.conbuildmat.2015.03.085
Omairey, E., Hughes, D., Hu, Y., and Airey, G. (2024). “Feasibility evaluation of bio-waste derived, plastic-waste modified binder rejuvenators,” in Advances in functional pavements (London: CRC Press), 58–61.
Omojola, A., Idoko, O. E., and Inambao, F. L. (2019). Comparative study of properties and fatty acid composition of some neat vegetable oils and waste cooking oils. Int. J. Low-Carbon Technol. 14 (3), 417–425. doi:10.1093/ijlct/ctz038
Pahlavan, F., Lamanna, A., Park, K. B., Kabir, S. F., Kim, J. S., and Fini, E. H. (2022). Phenol-rich bio-oils as free-radical scavengers to hinder oxidative aging in asphalt binder. Resour. Conservation Recycl. 187, 106601. doi:10.1016/j.resconrec.2022.106601
Pahlavan, F., Rajib, A., Deng, S., Lammers, P., and Fini, E. H. (2020). Investigation of balanced feedstocks of lipids and proteins to synthesize highly effective rejuvenators for oxidized asphalt. ACS Sustain. Chem. Eng. 8 (20), 7656–7667. doi:10.1021/acssuschemeng.0c01100
Park, K. B., Kim, J. S., Pahlavan, F., and Fini, E. H. (2022). Biomass waste to produce phenolic compounds as antiaging additives for asphalt. Acs Sustain. Chem. Eng. 10 (12), 3892–3908. doi:10.1021/acssuschemeng.1c07870
Peralta, J., Williams, R. C., Rover, M., and Silva, H. M. R. D. D. (2012). “Development of a rubber-modified fractionated bio-oil for use as noncrude petroleum binder in flexible pavements,” in Alternative binders for sustainable asphalt pavements (Washington DC, United States: Transportation Research Circular).
Portugal, A. C. X., Lucena, L. C. d.F. L., Lucena, A. E. d.F. L., and Costa, D. B. d. (2018). Rheological performance of soybean in asphalt binder modification. Road Mater. Pavement Des. 19 (4), 768–782. doi:10.1080/14680629.2016.1273845
Ramadhansyah, P. J., Masri, M. K., Wan Azahar, M., Mashros, N., Norhidayah, A. H., Mohd Warid, M. N., et al. (2020). Waste cooking oil as bio asphalt binder: a critical review. IOP Conf. Ser. Mater. Sci. Eng. 712, 012040. doi:10.1088/1757-899x/712/1/012040
Raouf, M. A., Metwally, M., and Williams, R. C. (2010). Development of non-petroleum based binders for use in flexible pavements. Iowa: Iowa State University Institute for Transportation.
Raouf, M. A., and Williams, R. C. (2010). Temperature and shear susceptibility of a nonpetroleum binder as a pavement material. Transp. Res. Rec. 2180, 9–18. doi:10.3141/2180-02
Rezvan, B., and Alireza, A. (2021). Influence of soybean oil on binder and warm mixture asphalt properties. Adv. Mater. Sci. Eng. 2021, 1–16. doi:10.1155/2021/6860878
Seidel, J. C., and Haddock, J. E. (2014). Rheological characterization of asphalt binders modified with soybean fatty acids. Constr. Build. Mater. 53, 324–332. doi:10.1016/j.conbuildmat.2013.11.087
Somé, C., Pavoine, A., Chailleux, E., Andrieux, L., DeMarco, L., Philippe, D., et al. (2016). “Rheological behaviour of vegetable oil-modified asphaltite binders and mixes,” in Proceedings of the 6th eurasphalt and eurobitume congress (Prague, Czech Republic: E&E Congress), 1–3.
Stankovikj, F., and Garcia-Perez, M. (2017). TG-FTIR method for the characterization of bio-oils in chemical families. Energy and Fuels 31 (2), 1689–1701. doi:10.1021/acs.energyfuels.6b03132
Stankovikj, F., McDonald, A. G., Helms, G. L., and Garcia-Perez, M. (2016). Quantification of bio-oil functional groups and evidences of the presence of pyrolytic humins. Energy and Fuels 30 (8), 6505–6524. doi:10.1021/acs.energyfuels.6b01242
Suo, Z., Chen, H., Yan, Q., Tan, Y., Li, X., and Zhang, A. (2021). Laboratory performance evaluation on the recovering of aged bitumen with vegetable oil rejuvenator. Front. Mater. 8, 650809. doi:10.3389/fmats.2021.650809
Suwarto, F., Parry, T., and Airey, G. (2023a). Review of methodology for life cycle assessment and life cycle cost analysis of asphalt pavements. Road Mater. Pavement Des. 2023, 1–27. doi:10.1080/14680629.2023.2278149
Suwarto, F., Parry, T., Thom, N., Airey, G., Abed, A., Rahman, T., et al. (2023b). Engineering characterization and environmental analysis of natural rubber latex modified asphalt mixture. Constr. Build. Mater. 402, 132970. doi:10.1016/j.conbuildmat.2023.132970
Tahir, M. H., Cheng, X., Irfan, R. M., Ashraf, R., and Zhang, Y. (2020). Comparative chemical analysis of pyrolyzed bio oil using online TGA-FTIR and GC-MS. J. Anal. Appl. Pyrolysis 150, 104890. doi:10.1016/j.jaap.2020.104890
Tarar, M. A., Khan, A. H., Ur Rehman, Z., Qamar, S., and Akhtar, M. N. (2020). Compatibility of sunflower oil with asphalt binders: a way toward materials derived from renewable resources. Mater. Struct. 53, 64–15. doi:10.1617/s11527-020-01506-8
Wang, H., Jing, Y. F., Zhang, J. P., Cao, Y. B., and Lyu, L. (2021). Preparation and performance evaluation of swine manure bio-oil modified rubber asphalt binder. Constr. Build. Mater. 294, 123584. doi:10.1016/j.conbuildmat.2021.123584
Xiao, Y., Yan, B., Zhang, X., Chang, X., and Li, M. (2020). Study the diffusion characteristics of rejuvenator oil in aged asphalt binder by image thresholding and GC–MS tracer analysis. Constr. Build. Mater. 249, 118782. doi:10.1016/j.conbuildmat.2020.118782
Xu, F., Zhao, Y., and Li, K. (2021). Using waste plastics as asphalt modifier: a review. Materials 15 (1), 110. doi:10.3390/ma15010110
Xu, H., Zou, Y., Airey, G., Wang, H., Zhang, H., Wu, S., et al. (2024). Wetting of bio-rejuvenator nanodroplets on bitumen: a molecular dynamics investigation. J. Clean. Prod., 141140. doi:10.1016/j.jclepro.2024.141140
Xu, J., Brodu, N., Abdelouahed, L., and Taouk, B. (2022). Investigation of the combination of fractional condensation and water extraction for improving the storage stability of pyrolysis bio-oil. Fuel 314, 123019. doi:10.1016/j.fuel.2021.123019
Yamamoto, A., Nemoto, K., Yoshida, M., Tominaga, Y., Imai, Y., Ata, S., et al. (2020). Improving thermal and mechanical properties of biomass-based polymers using structurally ordered polyesters from ricinoleic acid and 4-hydroxycinnamic acids. RSC Adv. 10 (60), 36562–36570. doi:10.1039/d0ra05671e
Yang, S. H., and Suciptan, T. (2016). Rheological behavior of Japanese cedar-based biobinder as partial replacement for bituminous binder. Constr. Build. Mater. 114, 127–133. doi:10.1016/j.conbuildmat.2016.03.100
Yang, X., Mills-Beale, J., and You, Z. (2017). Chemical characterization and oxidative aging of bio-asphalt and its compatibility with petroleum asphalt. J. Clean. Prod. 142, 1837–1847. doi:10.1016/j.jclepro.2016.11.100
Yang, X., You, Z., and Dai, Q. (2013). Performance evaluation of asphalt binder modified by bio-oil generated from waste wood resources. Int. J. Pavement Res. Technol. 6, 4. doi:10.6135/ijprt.org.tw/2013.6(4).431
Yang, X., You, Z., Dai, Q., and Mills-Beale, J. (2014). Mechanical performance of asphalt mixtures modified by bio-oils derived from waste wood resources. Constr. Build. Mater. 51, 424–431. doi:10.1016/j.conbuildmat.2013.11.017
Yang, X., and You, Z. P. (2015). High temperature performance evaluation of bio-oil modified asphalt binders using the DSR and MSCR tests. Constr. Build. Mater. 76, 380–387. doi:10.1016/j.conbuildmat.2014.11.063
Yoosuk, B., Boonpo, J., Udomsap, P., and Sukkasi, S. (2014). Investigation of operating parameters of water extraction processes for improving bio-oil quality. Korean J. Chem. Eng. 31 (12), 2229–2236. doi:10.1007/s11814-014-0190-4
Zhang, D., Chen, M., Wu, S., Liu, S., and Liu, J. (2018). “Effect of waste cooking oil viscosity on basic and rheological properties of aged asphalt,” in Advances in energy and environmental materials: proceedings of Chinese materials conference 2017 18th (Springer), 647–656.
Zhang, R., Ji, J., You, Z. P., and Wang, H. N. (2020). Modification mechanism of using waste wood-based bio-oil to modify petroleum asphalt. J. Mater. Civ. Eng. 32 (12), 3464. doi:10.1061/(asce)mt.1943-5533.0003464
Zheng, Y. (2019). Evaluation of sunflower oil’s healing effect in bituminous materials. IOP Conf. Ser. Mater. Sci. Eng. 493, 012021. doi:10.1088/1757-899x/493/1/012021
Zhi, S., Lei, N., Fanrong, X., and Xu, B. (2021). The effect of waste plant oil on the composition and micro-morphological properties of old asphalt composition. Buildings 11 (9), 407. doi:10.3390/buildings11090407
Zhou, X. X., Moghaddam, T. B., Chen, M. Z., Wu, S. P., Zhang, Y., Zhang, X. R., et al. (2021). Effects of pyrolysis parameters on physicochemical properties of biochar and bio-oil and application in asphalt. Sci. Total Environ. 780, 146448. doi:10.1016/j.scitotenv.2021.146448
Zhou, X. X., Zhao, G. Y., Wu, S. P., Tighe, S., Pickel, D., Chen, M. Z., et al. (2020). Effects of biochar on the chemical changes and phase separation of bio-asphalt under different aging conditions. J. Clean. Prod. 263, 121532. doi:10.1016/j.jclepro.2020.121532
Keywords: bio-binder, bitumen, ageing, rejuvenation, circularity
Citation: Zhang Y, Ding P, Zhang L, Luo X, Cheng X and Zhang H (2024) Green roads ahead: a critical examination of bio-bitumen for sustainable infrastructure. Front. Mater. 11:1382014. doi: 10.3389/fmats.2024.1382014
Received: 04 February 2024; Accepted: 14 February 2024;
Published: 26 February 2024.
Edited by:
Jianmin Ma, Queen’s University, CanadaReviewed by:
Ning Li, Xi’an University of Architecture and Technology, ChinaCopyright © 2024 Zhang, Ding, Zhang, Luo, Cheng and Zhang. This is an open-access article distributed under the terms of the Creative Commons Attribution License (CC BY). The use, distribution or reproduction in other forums is permitted, provided the original author(s) and the copyright owner(s) are credited and that the original publication in this journal is cited, in accordance with accepted academic practice. No use, distribution or reproduction is permitted which does not comply with these terms.
*Correspondence: Xu Cheng, WHUuY2hlbmcyMDIxQG91dGxvb2suY29t
Disclaimer: All claims expressed in this article are solely those of the authors and do not necessarily represent those of their affiliated organizations, or those of the publisher, the editors and the reviewers. Any product that may be evaluated in this article or claim that may be made by its manufacturer is not guaranteed or endorsed by the publisher.
Research integrity at Frontiers
Learn more about the work of our research integrity team to safeguard the quality of each article we publish.