- 1Department of Chemical Engineering–Nanomaterials, Catalysis, Electrochemistry, University of Liège, Liège, Belgium
- 2Departamento de Química Inorgánica e Instituto Universitario de Materiales, University of Alicante, Alicante, Spain
- 3Departamento de Química-Física e Instituto Universitario de Materiales, University of Alicante, Alicante, Spain
While carbon in itself appears as simple an element as it could possibly get, the undeniable truth is that carbon materials represent a plethora of possibilities both from the perspective of their structure and their applications. While we may believe that carbon is “just another element”, one should never forget that its special ability to coordinate through different hybridizations with apparent ease grants the element properties that no other element may even match. Taking this one step further into the materials realm opens up numerous avenues in terms of materials dimensionality, surface and bulk functionalization, or degree of structural order just to mention a few examples. If these properties are translated into the properties and applications field, the results are just as impressive, with new applications and variants appearing with growingly larger frequency. This has resulted in over a million scientific papers published in the last decade in which the term “carbon” was used either in the title, abstract or keywords. When the search is narrowed down to the field “title” alone, the results drop to just over 318.000 scientific papers. These are figures that no other element in the periodic table can equal, which is a clear indicative that the story of carbon materials is still under constant evolution and development. This review will present an overview of the works published in the Frontiers in Carbon-based materials section during its 10 years of life that reflect the advancements achieved during the last decade in the field of carbon materials.
1 Introduction
The first words used in what may be considered the seminal paper for this contribution (Cazorla-Amorós, 2014) stated “Carbon in all forms: Carbon materials constitute the most versatile material group”. While this affirmation may seem too tall a claim, especially since 10 years have passed after its publication, the undeniable fact still is that carbon materials constitute the benchmark in many “old” and “new” (or “emerging”) applications (Kordas and Pitkänen, 2019; Azam et al., 2021; Valero-Romero et al., 2021; Quílez-Bermejo et al., 2022; Bumajdad et al., 2023; Liu, 2023) due to their adaptability to a huge range of applications, which coupled to their cost-effectiveness make them an ideal testing candidate. Over 30 years ago carbon was referred to as an “old but new material” (Walker, 1990) in a revision of a previous communication from 1972 (Walker, 1972) so its “oldness” is clearly undisputable. Thus, the underlying question is, what is it with carbon that makes it such a fascinating element for scientists worldwide for such a long span of time? Abraham Lincoln once said “You can fool some of the people all of the time, and all of the people some of the time, but you cannot fool all of the people all of the time.” so there must be something special about carbon.
An indeed there is, for carbon is the lightest element in the periodic table which we may find in its native solid form in Earth’s crust, and we may do so not in one but primarily two allotropic forms, diamond and graphite. Out of the two, the latter is by far the most interesting to scientists (even though let us not forget that Davy and Lavoisier burned a diamond in a cylinder filled with oxygen out of sheer curiosity) and reflects what is perhaps the distinctive peculiarity of carbon: due to its small size and relatively high electronegativity its atomic orbitals can adopt a sp2 hybridization which results in the formation of two-dimensional graphene sheets. The stacking of these very well-known sheets results in the even better-known graphite. While the stacking itself is rather straightforward, the long-range ordering (or its absence) of the layers together with their functionalization of their edge sites (for example, carbon atoms located at the edges of the graphene layers react mostly with atmospheric oxygen to form a chemically-rich surface (Figueiredo et al., 1999)) provides carbon materials with a unique blend of physical and chemical properties such as high surface area, high electrical conductivity, good corrosion resistance, high thermal and chemical stability in non-oxidizing environments and peculiar mechanical properties. Not only this, but their processability and compatibility with a wide range of materials and elements have made them also a subject of interest in the development of advanced composites (Inagaki et al., 2014), even giving rise to terms such as “carbon alloys” (Yasuda et al., 2003) as a reflection of the possibilities of combining carbon with other heteroatoms into multicomponent systems.
It then comes as no surprise that the carbon materials family is a significantly numerous one given the large (and ever growing) synthetic possibilities. Inagaki et al. (2014) and Radovic (2005) devised an elegant strategy to illustrate this dividing the forms in which carbon could present itself as “classic” and “new” carbon forms, the former including activated carbons, graphite, and carbon black and the latter carbon forms that were described during the second half of the 20th century. The range of structures and textures that can be created by controlling the carbonization process are enormous. This has led to the discovery of numerous carbon nanoforms. Even considering only sp2-hybridized carbon nanoforms, over 30 different forms have been identified. Bringing all potential hybridizations into the pictures strongly highlighted the need for a standardized nomenclature in the field, as the aforementioned seminal paper mentioned a decade ago. While 1D and 3D materials were addressed together (Barhoum et al., 2019), 2D carbon nanoforms were dedicated a specialized editorial paper (Bianco et al., 2013). Still, a global nomenclature is amiss in the current scenario.
The objective of this Anniversary collection article is to present an overview of the works published in the Frontiers in Carbon-based materials section during its 10 years of life that reflect the advancements achieved during the last decade in the field of carbon materials in terms of new structures, novel preparation methods as well as emerging and potential applications. This review includes the progress in carbon materials during the past decade within the framework of Frontiers in Carbon-based materials, focusing on three main aspects: advances concerning new or updated characterization techniques and properties, preparation methods, and emerging applications.
2 Characterisation and properties
The field of carbon materials is a fascinating world full of possibilities offered by their versatility and outstanding properties, which is evidenced in the review articles and original research articles compiled in the Carbon-Based Materials section published in Frontiers in Materials journal. As highlighted by Cazorla-Amorós, carbon materials account for a unique combination of physicochemical properties, such as high surface area, high electrical conductivity, high thermal stability, high chemical stability in non-oxidizing environments, good corrosion resistance, and particular mechanical properties (Cazorla-Amorós, 2014).
Concerning the review articles encompassed in the Carbon-Based Materials section published in Frontiers in Materials journal, very significant contributions may be found in which the importance of knowing and modulating the properties of carbon materials are detailed. That is the case of the review published by Macovez (2018), who revisited some of the physical properties of organic fullerene cocrystals compared to pristine C60, which are endorsed by the incorporation of different types of molecules. Some other review papers emphasise the importance of modulating the surface chemical properties of carbon materials in order to attain promising catalysts for a given application. For instance, Valero-Romero et al. (2021) compiled relevant studies about carbon-based catalysts for the Fischer-Tropsch synthesis and by classifying the revised literature according to the carbon shape and structure. They emphasised the importance of incorporating anchoring sites to afford catalysts with highly dispersed metal species in which the atomic efficiency is optimised. In the review article published by Navlani-García et al. (2019) the possibilities of attaining excellent catalysts by means of choosing an adequate carbon-based material which can be actively involved in the target catalytic reaction (dehydrogenation of formic acid), or modulating the properties of the metal phases by incorporating different nitrogen groups and contents of nitrogen are summarised.
Other review articles have focused on revisiting relevant electrochemical properties. In this line, Otero et al. (2016) pointed out the controversy behind the mechanisms responsible for the electrochemical response of carbon nanotubes (CNTs) and, by reviewing numerous articles, tried to clarify the importance of faradic and capacitive processes in the electrochemical response of electrochemical devices based on CNTs.
Most of the articles reported included a section devoted to the results achieved by using numerous characterisation techniques (transmission and scanning electron microscopy, atomic force microscopy, X-ray diffraction, N2 physisorption, diffuse reflectance UV-Vis, Raman and infrared spectroscopies, etc.) that are useful to fully know the physicochemical properties of carbon-based materials (structure, porous texture, morphology, surface properties, etc.). Some of them also explored other important features such as the magnetic properties (Orfanakis et al., 2018; Cesano et al., 2019), piezoresistivity (Kordas and Pitkänen, 2019), and optical properties (Ong, 2017; Magesh et al., 2022), among other.
The Carbon-Based Materials section in Frontiers in Materials journal delves not only in experimental results, but also in some very relevant theoretical studies which have been published during the past decade. That is the case of the publication reported by Liu et al. (2022), who utilised non-equilibrium molecular dynamics simulations to explore the effect of disorder on the phonon transport in a 2D graphene/hexagonal boron nitride heterostructure, or the article of Quqa et al. (2022), in which two machine learning approaches were used to construct pressure maps and identify pressure hotspots using a thin film based on carbon nanotubes integrated with a commercial foam.
These are but a few examples that show the pivotal role that the properties of carbon materials play and the wide range of possibilities that can be explored from both fundamental and applied research standpoints to design and develop carbon materials with tailored properties for a target application.
Nevertheless, exploiting the potential of the extraordinary properties of carbon materials is necessarily linked to having the tools to obtain insight into them. The development of advanced characterisation techniques is the cornerstone for achieving the knowledge needed to unveil the property-performance relationships that is behind the behaviour of the materials, and which should be the roadmap for the scientific community to design and develop new materials or to explore new possibilities of already existing ones.
To provide a general outlook about the characterisation and properties of the carbon materials that have been included in the contributions compiled in the Carbon-Based Materials section published in Frontiers in Materials journal, the present section is devoted to summarising the most important aspects that have been highlighted along these years related to both their physical and chemical properties.
2.1 Surface properties
2.1.1 Physical surface properties
Analysis of the textural properties of carbon materials is a must when aiming at designing and preparing any material for almost any application since they will surely be correlated in one or another way with their performance. Therefore, most of the articles found included a section devoted to the characterisation of the textural properties of carbon materials which are used for a wide range of applications. For example, for the impact of the textural properties of materials devoted to electrochemical applications, it was thoroughly checked by Schutjajew et al. (2019), who synthesised a series of carbon materials with varying porosities and used them as electrode materials in supercapacitors using ionic liquid electrolytes, which are claimed to have great potential in solving the low energy density of the prepared supercapacitors.
Characterisation of the textural properties is also a routine analysis when synthesising new materials. In this sense, Matsuo et al. (2015) characterised the porous texture of pillared carbons that had been prepared from the thermal reduction of graphite oxide. The samples were silylated with methyltrichlorosilane at various times in a study in which the effect of the content of Si on the porous properties of the pillared carbons was evaluated. The BET surface area of the resulting materials was dependent on the pillar density and ranged from 77 to 723 m2/g, and the intercalation of n-alkylamines did not compromise the structural flexibility of the pillars.
The adsorption properties of carbon materials, prepared from by-products and residues for CO2 capture, were evaluated by Calvo-Muñoz et al. (2016). The characterisation of the porous texture of the developed material was carried out by means of N2 adsorption/desorption isotherms at −196°C and CO2 adsorption isotherm at 0°C, which were used to calculate the apparent surface area, the external surface area associated with the non-microporous structure, or the micropore and mesopore volumes, among other parameters that are frequently determined for porous materials. To investigate the applicability of the studied materials in the target application, the key points of that work were the results achieved with the CO2 adsorption experiments. Equilibrium tests were monitored by registering the equilibrium adsorption isotherms of pure CO2, from 0 to 101.3 kPa and 25°C, and the isotherms were fitted using the typical models of Langmuir and Freundlich. Also, breakthrough experiments (dynamic adsorption experiments) were performed by registering the breakthrough curves using a mixture of 15% CO2 and 85% N2 at 25°C.
The adsorption properties of a commercial granular activated carbon used for wastewater treatment were evaluated by Belarus et al. (2014). That study was focused on evaluating the electroadsorption of arsenic from natural water and using a filter-press electrochemical cell. This was among the first studies that reported the use of porous carbon materials for that application. The textural and the surface chemistry properties of the adsorbent were characterised in depth and its behaviour was assessed for the removal of arsenic in parts per billion (ppb) concentration from polluted natural water.
Ruiz-Rosas et al. (2019) investigated the role of the porosity and surface chemistry on the performance of phosphorus-containing activated carbons as adsorbents of paracetamol. The carbons used in that study were prepared from the chemical activation of olive stones and had a BET surface area of 1235 m2/g with a micro-mesoporous texture. Moulefera et al. (2020) achieved highly nanoporous activated carbons obtained by chemical activation of Alcell lignin with phosphoric acid. In that study an activated carbon with an apparent surface area as high as 2,551 m2/g was obtained, which surpassed many of the lignin-derived activated carbons synthesised using other activation methods. Ishii et al. (2019) characterised the textural properties of zeolite-templated carbon (ZTC), oxidised ZTC, N-doped, Cu-doped and Cu-N-codoped ZTC, with displayed an apparent surface area that ranged from 2,130 to 4,080 m2/g.
2.1.2 Chemical surface properties
The chemical surface properties of carbon materials are determined by the presence of surface functional groups. The surface properties of carbon materials are frequently characterised by the determination of the pH at the point of zero charge (pHPZC) and the hydrophobic character, which is determined by measuring the affinity to adsorb certain hydrophilic and hydrophobic molecules. In addition, other techniques such as Boehm titration, XPS or TPD are frequently employed. The incorporation of heteroatoms into carbon materials is a well-known strategy to enhance their performance in many applications, but specially in electrochemical applications, since they improve the conductivity of the materials and their wettability towards the electrolytes.
Oxygen-containing functional groups are ubiquitous, and they can be incorporated both accidentally (by exposure of the material to the ambient atmosphere) or deliberately (by an oxidative treatment). The incorporation of other heteroatoms, such as nitrogen, phosphorus or sulphur is a very common strategy to achieve carbon materials with tailored properties for a given application. Among them, nitrogen-functional groups have become very popular, since the resulting materials display an interesting behaviour in numerous applications.
Bumajdad et al. (2023) reviewed the synthesis of nitrogen-enriched activated carbons derived from plant biomasses and their applications in wastewater treatment. The strategies followed for the incorporation of nitrogen functional groups were classified as: 1) two-step approach using nitrogen functionalisation and subsequent chemical activation; 2) two-step approach encompassing chemical activation and subsequent nitrogen functionalisation; and 3) single-stage approach which consists in subjecting the biomass to a nitrogen precursor and chemical activator to direct heat treatment. Figure 1 includes the thermochemical nitrogen enrichment procedure of plant-based biomasses. That review article also covers the nitrogen enrichment techniques using different types of molecules (i.e., amines, nitrogen enrichment techniques, and nitric acid). Li et al. (2021) found that the incorporation of N-doped carbon in hybrid materials formed by few-layers MoS2@N-doped carbon resulted in high-performance lithium and sodium storage. It was claimed that the incorporation of N-doped carbon material avoided the agglomeration of MoS2 nanocrystals, improved the electron transport capacity, and the stress of MoS2 during charge/discharge process. Han et al. (2021) designed B,N-codoped coral-like carbon framework and the surface wettability was checked by using the low-field nuclear magnetic resonance relaxation technique. The surface wettability towards the electrolyte, afforded by the presence of surface functional groups, was claimed to be crucial for the supercapacitive performance of the materials developed in that study. Maboya et al. (2022) prepared N-doped carbon nanotubes using their boron-doped carbon counterparts as the starting materials. It was observed that the introduction of nitrogen functional groups during the decomposition of the B-containing molecule led to a reduction in B content, which was attributed to the competitive incorporation of both heteroatoms. The developed materials were characterised by physicochemical techniques (transmission and scanning electron microscopy, Raman spectroscopy, X-ray photoelectron spectroscopy, etc.) and their performance towards the oxygen reduction reaction was assessed. Ruiz-Rosas et al. (2019) explored the role of the surface chemistry of P-containing activated carbons in the removal of micropollutants or emerging pollutants. It was observed that the low surface acidity of the materials increased the affinity of the surface of activated carbon and the model molecule used (i.e., paracetamol).
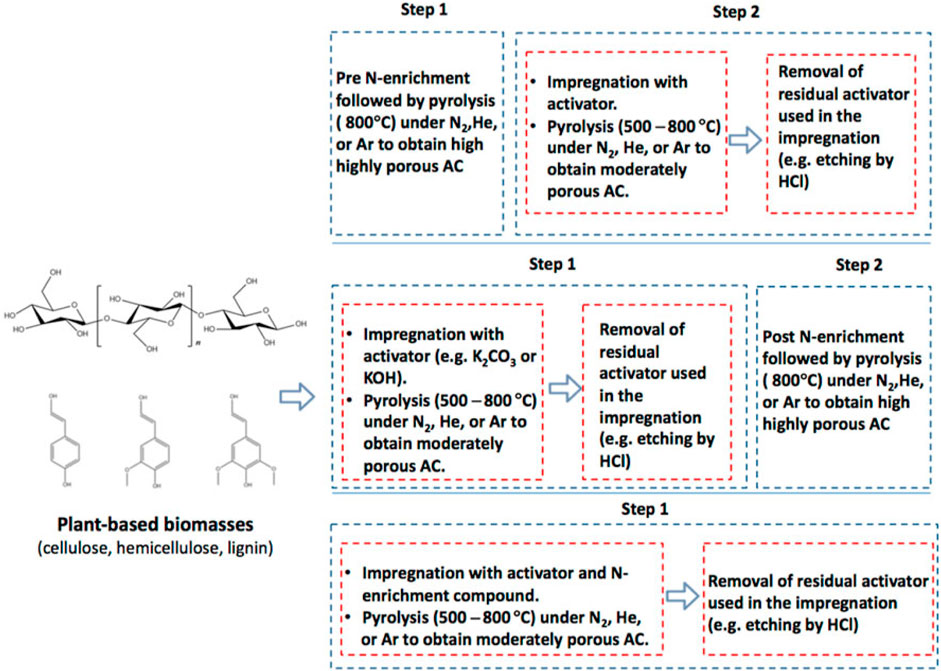
Figure 1. The thermochemical nitrogen enrichment procedure of plant-based biomasses. Reprinted from Bumajdad et al. (2023), Nitrogen-enriched activated carbon derived from plant biomasses: a review on reaction mechanism and applications in wastewater treatment. Front. Mater. 10:1218028.
2.2 Bulk properties
2.2.1 Thermal properties
The study of the thermal properties of materials is gaining more and more attention from both a fundamental and applied research viewpoint. The thermal properties of carbon materials are particularly interesting since their thermal conductivity covers a wide range depending on the structural properties (from ∼0.01 W mK−1 in amorphous carbon to above 2,000 W mK−1 at room temperature in diamond or graphene) (Baladin, 2011).
As it was contextualised in the study of Varshney et al. (2018), thermal properties, together with electrical properties are crucial for nanoelectronic device applications, and they can be modulated by means of chemical and structural modifications, such as dopants, defects, interface characteristics, etc. In the research article published by Varshney et al. (2018), they investigated the modulation of thermal conductance across a single walled carbon nanotube-single walled carbon nanotube (SWCNT-SWCNT) interface for nanotubes with different lengths, diameter, degree of radial deformation, chirality, and tensile strain. For that, a simulated methodology was used and the system consisted of physically interacting SWCNT pairs in a cross-contact geometry.
Zhang et al. (2020) highlighted the importance of the thermal properties of the materials used as thermal interface materials (TIMs) in electronic devices. As mentioned in their work, CNTs are frequently used as TIMs because of their unique thermal, electrical, and mechanical properties, but there are some issues related to the difficulties of having a proper orientation of the nanotubes to have an optimum thermal path. Vertically aligned CNT arrays (VACNT) were claimed to be a promising alternative and some surface modifications were performed to enhance the interface connection between the CNTs and the contact substrates.
2.2.2 Mechanical properties
The mechanical properties of carbon materials and carbon-based composites make them attractive for applications in everyday life, which has been particularly emphasised for CNTs. It has been pointed out that the arrangement/alignment of the CNTs plays a key role in the performance of the system, which can be controlled by assembling the CNTs within a matrix. In this line, Worajittiphon et al. (2015) prepared stretchable conductive networks of SWCNTs using plasticised colloidal templates and performed a mechanical and spectroscopic analysis to evaluate the strain transfer mechanisms between the CNT network and the polymer matrix. It was observed that the polymeric latex templates induce self-assembly of CNTs into 2D (hexagonal) and 3D (honeycomb) structures within the matrix. Several techniques, such as atomic force microscopy (AFM), scanning electron microscopy (SEM), and Raman spectroscopy were used. The degree of order in the composite was also checked with a fast Fourier transform (FFT) analysis, and the stress-strain curves for the latex substrates and SWCNT/latex composite films were obtained.
Kato et al. (2020) developed mechanically robust free-standing SWCNTs thin films with uniform mesh structure. The films were prepared by a blade-coating approach and the SWCNTs film bundle size distribution and the pore structure were controlled by controlling the dispersibility and entanglement of the SWCNTs in the suspensions used. In that study, four types of films were prepared using different types of suspensions, and their mechanical properties (i.e., tensile strength, fracture strain, and Young’s modulus) were checked. The results obtained indicated that the suspension with a higher fraction of smaller aggregates led to films with uniform mesh structures that displayed high mechanical performances. Among those investigated in that work, the most promising film had a tensile strength of 166 MPa, a fracture strain of 2.4%, and Young’s modulus of 7.6 GPa.
Dinu et al. (2023) developed thermosets that were environmentally friendly and chemically recyclable using the triglycidyl ether of phloroglucinol (TGPh), a renewable-based monomer, or a commercial non-toxic tris(4-hydroxyphenyl) methane triglycidyl ether (THPMTGE) monomer. The physicochemical and thermomechanical properties of the developed resins were characterised by means of differential scanning calorimetry (DSC), dynamic mechanical analysis (DMA), hardness tests, tensile testing, thermogravimetric analysis (TGA), scanning electron microscopy (SEM), among other techniques. These thermosets displayed remarkable mechanical properties, demonstrated by the tensile tests, with Young’s modulus ranging from 1.3 to 1.4 GPa, tensile stress of 55–69 MPa, and an elongation at break 3%–8%.
The mechanical properties of graphene oxide/epoxy carbon fibre-reinforced composites were reviewed by Keyte et al. (2019), who focused on the recent development in tailoring the mechanical properties of such composites by carbon fibre surface modification, epoxy polymer modification, composite processing techniques, and nanofiller dispersion in epoxy resin.
2.2.3 Other properties of carbon-based materials
Aside from the aforementioned properties, carbon materials present other interesting properties that have also been reflected in the articles published in the Carbon-Based Materials section, and that will be briefly mentioned in this section.
Magnetic properties are perhaps not among the most striking properties of carbon materials. However, interesting magnetic carbon-containing materials have been developed by incorporating some metal species. For instance, Orfanakis et al. (2018) developed hybrid nanomaterials of magnetic iron nanoparticles and graphene oxide. Those materials, which contained γ-Fe2O3 nanoparticles and amine-functionalised graphene oxide, were characterised by atomic force microscopy, X-ray diffraction, TG and DTA analysis, and FTIR measurement. Cesano et al. (2019) prepared hybrid magnetic materials based on microporous carbon spheres synthesised from a polymer waste and subsequent incorporation of iron salt followed by annealing. This study covered the characterisation of the morphology, structure, and textural properties of the samples. Also, the magnetic properties of the Fe3O4-containing materials were investigated by using magnetic force microscopy. In addition, the optical properties of the samples were also studied by recording the UV-vis absorption spectra.
Even though the optical properties of carbon materials are not the focus of many investigations, their impact on the utilisation of materials such as graphitic carbon nitride (g-C3N4) in photocatalytic applications has merited some recent studies. It has been reflected in the review article reported by Ong (2017), which covered the state-of-the-art research on two-dimensional g-C3N4 heterojunction nanohybrids (hybridization with 2D transition metal chalcogenides, 2D metal oxides, and 2D graphene). This review pointed out the potential of such materials in environmental remediation and artificial photosynthesis. Magesh et al. (2022) revisited some interesting properties in their review article on carbon quantum dots, such as UV-visible absorption, photoluminescence, photo-induced electron transfer, electroluminescence, and chemiluminescence, which make them interesting for many applications, covering fields such as energy, biological applications, optronics, and chemical sensing. The optical properties of carbon quantum dots were also reviewed in the publication of Azam et al. (2021), who emphasised the potential of those materials in biomedical applications. Chen and Song (2016) investigated the field emission from a lateral emitter made by a multi-walled carbon nanotube (MWCNT) yarn. It was claimed that such a lateral emitter could be utilised for figure display boards and lamps, among other applications.
The electronic properties of carbon materials have been also investigated in some articles (Ishii et al., 2019; Cesano et al., 2020a). For instance, Ishii et al. (2019) prepared heteroatom-doped zeolite templated carbons and applied them to an ion-sensitive field effect transistor to study the effect of heteroatom doping (i.e., oxygen, nitrogen) and copper incorporation on its electronic properties. Those materials were characterised by means of structural analysis, temperature programmed desorption, X-ray photoelectron spectroscopy, atomic absorption spectrometry, and work function measurement, and also the evaluation of ion sensing characteristics was considered. It was found that the materials doped with oxygen displayed p-type semiconductor behaviour, while the nitrogen-doped counterparts had n-type characteristics.
Cesano et al. (2020a) revisited in their review article the progress in the field of carbon-based materials used as electrical conductors. The role of doping such materials with metal and non-metal compounds in their electrical properties is also highlighted. That review included a section devoted to covering some of the most representative techniques used for the characterisation of those materials (3D X-ray tomography (3D XRT); X-ray scattering techniques, including X-ray diffraction (XRD), Wide-angle X-ray scattering (WAXS), and Small-angle X-ray scattering (SAXS); Raman spectroscopy; electron microscopies; X-ray photoelectron spectroscopy (XPS); contact angle measurements, etc.).
To sum up, concerning the characterization and the study of properties in carbon materials, the articles published in the Frontiers in Carbon-based materials section offer a detailed insight of several research efforts which, ultimately, pursue establishing a critical aspect in fundamental and applied science, which is the structure-performance (or structure-activity) relationship. Of course, the term “structure” falls short of the mark for depending on the desired application, scientist will analyze the bulk, surface, physical, chemical, or electrical properties as required, but at the end of the day, the goal remains fundamentally the same. In this respect, it must be mentioned that Machine Learning has already squeezed its way into the forefront of carbon-based materials research, with an article (Quqa et al., 2022) published in the journal delving on the topic.
3 Preparation of materials
Carbon-based materials play a fundamental role in society due to their multiple applications in a multitude of sectors, ranging from research to industry. Therefore, different synthesis procedures have been developed to obtain materials with different properties and morphologies depending on the final applications. The methods employed for the preparation of carbon materials are diverse including their synthesis from carbon sources such as organic compounds, biomass waste or polymers. Carbon materials can also be synthesized from existing carbon materials by changing their dimensionality or modifying their chemical composition. All this has been addressed in different review articles and original research articles included in the Carbon-Based Materials section published in Frontiers in Materials journal. Concerning the dimensionality of the carbon materials, the following section presents carbon materials classified according to their being, 0, 1, 2, or 3D materials along with their preparation procedures.
3.1 0D carbon materials
The carbon materials included in this classification are materials composed of carbon nanoparticles which are considered zero-dimensional materials such as fullerene, carbon black and carbon quantum dots.
Carbon quantum dots (CQDs) are interesting materials for various technological applications. Thereby, diverse methods of syntheses have been developed and these are compiled in the review article elaborated by Azam et al. (2021) and Magesh et al. (2022). Basically, the methods are divided into either top-down or bottom-up approaches. The top-down approach refers to methods that destroy or disperse carbon-based materials to form CQDs. Here, methods such as laser ablation (Cui et al. (2020), arc-discharge method (Chao-Mujica et al., 2021), ultrasonic synthesis (Dang et al., 2016) or acidic oxidation (Yang et al., 2014) method may be found. Among these methods, the latter has a problem related to the handling of strong oxidizers during synthesis. In contrast, bottom-up processes refer to the processes where small carbonaceous molecules can produce CQDs through chemical reactions including a combination of polymerization and carbonization reactions. In this case, methods such as combustion method, microwave pyrolysis and hydrothermal processes can be used. Commonly, reagents such as polyethylene glycol, saccharide and citric acid can be used as CQD precursors. The hydrothermal method is highlighted because it is simple and yields CQDs with high quantum yield. Moreover, the particle size is controllable and there is no need to use expensive chemicals. Finally, the electrochemical carbonization method is also interesting and uses low-molecular-weight alcohols as carbon precursors. In this latter case, the potential applied can be used to control the size of CQDs (Azam et al., 2021).
Regarding fullerene, few articles have been published that cover the preparation and use of these materials. Kouloumpis et al. (2015) reported the use of commercial Buckminsterfullerece (C60) to prepare composites with graphene. This method employed will be discussed later in the section on graphene-based materials. On the other hand, Macovez (2018) reviewed the synthesis of functionalized C60 consisting of cocrystals with small organic molecules such as solvates and hydrates. To this purpose, the gas-intercalation method is mentioned for the preparation of C60 with H2, NH4, and CO applying pressure to the molecular gas to favor its diffusion through C60. Moreover, C60 can also form solvates with a great number of solvents via precipitation or slow evaporation (Macovez, 2018). Meanwhile, cocrystals of fullerenes can be formed with non-solvent molecules from co-solutions in a common solvent by using a slow concentration of the solvent or by adding a bad solvent that favors precipitation (Macovez, 2018). Another important issue of the C60 is its hydrophobicity, so via a functionalization step is possible to improve the hydrophilicity of C60 and its solubility in water. The fullerenol (C60(OH)n) are interesting hydrophobic fullerenes such as (C60(OH)24) which can synthesize from the polybrominated fullerene (C60Br24) by substitution and neutralization reactions with sodium hydroxide (Macovez, 2018).
3.2 1D carbon materials
3.2.1 Preparation of 1D carbon materials
One-dimensional materials are structures that have two nanoscale dimensions, so they are linear nanostructures with a diameter of less than 1000 nm such as CNT and carbon nanofibers (CNF) and carbon fibers (CF) which size is typically in the micrometer range. The main difference between CNT and CNF structures lies in the different stacking of the carbon layers (Cesano et al., 2020a). CNFs may have different nanotextures, including herringbone, platelet, and tubular types (Inagaki et al., 2014), whereas the CNTs consist on rolled-up graphene layers with its edges connected, which can be constituted by single-, double-, and multi-walled tubes, in addition to having different chiralities in single-walled ones (Inagaki et al., 2014; Wu et al., 2020). Thus, a diversity of synthesis methods has been reported to obtain these different 1D carbon materials.
The review of Rosas et al. (2014) compiled different methods for the preparation of lignin-based carbon fibers (CFs). The four main manufacturing procedures for lignin CFs are wet-, dry-, melt-, and electrospinning. Moreover, the as-prepared lignin fibers need a procedure of oxidative thermostabilization prior to the carbonization step to avoid their melting. Usually, the process used is a thermal treatment under air at low temperatures with slow heating rates. Figure 2 illustrates the most common spinning techniques used for the preparation of fibers.
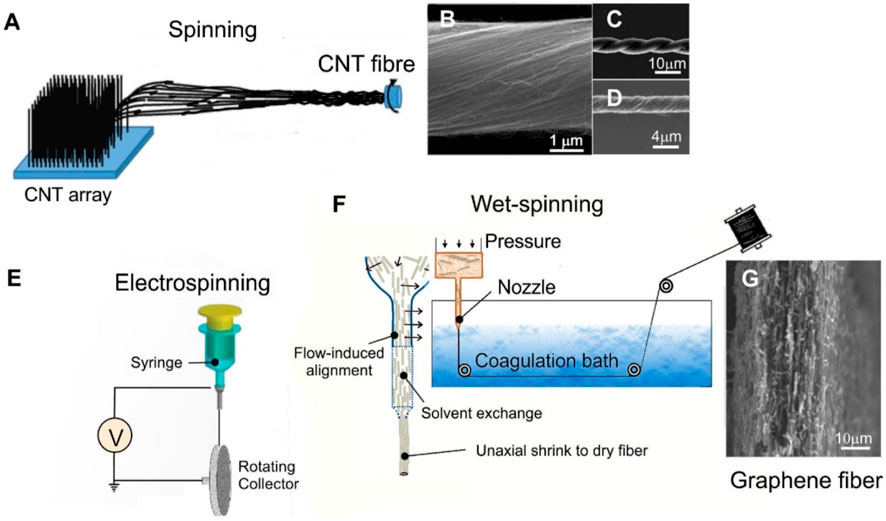
Figure 2. Macroscopic assembling methods of: (A) CNT fibers from a forest of CNT arrays by spinning; (B–D) SEM images of the CNT ropes after spinning and twisting steps; (E) CNT/graphene fibers by electrospinning and (F) graphene fibers by wet-spinning/coagulation methods; and (G) SEM image of the graphene fiber. Reprinted from Cesano et al. (2020a) All-Carbon Conductors for Electronic and Electrical Wiring Applications. Front. Mater. 7:219.
More recently, the most promising method for the preparation of CNFs due to its versatility is electrospinning which generally employs high-voltage electrical fields and a viscous and conductive solution containing the precursor. The review article of García-Mateos et al. (2019) is devoted to revising different porous carbon fibers prepared from Alcell lignin/ethanol solutions. It was commented on how the configuration (either coaxial or triaxial) could lead to the formation of microsized lignin-based carbon fibers or lignin-based carbon tubes, respectively. The chemical activation by the addition of H3PO4 in the lignin solution had two main effects 1) catalyze the crosslinking of the polymeric structure of lignin and 2) develop porosity. On the other hand, physical activation was carried out through the partial gasification of the fibers in diluted air during the carbonization step.
Apart from this review article, other research articles have used the electrospinning method to synthesize lignin-based nanofibers. In this context, Calvo-Muñoz et al. (2016) reported the preparation of lignin fibers manufactured by electrospinning of lignin/ethanol solution, followed by stabilization and carbonization processes. The as-prepared CNF resulted in good textural properties. On the other hand, Wei et al. (2019) prepared electrospun lignin-based carbon nanofibers from an alkali lignin precursor. It was observed that carbonization temperatures higher than 1200°C were required to increase the structural order and the graphite crystal size and to remove non-carbon elements of the nanofibers.
Moreover, there are parameters such as temperature and water that can affect the growth of CNT via the CVD. This fact was investigated by Geng et al. (2016) in the growth of SWCNTs revealing that the threshold temperature was around 970°C. Thus, above this value, no SWCNTs were detected. Moreover, the positive role of water impurities in the growth mechanism was also proven.
3.2.2 Functionalization of 1D carbon materials and their composites
Different approaches have been developed to modify the surface chemistry of the 1D carbon materials by introducing heteroatoms, supporting metal-based compounds or combination with polymers. The resulting materials have enhanced properties making them more suitable for certain applications. Thereby, diverse materials can be found in this section.
Metal oxide compounds such as WO3, ZnO, SiO2 and MnO2 can be combined with CNTs or CNFs through different methods. The simplest synthesis method is through physical mixing using an agate mortar. This was reported in the synthesis of the WO3/CNTs material (Carmona et al., 2016). However, generally, synthesis methods use solvents to favor the interaction between both compounds. Poirot et al. (2022) reported the synthesis of the TiO2/CNTs composite by mixing them with solvents together with a combination of sonication and reflux processes to produce CNTs with a thin layer of TiO2. Geng et al. (2023) designed the synthesis of SiO2/CNFs composite by a coating method using a SiO2 sol with carbon nanofibers. A much simpler way to form composites is by vacuum filtration of the solution to form a composite such as the MnO2/SWCNTs material reported in the review article prepared by Wu et al. (2020). Other synthesis methods can be found for the synthesis of MnO2/CNTs composites including electrodeposition, microwave-assisted method, chemical coprecipitation, thermal decomposition and hydrothermal treatment. Interestingly, the hydrothermal method can be useful for the preparation of adhesive-free electrodes by forming the composites on Ni foam (Wu et al., 2020). Apart from the mentioned methods, there are other not so well-known methods such as the vapor-liquid-solid (VLS) method to synthesize composites with a direct link between them. In the reported method, it consists of introducing in a quartz tube a holding boat with an Au-coated Si used as a substrate. Then, another holding boat with the material which would act as a source is also introduced. Then, the quartz tube is heated up to the evaporation of the material, and the vapor formed is deposited gradually forming nuclei on the Au drops. These nuclei would result in the formation of the nanostructures. Basit et al. (2022) reported the synthesis of the ZnO/CNTs by the VLS method resulting in different morphologies of ZnO due to the thickness of the Au layer. Kallumottakkal et al. (2021) also reported in their review article materials consisting of carbon nanotubes supporting metal oxides such as CoFe2O4 and BaTiO3 prepared via CVD and sol-gel methods, respectively.
The preparation of CFs decorated with metal nanoparticles may be performed by electrospinning and it was reviewed by García-Mateos et al. (2019). Pt-decorated lignin-based carbon fibers were synthesized by adding Pt precursor in the starting lignin solution. Pt can promote gasification which increases the mesoporosity and external surface area. In this case, the addition of the phosphorus precursor creates C-O-P groups that prevent and control gasification. Moreover, other cations such as Fe, Co or Pd can be also supported on the CFs by adding the metallic salts to the lignin solution.
Doping CNTs with B or N is also important for different applications. In this case, Maboya et al. (2022) designed the synthesis of CNT doped with N and B via a chlorination method using triphenylborane as the B source, whereas the N2 used for the bubbling provides the nitrogen source. The catalyst for the CNT was FeCo/CaCO3 catalyst, which was later removed by refluxing the material in 3M KOH.
Regarding composites with polymers, CNTs can be used for preparing composites with conducting polymers such as polyaniline (PANI), polypyrrole (PPy) and poly (3,4-ethylenedioxythiophene) (PEDOT) which have interesting electrochemical properties for their use in electrochemical capacitors (Salinas-Torres et al., 2019). A simple method of synthesis can be by ultrasonication of a solution containing both materials using a standard sonotrode (Sapalidis et al., 2018).
Finally, CNT can be employed for the synthesis of silica capillaries coated with carbon nanotubes on the inner surface. Sanchís et al. (2015) reported an interesting method which involved the use of a pH-switchable surfactant, previously prepared from 4-decylaniline and N,N-dimethylacetamide dimethylacetal, to produce the CNT deposition. The surfactant was necessary for stabilizing the CNT suspension used for the coating via an electrochemically assisted deposition method.
Carbon nanotubes can be used for the preparation of 2D materials, such as CNT buckypapers or films. Kato et al. (2020) designed single-walled carbon nanotube thin films prepared by wet process which consists of preparing a CNT suspension containing surfactant by ultrasonication and blade-coating it into films. To obtain uniform mesh structures it is necessary to have higher fractions of smaller aggregates and this is caused by the presence of the surfactant (flavin or SDOC). Furthermore, Zhang et al. (2020) designed a VACNT layer on a Cu foil substrate through a floating catalyst CVD (FCCVD). The VACNT layer was submitted to post-processing procedures such as plasma treatment or metallization to enhance the interfacial adhesion of the as-prepared material.
3.3 2D carbon materials
The review article elaborated by Kallumottakkal et al. (2021) reinforced the importance of graphene in promoting the creation of a group to classify materials with a 2D structure. Within this 2D material group, materials such as transition metal dichalcogenides, hexagonal boron nitride or MXene composites can be found. Therefore, for a better discussion of the 2D carbon materials, these will be separated into two subgroups: 1) graphene-based materials and 2) other 2D materials.
3.3.1 Graphene-based materials
Due to the importance of graphene-based materials in different applications, some review articles have been selected from those published in the Carbon-Based Materials section to describe different synthesis methods. The review articles of Kumunda et al. (2021) and Keyte et al. (2019) compiled the main synthesis methods for the preparation of graphene-based materials. Graphene synthesis can be performed through different approaches including mechanical exfoliation, epitaxial growth on crystalline metal, epitaxial growth on silicon carbide materials, CVD method using metal foils, and chemical synthesis from graphite oxide (Figure 3). A typical mechanical exfoliation method is the Scotch tape method. Although the CVD method generates anatomically pure graphene, it is not feasible for industrial application. Another emerging method is the electrochemical exfoliation method which provides graphene of good quality but with the presence of defects. Regarding the preparation of graphene oxide (GO), a modified Hummers method was mentioned consisting of aggressive oxidation of graphite in a concentration solution of H2SO4 and KMnO4 which results in a high yield of GO. The GO can be reduced to form reduced graphene oxide (rGO) via a thermal or chemical reduction. Moreover, the functionalization of graphene materials with N can be done with methods including CVD with ammonia gas, nitrogen plasma annealing of graphene or thermal heating of GO with melamine.
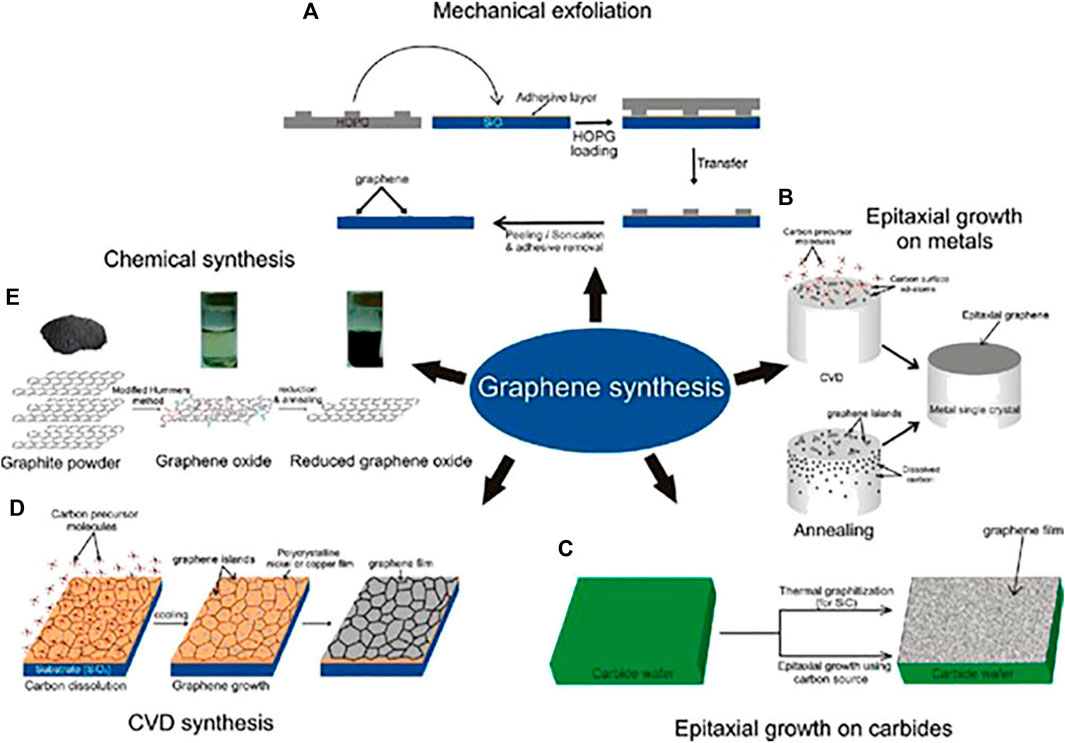
Figure 3. Schematic illustration of different graphene synthesis approaches, (A) mechanical exfoliation of graphene flakes, (B) epitaxial graphene growth on metal crystal, (C) epitaxial growth on carbon sources like silicon carbide wafers, (D) CVD method utilizing metal foils to prepare graphene possessing large surface area, and (E) chemical synthesis of graphene from graphite oxide. Reprinted from Kumunda et al. (2021) Electrochemical Detection of Environmental Pollutants Based on Graphene Derivatives: A Review. Front. Mater. 7:616787.
Elumalai et al. (2019) reported an outstanding one-pot synthesis method that simultaneously generates graphene nanosheets, from the graphite exfoliation, and co-doped with N and B. This was performed by micro-plasma discharge generated by an applied voltage to a graphite tip submerged in liquid plasma exfoliation (SLPE) which contains acetonitrile and sodium tetraphenylborate.
Although graphene-based materials possess interesting properties, for certain uses it is necessary to employ diverse approaches to promote their applicability such as the combination with other carbon-based materials. Regarding this fact, Kouloumpis et al. (2015) developed a method for creating a composite consisting of organo-modified GO layers with pure Buckminsterfullerece molecules hosted in the interlayer space. The outstanding method is a combination of the Langmuir-Schaefer (LS) deposition technique combined with two self-assembly steps. In another report, Salavagione et al. (2018) reported diverse methods to prepare functional fibers and fabrics that contain graphene. Generally, graphene is incorporated into a polymer solution that is later subjected to wet spinning or electrospinning to obtain the composite fibers. On the contrary, melt spinning was reported to be employed for the fabrication of conducting textile fibers. On the other hand, Yasri et al. (2014) prepared composites of partially oxidized graphene flakes (po-Gr) with poly(3,4-ethylenedioxythiophene)/poly(styrenesulfonate) (PEDOT:PSS). The po-Gr flakes were obtained from the electrochemical exfoliation of a graphite sheet in an HClO4/NaCl solution, and then, the po-Gr flakes were dispersed in the polymer solution with a probe sonicator to obtain the composite. Moreover, graphene can also be used in the preparation of fibers. In this sense, previous studies reviewed by Cesano et al. (2020a). Reported the preparation of fibers composed of CNTs and graphene through electrospinning or wet-spinning procedures. Moreover, the functionalization of carbon fibers with GO and CNT materials is also an interesting way of preparing fiber composites as was reviewed by Keyte et al. (2019). Electrophoretic deposition (EPD) is a popular method to modify the carbon fiber surface with GO and CNT. Moreover, the samples can be prepared with epoxy resin to reinforce them. To favor the incorporation of graphene into the carbon fiber, this needs to be previously treated chemically to create oxygen groups such as hydroxyl and carboxyl functional groups. These composites can also contain particles such as the CF/Ag/GO/epoxy through three different steps: 1) deposition of Ag particles on carbon fibers via electrophoresis; 2) addition of the GO through EPD; and 3) mixing with epoxy. Other interesting methods to functionalize the surface of carbon fibers are dipping and spraying techniques.
Graphene-based materials can be interesting supports for metal oxide nanoparticles or for composites preparation due to their properties. In this context, Orfanakis et al. (2018) reported the synthesis of the GO/γ-Fe2O3 composite through an incubation for 24 h under stirring of both components modified with oleylamine (OA). The presence of OA generates surface groups that enhance hydrophobic interactions. Gouda et al. (2020) also synthesized graphene-based materials containing iron oxide through an inverse co-precipitation method. The reduced graphene oxide used for this composite resulted from the hydrothermal treatment of polyethene terephthalate (PET) from plastic bottle waste, making it a sustainable rGO. The review article elaborated by Wu et al. (2020) compiled diverse MnO2/graphene composites prepared through microwave irradiation, low-temperature hydrothermal treatment, in situ reduction method and electrospinning technology. Interestingly, metal oxides can also be used as substrates for the synthesis of graphene composites such as the LiNbO3 perovskite (LN) (Xu et al., 2020). In this case, the layers of graphene were synthesized by annealing a post-carbon-implantation sample that consists of a layer of Ni over the LiNbO3 perovskite. As the graphene is formed in the interface of Ni/LN, the removal of Ni leads to the formation of graphene/LN composite. Apart from metal oxides, Si can be also used for preparing graphene. Tsirka et al. (2018) reported the preparation of graphene flakes/Si composite from the deposition of graphene oxide flakes on Si substrate through the Langmuir-Blodgett method. Then, the layers of graphene flakes were formed from GO through a combination of chemical reduction, thermal annealing, healing and second thermal annealing processes.
3.3.2 Other 2D materials
Graphitic carbon nitride (g-C3N4) (CN) is another interesting 2D material that can be synthesized from precursors rich in nitrogen including melamine, thiourea and cyanamide (Santiago-Ramírez et al., 2022). There are different methods reported for the preparation of CN such as the hydrothermal method, photo deposition procedure and pyrolysis (Zheng et al., 2012). However, despite CN being already interesting as a material, there is a huge research on preparing composites between CN and other 2D materials for practical applications as was reviewed by Ong (2017). This relies on the fact that the heterojunction between both 2D materials shows better properties in terms of better electron-hole mobility due to better contact between the faces. Therefore, there are diverse examples of these composites including 2D/2D CN/transition metal chalcogenide composites where commonly the latter is grown as ultrathin films onto CN sheets such as the 2D/2D ZnIn2S4/g-C3N4 material (Ong, 2017). 2D metal oxides such as TiO2, WO3, SnSb2O6 and N-doped La2Ti2O7 have also been used for the formation of composites with CN (Ong, 2017). A 2D/2D WO3/CN material was also prepared through a hydrothermal and deposition-heating technique, whereas the 2D/2D N-doped La2Ti2O7/CN material was synthesized by a facile two-step hydrothermal method and a thermal treatment. Moreover, 2D/2D graphene/CN nanocomposites have been developed through different methods such as a one-step impregnation-thermal reduction process using as precursors graphene oxide and urea (Ong, 2017). However, metals can also be supported on CN just as Navlani-García et al. (2019) reported in their review article. The metal/CN composites were commonly prepared by impregnation with the metal precursors and subsequently, a reduction with NaBH4 was performed.
Interestingly, composites of CN with chitosan (CS) are also attractive. Consequently, different synthesis methods have been developed and compiled in the review article of Santiago-Ramírez et al. (2022). The main synthesis methods described are 1) direct polycondensation followed by the sol-gel method; 2) calcination followed by the simple solution cast method; and 3) condensation-polymerization followed by the casting method. In general, the presence of CS improves the properties of the CN material. However, the as-prepared composite had some drawbacks related to the formation of aggregates and chemical damage in an acidic pH. Therefore, to mitigate these disadvantages, the addition of TiO2 to the composite through a two-step method of electrospinning was proposed.
On the other hand, among the chalcogenides, molybdenum sulfide is an interesting 2D material that attracts significant attention due to its properties. However, these solids should be synthesized in the form few-layered materials and deposited on carbon materials to make the most of their excellent properties. In this sense, Tzitzios et al. (2018) reported the preparation of MoS2/r-GO via a facile liquid phase method. Firstly, the r-GO was functionalized with oleyl amine via probe sonication, then it reacted with (NH4)2MoS4 in hot oleyl amine under a high-purity N2 to form the composite. The functionalization is necessary for the growth of flat MoS2. Another strategy to enhance the quality of MoS2 structure was performed by Li et al. (2021), who observed that the existence of N-doped carbon promotes the formation of few-layered MoS2 structures in the solvothermal synthesis of MoS2@NC hybrid material. On the other hand, Jangir et al. (2019) prepared a nitrogen-doped reduced graphene oxide matrix from Tassar silk cocoon containing Li and S. Then, Mo was introduced in the carbon matrix which produced the formation of MoS2 layers on the surface of the material.
Finally, MXene materials formed also part of this section because these are defined as 2D layered materials of early transitional metal carbide/nitride or carbon nitrides (Kallumottakkal et al., 2021). These materials are formed from the etching of the A layers from the MAX phases (M = Ti, V, Cr, Nb or Mo; A = Al, Si, Sn or In; X = C or N). The etching methods used for the preparation of MXenes can be chemical etching, molten salt etching and electrochemical etching. These are materials that are gaining great interest nowadays.
3.4 3D carbon materials
Three-dimensional carbon materials are materials with a three-dimensional sp2 carbon structure which includes materials such as activated carbons (AC) (where an important number of examples can be found), carbon schwarzites (Boonyoung et al., 2019), ordered mesoporous carbon (Khan et al., 2020), carbon networks (Worajittiphon et al., 2015; Ishii et al., 2019), graphene aerogels (Kotal et al., 2016), metal-organic frameworks (Zheng et al., 2021) and diamond-based materials (Brosler et al., 2023).
3.4.1 3D carbon materials derived from biomass
Regarding the precursor used for the synthesis, the preparation of carbon compounds from biomass waste is an outstanding topic in terms of sustainability and encompasses a green initiative to produce materials with interesting properties for different applications. In this sense, several review articles about the valorization of biomass into carbon materials have been published in the Carbon-Based Materials section. Mukherjee et al. (2022) reviewed the synthesis of the so-called biochar from lignocellulosic biomass where the three major components are hemicellulose, cellulose and lignin. Firstly, it is important to define biochar which is a carbon material with a high degree of aromatization obtained via thermochemical or biochemical conversion methods and prepared from biomass. In this review article, different pyrolysis treatments to obtain biochar from biomass waste were reviewed, including slow, intermediate, and fast pyrolysis approaches. These three types of pyrolysis produce biochar with different physico-chemical properties and yields in terms of solid, liquid and gas products (Figure 4). Moreover, the pyrolysis temperature, residence time and heating rate of the process together with the biomass nature are determining parameters for preparing a biochar with specific properties for different applications. Concerning textural properties, biochar has undeveloped porosity and very low pore volume, thus some strategies are mandatory such as the treatment at high temperatures during slow pyrolysis to get some porosity development. However, this approach needs to be optimized and depends on the biomass nature.
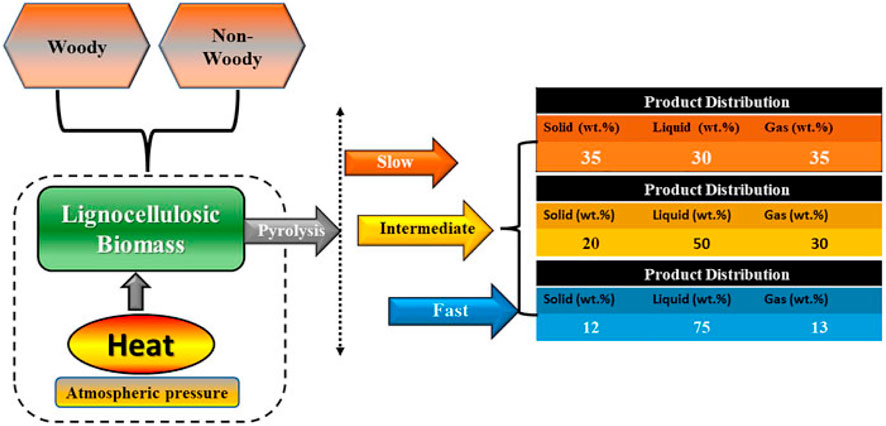
Figure 4. Influence of the pyrolysis type on the final product distribution. Reprinted from Mukherjee et al. (2022) Synthesis of Biochar From Lignocellulosic Biomass for Diverse Industrial Applications and Energy Harvesting: Effects of Pyrolysis Conditions on the Physicochemical Properties of Biochar. Front. Mater. 9:870184.
Post-synthesis approaches are the most employed approach to produce AC materials which have great textural properties, together with high thermal and chemical stabilities. In this sense, Rosas et al. (2014) compiled different methods for activating carbon materials synthesized from lignin via chemical or physical approaches. The review article focused on lignin-based materials due to lignin representing 30% of all non-fossil organic carbon on Earth. For the chemical activation, they analyzed the activation with ZnCl2, alkali metal compounds (NaOH, KOH, Na2CO3, and K2CO3) and H3PO4. The activation with KOH and NaOH was reported to give AC with surface area values close to 3,000 m2 g-1, whereas the H3PO4 activation produced porous structures with high surface acidity due to oxygen-phosphorus-containing groups. On the other hand, Quílez-Bermejo et al. (2022) reviewed the preparation of carbon materials from biosourced phenolic molecules (lignin and tannin) or from phenolic resins produced by combining the phenolic molecules with hydroxymethylfurfural (HMF). Two types of carbonization approaches can be applied such as carbonization at high temperatures under an inert atmosphere or hydrothermal carbonization under mild conditions. The former is more common for the preparation of carbon materials from phenolic resins. This approach also includes a curing process to increase the carbonization yield. Generally, the as-prepared carbon materials require an activation step to improve their textural properties.
It is also important to highlight that not all biomass components can form porous biochars. The hypothesis and theory article of Ilnicka and Lukaszewicz, (2015) suggested that cellulose and chitosan cannot form porous carbon materials by themselves, making activation procedures mandatory to create porosity. However, other components such as hemicellulose or peptides could act as natural soft templates.
Different examples of carbon materials from biomass waste can be found in research articles. Parthasarathy et al. (2021) reported the synthesis of biochar and activated carbon materials from discarded waste bamboo furniture. The biochars were prepared in a furnace under N2 giving materials with surface areas between 88.5 and 350 m2 g-1. However, a chemical activation with H2SO4 was necessary to increase the surface area up to 1106 m2 g-1. This fact shows the importance of the activation process of biochar to improve its textural properties.
In this sense, Karami et al. (2022) developed an activated carbon from corn biomass waste. This carbon material resulted in the conversion of vomitoxin-contaminated waste corn grains into added value activated carbon via pyrolysis and CO2 activation at 900°C. Another potential agricultural residue is olive stones, Calvo-Muñoz et al. (2016) reported the preparation of activated carbon through pyrolysis and CO2 activation at 700°C. Meanwhile, Ruiz-Rosas et al. (2019) designed a phosphorus-containing activated carbon from olive stones. For this purpose, residues were impregnated with H3PO4 and subsequently activated at 500°C under N2 flow. Later the material was thermal treated under diluted air at high temperature which results in a material with interesting surface chemistry and porosity development. Another interesting example of chemical activation with H3PO4 is the activated carbon of 2,550 m2 g-1 obtained from lignin reported by Moulefera et al. (2020). The activated carbon was synthesized through two-step thermal treatments consisting of a first oxidative stabilization at 200°C in air and a second one under N2 at 400°C.
As it can be deduced, any biomass waste can be converted into a carbon material, thus unlimited materials could be formed leading to different physicochemical properties. Even municipal solid wastes could be used as a carbon source. Interestingly, Yu et al. (2018) designed N-doped carbon materials from waste cigarette butts via one-step facile carbonization under the N2 atmosphere and subsequent elimination of inorganic impurities with a 10% HCl solution.
Activated carbon materials already have interesting properties in terms of surface chemistry and textural properties. However, to improve their performance and increase the number of possible applications, it is necessary to dope or support the activated carbon materials with heteroatoms or metal-based compounds. The introduction of heteroatoms such as P or N can be performed from the activating agent or taking profit of the N content of the starting material, respectively. However, the incorporation of nitrogen atoms into the carbon matrix can be done by utilizing different N-based compounds. In this sense, Bumajdad et al. (2023) elaborated a review article describing different approaches to increase the N content. The importance of the N precursor was remarked, offering diverse reagents such as amines, amides, N-based surfactants and nitric acid as potential agents. Among them, it was concluded that the amide family are the most interesting because they offer significant N-functionalities for the carbon matrix. On the contrary, the review article elaborated by Salinas-Torres et al. (2019) described other procedures to introduce or create N-functional groups including a reaction with an N-containing source, carbonization of a N-containing precursor and thermal treatment of N-containing precursors. These methods contribute to the formation of N-functionalities such as pyridine, quaternary N, pyrrole and oxidized N species which are differently important for certain applications. In the case of metal oxides, the review article elaborated by Wu et al. (2020) provides a synthesis method to prepare MnO2/activated carbon composites via an electrodeposition technique. However, easier methods can be applied for the preparation of composites such as the physical mixture reported by Carmona et al. (2016) in the design of WO3/activated carbon.
From the above discussion, we can conclude that recently there has been a huge interest in developing carbon materials from renewable carbon sources such as biomass wastes to contribute to zero net CO2 emissions. However, it cannot be overlooked that biomass waste-based materials do not have yet certain features to compete with carbon materials prepared from other sources in some applications. In this sense, Piñedo-Prado et al. (2016) designed activated carbons from Spanish anthracite via KOH activation using a ratio of 4:1 (activating agent/precursor) at 750°C in an N2 atmosphere. As a result of the activation, the as-prepared material has a high content of surface oxygen-containing functional groups (SOGs) and a high apparent surface area which are important parameters for specific applications. Regarding SOGs, Berenguer and Morallon, (2019) investigated different chemical and electrochemical oxidative functionalization procedures in microporous carbon materials. The results indicate that both methods decrease the specific surface area through the partial or full blocking of micropores with SOGs. However, the electrochemical oxidative method is preferable because it can be done at room temperature which allows for control of the degree of oxidation and minimizes the degradation of the carbon structure.
3.4.2 3D carbon materials derived from polymers
Another source of activated carbon materials could be polymers as described by Cesano et al. (2019). Microporous carbon microspheres were obtained from a thermal treatment at 800°C under N2 flow of poly(4-ethylstyrene-co-divinylbenzene) (PS-co-DVB) microspheres. Firstly, the polymer was impregnated with ZnCl2 i) to prevent collapse and ii) to act as an activating agent. Moreover, to prepare magnetic carbon materials, the porous carbon microspheres were impregnated with an iron solution with a subsequent thermal treatment.
In the case of activated carbons, it is very difficult to control the structure of the materials which are considered as amorphous or low-crystallinity carbon forms. One approach for synthesizing carbon materials with an ordered 3D structure is through the use of zeolites as templates. In this sense, Ishii et al. (2019) reported the synthesis of a three-dimensional graphene nanoribbon network commonly named zeolite-templated carbon (ZTC). Firstly, a furfuryl alcohol/zeolite composite was prepared via drop casting. Then, the composite was thermally treated under nitrogen flow with 2 vol% in propylene, being FA and propylene both carbon sources. The as-prepared ZTC can be oxidized electrochemically in H2SO4 to create oxygen groups achieving a good control over the surface chemistry. N-doped ZTC was also prepared by employing acetonitrile (ACN) for both the drop-casting and the carbonization treatment with a 3 vol% of ACN in N2. In all cases, the zeolite was removed with hydrofluoric acid. Another example of the use of zeolite as a carbon template is the carbon material reported by Shutjajew et al. (2019). The carbon material was reported to employ Aerosil fumed silica as a template and sucrose as carbon source. Zeolites can also be used for the preparation of carbon schwarzites which are defined as carbon structures with negative Gaussian curvatures. Boonyoung et al. (2019) reported the synthesis of these structures through a chemical vapor deposition (CVD) on a zeolite Y (FAU) template which was previously synthesized by hydrothermal method. The study concludes that for effective carbon loading of carbon, a low acetylene gas concentration and prolonged periods time of CVD are necessary. Although zeolites are the most common templates used for the preparation of carbon materials, materials such as aluminum oxide (Castro-Muñiz et al., 2016) or copolymers (Khan et al., 2020) can also be employed for this purpose. In this context, Castro-Muñiz et al., 2016 reported the preparation of carbon-coated anodic aluminum oxide films through a CVD process using acetylene gas at 20 vol% in N2 flow as a carbon source. Previously, the anodic aluminum oxide films were prepared by employing a two-step anodization process. Khan et al. (2020) reported the formation of triamine-iron-mesoporous carbon material via the soft Triblock F-127 copolymer template. The mesoporous carbon material was obtained after thermally treating a solution containing the phenolic resin and the copolymer template. The incorporation of iron and triamine in the carbon material was performed afterwards through thermal treatment and reflux, respectively.
As mentioned above, 3D materials can be developed from a network of existing carbon materials. In this sense, Worajittiphon et al. (2015) developed a network of highly ordered, segregated single-walled carbon nanotubes (SWCNTs) induced by polymerized latex templates. The assembly of the SWCNTs in the matrix resulted in hexagonal (2D) and honeycomb (3D) networks. The preparation of the composite was performed by a tip sonication of a dispersion containing both materials in an ice bath.
Following the idea of a network, 3D carbon materials can be formed interestingly by pillaring carbon materials. Matsuo et al. (2015) reported the synthesis of pillared carbons with different pillar densities from the thermal reduction of graphite silylated with methyltrichlorosilane. A further study by Matsuo and Araki (2017) reported the use of different silanes to obtain pillared carbon thin films with hydrophobic pillars that prevent the intercalation of smaller water molecules. In this case, octyltrichlorosilane and then dimethyldichlorosilane were used for the silylation. On the other hand, Chen and Song (2016) described a process to obtain a MWCNT yarn. This yarn was prepared through a spinning technique to form super-aligned MWCNTs, then the resulting yarn was soaked in ethylene glycol to make it stiffer and more tightly packed. Finally, it was thermally treated at a low temperature in air to remove the solvent.
Carbon frameworks are also important 3D structures, so there is a huge interest in their synthesis. Regarding the synthesis method, Han et al. (2021) designed a coral-like carbon framework structure doped with B and N via a simple heat treatment of urea, polyethylene glycol and boric acid. The temperature used for this treatment was 900°C under a N2 atmosphere, and subsequently, the material was washed with HCl to remove boron trioxide species. Other framework structures that attract interest for their versatility in the synthesis of materials with diverse compositions and properties are metal-organic frameworks (MOFs). The review article of Zheng et al. (2021) compiled diverse examples of MOFs containing metals such as Mn, Cu, Zn, Co or Ni. Some examples of synthesis methods are named including a solvothermal method together with an etching process or EPD.
Another example of 3D structure is that of carbon foams that offer the possibility of tuning the mechanical and electromechanical properties. Kordas and Pitkänen (2019) designed carbon foams by pyrolyzing melamine foam under an N2 flow. Then, the Na+ impurities were removed by rinsing and sonicating in deionized water. To create carbon nanotubes/nanofibers in the pores of the carbon foams, the latter was impregnated with a solution of nickel acetylacetonate followed by a thermal treatment under a flow of a mixture of acetylene and 15% H2/Ar.
In this section, polymer-based materials such as thermosets are also included. Dinu et al. (2023) reported the outstanding preparation of sustainable and recyclable thermosets which were synthesized by crosslinking triglycidyl ether of phloroglucinol (TGPh) with either hexahydro-4-methylphthalic anhydride (HMPA) or methyl nadic anhydride (MNA).
3.4.3 3D carbon materials derived from other methods/materials
Graphene aerogels (GAs) are also classified as 3D materials because these are a conducting network of graphene nanosheets with excellent textural properties, making them interesting for different applications. Therefore, different synthesis methods have been developed and these are included in the review article elaborated by Kotal et al. (2016). The synthesis methods were divided into two main groups: 1) self-assembly methods and 2) template-directed approaches. Self-assembly methods are the direct self-assembly of GO, the hydrothermal-reduction-induced self-assembly, the cross-linking agent-induced self-assembly, the reducing agent-assisted self-assembly and polymer-assisted self-assembly. Meanwhile, template-directed CVD approach, ice template, emulsion template, SiO2 template and lithographical template are classified into the template-directed approaches. An example of GAs synthesized through a self-assembly method was reported by Vrettos et al. (2018). In this research article, it was observed that aliphatic and aromatic diamines are excellent gelation promoters to form stable aerogel monoliths in the hydrothermal synthesis of GAs. Also, these functional groups acted as crosslinkers of GO sheets and as reducing agents.
The diamond structure is a well-known 3D carbon structure, being the boron-doped diamond (BDD) materials highly used for electrochemical applications. The review article published by Brosler et al. (2023) detailed the synthesis of these electrodes by CVD being able to control properties such as the sp3/sp2 ratio, thickness, boron doping level, growth rate and grain size. It also compiled the importance of metals as substrates for the CVD process, suggesting that Ti can be a suitable substrate due to its lower cost, high conductivity, corrosion resistance, and excellent mechanical properties. The CVD process is performed under a mixture of methane (low concentration) with molecular hydrogen. However, the introduction of other gases in the mixture such as Ar or O2 (low concentration) could prevent the enlargement of the crystallites and limit the impurity incorporation, respectively. Among the different CVD configurations, microwave plasma CVD (MPCVD) and hot-filament CVD (HFCVD) are highlighted due to their multiple advantages. MPCVD have several advantages related to higher growth rates, higher phase purity and better reproducibility. However, the wavelength of the microwave radiation can limit the plasma area making it difficult to use at large scale. In this sense, despite the limitation brought forth by the degradation of filaments, HFCVD is industrially preferable due to its simplicity, lower cost and the possibility of growing films over larger surface areas. Finally, gaseous boron sources are preferable because they offer control of the boron doping level such as trimethylborane, trimethylborate and diborane.
Progress in the development of carbon materials from the perspective of the Frontiers in Materials journals sheds a very promising picture in this field. On the one hand, there is a very strong drive in the search for affordable and sustainable precursors for the preparation of advanced carbon materials, with their dimensionality encompassing the whole available range, which speaks for a clear focus on producing carbon material-based samples in a clean and cost-efficient way. On the other hand, the advent of novel carbon forms (being perhaps graphene the most notorious in the past 10 years) has also spurred researchers to look for ways to use these forms as building blocks to develop hierarchical and structured carbon forms with unprecedented properties.
4 Applications
The outstanding properties of carbon materials give them a main role in applications of diverse nature. The present section is devoted to summarising the applications of carbon-based materials that have been included in the review articles and research articles of the Carbon-Based Materials section. As it can be seen from the information included below, most of these contributions emphasised the role of carbon-based materials in a broad range of applications such as catalysis and photocatalysis, wastewater treatment and environmental protection, electrochemical applications, and biological and medical applications. The application of carbon materials in some other fields of research has also been included in some publications of the Carbon-Based Materials section and they have been collected in the last subsection.
4.1 Catalytic applications
Catalysis is a fascinating field of research in which carbon materials are widely used. The unique properties of carbon materials make them ideal catalytic supports or catalysts themselves, but they are also used as additives to modify/enhance the catalytic or photocatalytic performance of certain materials for a given application.
Some interesting review articles are devoted to revisiting the role of carbon-based materials for important catalytic and photocatalytic applications. For instance, Valero-Romero et al. (2021) reviewed the utilisation of carbon support for the Fischer-Tropsch synthesis by classifying the results found in the literature on the basis of the carbon material shape and structure. The results achieved with carbon materials such as activated carbons, ordered mesoporous carbons, carbon nanotubes, carbon nanofibers, carbon spheres, graphene, graphite, and diamond were included while revisiting the effect of metal-support interactions and the porous texture of activated carbon, the presence of metal promoters, functionalisation of the carbon materials, method used for the preparation of the catalysts, and metal particle size, among others. Figure 5 summarizes the different carbon support materials used in the Fischer-Tropsch synthesis classified according to the carbon structure and morphology that were included in that review article.
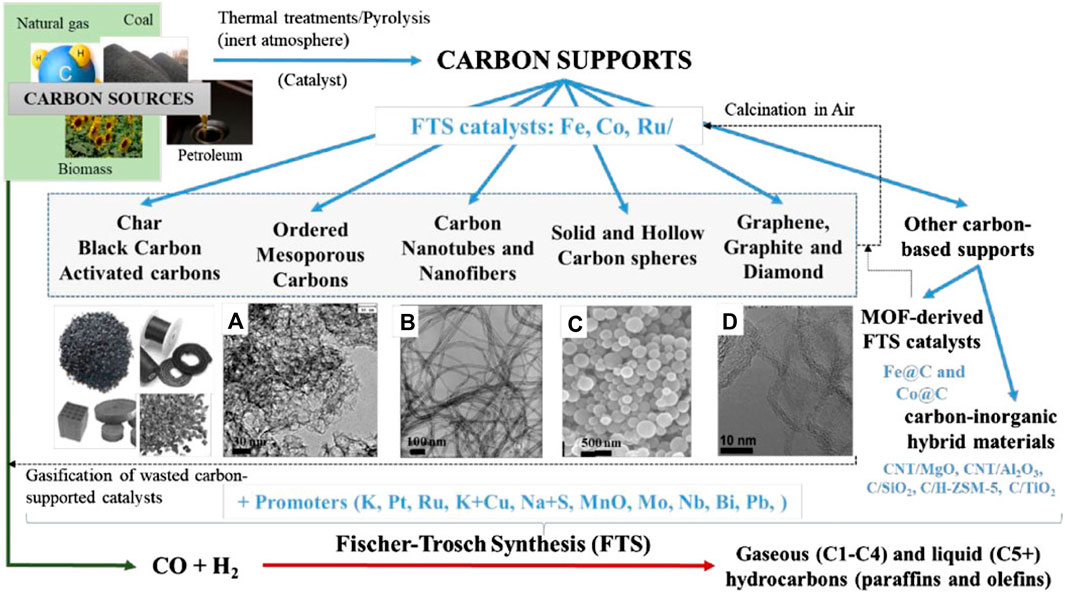
Figure 5. Carbon-based supports for FT catalysts. TEM images (A, B, D) are adapted from (A) Valero-Romero, M.J., Márquez-Franco, E.M., Bedia, J., Rodríguez-Mirasol, J., and Cordero, T. Hierarchical porous carbons by liquid phase impregnation of zeolite templates with lignin solution. Microporous and Mesoporous Materials, 2014, 196, 68-78; (B) Serp, P., Corrias, M., and Kalck, P. Carbon nanotubes and nanofibers in catalysis. Applied Catalysis A: General, 2003, 253, 337-358; and (C) Chernyak, S.A., Stolbov, D.N., Ivanov, A.S., Klokov, S.V., Egorova, T.B., Maslakov, K.I., Eliseev, O.L., Maximov, V.V., Savilov, S.V. and Lunin, V.V. Effect of type and localization of nitrogen in graphene nanoflake support on structure and catalytic performance of Co-based Fischer-Tropsch catalysts. Catalysis Today, 2020, 357, 193-202. Reprinted from Valero-Romero et al. (2021) Carbon-Based Materials as Catalyst Supports for Fischer–Tropsch Synthesis: A Review. Front. Mater. 7:617432.
Navlani-García et al. (2019) summarised some of the most representative studies on carbon-supported heterogeneous catalysts for the production of hydrogen from the dehydrogenation of formic acid. These studies included the use of commercial activated carbons, carbon black, carbon nanospheres, carbon xerogels, graphene, g-C3N4, among others. Important features of the catalysts, such as the composition of the metal active phases, the size of the nanoparticles, and the functionalisation of the supports were considered. In a review article Ong (2017) highlighted the role of g-C3N4 in heterojunction composites for photocatalytic applications. Properties of said material, such as visible-light absorption, high thermal and chemical stability, and appropriate band structures were mentioned. Several composite materials formed by 2D/2D structures and their photocatalytic application in water splitting, CO2 reduction, and degradation for pollution were covered in that article. Santiago-Ramírez et al. (2022) also pointed out the benefits of using g-C3N4-based materials for photocatalytic applications. In that case, the potential applications of g-C3N4-chitosan composites in the photodegradation of methyl orange and methylene blue, photocatalytic reduction of 4-nitrophenol, and the photocatalytic production of hydrogen were considered, among other fields in which the use of such composites are also of great interest. Fan (2015) highlighted the benefits of using graphene as catalytic support in heterogeneous catalysts and underlined its unique physical and chemical properties, such as its high surface area, 2D structure, possibility of surface functionalisation, and high electron mobility. The photocatalytic applicability of carbon quantum dots in the degradation of methylene blue was mentioned by Magesh et al. (2022) in a review article in which several aspects related to those materials (synthesis, properties, and potential applications) were covered.
Some research articles dealing with the use of carbon materials in catalytic applications may also be found. That is the case of the article reported by Carmona et al. (2016), who designed photocatalysts based on WO3 and explored the enhancement of the performance towards the photocatalytic degradation of phenol and rhodamine upon incorporation of carbon materials as additives. For that purpose, a low content (2 wt%) of three carbon materials (i.e., nanoporous carbon, hydrochar, and multi-walled carbon nanotubes) with different properties (porosity, structural order and surface chemistry) were incorporated in the different photocatalysts. The enhancement of the photocatalytic performance under simulated solar light was attributed to the following aspects: 1) the chemical and textural features of the carbon materials; 2) the formation of reactive oxygen species upon irradiation of the photocatalysts; 3) the role of the carbon materials as acceptors, improving the separation of charge through the delocalisation in the π-electron density of the graphitic sheets.
4.2 Wastewater treatment and environmental remediation
The role of the features (porosity, surface functional groups, etc.) of different carbon materials in their behaviour in water treatment and environmental remediation are clearly reflected in the articles compiled in the Carbon-Based Materials section. For instance, Karami et al. (2022) reported the preparation of activated bio-char synthesised from waste corn biomass and the application of the developed materials in the adsorption of three model molecules (i.e., methylene blue, methyl orange, and ibuprofen). Evaluation of the adsorption capacity revealed that these materials displayed a behaviour similar to those achieved by commercial activated carbons. Khan et al. (2020) explored the performance of ordered mesoporous carbon, amine-modified ordered mesoporous carbon and iron-ordered mesoporous carbon for the adsorption of arsenic and lead from aqueous solution. The performance of the developed materials was assessed by fitting the data to the Langmuir non-linear model and Freundlich non-linear model. Brosler et al. (2023) presented a discussion of the most relevant parameters that influence the performance of boron-doped diamond electrodes in the electrooxidation of water pollutants. In the case of the study reported by Ruiz-Rosas et al. (2019) the effect of two important features of the carbon materials, such as the porosity and the surface chemistry, towards the performance of P-containing activated carbon for the removal of paracetamol was explored.
In a more electrochemistry-focused paper, Beralus et al. (2014) employed a commercial granular activated carbon for the electroadsorption of arsenic from natural water on a electrochemical filter-press cell. The results indicated that the carbon material under specific polarization conditions can adsorb As species down to the ppb range, making it feasible for operations under real conditions. Meanwhile, Yasri et al. (2014) designed a po-Gr/PEDOT:PSS nanocomposite deposited on a glassy carbon electrode for the detection of mercury ions (Hg2+) in water samples. The presence of po-Gr enhances the electrocatalytic response of PEDOT:PSS. Moreover, the as-prepared materials exhibited a stable, selective and reproducible determination of Hg2+ at micromolar levels.
The review article published by Bumajdad et al. (2023) treats the use of nitrogen-enriched activated carbons derived from plant biomasses and their applications in wastewater treatment. Several pollutants, including pharmaceutical pollutants (chlorpheniramine, ofloxacin and ibuprofen), heavy metals (Pb(II) and Cr(VI)), volatile organic compounds (toluene) organic pollutants (bisphenol A, phenol, acetaminophen, and sulfamethoxazole), were considered in that article. The review article of Kumunda et al. (2021) is devoted to revising the advances in the electrochemical sensing of environmental pollutants, including heavy metal ions, pesticides, nitroaromatics, and other emerging contaminants (namely, triclosan, parabens, and bisphenol A). That review article includes the synthesis of graphene-based materials (graphene, graphene oxide, reduced graphene oxide, and nitrogen-doped graphene) and the use of several electrodes modified with graphene-derived nanocomposites.
Calvo-Muñoz et al. (2016) prepared porous carbon materials with different morphologies and structural properties from biomass waste (namely, lignin, olive stones, wood, and denim cloth) and they were evaluated in CO2 capture in post-combustion conditions. Carbon materials, in the form of granular carbon, fibres and cloths were prepared and their adsorption capacity was assessed by equilibrium studies and dynamic adsorption-desorption studies. Among the investigated applications, the carbon fibre prepared from Alcell lignin displayed outstanding behaviour and also good regeneration capacity.
4.3 Electrochemical applications
Carbon-based materials have a significant role in the development of electrochemical devices because these are used in supercapacitors, batteries or fuel cell devices. Therefore, carbon materials are important for a transition towards a more sustainable and eco-friendly way of producing and storing energy. Therefore, a significant number of contributions on these topics were published in the Carbon-Based Materials section. Regarding energy applications, the editorial article elaborated by Cesano et al. (2020b) revealed an exponential growth of articles related to energy materials including applications in supercapacitors, lithium-ion batteries (LIBs), and electrochemical reactions such as OER. On the other hand, Kotal et al. (2016) reviewed different applications of graphene aerogel-based materials including sensors, actuators and energy storage such as batteries and supercapacitors. Meanwhile, Quílez-Bermejo et al. (2022) described in their review article the applications of the carbon-based materials obtained from biosourced phenolic resins or phenolic molecules. These materials can be used in supercapacitors and rechargeable batteries including lithium-ion batteries (LIBs) and sodium-ion batteries (NIBs).
Regarding supercapacitors, the review of Díaz-Delgado and Doherty, (2016) detailed the importance of combining carbons, ionic liquids and quinones for the development of these electrochemical devices for applications in smart textiles. Additionally, the performance of the supercapacitor can be enhanced by increasing the pseudocapacitance of the carbon materials either by introducing metal oxides or by doping with heteroatoms. Concerning metal oxides, the review article of Wu et al. (2020) reported the use of MnO2 to form diverse MnO2/carbon composites with carbon materials like CNT, graphene, CNF, and activated carbon as promising electrodes for supercapacitors. Meanwhile, Poirot et al. (2022) reported the TiO2/CNTs as an interesting electrode for supercapacitors due to the high pseudocapacitance provided by the metal oxide. In the case of doping, Han et al. (2021) designed a B,N dual doped coral-like carbon framework (BN-CCF) which offered an excellent performance as an electrode in supercapacitor. This was ascribed to the porous structure, high hydrophilicity and enhanced pseudocapacitance provided by B-containing groups.
Zheng et al. (2021) also reviewed the use of MOF nanomaterials as supercapacitors. This review article reported different pristine MOFs, MOF-derived carbons and CNTs for their use as electrodes for supercapacitors. Moreover, it was emphasized the use of transition metal oxides to enhance the performance of carbon materials.
Carbon materials can also be utilized to study other parameters involved in the functioning of supercapacitors such as the electrolyte or type of supercapacitor. Karamanova et al. (2021) designed supercapacitors based on the commercial YP-50F to study the influence of the lithium and sodium organic electrolytes on the capacitance performance. Shutjajew et al. (2019) reported the advantage of using room-temperature ionic liquids (RTILs) as electrolytes in carbon materials with high mesopore volumes rather than micropore ones. The RTIL phase transitions occurring inside the mesopores were also observed to contribute to the energy storage in supercapacitors. Whereas, Piñedo-Prado et al. (2016) prepared asymmetric supercapacitors from activated carbon with energy density values comparable to commercial and symmetrical supercapacitors. Moreover, the measurements were done in an aqueous medium which is eco-friendlier than the organic medium used for commercial supercapacitors. The development of electrodes for LIBs is an issue of great interest due to the encouraging performance of these devices. The research article elaborated by Yu et al. (2018) reported a N-doped carbon anode for LIBs with a satisfactory electrochemical performance due to the presence of N and O functional groups. Meanwhile, Li et al. (2021) reported that N-doped carbon materials can boost the formation of a few-layered MoS2 structure during the synthesis of the F-MoS2@NC material used for anodes of LIBs. Moreover, the carbon material apart from improving the electron transport capacity, avoided the agglomeration of MoS2 nanocrystals and reduced their stress in the charge/discharge process. Jangir et al. (2019) designed an N-doped reduced graphene oxide matrix with Li, S and Mo as a cathode for LIBs. The charge process is governed by the “induced electron transfer” produced by the different components of the electrode.
The oxygen reduction reaction (ORR) that occurs in the cathode of a fuel cell requires an electrocatalyst to proceed, being this the most demanding reaction compared to the hydrogen oxidation reaction produced in the anode. Thus, it is important to develop materials for ORR. In this sense, Maboya et al. (2022) reported the synthesis of CNTs doped with N and B which proceeded the ORR via a 2-electron pathway ascribed to the presence of pyridinic N species. Meanwhile, Gouda et al. (2020) synthesized rGO and rGO with magnetic iron oxide materials for preparing the anode and the cathode of a membrane electrode assembly (MEA). The MEA with the best performance resulted from a configuration where a gas diffusion layer (GDL) with a microporous layer that increased the mass transport through the doubler layer electrode was used.
Finally, Otero et al. (2016) reviewed the importance of faradic, capacitive and structural components in the electrochemical responses of CNTs. It was stated that any of these components can be the rate-limiting process of the electrochemical devices such as supercapacitors, smart membranes or drug delivery. Therefore, the detection of the rate-limiting process could help enhance the performance of the device. On the other hand, Lenz et al. (2020) investigated the kinetic behavior in capacitive deionization processes through a new method to interpret EIS measurements. Activated carbon fibers and carbon aerogel were selected for this study. This method could be further used for other applications such as electrochemical capacitors or batteries.
4.4 Biological and medical applications
Several carbon materials have also been applied in biological and medical applications, showing their potential in the field of nanobiotechnology. Most of the publications addressing biological and medical applications of carbon materials published in the Carbon-Based Materials are review articles. In this line, the review article of Azam et al. (2021) revisited the biomedical application of carbon quantum dots, which covers biosensing, electrochemical biosensing, bioimaging, gene delivery, drug delivery, inflammation treatment, pharmaceutical formulations, and photodynamic therapy in the treatment of cancer. The biological properties of carbon quantum dots were also mentioned in the review of Magesh et al. (2022), who pointed out the role of such carbon materials in bio-imaging for visualising the biological system in actual time, and drug delivery.
The minireview reported by Santiago-Ramírez et al. (2022) includes some of the biological applications of g-C3N4-chitosan biocomposite, which included antibacterial and antimicrobial activity.
Yang and Zhang (2019), in an attempt to extend the application of CNTs to the biological field, revisited their biodegradability by macrophages and summarized the current in vitro and in vivo research. As for the biodegradation of CNTs in vitro, the degradation by enzymes and macrophages was considered. In the case of biodegradation of CNTs by macrophages in vivo, the degradation in the lung, the brain, and the liver was covered. That review article also included some factors that affect the biodegradability of the CNTs, such as the types and lengths of the nanotubes, surface functionalization, presence of impurities, etc.
Castro-Muñiz et al. (2016) designed monolithic electrodes to immobilize the D-fructose dehydrogenase (FDH) enzyme using carbon-coated AAO films. The results indicated that the as-prepared carbon-based material immobilized properly the enzyme due to its larger pores that favors the accessibility of FDH into the nanochannels.
The following two research articles addressing the utilisation of carbon-based materials in bio application were also published in the Carbon-Based Materials section. Al-zharani et al. (2023) reported in their research article the potential of MWCNTs to target breast and lung cancer cell lines. In that study, MWCNTs were dispersed in a colloidal solution of Arabic gum, which is a natural and biocompatible polymer, and the cytotoxic effect of MWCNTs on both types of cells was demonstrated. Orfanakis et al. (2028) studied the biocatalytic activity of hybrid materials formed by magnetic iron nanoparticles and graphene oxide (and oleylamine-modified graphene oxide) used for the covalent immobilisation of β-glucosidase. That study was focused on obtaining an insight into the effects of the structural characteristics of the hybrid material on the behaviour of the β-glucosidases. It was observed that the oleylamine-containing materials showed better operational and thermal stability of the enzyme, due to the enzyme-oleylamine hydrophilic interaction.
4.5 Other applications of carbon-based materials
Aside from the applications included in previous sections, other applications of carbon-based materials have also been considered in some of the publications of this journal. The present section is devoted to collecting those applications that, while relevant, have been less profusely reflected upon in the publications included in the Carbon-Based Materials section.
The publication of Kordas and Pitkänen (2019) addressed the piezoresistivity of two carbon foams, namely, a carbon foam made by the pyrolysis of an open pore structure melamine foam, and a carbon foam obtained by growing carbon nanotubes/nanofibers in pores of the first one. The importance of those materials in mechanical strain sensing in numerous applications (i.e., analysis of body part motion, heart rate monitoring, dilatation in buildings and large infrastructure, vibration of machines, etc.) is mentioned.
Carbon materials have also been shown to be useful in sensing applications. In this sense, Matsuo and Araki (2017) prepared hydrophobised pillared carbon thin films from the thermal reduction of graphite oxide silylated with octyltrichlorosilane and then dimethyldichlorosilane, and the obtained pillared carbons were used for the detection of acetone in the presence of water vapour. Basit et al. (2022) developed ultraviolet sensors based on ZnO/CNT composites prepared from a vapour-liquid-solid method. That research article included, among other sections, the description of the synthesis of ZnO nanostructures, a detailed characterisation of the samples, the description of the mechanism behind the UV-sensing, and the fabrication of the sensor. Santiago-Ramírez et al. (2022) included in their review article a section covering the sensing applications of g-C3N4-chitosan biocomposite, which have been used as photoelectric chemical sensors.
Carbon-based materials have been also used for electromagnetic shielding and absorption. The research progress in that field was revisited in the review article reported by Kallumottakkal et al. (2021). In that review, the absorption of microwave radiation by graphene, MXenes, carbon nanotubes (CNTs), carbides, and ferromagnetic metals was covered.
The application of carbon-based materials on smart textiles was reported by Salavagione et al. (2018). In particular, that review is focused on polymer/graphene composite fibres and highlights the importance of molecular-level control of the interphase between polymer and graphene. Liu (2023) designed a boxing suit based on graphene composite fibre seamless knitted fabric that reduced the injury of boxers during training. That work is focused on the properties and structure of graphene composites and a comparison of the appearance of graphene nylon with conventional nylon was also conducted. A comparison of moisture absorption and tensile fracture of graphene nylon, graphene nylon spandex coated yarn, graphene nylon/graphene amino spandex coated yarn, nylon, and nylon coated yarn was included.
Zhang et al. (2021) highlighted the suitability of carbon nanoarchitectures (i.e., activated carbons, CNTs, and MOFs) as adsorption hydrogen storage materials. In particular, the promoting effect of MOFs on the hydrogen storage properties of MgH2 was highlighted in that article.
Mukherjee et al. (2022) in their review paper pointed out the potential application of biochar prepared from lignocellulosic biomass in industrial applications and energy harvesting. Among them, the application of biochars and biochar-derived activated carbon for soil remediation, soil nutrition and fertility, use as adsorbent for wastewater treatment, carbon sequestration, carbon dioxide adsorption, gold recovery, hydrogen storage, and electrode material for supercapacitor, was discussed.
The mini-review article of Caballero-Briones et al. (2023) compiled different applications of graphene oxide-based materials including photovoltaic and photoelectrochemical cells, coating for thermonuclear materials, electromagnetic interference (EMI) insulation, and aeronautics.
Carbon-based materials can also be useful for preparing cold cathode materials as reported by Chen and Song (2016). This research article used an MWCNT yarn as a lateral emitter showing high emission current, long-time stability and uniform line emission pattern. Nevertheless, all the applications compiled in this review involve the use of carbon-based materials in a solid state. Khan et al. (2023) designed a sustainable gas as an alternative to SF6 in High Voltage (HV) applications. The dichlorodifluoromethane (R-12) mixed with CO2 was proposed as an alternative which exhibited self-recoverability properties. Also, CO2 enhances the properties of R-12 by increasing the boiling point and the dielectric strength. Zhang et al. (2020) reported the preparation of a VACNT layer supported on Cu foil to be used as thermal interface materials (TIMs) due to the high thermal conductivity property of VACNT that maintains a mechanically flexible/compliant interface. Moreover, it was observed that plasma treatment to the VACNT layer promotes a great thermal performance under continued stresses with a reduction of thermal resistance.
The review article of Cesano et al. (2020a) is focused on revising the different electronic and electrical applications of fibers, yarns and ropes prepared from the assembling of nanoscale carbons such as CNTs and graphene. These structures are employed in conductive cables and they are expected to be used frequently in multifunctional fabrics, power cables, energy storage and sensing device fields.
5 Conclusion and outlook
Looking back from this starting point, nearly 90 articles have been published in the Frontiers in Carbon-based materials section alone during its 10 years of life. The breadth in terms of topics and perspectives clearly reflects that carbon materials may indeed be “old”, but it is beyond any shadow of doubt a family of materials that ages exceedingly well, ever growing and expanding to find applications covering all current topics which nowadays include sustainable energy generation and storage, environmental protection and biomedical applications, to name a few select examples. The articles which have been published in the aforementioned section cover all types of publications including 27 reviews and mini-reviews, 1 Hypothesis and Theory, 4 perspectives, 1 Specialty Grand Challenge, 1 Editorial, and 54 original research articles. All these articles deal with very timely topics related to the synthesis, characterization, and applications of carbon materials, which is supported by the high number of citations/views/downloads they account for (to cite one illustrative indicator, more than 830 citations gathered by the reviews and mini-reviews articles, and more than 530 citations gathered by the original research articles), which serves as an excellent indicator of the fact that the articles published in Frontiers in Carbon-based materials over the past decade depict a faithful representation of the current state-of-the-art in this exciting research area.
Regarding the synthesis methods, it can be deduced from the articles that specific methods depending on the dimensionality of the carbon material can be applied. However, more general methods such as hydrothermal and sol-gel can be used for synthesizing any carbon material due to their simplicity, low cost, speed and great yield. Among the more specific methods, the electrospinning method is preferred for the preparation of CNFs starting from a lignin solution together with the addition of H3PO4 to the solution for catalyzing the crosslinking and developing porosity. In the same way, H3PO4 is the most used chemical for the chemical activation of carbon materials due to it increases surprisingly the porosity of carbon materials. Meanwhile, the CVD method is useful for the synthesis of CNTs, graphene, boron-doped diamond and ordered mesoporous carbon. However, the necessity of developing carbon materials, including composite materials, with better properties has led to the improvement of existing methods or the creation of new ones for the preparation of carbon materials such as VLS method, SLPE process, EPD, and the chlorination method. This fact suggests that a great number of carbon materials will be synthesized thanks to the synthesis procedures that are also evolving and new synthesis equipment is available compared to 10 years ago. On the other hand, there is important progress in the selection of waste for the preparation of activated carbon, since not only biomass waste is used but municipal solid wastes can also be potential precursors such as waste cigarette butts. Therefore, the selection of wastes for activated carbon would be a challenging and motivating mission for future studies that would lead to the increase of carbon materials with outstanding properties and the reduction of waste from any origin. Finally, another point that motivates the researcher to synthesize materials is to enhance materials that are already used in industry such as the preparation of fibers and fabrics for smart textiles.
Concerning the articles dealing with the characterization of carbon materials, the main properties, both surface properties (i.e., physicochemical properties such as textural properties) and bulk properties have been covered. Most of the articles include a section devoted to the results achieved by using numerous characterization techniques, which are used to find the ubiquitous properties/performance relationship in a target application. Among them, the analysis of the textural properties of carbon materials is one of the most studied features, which has been reflected in several articles of great relevance. Other studies are focused on assessing the adsorption properties of carbon materials for CO2 capture and wastewater treatment. The importance of the chemical surface properties of carbon materials has also been highlighted in some of the articles, in which the role of surface functional groups (mainly N, B, and P functional groups) in attaining materials with tailored properties has been discussed. Regarding the bulk properties, articles dealing with the thermal, mechanical, magnetic, optical, and electronic properties have been included, with a few articles covering each of these properties of carbon materials.
The broad range of applications of carbon materials has been also captured in many publications, among which those addressing the use of carbon materials in energy generation and storage, catalytic, electrochemical, biological and medical applications, and wastewater treatment and environmental remediation have received great attention, both in terms of the number of publications and citations. Also, very interesting publications addressing the use of carbon materials in other applications, including sensing, textile, soil remediation, soil nutrition and fertility, photovoltaic and photoelectrochemical, etc., have been published in the Frontiers in Carbon-based materials section during its 10 years of life. These publications are a good indicator of the versatility of carbon materials and reflect their relevance in the material science scenario by providing the state-of-the art of these fascinating materials.
To summarize, carbon has been in the limelight for several decades (take the seminal review paper by Walker Jr. which dates back to 1972), and it has somehow managed to continue surprising the scientific community thanks to its adaptability and flexibility, which arises from its peculiar atomic structure and position in the periodic table, as discussed above. No matter the current challenge which carbon materials have had to face over the past 60 or 70 years, they have always risen to meet demands in environmental remediation, biomedicine, sustainable generation of fuels, clean energy generation and storage, or photocatalysis, and have, in several cases, even risen as the most promising and best performing option. We cannot know what will the “next big thing” be in Science or Technology, but given their current performance and applications record, it may be safe to assume that carbon materials will answer the call when the time comes once again.
Author contributions
JF-L: Writing–original draft and editing. MN-G: Writing–original draft and editing. ÁB-M: Writing–original draft and editing. EM: Conceptualization, Supervision, Writing–review and editing. DC-A: Conceptualization, Supervision, Writing–review and editing.
Funding
The author(s) declare financial support was received for the research, authorship, and/or publication of this article. The authors would like to thank PID2022-137566OB-I00 and PID2022-137566OB-I00 projects funded by MCIN/AEI/10.13039/501100011033 and by “ERDF A way of making Europe”, by the “European Union”. The authors thank the MFA/2022/001 project for financing this work, which is part of the Advanced Materials programme and was supported by MCIN with funding from European Union NextGenerationEU (PRTR-C17.I1) and by Generalitat Valenciana.
Conflict of interest
The authors declare that the research was conducted in the absence of any commercial or financial relationships that could be construed as a potential conflict of interest.
The author(s) declared that they were an editorial board member of Frontiers, at the time of submission. This had no impact on the peer review process and the final decision.
Publisher’s note
All claims expressed in this article are solely those of the authors and do not necessarily represent those of their affiliated organizations, or those of the publisher, the editors and the reviewers. Any product that may be evaluated in this article, or claim that may be made by its manufacturer, is not guaranteed or endorsed by the publisher.
References
Al-zharani, M., Alyami, N. M., Qurtam, A. A., Aljarba, N. H., Alkahtani, S., Mubarak, M., et al. (2023). Use of multi-walled carbon nanotubes (MWCNTs) stabilized in Arabic gum colloidal solution to induce genotoxicity and apoptosis of human breast and lung cancer cell lines. Front. Mat. 10, 1229637. doi:10.3389/fmats.2023.1229637
Azam, N., Najabat Ali, M., and Javaid Khan, T. (2021). Carbon quantum dots for biomedical applications: review and analysis. Front. Mat. 8, 700403. doi:10.3389/fmats.2021.700403
Baladin, A. A. (2011). Thermal properties of graphene and nanostructured carbon materials. Nat. Mat. 10, 569–581. doi:10.1038/nmat3064
Barhoum, A., Shalan, A. E., El-Hout, S. I., Ali, G. A. M., Abdelbasir, S. M., Abu-Serea, E. S., et al. (2019). “A broad family of carbon nanomaterials: classification, properties, synthesis, and emerging applications,” in Handbook of nanofibers (Berlin, Germany: Springer International Publishing), 1–40. doi:10.1007/978-3-319-42789-8_59-1
Basit, M., Abbas, M., Ahmad, N., Javed, S., and Shah, N. A. (2022). Synthesis of ZnO/CNT nanocomposites for ultraviolet sensors. Front. Mat. 9, 835521. doi:10.3389/fmats.2022.835521
Beralus, J.-M., Ruiz-Rosas, R., Cazorla-Amorós, D., and Morallón, E. (2014). Electroadsorption of arsenic from natural water in granular activated carbon. Front. Mat. 1, 28. doi:10.3389/fmats.2014.00028
Berenguer, R., and Morallón, E. (2019). Oxidation of different microporous carbons by chemical and electrochemical methods. Front. Mat. 6, 130. doi:10.3389/fmats.2019.00130
Bianco, A., Cheng, H., Enoki, T., Gogotsi, Y., Hurt, R., Koratkar, N., et al. (2013). All in the graphene family – a recommended nomenclature for two-dimensional carbon materials. Carbon 65, 1–6. doi:10.1016/j.carbon.2013.08.038
Boonyoung, P., Kasukabe, T., Hoshikawa, Y., Berenguer-Murci, Á., Cazorla-Amorós, D., Boekfa, B., et al. (2019). A simple “nano-templating” method using zeolite Y toward the formation of carbon schwarzites. Front. Mat. 6, 104. doi:10.3389/fmats.2019.00104
Brosler, P., Girão, A. V., Silva, R. F., Tedim, J., and Oliveira, F. J. (2023). In-house vs. commercial boron-doped diamond electrodes for electrochemical degradation of water pollutants: a critical review, Front. Mat. 10, 1020649. doi:10.3389/fmats.2023.1020649
Bumajdad, A., Khan, M. J. H., and Lukaszewicz, J. P. (2023). Nitrogen-enriched activated carbon derived from plant biomasses: a review on reaction mechanism and applications in wastewater treatment. Front. Mat. 10, 1218028. doi:10.3389/fmats.2023.1218028
Caballero-Briones, F., Kaftelen-Odabaşı, H., Gnanaseelan, N., Ruiz-Perez, F., Tolentino-Hernandez, R. V., Kamaraj, S. K., et al. (2023). International research in graphene-oxide based materials for net-zero energy, military and aeronautic applications catalysed by Tamaulipas, Mexico: a mini review. Front. Mat. 10, 1192724. doi:10.3389/fmats.2023.1192724
Calvo-Muñoz, E. M., García-Mateos, F. J., Rosas, J. M., Rodríguez-Mirasol, J., and Cordero, T. (2016). Biomass waste carbon materials as adsorbents for CO2 capture under post-combustion conditions. Front. Mat. 3, 23. doi:10.3389/fmats.2016.00023
Carmona, R. J., Velasco, L. F., Laurenti, E., Maurino, V., and Ania, C. O. (2016). Carbon materials as additives to WO3 for an enhanced conversion of simulated solar light. Front. Mat. 3, 9. doi:10.3389/fmats.2016.00009
Castro-Muñiz, A., Hoshikawa, Y., Komiyama, H., Nakayama, W., Itoh, T., and Kyotani, T. (2016). Improving the direct electron transfer in monolithic bioelectrodes prepared by immobilization of FDH enzyme on carbon-coated anodic aluminum oxide films. Front. Mat. 3, 7. doi:10.3389/fmats.2016.00007
Cazorla-Amorós, D. (2014). Grand challenges in carbon-based materials research. Front. Mat. 1, 6. doi:10.3389/fmats.2014.00006
Cesano, F., Cravanzola, S., Brunella, V., Damin, A., and Scarano, D. (2019). From polymer to magnetic porous carbon spheres: combined microscopy, spectroscopy, and porosity studies. Front. Mat. 6, 84. doi:10.3389/fmats.2019.00084
Cesano, F., Uddin, M. J., Lozano, K., Zanetti, M., and Scarano, D. (2020a). All-carbon Conductors for electronic and electrical wiring applications. Front. Mat. 7, 219. doi:10.3389/fmats.2020.00219
Cesano, F., Uddin, M. J., Mao, Y., and Huda, M. N. (2020b). Editorial: carbon- and inorganic-based nanostructures for energy applications. Front. Mat. 7, 609576. doi:10.3389/fmats.2020.609576
Chao-Mujica, F. J., Garcia-Hernández, L., Camacho-López, S., Camacho-López, M., Camacho-López, M. A., Reyes Contreras, D., et al. (2021). Carbon quantum dots by submerged arc discharge in water: synthesis, characterization, and mechanism of formation. J. Appl. Phys. 129, 163301. doi:10.1063/5.0040322
Chen, G., and Song, Y. (2016). Field emission from lateral multiwalled carbon nanotube yarn emitters. Front. Mat. 3, 48. doi:10.3389/fmats.2016.00048
Cui, L., Ren, X., Wang, J., and Sun, M. (2020). Synthesis of homogeneous carbon quantum dots by ultrafast dual-beam pulsed laser ablation for bioimaging. Mat. Today Nano 12, 100091. doi:10.1016/j.mtnano.2020.100091
Dang, H., Huang, L.-K., Zhang, Y., Wang, C.-F., and Chen, S. (2016). Large-scale ultrasonic fabrication of white fluorescent carbon dots. Ind. Eng. Chem. Res. 55 (18), 5335–5341. doi:10.1021/acs.iecr.6b00894
Díaz-Delgado, R., and Doherty, A. P. (2016). Carbons, ionic liquids, and quinones for electrochemical capacitors. Front. Mat. 3, 18. doi:10.3389/fmats.2016.00018
Dinu, R., Lafont, U., Damiano, O., and Mija, A. (2023). Sustainable and recyclable thermosets with performances for high technology sectors. An environmental friendly alternative to toxic derivatives. Front. Mat. 10, 1242507. doi:10.3389/fmats.2023.1242507
Elumalai, S., Su, C.-Y., and Yoshimura, M. (2019). Scalable one-pot synthesis of nitrogen and boron Co-doped few layered graphene by submerged liquid plasma exfoliation. Front. Mat. 6, 216. doi:10.3389/fmats.2019.00216
Fan, X. (2015). Graphene: a promising two-dimensional support for heterogeneous catalysts. Front. Mater 1, 39. doi:10.3389/fmats.2014.00039
Figueiredo, J. L., Pereira, M., Freitas, M., and Órfão, J. J. M. (1999). Modification of the surface chemistry of activated carbons. Carbon 37 (9), 1379–1389. doi:10.1016/S0008-6223(98)00333-9
García-Mateos, F. J., Ruiz-Rosas, R., Rosas, J. M., Rodríguez-Mirasol, J., and Cordero, T. (2019). Controlling the composition, morphology, porosity, and surface chemistry of lignin-based electrospun carbon materials. Front. Mat. 6, 114. doi:10.3389/fmats.2019.00114
Geng, J., Motta, M., Engels, V., Luo, J., and Johnson, B. F. G. (2016). Temperature threshold and water role in CVD growth of single-walled carbon nanotubes. Front. Mat. 3, 4. doi:10.3389/fmats.2016.00004
Geng, Y., Ren, Q., Yang, Z., He, D., Wu, Z., Cai, J., et al. (2023). Preparation of SiO2 coated carbon fibers and its interfacial properties with cement paste matrix. Front. Mat. 10, 1128054. doi:10.3389/fmats.2023.1128054
Gouda, M. H., Elnouby, M., Aziz, A. N., Youssef, M. E., Santos, D. M. F., and Elessawy, N. A. (2020). Green and low-cost membrane electrode assembly for proton exchange membrane fuel cells: effect of double-layer electrodes and gas diffusion layer. Front. Mat. 6, 337. doi:10.3389/fmats.2019.00337
Han, L., Chen, X., Zeng, S., Liu, J., Yang, Z., Wang, Z., et al. (2021). B, N dual doped coral-like carbon framework with superior pseudocapacitance and surface wettability. Front. Mat. 8, 705930. doi:10.3389/fmats.2021.705930
Ilnicka, A., and Lukaszewicz, J. P. (2015). Discussion remarks on the role of wood and chitin constituents during carbonization. Front. Mat. 2, 20. doi:10.3389/fmats.2015.00020
Inagaki, M., Kang, F., Toyoda, M., and Konno, H. (2014). Advanced materials science and engineering of carbon. Amsterdam, Netherlands: Elsevier Science. doi:10.1016/C2012-0-03601-0
Ishii, T., Horiuchi, A., and Ozaki, J. (2019). An ion-sensitive field effect transistor using metal-coordinated zeolite-templated carbons as a three-dimensional graphene nanoribbon network. Front. Mat. 6, 129. doi:10.3389/fmats.2019.00129
Jangir, H., Bhardwaj, A., Ramkumar, J., Sarkar, S., and Das, M. (2019). “Induced electron transfer” in silk cocoon derived N-doped reduced graphene oxide-Mo-Li-S electrode. Front. Mat. 6, 217. doi:10.3389/fmats.2019.00217
Kallumottakkal, M., Hussein, M. I., and Iqbal, M. Z. (2021). Recent progress of 2D nanomaterials for application on microwave absorption: a comprehensive study. Front. Mat. 8, 633079. doi:10.3389/fmats.2021.633079
Karamanova, B., Shipochka, M., Georgiev, M., Stankulov, T., Stoyanova, A., and Stoyanova, R. (2021). Biomass-derived carbonaceous materials to achieve high-energy-density supercapacitors. Front. Mat. 8, 654841. doi:10.3389/fmats.2021.654841
Karami, S., Papari, S., and Berruti, F. (2022). Conversion of waste corn biomass to activated bio-char for applications in wastewater treatment. Front. Mat. 9, 839421. doi:10.3389/fmats.2022.839421
Kato, Y., Sekiguchi, A., Kobashi, K., Sundaram, R., Yamada, T., and Hata, K. (2020). Mechanically robust free-standing single-walled carbon nanotube thin films with uniform mesh-structure by blade coating. Front. Mat. 7, 562455. doi:10.3389/fmats.2020.562455
Keyte, J., Pancholi, K., and Njuguna, J. (2019). Recent developments in graphene oxide/epoxy carbon fiber-reinforced composites. Front. Mat. 6, 224. doi:10.3389/fmats.2019.00224
Khan, H. O. S., Zhu, J., Jalil, A., Sarwar, R. T., Hameed, F., and Xu, F. (2020). Optimal synthesis and evaluation of tri-amine modified ordered mesoporous carbon (TriFeOMC) and its application for the adsorption of arsenic and lead from aqueous solution. Front. Mat. 7, 112. doi:10.3389/fmats.2020.00112
Khan, S. A., Habib, S., Khan, M. Y. A., Chauhdary, S. T., Ali, A., Kamel, S., et al. (2023). Dichlorodifluoromethane-carbon dioxide: a dielectric mixture as a sustainable alternative to SF6 in high voltage applications. Front. Mat. 10, 1129739. doi:10.3389/fmats.2023.1129739
Kordas, K., and Pitkänen, O. (2019). Piezoresistive carbon foams in sensing applications. Front. Mat. 6, 93. doi:10.3389/fmats.2019.00093
Kotal, M., Kim, J., Oh, J., and Oh, I.-K. (2016). Recent progress in multifunctional graphene aerogels. Front. Mat. 3, 29. doi:10.3389/fmats.2016.00029
Kouloumpis, A., Spyrou, K., Dimos, K., Georgakilas, V., Rudolf, P., and Gournis, D. (2015). A bottom-up approach for the synthesis of highly ordered fullerene-intercalated graphene hybrids. Front. Mat. 2, 10. doi:10.3389/fmats.2015.00010
Kumunda, C., Adekunle, A. S., Mamba, B. B., Hlongwa, N. W., and Nkambule, T. T. I. (2021). Electrochemical detection of environmental pollutants based on graphene derivatives: a review. Front. Mat. 7, 616787. doi:10.3389/fmats.2020.616787
Lenz, M., Zabel, J., and Franzreb, M. (2020). New approach for investigating diffusion kinetics within capacitive deionization electrodes using electrochemical impedance spectroscopy. Front. Mat. 7, 229. doi:10.3389/fmats.2020.00229
Li, J., Zhou, X., Lu, K., Ma, C., Li, L., Wang, H., et al. (2021). N-doped carbon boosted the formation of few-layered MoS2 for high- performance lithium and sodium storage. Front. Mat. 8, 739859. doi:10.3389/fmats.2021.739859
Liu, T. (2023). Properties of graphene composite fiber seamless knitted fabric and its application in boxing training. Front. Mat. 10, 1098652. doi:10.3389/fmats.2023.1098652
Liu, Y., Ren, W., An, M., Dong, L., Gao, L., Shai, X., et al. (2022). A qualitative study of the disorder effect on the phonon transport in a two-dimensional graphene/h-BN heterostructure. Front. Mat. 9, 913764. doi:10.3389/fmats.2022.913764
Maboya, W. K., Maubane-Nkadimeng, M. S., Jijana, A. N., and Mmako, H. K. (2022). Nitrogen inclusion in carbon nanotubes initiated by boron doping and chlorination: their use as electrocatalysts for oxygen reduction reaction. Front. Mat. 9, 886471. doi:10.3389/fmats.2022.886471
Macovez, R. (2018). Physical properties of organic fullerene cocrystals. Front. Mat. 4, 46. doi:10.3389/fmats.2017.00046
Magesh, V., Sundramoorthy, A. K., and Ganapathy, D. (2022). Recent advances on synthesis and potential applications of carbon quantum dots. Front. Mat. 9, 906838. doi:10.3389/fmats.2022.906838
Matsuo, Y., and Araki, M. (2017). Selective detection of acetone vapor using hydrophobized pillared carbon thin films. Front. Mat. 4, 17. doi:10.3389/fmats.2017.00017
Matsuo, Y., Hayashida, A., and Konishi, K. (2015). Porous properties of pillared carbons prepared from the thermal reduction of graphite oxide repeatedly silylated with methyltrichlorosilanes. Front. Mat. 2. doi:10.3389/fmats.2015.00021
Moulefera, I., García-Mateos, F. J., Benyoucef, A., Rosas, J. M., Rodríguez-Mirasol, J., and Cordero, T. (2020). Effect of Co-solution of carbon precursor and activating agent on the textural properties of highly porous activated carbon obtained by chemical activation of lignin with H3PO4. Front. Mat. 7, 153. doi:10.3389/fmats.2020.00153
Mukherjee, A., Patra, B. R., Podder, J., and Dalai, A. K. (2022). Synthesis of biochar from lignocellulosic biomass for diverse industrial applications and energy harvesting: effects of pyrolysis conditions on the physicochemical properties of biochar. Front. Mat. 9, 870184. doi:10.3389/fmats.2022.870184
Navlani-García, M., Mori, K., Salinas-Torres, D., Kuwahara, Y., and Yamashita, H. (2019). New approaches toward the hydrogen production from formic acid dehydrogenation over Pd-based heterogeneous catalysts. Front. Mat. 6, 44. doi:10.3389/fmats.2019.00044
Ong, W.-J. (2017). 2D/2D graphitic carbon nitride (g-C3N4) heterojunction nanocomposites for photocatalysis: why does face-to-face interface matter? Front. Mat. 4, 11. doi:10.3389/fmats.2017.00011
Orfanakis, G., Patila, M., Catzikonstantinou, A. V., Lyra, K.-M., Kouloumpis, A., Spyrou, K., et al. (2018). Hybrid nanomaterials of magnetic iron nanoparticles and graphene oxide as matrices for the immobilization of β-glucosidase: synthesis, characterization, and biocatalytic properties. Front. Mat. 5, 25. doi:10.3389/fmats.2018.00025
Otero, T. F., Martinez, J. G., and Asaka, K. (2016). Faradaic and capacitive components of the CNT electrochemical responses. Front. Mat. 3, 3. doi:10.3389/fmats.2016.00003
Parthasarathy, P., Mackey, H. R., Mariyam, S., Zuhara, S., Al-Ansari, T., and McKay, G. (2021). Char products from bamboo waste pyrolysis and acid activation. Front. Mat. 7, 624791. doi:10.3389/fmats.2020.624791
Piñeiro-Prado, I., Salinas-Torres, D., Ruiz-Rosas, R., Morallón, E., and Cazorla-Amorós, D. (2016). Design of activated carbon/activated carbon asymmetric capacitors. Front. Mat. 3, 16. doi:10.3389/fmats.2016.00016
Poirot, N., Rajalingam, V., Murgu, R. N., Omnée, R., and Raymundo-Piñero, E. (2022). Nanotexturing TiO2 over carbon nanotubes for high-energy and high-power density pseudocapacitors in organic electrolytes. Front. Mat. 9, 1011782. doi:10.3389/fmats.2022.1011782
Quílez-Bermejo, J., Pérez-Rodríguez, S., Celzard, A., and Fierro, V. (2022). Progress in the use of biosourced phenolic molecules for electrode manufacturing. Front. Mat. 9, 810575. doi:10.3389/fmats.2022.810575
Quqa, S., Shu, Y., Li, S., and Loh, K. J. (2022). Pressure mapping using nanocomposite-enhanced foam and machine learning. Front. Mat. 9, 862796. doi:10.3389/fmats.2022.862796
Radovic, L. R. (2005). The mechanism of CO2 chemisorption on zigzag carbon active sites: a computational chemistry study. Carbon 43 (5), 907–915. doi:10.1016/j.carbon.2004.11.011
Rosas, J. M., Berenguer, R., Valero-Romero, M. J., Rodríguez-Mirasol, J., and Cordero, T. (2014). Preparation of different carbon materials by thermochemical conversion of lignin. Front. Mat. 1, 29. doi:10.3389/fmats.2014.00029
Ruiz-Rosas, R., García-Mateos, F. J., Gutiérrez, M. C., Rodríguez-Mirasol, J., and Cordero, T. (2019). About the role of porosity and surface chemistry of phosphorus-containing activated carbons in the removal of micropollutants. Front. Mat. 6, 134. doi:10.3389/fmats.2019.00134
Salavagione, H. J., Gómez-Fatou, M. A., Shuttleworth, P. S., and Ellis, G. J. (2018). New perspectives on graphene/polymer fibers and fabrics for smart textiles: the relevance of the polymer/graphene interphase. Front. Mat. 5, 18. doi:10.3389/fmats.2018.00018
Salinas-Torres, D., Ruiz-Rosas, R., Morallón, E., and Cazorla-Amorós, D. (2019). Strategies to enhance the performance of electrochemical capacitors based on carbon materials. Front. Mat. 6, 115. doi:10.3389/fmats.2019.00115
Sanchís, C., Ruiz-Rosas, R., Berenguer-Murcia, Á., Morallón, E., and Cazorla-Amorós, D. (2015). Switchable surfactant-assisted carbon nanotube coatings: innovation through pH shift. Front. Mat. 2, 1. doi:10.3389/fmats.2015.00001
Santiago-Ramírez, C. R., Nair, P. R., Vela-Monroy, C. A., Aba-Guevara, C. G., Ramos-Delgado, N. A., and Gracia-Pinilla, M. A. (2022). A minireview on the use of g-C3N4–chitosan biocomposite for potential applications. Front. Mat. 9, 856350. doi:10.3389/fmats.2022.856350
Sapalidis, A., Sideratou, Z., Panagiotaki, K. N., Sakellis, E., Kouvelos, E. P., Papageorgiou, S., et al. (2018). Fabrication of antibacterial poly(vinyl alcohol) nanocomposite films containing dendritic polymer functionalized multi-walled carbon nanotubes. Front. Mat. 5, 11. doi:10.3389/fmats.2018.00011
Schutjajew, K., Yan, R., Antonietti, M., Roth, C., and Oschatz, M. (2019). Effects of carbon pore size on the contribution of ionic liquid electrolyte phase transitions to energy storage in supercapacitors. Front. Mat. 6, 65. doi:10.3389/fmats.2019.00065
Tsirka, K., Katsiki, A., Chalmpes, N., Gournis, D., and Paipetis, A. S. (2018). Mapping of graphene oxide and single layer graphene flakes—defects annealing and healing. Front. Mat. 5, 37. doi:10.3389/fmats.2018.00037
Tzitzios, V., Dimos, K., Alhassan, S. M., Mishra, R., Kouloumpis, A., Gournis, D., et al. (2018). Facile MoS2 growth on reduced graphene-oxide via liquid phase method. Front. Mat. 5, 29. doi:10.3389/fmats.2018.00029
Valero-Romero, M. J., Rodríguez-Cano, M. Á., Palomo, J., Rodríguez-Mirasol, J., and Cordero, T. (2021). Carbon-based materials as catalyst supports for fischer–tropsch synthesis: a review. Front. Mat. 7, 617432. doi:10.3389/fmats.2020.617432
Varshney, V., Lee, J., Brown, J. S., Farmer, B. L., Voevodin, A. A., and Roy, A. K. (2018). Effect of length, diameter, chirality, deformation, and strain on contact thermal conductance between single-wall carbon nanotubes. Front. Mat. 5, 17. doi:10.3389/fmats.2018.00017
Vrettos, K., Karouta, N., Loginos, P., Donthula, S., Gournis, D., and Georgakilas, V. (2018). The role of diamines in the formation of graphene aerogels. Front. Mat. 5, 20. doi:10.3389/fmats.2018.00020
Walker, P. L. (1972). Carbon: an old but new material. Carbon 10 (4), 369–370. doi:10.1016/0008-6223(72)90052-8
Walker, P. L. (1990). Carbon: an old but new material revisited. Carbon 28 (2–3), 261–279. doi:10.1016/0008-6223(90)90001-F
Wei, J., Geng, S., Kumar, M., Pitkänen, O., Hietala, M., and Oksman, K. (2019). Investigation of structure and chemical composition of carbon nanofibers developed from renewable precursor. Front. Mat. 6, 334. doi:10.3389/fmats.2019.00334
Worajittiphon, P., Large, M. J., King, A. A. K., Jurewicz, I., and Dalton, A. B. (2015). Stretchable conductive networks of carbon nanotubes using plasticized colloidal templates. Front. Mat. 2, 15. doi:10.3389/fmats.2015.00015
Wu, D., Xie, X., Zhang, Y., Zhang, D., Du, W., Zhang, X., et al. (2020). MnO2/Carbon composites for supercapacitor: synthesis and electrochemical performance. Front. Mat. 7, 2. doi:10.3389/fmats.2020.00002
Xu, Y., Lu, F., Liu, K., and Ma, C. (2020). Direct graphene synthesis on lithium niobate substrate by carbon ion implantation. Front. Mat. 7, 572280. doi:10.3389/fmats.2020.572280
Yang, M., and Zhang, M. (2019). Biodegradation of carbon nanotubes by macrophages. Front. Mat. 6, 225. doi:10.3389/fmats.2019.00225
Yang, S., Sun, J., Li, X., Zhou, W., Wang, Z., He, P., et al. (2014). Large-scale fabrication of heavy doped carbon quantum dots with tunable-photoluminescence and sensitive fluorescence detection. J. Mat. Chem. A (2), 8660–8667. doi:10.1039/C4TA00860J
Yasri, N. G., Sundramoorthy, A. K., Chang, W.-J., and Gunasekaran, S. (2014). Highly selective mercury detection at partially oxidized graphene/poly(3,4-ethylenedioxythiophene):poly(styrenesulfonate) nanocomposite film-modified electrode. Front. Mat. 1, 33. doi:10.3389/fmats.2014.00033
Yasuda, E., Inagaki, M., Kaneko, K., Endo, M., Oya, A., and Tanabe, Y. (2003). Carbon alloys: novel concepts to develop carbon science and technology. Oxford: Elsevier Science Ltd. doi:10.1016/B978-0-08-044163-4.X5000-1
Yu, C., Hou, H., Liu, X., Han, L., Yao, Y., Dai, Z., et al. (2018). The recovery of the waste cigarette butts for N-doped carbon anode in lithium ion battery. Front. Mat. 5, 63. doi:10.3389/fmats.2018.00063
Zhang, Z., Wang, Y., Wang, H., Xue, X., and Lin, Q. (2021). Metal-organic frameworks promoted hydrogen storage properties of magnesium hydride for in-situ resource utilization (ISRU) on mars. Front. Mat. 8, 766288. doi:10.3389/fmats.2021.766288
ZhangCalderon, Q. B., Ebbing C, R., Elston L, J., Byrd L, W., and Tsao, B.-H. (2020). Thermal properties enhancement of vertically aligned carbon nanotubes-based metal nanocomposites as thermal interface materials. Front. Mat. 7, 572956. doi:10.3389/fmats.2020.572956
Zheng, S. Q., Lim, S. S., Foo, C. Y., Haw, C. Y., Chiu, W. S., Chia, C. H., et al. (2021). Recent progress on the applications of carbonaceous and metal-organic framework nanomaterials for supercapacitors. Front. Mat. 8, 777149. doi:10.3389/fmats.2021.777149
Keywords: carbon nanotubes, graphene, activated carbon, heteroatoms, adsorption, catalysis
Citation: Flores-Lasluisa JX, Navlani-García M, Berenguer-Murcia Á, Morallón E and Cazorla-Amorós D (2024) 10 years of frontiers in carbon-based materials: carbon, the “newest and oldest” material. The story so far. Front. Mater. 11:1381363. doi: 10.3389/fmats.2024.1381363
Received: 03 February 2024; Accepted: 25 March 2024;
Published: 09 April 2024.
Edited by:
Nicola Maria Pugno, University of Trento, ItalyReviewed by:
Jingzhe Li, Lam Research, United StatesJian Wang, University of Science and Technology Liaoning, China
Copyright © 2024 Flores-Lasluisa, Navlani-García, Berenguer-Murcia, Morallón and Cazorla-Amorós. This is an open-access article distributed under the terms of the Creative Commons Attribution License (CC BY). The use, distribution or reproduction in other forums is permitted, provided the original author(s) and the copyright owner(s) are credited and that the original publication in this journal is cited, in accordance with accepted academic practice. No use, distribution or reproduction is permitted which does not comply with these terms.
*Correspondence: Diego Cazorla-Amorós, Y2F6b3JsYUB1YS5lcw==