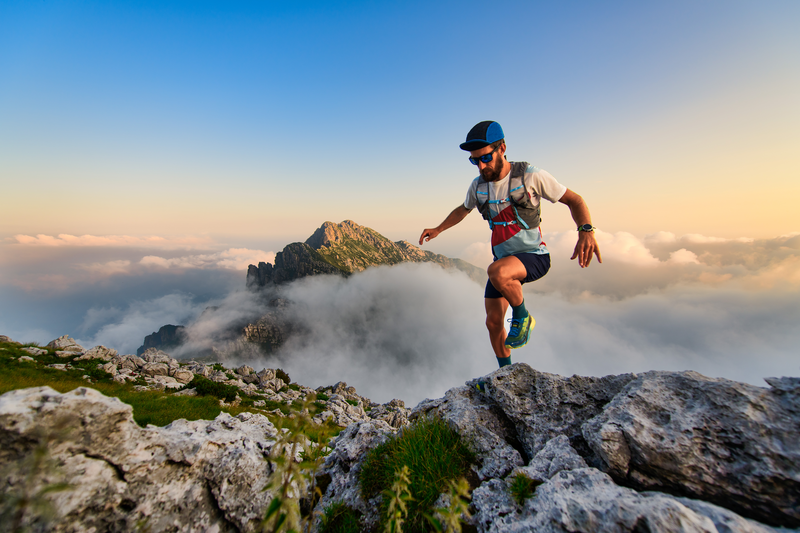
94% of researchers rate our articles as excellent or good
Learn more about the work of our research integrity team to safeguard the quality of each article we publish.
Find out more
ORIGINAL RESEARCH article
Front. Mater. , 31 July 2024
Sec. Biomaterials and Bio-Inspired Materials
Volume 11 - 2024 | https://doi.org/10.3389/fmats.2024.1354560
In this study, Co3O4 nanoparticles were used as nanocatalyst for two different series of nitrogen-containing heterocyclic compounds, including pyrrole (Pyo) derivatives and pyrano [2, 3-c]pyrazole (Pya[2, 3-c]Pyz) derivatives. In the synthesis of derivatives, using 15 mol% and 10 mol% of the catalyst for Pyo derivatives and Pya[2, 3-c]Pyz derivatives, respectively, an efficiency between 83% and 96%, were observed. In addition, novel derivatives of Pyo and Pya[2,3-c]Pyz were synthesized and their structures were confirmed. In general, the advantages of using cobalt nanoparticles compared to previous reports include the synthesis of new derivatives, lower temperature used in the synthesis of derivatives, shorter synthesis time and high efficiency. The biological properties of the synthesized products, such as antibacterial, antifungal, and antioxidant properties, were tested and investigated. In antibacterial and antifungal tests, IZD, MIC, MBC, and MFC were measured and reported. In antioxidant activity, IC50 was calculated and reported. High reusability, green and environmentally friendly, synthesis of new derivatives and synthesis of products with higher efficiency and shorter time were the important benefits of using cobalt nanoparticles as a catalyst. In antioxidant tests, the IC50 for synthesized Pyo derivatives and Pya[2, 3-c] Pyz derivatives were between 12.2 and 13.71 μg/mL, and 16.18–17.75 μg/mL, respectively. In antimicrobial testes, the MIC for synthesized Pyo derivatives and Pya[2, 3-c]Pyz derivatives were between 2 and 4,096 μg/mL, and 2–2048 μg/mL, respectively. The results showed that the antioxidant property of Pyo derivatives were more than Pya[2, 3-c] Pyz derivatives, but the antimicrobial effect of Pya[2,3-c] Pyz derivatives were more than Pyo derivatives. The antioxidant results proved that the activity of Pyo derivatives and Pya[2, 3-c] Pyz derivatives does not depend on the substitutions of the derivatives and is close to each other. Therefore, based on this, a proposed mechanism for stability of DPPH by Pyo derivatives and Pya[2, 3-c] Pyz derivatives were suggested. Finally, based on the more stable resonance structures of Pyo derivatives, compared to Pya[2, 3-c] Pyz derivatives, its high antioxidant property was justified. Pya[2, 3-c] Pyz derivatives has two heterocyclic rings connected together pyrano and pyrazole, but Pyo derivatives has only one heterocyclic ring (pyrrole). So high antimicrobial property of Pya[2, 3-c] Pyz derivatives compared to Pyo derivatives can be attributed to having two bioactive heterocyclic rings.
Pyrrole (Pyo) is a five-member heterocyclic compound with one nitrogen in its structure. Biological activity such as anticancer, antitumor, anti-inflammatory, antiviral, antimicrobial, antituberculosis, and antioxidant have been reported from compounds containing Pyo derivatives (Petri et al., 2020; Zhou et al., 2020; Boichuk et al., 2021; Rawat et al., 2021; Reddy et al., 2021; Jeelan Basha et al., 2022). Pyo derivatives were found abundantly in nature. For example, Pyo derivatives were found in marine-derived fungi, such as Penicillium citrinum and Aspergillus sclerotiorum (Seipp et al., 2021). The use of Pyo derivatives as solvents for resin, polymerization processes, and erosion inhibitors is prevalent in industries (Bhardwaj et al., 2015).
Another five-member heterocyclic compound that has two nitrogen atoms in positions one and two in its structure is pyrazole. Pyrazole derivatives are present in the construction of commercial drugs such as Celecoxib and Fomepizole (Alam et al., 2015). Other biological properties reported for this heterocyclic compound include anticancer activity, antiviral, antimicrobial activity, anticonvulsant and antidepressant activity, anti-inflammatory activity, and antimicrobial activity (Faria et al., 2017; Faisal et al., 2019). Many multi-ring structures containing pyrazole derivatives with five-member heterocyclic compounds, such as pyrazolopyridine derivatives, pyrazolopyrimidine derivatives, pyranopyrazole derivatives, etc., have been reported with biological properties (Faisal et al., 2019; Rao and Chanda, 2020; Biswas and Das, 2022).
Pyrano [2,3-c]pyrazole (Pya[2,3-c]Pyz) derivatives, which are formed by connecting two heterocyclic compounds of pyran and pyrazole, is a heterocyclic compound with outstanding biological properties that retain the properties of both heterocyclic compounds of pyrazole and pyran. Several methods have been reported for synthesizing Pya[2,3-c]Pyz derivatives, including, the use of various metals and metal oxides (Muthuraja et al., 2017; Veeramani et al., 2018; Vairaperumal et al., 2019). The previous literature demonstrated that this heterocyclic compound has various biological properties, including anticancer, anti-malarial agents, anticonvulsant, analgesic, antimicrobial, and anti-inflammatory (Biswas and Das, 2022).
Pyrans, which play an essential role in the properties of Pya[2,3-c]Pyz derivatives, are 6-membered heterocyclic compounds containing one oxygen. The most important natural compound containing pyran is vitamin E, essential for humans (Garazd and Garazd, 2016).
Several methods have been reported for Pya[2,3-c]Pyz derivatives and Pyo derivatives, but multicomponent reactions are the most important method and scientists have focused on it. Multicomponent reactions that are one-pot reactions align with the rules of green chemistry (Baral et al., 2020; Sikandar and Zahoor, 2021). In addition to the green nature of multi-component reactions, it is possible to mention the convenience of the synthesis steps of the final product, high efficiency, and cost-effectiveness in terms of economy, etc (Javahershenas and Nikzat, 2023). In multicomponent reactions, the suitable catalyst plays a key role (Costanzo et al., 2018; Rahimi et al., 2022).
Using metal oxide nanoparticles is a suitable option as a catalyst in these reactions (Damera et al., 2023; Soltani et al., 2023). Cobalt oxide nanoparticles are a good choice due to their high catalytic properties. This oxidized metal catalyst has been used in the synthesis of heterocyclic compounds and organic reactions such as, arylidene barbituric and Meldrum’s acid derivatives, benzochromenes derivatives, and Ugi adduct from aryl alcohols (Chacko and Shivashankar, 2017; Shahbazi et al., 2019; Yahyazadehfar et al., 2019; Kafi-Ahmadi et al., 2021).
Considering the importance of using green chemistry for the synthesis of Pyo derivatives and Pya[2,3-c]Pyz derivatives, with biological properties, novel Pyo derivatives and Pya[2,3-c]Pyz derivatives were synthesized using cobalt oxide nanoparticles as a green, efficient, and recyclable catalyst during a multicomponent reaction. In studying biological activities, in vitro antioxidant and in vitro antimicrobial properties of synthesized derivatives were evaluated.
The required solvents, materials, and antibiotics were prepared from Merck and Sigma. The microorganisms studied were prepared from ATCC (American Type Culture Collection).
Fourier transform infra-red spectra of Co3O4 nanoparticles and synthesized derivatives were recorded using Nicolet IS10. The Shimadzu XRD-7000 was used for X-ray diffraction of Co3O4 nanoparticles. The scanning electron microscope image of Co3O4 nanoparticles using Gemini SEM 500 was prepared. The carbon and hydrogen nuclear magnetic resonance of synthesized derivatives using Bruker FT-NMR ultra shield- 400 spectrometer were obtained. The melting points of a synthesized compound using Electrothermal IA9200 were obtained. Element analysis of synthesized derivatives using Thermo FlashSmart CHNS/O elemental analyzer was done. The Shimadzu UV-3600 Plus was used in biological evaluations.
To synthesize Co3O4 nanoparticles, in a flask containing 100 mL of double distilled water, 1 g pluronic F-127 was added and stirred (1,000 rpm) at room temperature for 30 min. Then add 100 mL of cobalt nitrate solution (0.1 M) and stir) at room temperature for 30 min. Drop by drop, add 100 mL of NaOH solution 2 M and stir at 100°C for 72 h. In the next step, methanol (100 mL) was added to the mixture and stirred at room temperature for 4 h. The mixture was kept at room temperature for 12 h. The sediments were separated using Whatman filter paper and washed with double distilled water until they reached pH 7. Sediments obtained dried in an oven at 100°C for 60 min. Finally, it was placed in a muffle furnace at a temperature of 500°C for 4 h (Sadasivan et al., 2013; Uddin and Baig, 2019).
1 mmol cyclohexanamine or phenylmethanamine, 1 mmol dimethyl but-2-ynedioate or diethyl but-2-ynedioate were added to the 2 mL water/ethanol (1:1) and were stirred at room temperature for 15 min. To the mixture, 10 mol percent of Co3O4 nanoparticles, 1 mmol of formaldehyde and 1 mmol of aromatic amine nanoparticles were added and stirred at room temperature. After the completion of the reaction, which was monitored by TLC, acetone (5 mL) was added to the mixture, and by using Whatman filter paper, Co3O4 nanoparticles were separated. The desired product, which was in solution, was isolated by removing the solvents under vacuum at room temperature. A recrystallization technique in water/ethanol (1:1) was used to purify the synthesized derivatives.
Separated Co3O4 nanoparticles were washed thrice with ethanol and water and placed in the oven at 100°C for 24 h for reuse.
Yield: 89%; White powder; FT-IR (KBr, cm-1): 3,275, 1,672, 1,628, 1,564, 1,351; 1H NMR (DMSO-d6, 400 MHz), δ (ppm):1.34 (t, J = 8.4 Hz, 3H, CH3), 2.41 (s, 3H, CH3), 3.26–3.29 (m, 2H, OCH2), 4.41 (s, 2H, NCH2), 5.14 (s, 2H, NCH2), 6.69 (s, 1H, NH), 7.10 (d, J = 7.6 Hz, 2H, Ar-H), 7.25–7.32 (m, 7H, Ar-H); 13C NMR (DMSO-d6, 100 MHz), δ (ppm):14.7, 20.6, 46.1, 48.3, 51.4, 97.6, 119.8, 127.7, 127.2, 128.6, 129.2, 134.9, 136.3, 139.8, 164.5, 165.1; Element analysis. Calcd for C21H22N2O3: C, 71.98; H, 6.33; N, 7.99; O, 13.70; Found: C, 71.96; H, 6.31; N, 7.98; O, 13.75.
Yield: 87%; Light yellow powder; FT-IR (KBr, cm−1): 3,301, 1,682, 1,637, 1,549, 1,377; 1H NMR (DMSO-d6, 400 MHz), δ (ppm): 1.29 (t, J = 7.4 Hz, 3H, CH3), 3.34–3.38 (m, 2H, CH2), 3.79 (s, 3H, OCH3), 4.46 (s, 2H, NCH2), 5.19 (s, 2H, NCH2), 6.63 (s, 1H, NH), 6.87 (d, J = 8.4 Hz, 2H, Ar-H), 7.31–7.34 (m, 5H, Ar-H), 7.59 (d, J = 8.4 Hz, 2H, Ar-H); 13C NMR (DMSO-d6, 100 MHz), δ (ppm):15.16, 46.4, 48.5, 51.7, 55.9, 95.1, 114.6, 120.6, 121.3, 127.4, 127.8, 128.5, 131.2, 135.1, 157.6, 164.4, 165.7; Element analysis. Calcd for C21H22N2O4: C, 68.84; H, 6.05; N, 7.65; O, 17.46; Found: C, 68.87; H, 6.04; N, 7.63; O, 17.46.
Yield: 92%; White powder; FT-IR (KBr, cm−1): 3,324, 1,677, 1,625, 1,531, 1,386; 1H NMR (DMSO-d6, 400 MHz), δ (ppm): 1.07–1.22 (m, 8H, CH2), 1.41–1.47 (m, 2H, CH2), 2.36 (s, 3H, CH3), 3.17–3.22 (m, 1H, CH), 3.71 (s, 3H, OCH3), 4.35 (s, 2H, NCH2), 6.54 (s, 1H, NH), 7.11–7.16 (m, 2H, Ar-H), 7.51–7.58 (m, 2H, Ar-H); 13C NMR (DMSO-d6, 100 MHz), δ (ppm): 20.5, 24.3, 25.6, 34.5, 48.5, 50.7, 59.3, 100.5, 119.7, 129.3, 134.2, 136.1, 164.3, 165.6; Element analysis. Calcd for C19H24N2O3: C, 69.49; H, 7.37; N, 8.53; O, 14.61; Found: C, 69.51; H, 7.35; N, 8.49; O, 14.65.
10 mol percent of Co3O4 nanoparticles, 1 mmol ethyl acetoacetate, 1 mmol phenylhydrazine were added to the 2 mL water/ethanol (1:1) and were stirred at 50°C. After the completion of the reaction, which was monitored by TLC, 1 mmol of malononitrile and 1 mmol of aldehyde were added and stirred at 50°C. After the completion of the reaction, which was monitored by TLC and cooled to room temperature, acetone (5 mL) was added to the mixture, and by using Whatman filter paper, Co3O4 nanoparticles were separated. The desired product, which was in solution, was isolated by removing the solvents under vacuum at room temperature. A recrystallization technique in water/ethanol (1:1) was used to purify the synthesized derivatives.
Separated Co3O4 nanoparticles were washed thrice with ethanol and water and placed in the oven at 100°C for 24 h for reuse.
Yield: 90%; Light yellow powder; FT-IR (KBr, cm-1): 3,431, 3,392, 3,112, 2,946, 2,911, 2,197, 1,663, 1,521, 1,452, 1,327, 1,232, 1,146; 1H NMR (300 MHz, DMSO-d6) δ = 1.91 (s, 3H, CH3), 3.75 (s, 3H, OCH3), 3.79 (s, 3H, OCH3), 3.82 (s, 3H, OCH3), 4.96 (s, 1H, -CH), 6.92 (s, 2H, Ar-H), 7.38 (s, 2H, NH2), 7.65–7.79 (m, 5H, Ar-H); 13C NMR (75 MHz, DMSO-d6) δ = 11.4, 33.9, 54.7, 55.3, 56.5, 59.6, 98.8, 111.7, 111.9, 119.7, 121.7, 122.3, 126.1 128.6, 129.4, 131.9, 137.7, 144.6, 145.5, 147.3, 148.7, 150.1, 163.2; Anal. Calcd for C23H22N4O4: C, 66.02; H, 5.30; N, 13.39; O, 15.29. Found: C, 66.05; H, 5.27; N, 13.42; O, 15.26.
Yield: 87%; yellow powder; FT-IR (KBr, cm-1): 3,429, 3,301, 32,778, 3,106, 2,981, 2,937, 2,144, 1,652, 1,557, 1,474, 1,360, 1,237, 1,118; 1H NMR (300 MHz, DMSO-d6) δ = 1.78 (s, 3H, CH3), 3.65 (s, 3H, OCH3), 4.85 (s, 1H, CH), 6.59 (d, 1H, J = 8.4 Hz, Ar-H), 6.62 (s, 1H, Ar-H), 6.71 (d, 1H, J = 8.4 Hz, Ar-H) 7.24 (s, 2H, NH2), 7.35–7.76 (m, 5H, Ar-H), 8.67 (s, 1H, OH); 13C NMR (75 MHz, DMSO-d6) δ = 13.4, 36.5, 55.9, 99.6, 112.7, 115.8, 117.1, 120.4, 121.7, 126.2, 129.5, 130.1, 135.2, 138.5, 144.7, 145.6, 146.1, 152.1, 160.8; Anal. Calcd for C21H18N4O3: C, 67.37; H, 4.85; N, 14.96; O, 12.82. Found: C, 67.40; H, 4.83; N, 14.98; O, 12.79.
By using the DPPH method, invitro antioxidant evaluation of derivatives was tested. For this purpose, previously reported methods were used, and the initial concentration of derivatives was 25, 50, 75, and 100 μg/mL in methanol (Bhaskara Reddy et al., 2015; Hosseinzadegan et al., 2020a). In the evaluations, Eq. 1 was used for the percent inhibition of the derivatives.
Calculation of percent inhibition (I %) of DPPH free radical by derivatives.
The CLSI (Clinical and Laboratory Standards Institute) standards and guidelines to evaluate the antimicrobial and antifungal activity of the derivatives were used. For this purpose, previous studies were used, and Inhibition Zone Diameter, Minimum Inhibitory Concentration, Minimum Bactericidal Concentration, and Minimum Fungicidal Concentration were reported after the tests. The microorganism strains used were Escherichia coli, Proteus mirabilis, and Yersinia enterocolitica as Gram-negative bacteria, Bacillus cereus, Staphylococcus aureus, and Rhodococcus equi as Gram-positive bacteria, and Candida albicans, Aspergillus fumigatus, and Fusarium oxysporum as fungal (Etemadi et al., 2016; Moghaddam-Manesh et al., 2020; Abdieva et al., 2022).
Co3O4 nanoparticles, which were synthesized by the previously reported method using cobalt nitrate and Pluronic F-127 (Sadasivan et al., 2013; Uddin and Baig, 2019)., were used as catalysts for the synthesis of pyrrole (Pyo) derivatives and pyrano [2,3-c]pyrazole (Pya[2,3-c]Pyz) derivatives after confirming the structure. FTIR spectrum, XRD pattern, BET analysis, XPS analysis, DLS analysis, SEM, and TEM images were used to verify the structure and characterization of Co3O4 nanoparticles.
In the FTIR spectrum of Co3O4 nanoparticles (Figure 1), Peaks related to Co(III)–O and Co(II)–O were observed in regions 534.25 cm−1 and 686.47 cm−1 (Salavati-Niasari et al., 2009).
Using the XRD pattern of synthesized Co3O4 nanoparticles (Figure 2) and the Scherrer equation, the size of nanoparticles 31 nm was calculated. The peaks at 19.44°, 31.66°, 37.48°, 38.87°, 45.27°, 55.75°, 59.48°, 65.31°, 68.46°, 74.12°, 77.73°, and 78.35° due to plates to [111], [220], [311], [222], [400], [422], [511], [440], [531], [620], [533], and [622] in XRD pattern of synthesized Co3O4 were observed. The cubic Co3O4 spinel oxide and JCPDS card number 1467–42, for synthesized cobalt oxide nanoparticles, were observed in the XRD pattern (Sadasivan et al., 2013; Uddin and Baig, 2019).
The same morphology of the synthesized Co3O4 nanoparticles was proved by using SEM and TEM images (Figure 3).
Based on the TEM image, the samples gradually move from the tube state to the plate state, which indicates the completion of the nucleation cycle. According to information in SEM and TEM images, there are no evidences of agglomeration in the structures. It can be related to optimal preparation rout of the final products as well as the proportions of initial materials used in the procedure (Mirhosseini et al., 2022).
The particle size distribution of Co3O4 nanoparticles (Figure 4) was measured using dynamic light scattering (DLS) technique, and the average size of cobalt nanoparticles was 35 nm.
Figure 5 shows X-ray photoelectron spectroscopy analysis (XPS) of Co3O4 nanoparticles. The binding energy peaks related to the 2p1/2 of Cobalt and the 2p3/2 of Cobalt were observed in 780.1 eV and 795.4 eV, respectively (Fan et al., 2016; Yang et al., 2016; Aboelazm et al., 2018).
The nitrogen adsorption/desorption isotherms of Co3O4 nanoparticles are shown in Figure 6.
Based on the analysis, nitrogen adsorption/desorption isotherms were IV-type (Alfaifi et al., 2023) and its surface area was 18.21 m2 g−1. The pore diameter, and pore volume of Co3O4 nanoparticles were 36.12 nm and 0.12 cm3 g−1, respectively. The surface area is an important factor in the properties and activity of nanoparticles and depends on their synthesis method (Khan et al., 2019). As the surface area increases, the contact surface of the nanoparticle increases, thereby increasing its reactivity (Tee et al., 2022). So, the high surface area of Co3O4 nanoparticles can increase its catalytic properties.
After confirming the structure of Co3O4 nanoparticles, their catalytic application was investigated in the synthesis of pyrrole (Pyo) derivatives and pyrano [2,3-c]pyrazole (Pya[2,3-c]Pyz) derivatives.
The optimization of reaction conditions, such as mol% of catalyst, solvent, and reaction temperature, was conducted to synthesize derivatives with the highest efficiency and conditions. For this purpose, the solvent and reaction temperature were maintained constant (ethanol solvent, room temperature), and the reactions were examined in conditions without catalyst, 5, 10, 15, 20, 25, 50, and 100 mol% of catalyst. The highest efficiency for synthesizing Pyo and Pya[2,3-c]Pyz was obtained when 15 mol% and 10 mol% of catalyst were used, respectively. Then, at a temperature of 25°C, the amount of 15 mol% of catalyst in the synthesis of Pyo and 10 mol% of Co3O4 nanoparticles in pyrano [2,3-c]pyrazole, using solvents of water, methanol, ethanol, 1:1 water/ethanol mixture, and dimethylformamide (DMF), reactions were carried out. The highest efficiency obtained in the reactions was when a 1:1 water/ethanol mixture was used. Finally, by keeping the amount of catalyst constant according to the previous step and using water/ethanol mixed solvent, the reactions were evaluated at 40°C, 50°C, 60°C, 70°C and reflux temperatures. The synthesis of Pyo at 25°C and Pya[2,3-c]Pyz at 40°C had the highest efficiency. The evaluation results are given in Table 1 for synthesizing Pyo and pyrano [2,3-c]pyrazole.
Table 1. Optimization of catalyst, solvent, and temperature in synthesis pyrrole (Pyo) (5a) and pyrano [2, 3-c]pyrazole (Pya[2, 3-c]Pyz) (10a).
Therefore, the optimal conditions, including using a 15 mol% catalyst, a 1:1 mixture of water/ethanol, and a temperature of 25 °C, were used to synthesize other Pyo derivatives (Table 2).
In synthesizing Pya[2,3-c]Pyz derivatives, optimal conditions, including 10 mol% of catalyst, 1:1 mixture of water/ethanol, and temperature of 40 °C were used (Table 3).
In all reactions to obtain optimal conditions in the synthesis of Pyo (5a), raw materials including cyclohexanamine, dimethyl but-2-ynedioate, formaldehyde and aniline have been used in the amount of 1 mol.
In all reactions to obtain optimal conditions in the synthesis of Pya[2,3-c]Pyz (10a), raw materials including ethyl acetoacetate, phenylhydrazine, malononitrile, and benzaldhyde have been used in the amount of 1 mol.
The structure of the derivatives has been confirmed using 1H NMR, 13C NMR, and element analysis.
Based on previous studies, Co3O4 nanoparticles act as Lewis acid catalysts in organic chemistry reactions (Rajabi et al., 2015; Gong et al., 2023). Here, too, Co3O4 nanoparticles enter the reaction as a Lewis acid, and Scheme 2 and 3 mechanisms are proposed for synthesizing Pyo derivatives and Pya[2,3-c]Pyz derivatives, respectively.
Scheme 1. Co3O4 nanoparticles as a catalyst in the synthesis of pyrrole (Pyo) derivatives (I) and pyrano [2, 3-c]pyrazole (Pya[2, 3-c]Pyz) derivatives (II).
Scheme 3. Proposed mechanism and use of Co3O4 nanoparticles in synthesis of pyrano [2, 3-c]pyrazole (Pya[2, 3-c]Pyz) derivatives.
The but-2-ynedioate derivatives and aliphatic amine derivatives created intermediate (I). The formaldehyde and aromatic amine derivatives created intermediate (II). Intermediate (III) was synthesized from the reaction of intermediate (I) with intermediate (II). From the cyclization reaction of intermediate (III), and tautomerization (IV), the final product was synthesized.
First, using ethyl acetoacetate and phenylhydrazine, the pyrazole was synthesized that were equilibrium in two forms (I and II). Using condensation of malononitrile and aldehyde derivative, intermediate (III) was synthesized. The intermediate (IV) was formed from Michael addition reaction of intermediate (II) with intermediate (III). The final product was synthesized through the cyclization reaction of intermediate (IV) and tautomerization (V).
Risibility and reuse of the catalyst in synthesizing Pyo derivatives and Pya[2, 3-c]Pyz derivatives were investigated. The Co3O4 nanoparticles after separation, washing, and drying, were reuse in the synthesis of derivatives. The results of reuse and reusability of the catalyst up to six times are shown in Figure 7.
Figure 7. The ability to reuse of Co3O4 nanoparticles in synthesis pyrrole (Pyo) [(I)-5a] and pyrano [2, 3-c]pyrazole (Pya[2, 3-c]Pyz) [(II)-10a].
After recycling, the XRD and XPS of Co3O4 nanoparticles were prepared, and as can be seen in Figure 8, were similar to before being used as a catalyst.
According to the reported methods, a hot filtration test for Co3O4 nanoparticles was done, and results showed no enhancement in conversion was noticed in the filtrate (Babaei and Mirjalili, 2020).
Comparison of synthesis methods of derivatives Pyo and Pya[2,3-c]Pyz reported in recent literature with Co3O4 nanoparticles used in this study are given in Tables 4,5.
Table 4. Comparison of recently reported conditions in the synthesis of pyrrole (Pyo) (5a) with Co3O4 nanoparticles.
Table 5. Comparison of recently reported conditions in the synthesis of pyrano [2,3-c]pyrazole (Pya[2,3-c]Pyz) (10a) with Co3O4 nanoparticles.
To summarize, nanoparticles have a high capacity to synthesize Pyo derivatives and Pya[2,3-c]Pyz derivatives with high efficiency and more suitable conditions than other catalysts (Table 5; Table 6). Suitable conditions include less reaction time, lower temperature used in the synthesis of derivatives and higher efficiency. The synthesis of new derivatives is another advantage of this study. It is suggested that nanoparticles can synthesize other heterocyclic compounds under suitable conditions as a recyclable green catalyst. The reason for the high catalytic property of cobalt nanoparticles, as explained in Section 3.1, can be attributed to its high active surface (Khan et al., 2019; Tee et al., 2022).
Table 6. The percent inhibition and IC50 of pyrrole (Pyo) derivatives and pyrano [2,3-c]pyrazole (Pya[2,3-c]Pyz) derivatives against DPPH free radical.
To check the antioxidant activity of derivatives, the DPPH method was used. 1 mL of derivatives with concentrations prepared as given in Section 2.5 was mixed with 4 mL of DPPH solution, and after half an hour of stirring in the dark, its absorbance was checked at 517 nm. The percent inhibition was calculated for each derivative concentration using the equation presented in Section 2.5, and the results are given in Table 6. In addition to percent inhibition, using concentration and percent inhibition, the value of IC50 for each of the derivatives was calculated (Beyzaei et al., 2018) and listed in Table 6.
Comparing the results of Pyo derivatives shows that the value of IC50 for them was very close, so it is not dependent on R1, R2, and Aryl groups. Therefore, mechanism Scheme 4 is suggested for DPPH free radical stability by Pyo derivatives. The IC50 value for Pya[2,3-c]Pyz derivatives was very close to each, and were not dependent on R3 groups. Therefore, mechanism Scheme 5 is suggested for DPPH free radical stability by Pya[2,3-c]Pyz derivatives.
Scheme 4. Suggested mechanism and resonance structures for DPPH free radical stabilization using pyrrole (Pyo) derivatives.
Scheme 5. Suggested mechanism and resonance structures for DPPH free radical stabilization using pyrano [2, 3-c]pyrazole (Pya[2, 3-c]Pyz) derivatives.
Although based on the proposed mechanisms, the resonance structures for Pyo derivatives and Pya[2,3-c]Pyz derivatives are equal in DPPH free radical stability, but as can be seen from the results, the antioxidant property of Pyo derivatives is more than that of Pya[2,3-c]Pyz derivatives. The obtained results are consistent with the proposed mechanisms because the resonance structures of Pyo derivatives are more stable than the resonance structures of Pya[2,3-c]Pyz derivatives (Hosseinzadegan et al., 2020b; Gani and Al-Obaidi, 2023).
The bacterial and fungal strains mentioned in Section 2.6 were used in the evaluation and antimicrobial tests of synthesized Pyo derivatives and Pya[2,3-c]Pyz derivatives. The results of antibacterial activity (against Gram-positive strains and Gram-negative strains) of Pyo derivatives were given in Table 7, antibacterial activity (against Gram-positive strains and Gram-negative strains) of Pya[2,3-c]Pyz derivatives were presented in Table 8, and antifungal activity of derivatives Pyo derivatives and Pya[2,3-c]Pyz derivatives were shown in Table 9. In the investigation of the antibacterial activity of the derivatives IZD, MIC, and MBC, and the investigation of the antifungal activity of the derivatives, IZD, MIC, and MFC have been tested and reported. To comparison of antimicrobial studies, tests were performed on commercial drugs such as Gentamicin and Terbinafin.
Table 7. The obtained IZD, MIC, and MBC values in antibacterial properties of pyrrole (Pyo) derivatives.
Table 8. The obtained IZD, MIC, and MBC values in antibacterial properties of pyrano [2,3-c]pyrazole (Pya[2,3-c]Pyz) derivatives.
Table 9. The obtained IZD, MIC, and MFC values in antifungal properties of pyrrole (Pyo) derivatives and pyrano [2,3-c]pyrazole (Pya[2,3-c]Pyz) derivatives.
The results proved that the antibacterial and antifungal properties of some synthesized Pyo derivatives and Pya[2,3-c]Pyz derivatives were more effective than commercial drugs. As shown in Tables 5–7, 5o and 10j were more effective than Gentamicin and Terbinafin.
Based on the results, in contrast to antioxidant activity, the high antimicrobial activity of Pya[2,3-c]Pyz derivatives compared to Pyo derivatives is significant and can be attributed to the heterocyclic rings present in their structure. Pyo derivatives contain one heterocyclic ring, but Pya[2,3-c]Pyz derivatives comprise two (Sharma et al., 2010; Al-Mulla, 2017).
The results of the antimicrobial activity of Pyo derivatives showed that they have more potent antibacterial properties than antifungal properties. The comparison of the antimicrobial effects of Pyo derivatives showed that the antimicrobial properties have a direct relationship with the substitutions of the aryl group and derivatives with chlorine, and methoxy had the highest effectiveness, respectively.
Comparing the antibacterial activity and antifungal activity of Pya[2,3-c]Pyz derivatives was similar to Pyo derivatives and antibacterial activity was more than antifungal activity. From the comparison of the antimicrobial activity of Pya[2,3-c]Pyz derivatives, it was also proved that the activity of derivatives has a direct relationship with R3 groups. The derivatives with chlorine, fluorine, hydroxyl, methoxy, and bromine had the highest effectiveness, respectively.
In this study, Co3O4 nanoparticles were used as recyclable and green catalyst for the synthesis of pyrrole (Pyo) derivatives and pyrano [2,3-c]pyrazole (Pya[2,3-c]Pyz) derivatives and 5 novel derivatives were synthesized. Efficiency of 83%–96%, and 87%–95% in synthesizing Pyo derivatives and Pya[2,3-c]Pyz derivatives, respectively, were observed. Less synthesis time and higher efficiency compared to recent studies were the advantages of using Co3O4 nanoparticles. Recycling up to 6 times without noticeable change in efficiency was another advantage of using Co3O4 nanoparticles. Antioxidant tests of derivatives using DPPH free radical method and antimicrobial tests of derivatives on Gram-positive, Gram-negative bacterial strains, and fungal species were performed. In the antioxidant tests, percentage inhibition, and IC50, and in antimicrobial tests, IZD, MIC, MBC, and MFC were investigated and reported. In antioxidant tests, it was proved that Pyo derivatives have a higher antioxidant activity than Pya[2,3-c]Pyz derivatives, which can be fully verified by the proposed mechanisms for the stability of DPPH free radicals according to their structure. The antimicrobial activity of Pyo derivatives and Pya[2,3-c]Pyz derivatives were the opposite of antioxidant activity. The Pya[2,3-c]Pyz derivatives were generally more effective on the studied species than Pyo derivatives. In antimicrobial activity, the derivatives with chlorine, fluorine, hydroxyl, and methoxy had the highest effectiveness. In antimicrobial activity, some derivatives, for example, 5o and 10j, have high effectiveness than Gentamicin and Terbinafin which are known as commercial drugs.
The original contributions presented in the study are included in the article/Supplementary Material, further inquiries can be directed to the corresponding author.
TA: Resources, Writing–review and editing. MJ: Writing–original draft. SS: Conceptualization, Writing–review and editing. MA: Supervision, Writing–review and editing. UA: Formal Analysis, Writing–review and editing. MS: Methodology, Writing–review and editing. FR: Project administration, Writing–review and editing. AA: Writing–original draft. IA: Writing–original draft, Writing–review and editing. AlA: Validation, Writing–original draft, Writing–review and editing.
The author(s) declare that no financial support was received for the research, authorship, and/or publication of this article.
The authors extend their appreciation to the Deanship of Scientific Research at King Khalid University for funding this work through the large research group program under grant number (R.G.P.02/535/44).
The authors declare that the research was conducted in the absence of any commercial or financial relationships that could be construed as a potential conflict of interest.
All claims expressed in this article are solely those of the authors and do not necessarily represent those of their affiliated organizations, or those of the publisher, the editors and the reviewers. Any product that may be evaluated in this article, or claim that may be made by its manufacturer, is not guaranteed or endorsed by the publisher.
Abdieva, G. A., Patra, I., Al-Qargholi, B., Shahryari, T., Chauhan, N. P. S., and Moghaddam-Manesh, M. (2022). RETRACTED: an efficient ultrasound-assisted synthesis of Cu/Zn hybrid MOF nanostructures with high microbial strain performance. Front. Bioeng. Biotechnol. 10, 861580. doi:10.3389/fbioe.2022.861580
Aboelazm, E. A., Ali, G. A., and Chong, K. F. (2018). Cobalt oxide supercapacitor electrode recovered from spent lithium-ion battery. Chem. Adv. Mater 3, 67–73.
Alam, M. J., Alam, O., Alam, P., and Naim, M. J. (2015). A review on pyrazole chemical entity and biological activity. Int. J. Pharm. Sci. Res. 6, 1433–1442.
Alfaifi, S. Y., Adeosun, W. A., Asiri, A. M., and Rahman, M. M. (2023). Sensitive and rapid detection of aspartic acid with Co3O4-ZnO nanorods using differential pulse voltammetry. Biosensors 13, 88. doi:10.3390/bios13010088
Al-Mulla, A. (2017). A review: biological importance of heterocyclic compounds. Der Pharma Chem. 9, 141–147.
Azarifar, D., Badalkhani, O., and Abbasi, Y. (2018). Amino acid ionic liquid-based titanomagnetite nanoparticles: an efficient and green nanocatalyst for the synthesis of 1, 4-dihydropyrano [2, 3-c] pyrazoles. Appl. Organomet. Chem. 32, e3949. doi:10.1002/aoc.3949
Babaei, E., and Mirjalili, B. B. F. (2020). Fe3O4@ nano-dextrin/Ti (IV) as a bio-based magnetic nano-catalyst for facile synthesis of 2, 3-dihydroquinazolin-4 (1H)-ones. Iran. J. Catal. 10, 219–226.
Baral, N., Mishra, D. R., Mishra, N. P., Mohapatra, S., Raiguru, B. P., Panda, P., et al. (2020). Microwave-assisted rapid and efficient synthesis of chromene-fused pyrrole derivatives through multicomponent reaction and evaluation of antibacterial activity with molecular docking investigation. J. Heterocycl. Chem. 57, 575–589. doi:10.1002/jhet.3773
Basirat, N., Sajadikhah, S. S., and Zare, A. (2020). Multi-component synthesis of piperidines and dihydropyrrol-2-one derivatives catalyzed by a dual-functional ionic liquid. J. Chem. Res. 44, 20–24. doi:10.1177/1747519819883881
Beyzaei, H., Deljoo, M. K., Aryan, R., Ghasemi, B., Zahedi, M. M., and Moghaddam-Manesh, M. (2018). Green multicomponent synthesis, antimicrobial and antioxidant evaluation of novel 5-amino-isoxazole-4-carbonitriles. Chem. Central J. 12, 1–8. doi:10.1186/s13065-018-0488-0
Bhardwaj, V., Gumber, D., Abbot, V., Dhiman, S., and Sharma, P. (2015). Pyrrole: a resourceful small molecule in key medicinal hetero-aromatics. Rsc Adv. 5, 15233–15266. doi:10.1039/c4ra15710a
Bhaskara Reddy, M. V., Srinivasulu, D., Peddanna, K., Apparao, C., and Ramesh, P. (2015). Synthesis and antioxidant activity of new thiazole analogues possessing urea, thiourea, and selenourea functionality. Synth. Commun. 45, 2592–2600. doi:10.1080/00397911.2015.1095929
Biswas, S. K., and Das, D. (2022). One-pot synthesis of pyrano [2, 3-c] pyrazole derivatives via multicomponent reactions (MCRs) and their applications in medicinal chemistry. Mini-Reviews Org. Chem. 19, 552–568. doi:10.2174/1570193x19666211220141622
Boichuk, S., Galembikova, A., Syuzov, K., Dunaev, P., Bikinieva, F., Aukhadieva, A., et al. (2021). The design, synthesis, and biological activities of pyrrole-based carboxamides: the novel tubulin inhibitors targeting the colchicine-binding site. Molecules 26, 5780. doi:10.3390/molecules26195780
Chacko, P., and Shivashankar, K. (2017). Nano structured spinel Co3O4-catalyzed four component reaction: a novel synthesis of Ugi adducts from aryl alcohols as a key reagent. Chin. Chem. Lett. 28, 1619–1624. doi:10.1016/j.cclet.2017.04.015
Costanzo, P., Calandruccio, C., Di Gioia, M. L., Nardi, M., Oliverio, M., and Procopio, A. (2018). First multicomponent reaction exploiting glycerol carbonate synthesis. J. Clean. Prod. 202, 504–509. doi:10.1016/j.jclepro.2018.08.120
Damera, T., Pagadala, R., Rana, S., and Jonnalagadda, S. B. (2023). A concise review of multicomponent reactions using novel heterogeneous catalysts under microwave irradiation. Catalysts 13, 1034. doi:10.3390/catal13071034
Eftekhari Far, B., and Nasr-Esfahani, M. (2020). Synthesis, characterization and application of Fe3O4@ SiO2@ CPTMO@ DEA-SO3H nanoparticles supported on bentonite nanoclay as a magnetic catalyst for the synthesis of 1, 4-dihydropyrano [2, 3-c] pyrazoles. Appl. Organomet. Chem. 34, e5406. doi:10.1002/aoc.5406
Etemadi, Y., Shiri, A., Eshghi, H., Akbarzadeh, M., Saadat, K., Mozafari, S., et al. (2016). Synthesis, characterisation, and in vitro antibacterial evaluation of a new class of 2-substituted-4-methyl-7, 8-dihydro-5H-pyrimido [4, 5-d] thiazolo [3, 2-a] pyrimidines. J. Chem. Res. 40, 600–603. doi:10.3184/174751916x14737838285904
Faisal, M., Saeed, A., Hussain, S., Dar, P., and Larik, F. A. (2019). Recent developments in synthetic chemistry and biological activities of pyrazole derivatives. J. Chem. Sci. 131, 70–30. doi:10.1007/s12039-019-1646-1
Fan, H., Quan, L., Yuan, M., Zhu, S., Wang, K., Zhong, Y., et al. (2016). Thin Co3O4 nanosheet array on 3D porous graphene/nickel foam as a binder-free electrode for high-performance supercapacitors. Electrochimica Acta 188, 222–229. doi:10.1016/j.electacta.2015.12.011
Faria, J. V., Vegi, P. F., Miguita, A. G. C., Dos Santos, M. S., Boechat, N., and Bernardino, A. M. R. (2017). Recently reported biological activities of pyrazole compounds. Bioorg. Med. Chem. 25, 5891–5903. doi:10.1016/j.bmc.2017.09.035
Gani, I. H., and Al-Obaidi, Z. (2023). MgO NPs catalyzed the synthesis of novel pyridin-3-yl-pyrimidin-2-yl-aminophenyl-amide derivatives and evaluation of pharmacokinetic profiles and biological activity. Front. Mater. 10, 1057677. doi:10.3389/fmats.2023.1057677
Garazd, Y. L., and Garazd, M. (2016). Natural dibenzo [b, d] pyran-6-ones: structural diversity and biological activity. Chem. Nat. Compd. 52, 1–18. doi:10.1007/s10600-016-1536-4
Gong, Y., Wang, N., Gao, E., Wan, Q., Bai, X., He, X., et al. (2023). Nitrogen-doped carbon confined cobalt nanoparticles as the steric acid-base multifunctional catalysts for Knoevenagel condensation. Mol. Catal. 550, 113521. doi:10.1016/j.mcat.2023.113521
Heravi, M. M., Malakooti, R., Kafshdarzadeh, K., Amiri, Z., Zadsirjan, V., and Atashin, H. (2022). Supported palladium oxide nanoparticles in Al-SBA-15 as an efficient and reusable catalyst for the synthesis of pyranopyrazole and benzylpyrazolyl coumarin derivatives via multicomponent reactions. Res. Chem. Intermed. 48, 203–234. doi:10.1007/s11164-021-04619-z
Hosseinzadegan, S., Hazeri, N., and Maghsoodlou, M. T. (2020a). Synthesis and evaluation of antimicrobial and antioxidant activity of novel 7-Aryl-6H, 7H-benzo [f] chromeno [4, 3-b] chromen-6-one by MgO nanoparticle as green catalyst. J. Heterocycl. Chem. 57, 621–626. doi:10.1002/jhet.3796
Hosseinzadegan, S., Hazeri, N., Maghsoodlou, M. T., Moghaddam-Manesh, M., and Shirzaei, M. (2020b). Synthesis and evaluation of biological activity of novel chromeno [4, 3-b] quinolin-6-one derivatives by SO 3 H-tryptamine supported on Fe 3 O 4@ SiO 2@ CPS as recyclable and bioactive magnetic nanocatalyst. J. Iran. Chem. Soc. 17, 3271–3284. doi:10.1007/s13738-020-01990-3
Javahershenas, R., and Nikzat, S. (2023). Recent advances in the multicomponent synthesis of heterocycles using tetronic acid. RSC Adv. 13, 16619–16629. doi:10.1039/d3ra02505e
Jeelan Basha, N., Basavarajaiah, S., and Shyamsunder, K. (2022). Therapeutic potential of pyrrole and pyrrolidine analogs: an update. Mol. Divers. 26, 2915–2937. doi:10.1007/s11030-022-10387-8
Kafi-Ahmadi, L., Poursattar Marjani, A., and Nozad, E. (2021). Ultrasonic-assisted preparation of Co3O4 and Eu-doped Co3O4 nanocatalysts and their application for solvent-free synthesis of 2-amino-4H-benzochromenes under microwave irradiation. Appl. Organomet. Chem. 35, e6271. doi:10.1002/aoc.6271
Khan, A. T., Ghosh, A., and Khan, M. M. (2012). One-pot four-component domino reaction for the synthesis of substituted dihydro-2-oxypyrrole catalyzed by molecular iodine. Tetrahedron Lett. 53, 2622–2626. doi:10.1016/j.tetlet.2012.03.046
Khan, I., Saeed, K., and Khan, I. (2019). Nanoparticles: properties, applications and toxicities. Arabian J. Chem. 12, 908–931. doi:10.1016/j.arabjc.2017.05.011
Khan, M. M., Khan, S., Iqbal, S., and Yousuf, R. (2016). Synthesis of functionalized dihydro-2-oxypyrroles and tetrahydropyridines using 2, 6-pyridinedicarboxylic acid as an efficient and mild organocatalyst. New J. Chem. 40, 7504–7512. doi:10.1039/c6nj01170e
Mirhosseini, H., Mostafavi, A., Shamspur, T., and Sargazi, G. (2022). Fabrication of an efficient ternary TiO2/Bi2WO6 nanocomposite supported on g-C3N4 with enhanced visible-light-photocatalytic activity: modeling and systematic optimization procedure. Arabian J. Chem. 15, 103729. doi:10.1016/j.arabjc.2022.103729
Moghaddam-Manesh, M., Ghazanfari, D., Sheikhhosseini, E., and Akhgar, M. (2020). Synthesis, characterization and antimicrobial evaluation of novel 6'-Amino-spiro [indeno [1, 2-b] quinoxaline [1, 3] dithiine]-5'-carbonitrile derivatives. Acta Chim. Slov. 67, 276–282. doi:10.17344/acsi.2019.5437
Mohamadpour, F. (2020a). Imin-based synthesis of polyfunctionalized dihydro-2-oxypyrroles catalyzed by glycine amino acid via tandem Michael–Mannich cyclocondensation reaction under ambient temperature. Res. Chem. Intermed. 46, 1931–1940. doi:10.1007/s11164-019-04072-z
Mohamadpour, F. (2020b). Potassium alum as a naturally mineral and economical catalyst for the one-pot, multi-component and clean synthesis of 2-oxo (thio)-1, 2, 3, 4-tetrahydropyrimidines and N-aryl-3-aminodihydropyrrol-2-one-4-carboxylates. Indian J. Chemistry-Section B (IJC-B) 59, 1183–1190.
Mohamadpour, F. (2022). The development of imin-based tandem Michael–Mannich cyclocondensation through a single-electron transfer (SET)/energy transfer (EnT) pathway in the use of methylene blue (MB+) as a photo-redox catalyst. RSC Adv. 12, 10701–10710. doi:10.1039/d2ra01190e
Mohamadpour, F., Lashkari, M., Maghsoodlou, M. T., and Heydari, R. (2018). Phthalic acid: a green, biodegradable and environmentally benign nature di-functional brønsted acid catalyst for the one-pot synthesis of 3, 4-dihydropyrimidin-2- (1h)-one derivatives and substituted dihydro-2-oxypyrroles. J. Chil. Chem. Soc. 63, 3811–3818. doi:10.4067/s0717-97072018000103811
Muthuraja, P., Prakash, S., Siva, G., Muthusubramanian, S., and Manisankar, P. (2017). Expedient ytterbium triflate catalyzed one-pot mulicomponent synthesis of spiro [indoline-3, 4′-pyrano [2, 3-c] pyrazole]. ChemistrySelect 2, 10071–10075. doi:10.1002/slct.201701261
Nickraftar, M., Hajivar, N. N., Aboonajmi, J., and Fereidooni, E. (2016). Nano Fe 3 O 4 as a magnetically recyclable, powerful, and stable catalyst for the multi-component synthesis of highly functionalized dihydro-2-oxopyrroles. Res. Chem. Intermed. 42, 2899–2908. doi:10.1007/s11164-015-2185-0
Paul, S., Pradhan, K., Ghosh, S., De, S., and Das, A. R. (2014). Uncapped SnO2 quantum dot catalyzed cascade assembling of four components: a rapid and green approach to the pyrano [2, 3-c] pyrazole and spiro-2-oxindole derivatives. Tetrahedron 70, 6088–6099. doi:10.1016/j.tet.2014.02.077
Petri, G. L., Spanò, V., Spatola, R., Holl, R., Raimondi, M. V., Barraja, P., et al. (2020). Bioactive pyrrole-based compounds with target selectivity. Eur. J. Med. Chem. 208, 112783. doi:10.1016/j.ejmech.2020.112783
Rahimi, Z., Bayat, M., and Hosseini, H. (2022). New multicomponent reactions in water: a facile synthesis of 1, 3-dioxo-2-indanilidene-heterocyclic scaffolds and indenoquinoxalines through reaction of ninhydrin-malononitrile adduct with diverse N-binucleophiles. RSC Adv. 12, 33772–33779. doi:10.1039/d2ra06469c
Rajabi, F., Raessi, M., Arancon, R. A., Saidi, M. R., and Luque, R. (2015). Supported cobalt oxide nanoparticles as efficient catalyst in esterification and amidation reactions. Catal. Commun. 59, 122–126. doi:10.1016/j.catcom.2014.09.044
Rao, R. N., and Chanda, K. (2020). An assessment study of known pyrazolopyrimidines: chemical methodology and cellular activity. Bioorg. Chem. 99, 103801. doi:10.1016/j.bioorg.2020.103801
Rawat, P., Singh, R., Ranjan, A., Gautam, A., Trivedi, S., and Kumar, M. (2021). Study of antimicrobial and antioxidant activities of pyrrole-chalcones. J. Mol. Struct. 1228, 129483. doi:10.1016/j.molstruc.2020.129483
Reddy, G. M., Camilo Jr, A., and Garcia, J. R. (2021). Pyrrole-2, 5-dione analogs as a promising antioxidant agents: microwave-assisted synthesis, bio-evaluation, SAR analysis and DFT studies/interpretation. Bioorg. Chem. 106, 104465. doi:10.1016/j.bioorg.2020.104465
Sadasivan, S., Bellabarba, R. M., and Tooze, R. P. (2013). Size dependent reduction–oxidation–reduction behaviour of cobalt oxide nanocrystals. Nanoscale 5, 11139–11146. doi:10.1039/c3nr02877a
Salavati-Niasari, M., Mir, N., and Davar, F. (2009). Synthesis and characterization of Co3O4 nanorods by thermal decomposition of cobalt oxalate. J. Phys. Chem. Solids 70, 847–852. doi:10.1016/j.jpcs.2009.04.006
Sameri, F., Bodaghifard, M. A., and Mobinikhaledi, A. (2021). Zn (II)-Schiff base covalently anchored to CaO@ SiO2: a hybrid nanocatalyst for green synthesis of 4H-pyrans. Appl. Organomet. Chem. 35, e6394. doi:10.1002/aoc.6394
Sedighinia, E., Badri, R., and Kiasat, A. (2019). Application of yttrium iron garnet as a powerful and recyclable nanocatalyst for one-pot synthesis of pyrano [2, 3-c] pyrazole derivatives under solvent-free conditions. Russ. J. Org. Chem. 55, 1755–1763. doi:10.1134/s1070428019110186
Seipp, K., Geske, L., and Opatz, T. (2021). Marine pyrrole alkaloids. Mar. Drugs 19, 514. doi:10.3390/md19090514
Shahbazi, S., Ghasemzadeh, M. A., Shakib, P., Zolfaghari, M. R., and Bahmani, M. (2019). Synthesis and antimicrobial study of 1, 4-dihydropyrano [2, 3-c] pyrazole derivatives in the presence of amino-functionalized silica-coated cobalt oxide nanostructures as catalyst. Polyhedron 170, 172–179. doi:10.1016/j.poly.2019.04.063
Sharghi, H., Aboonajmi, J., Mozaffari, M., Doroodmand, M. M., and Aberi, M. (2018). Application and developing of iron-doped multi-walled carbon nanotubes (Fe/MWCNTs) as an efficient and reusable heterogeneous nanocatalyst in the synthesis of heterocyclic compounds. Appl. Organomet. Chem. 32, e4124. doi:10.1002/aoc.4124
Sharma, V., Kumar, P., and Pathak, D. (2010). Biological importance of the indole nucleus in recent years: a comprehensive review. J. Heterocycl. Chem. 47, 491–502. doi:10.1002/jhet.349
Shi, D., Mou, J., Zhuang, Q., Niu, L., Wu, N., and Wang, X. (2004). Three-component one-pot synthesis of 1, 4-dihydropyrano [2, 3-c] pyrazole derivatives in aqueous media. Synth. Commun. 34, 4557–4563. doi:10.1081/scc-200043224
Sikandar, S., and Zahoor, A. F. (2021). Synthesis of pyrano [2, 3-c] pyrazoles: a review. J. Heterocycl. Chem. 58, 685–705. doi:10.1002/jhet.4191
Soltani, N., Rahman, J. U., Carvalho, P. A., and Finstad, T. G. (2023). A highly active and reusable multicomponent high entropy metal oxide catalyst for nitroarenes hydrogenation. Top. Catal. 67, 572–581. doi:10.1007/s11244-023-01846-z
Tee, G. T., Gok, X. Y., and Yong, W. F. (2022). Adsorption of pollutants in wastewater via biosorbents, nanoparticles and magnetic biosorbents: a review. Environ. Res. 212, 113248. doi:10.1016/j.envres.2022.113248
Uddin, M. K., and Baig, U. (2019). Synthesis of Co3O4 nanoparticles and their performance towards methyl orange dye removal: characterisation, adsorption and response surface methodology. J. Clean. Prod. 211, 1141–1153. doi:10.1016/j.jclepro.2018.11.232
Vairaperumal, V., Perumal, M., Sengodu, P., Shanumuganthan, S., and Paramasivam, M. (2019). V2O5-Catalyzed one-pot multicomponent of pyrazol naphthoquinone as scaffolds for potential bioactive compounds: synthesis, structural study and cytotoxic activity. ChemistrySelect 4, 3006–3010. doi:10.1002/slct.201803942
Veeramani, V., Muthuraja, P., Prakash, S., Senthil Kumar, M., Susaimanickam, A., and Manisankar, P. (2018). NbCl5-Catalyzed one-pot four component synthesis of spiro pyrazole and benzo [7, 8] chromene derivatives. ChemistrySelect 3, 10027–10031. doi:10.1002/slct.201802036
Yahyazadehfar, M., Sheikhhosseini, E., Ahmadi, S. A., and Ghazanfari, D. (2019). Microwave-associate synthesis of Co3O4 nanoparticles as an effcient nanocatalyst for the synthesis of arylidene barbituric and Meldrum's acid derivatives in green media. Appl. Organomet. Chem. 33, e5100. doi:10.1002/aoc.5100
Yang, J., Wei, F., Sui, Y., Qi, J., He, Y., Meng, Q., et al. (2016). Co 3 O 4 nanocrystals derived from a zeolitic imidazolate framework on Ni foam as high-performance supercapacitor electrode material. RSC Adv. 6, 61803–61808. doi:10.1039/c6ra11272b
Zhang, J.-N., Yang, X.-H., Guo, W.-J., Wang, B., and Zhang, Z.-H. (2017). Magnetic metal–organic framework CoFe2O4@ SiO2@ IRMOF-3 as an efficient catalyst for one-pot synthesis of functionalized dihydro-2-oxopyrroles. Synlett 28, 734–740. doi:10.1055/s-0036-1588924
Zhou, Q., Jia, L., Du, F., Dong, X., Sun, W., Wang, L., et al. (2020). Design, synthesis and biological activities of pyrrole-3-carboxamide derivatives as EZH2 (enhancer of zeste homologue 2) inhibitors and anticancer agents. New J. Chem. 44, 2247–2255. doi:10.1039/c9nj04713a
Keywords: green chemistry, multi-component reaction, antimicrobial evaluation, antioxidant evaluation, Co3O4 nanoparticles, pyrrole derivatives, Pyrano[2,3-c]pyrazole derivatives
Citation: Almeleebia TM, Jasim Naser M, Saeed SM, Abid MM, Altimari US, Shaghnab ML, Rasen FA, Alawadi A, Ahmad I and Alsalamy A (2024) Multi-component synthesis and invitro biological assessment of novel pyrrole derivatives and pyrano[2,3-c]pyrazole derivatives using Co3O4 nanoparticles as recyclable nanocatalyst. Front. Mater. 11:1354560. doi: 10.3389/fmats.2024.1354560
Received: 12 December 2023; Accepted: 25 June 2024;
Published: 31 July 2024.
Edited by:
Luiz Fernando Romanholo Ferreira, Catholic University of Brasilia (UCB), BrazilReviewed by:
Perumal Muthuraja, Yeungnam University, Republic of KoreaCopyright © 2024 Almeleebia, Jasim Naser, Saeed, Abid, Altimari, Shaghnab, Rasen, Alawadi, Ahmad and Alsalamy. This is an open-access article distributed under the terms of the Creative Commons Attribution License (CC BY). The use, distribution or reproduction in other forums is permitted, provided the original author(s) and the copyright owner(s) are credited and that the original publication in this journal is cited, in accordance with accepted academic practice. No use, distribution or reproduction is permitted which does not comply with these terms.
*Correspondence: Ali Alsalamy, YWxpaGFzaGltYWxzYWxhbXk3OEBnbWFpbC5jb20=
Disclaimer: All claims expressed in this article are solely those of the authors and do not necessarily represent those of their affiliated organizations, or those of the publisher, the editors and the reviewers. Any product that may be evaluated in this article or claim that may be made by its manufacturer is not guaranteed or endorsed by the publisher.
Research integrity at Frontiers
Learn more about the work of our research integrity team to safeguard the quality of each article we publish.