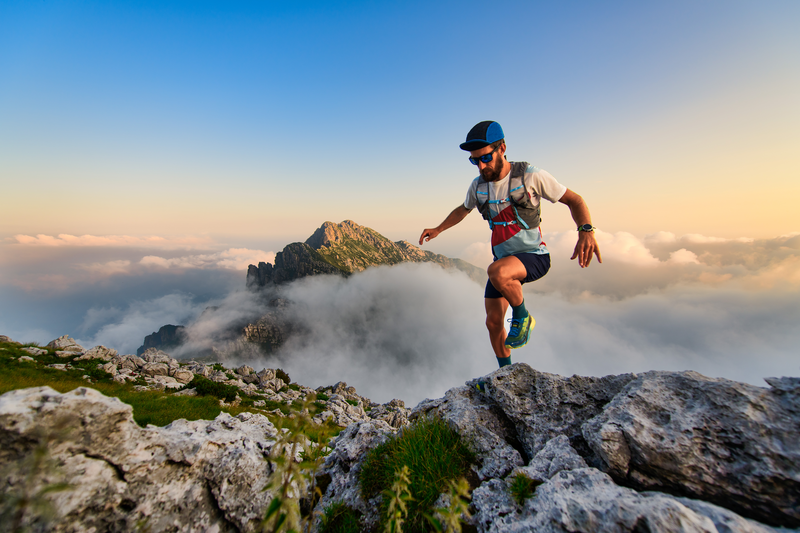
94% of researchers rate our articles as excellent or good
Learn more about the work of our research integrity team to safeguard the quality of each article we publish.
Find out more
ORIGINAL RESEARCH article
Front. Mater. , 13 December 2023
Sec. Environmental Degradation of Materials
Volume 10 - 2023 | https://doi.org/10.3389/fmats.2023.1302072
With the development of economy, the problem of heavy metal pollution in water environment is becoming more and more serious, so it is urgent to find a kind of efficient water purification material. The current work aimed to investigate the potential power of sodium vanadate nanowire arrays (Na5V12O32) to remove cadmium (Cd2+) and chromium (Cr3+) from simulated aqueous solutions. The adsorption effects of Na5V12O32 on Cd2+ and Cr3+ under different adsorption conditions were analyzed. The products before and after adsorption were compared by XRD, SEM, TEM, FTIR and XPS. The results showed that the irregular grass-like structure of Na5V12O32 nanowire arrays provided more active sites for the ion exchange reaction, and the maximum adsorption capacity of Cd2+ and Cr3+ was 541.2 and 251.8 mg·g-1, respectively. The pseudo-second-order kinetic model was more suitable to describe the adsorption behavior by kinetic study. The research demonstrated that Na5V12O32 nanowire arrays exhibited excellent adsorption performance, which provided an effective parameter basis for the future adsorption of heavy metal ions.
With the rapid economic developments, the ecological environment we live in is gradually being destroyed, and heavy metal pollution in water from metallurgy, mining, and other industries has aroused wide concern all over the world (Bensalah et al., 2023; Jebli et al., 2023; Lebkiri et al., 2023). Cadmium (Cd2+) and chromium (Cr3+) are common toxic heavy metals and have been identified as harmful to the human body (Elfeghe et al., 2022; Kocaoba et al., 2022). Studies have shown that small amounts of chromium and cadmium can have a significant toxic effect on humans (El Gaayda et al., 2023). In addition, chromium and cadmium can cause more serious health problems and even death after accumulation because the human body finds them difficult to discharge (Makarenko et al., 2019; Shahryari et al., 2019). Therefore, how to remove these heavy metal ions becomes extremely urgent.
Recently, a variety of effective methods have been developed to remove heavy metal ions from water, such as chemical precipitation (Wu, 2019), electrochemical treatment (Liu et al., 2019a), solvent extraction (Ishfaq et al., 2019), membrane separation (Song et al., 2022), and ion exchange (Rengaraj et al., 2002; Bentouami and Oulai, 2006; Elkady et al., 2011; Arim et al., 2018; Liu et al., 2019b; Priastomo et al., 2020). In the above methods, the ion exchange technique is the most widely used method in removing heavy metal ions because it is environmentally friendly, economically applicable, renewable, and highly efficient. Elkady et al. (Elkady et al., 2011) prepared nano-poly (glycidyl methacrylate) cation exchange resin to remove Cd2+ and the adsorption capacity could reach 90%. The results showed that initial cadmium concentration, pH value, and resin dosage had a great influence on adsorption. Bentouami et al. (Bentouami and Oulai, 2006) modified the bentonite and prepared bentonite-oxine complex for cadmium removal. After the batch test, the maximum adsorption capacity reached 61.35 mg·g-1. Liu et al. (Liu et al., 2019b) synthesized an eco-friendly gamma-cyclodextrin metal-organic framework (MOF)-based nanoporous carbon material to remove Cd2+ from the solution, and used the Langmuir model to calculate the maximum adsorption capacity which was 140.85 mg·g-1. Ion exchange technology can also be used to remove chromium ions. Rengaraj et al. (Rengaraj et al., 2002) studied the adsorption of Cr3+ by IRN77 cation-exchange resin, and it was found that the adsorption efficiency could exceed 95% under the best reaction conditions. Arim et al. (Arim et al., 2018) found that chemically modified pine bark had a good adsorption effect on chromium. Under the action of ion exchange, the maximum adsorption capacity was 31.40 mg·g-1 when pH was 5. Jumina et al. (Priastomo et al., 2020) successfully prepared a simple adsorbent named C-Phenylcalix (Elfeghe et al., 2022) pyrogallolarene, and evaluated for the simultaneous adsorption of Cr3+ in acidic media. The results indicated that the adsorption capacity of Cr3+ was found to be 14.31 mg·g-1. However, there are still some problems regarding the aforementioned adsorbent materials, which have complex preparation and relatively low adsorption capacity (Kadiri et al., 2021; El amri et al., 2023). Therefore, adsorbent materials with low cost, high performance, and simple preparation are highly required.
In the present study, sodium vanadate (Na5V12O32) nanowire arrays were fabricated by means of a hydrothermal method. The samples were characterized by using scanning electron microscopy, Fourier transform infrared spectrum, and X-ray diffraction. Different methods were used to characterize the Na5V12O32 nanowire arrays before and after the adsorption of Cd2+ and Cr3+. By examining the effects of adsorbent dosage and adsorption time during the adsorption experiments to confirm the advantage of Na5V12O32 nanowire arrays.
Ammonium metavanadate (NH4VO3, ≥99.9%), sodium chloride (NaCl, ≥99.9%), oxalic acid (H₂C₂O₄, ≥99.9%), hexamethylene tetramine (C6H12N4, HMTA, ≥99.7%), cadmium nitrate (CdN2O6, ≥99.9%), and chromium trichloride (CrCl3, ≥99.9%) were all purchased from the Sinopharm. Co. Ltd. All chemicals were used as received. Deionized water was prepared in the laboratory. The simulated wastewater containing Cd2+ and Cr3+ was prepared by dissolving amounts of CdN2O6 and CrCl3 in deionized water, respectively.
As shown in Figure 1, Na5V12O32 nanowire arrays were prepared by a hydrothermal method (Cao et al., 2015). Ammonium metavanadate, sodium chloride, oxalic acid, and HMTA in a molar ratio of 1:6:2:0.2 were mixed in proportion with deionized water and stirred for 6 h to obtain the precursor solution. Then the precursor solution was added into a 25 mL polytetrafluoroethylene stainless steel autoclave (adding Ti foils), and heated 150°C for 1 h to synthesize Na5V12O32 nanowire arrays on Ti foil. After the reaction, the Ti foils were taken out when the temperature cooled down to room temperature, and the samples were washed and dried to obtain Na5V12O32 nanowire arrays.
X-ray diffraction (XRD, BrukerD8) was applied to check the possible composition of the samples before and after use. A scanning electron microscope (SEM, Hitachi) with an energy dispersive spectrometer (EDS) was used to analyze surface morphology and chemical elements composition. The structure of the products was characterized by transmission electron microscopy (TEM, Tecnai G2 F30S-TWIN). The chemical compositions of the samples were analyzed by X-ray photoelectron spectroscopy (XPS, KAlpha 1063). Fourier transform infrared spectroscopy (FTIR, ALPHA) was employed to investigate the functional groups of the sample. The concentration of metal ions in the solution was acquired by inductively coupled plasma optical emission spectroscopy (ICP-OES, 7400).
Stock solution A containing 1000 mg·L-1 Cd2+ was prepared by dissolving CdN2O6 in distilled water. Similarly, stock solution B containing 1000 mg·L-1 Cr3+ was obtained by adding CrCl3. Then, the desired solutions were prepared by dilution of the stock solution.
A series of adsorption experiments of Na5V12O32 nanowire arrays on the desired solutions were conducted, and the samples after adsorption were named Na5V12O32-Cd and Na5V12O32-Cr. The adsorption capacity of sodium vanadate was evaluated by the adsorbent dose and adsorption time. All experiments were performed at room temperature. After the reaction, the supernatant was collected for characterization and analysis, and the amount of adsorption was determined by ICP.
Sodium vanadate was used to adsorb heavy metal ions (Cd2+ and Cr3+) to determine the adsorption equilibrium time. At the stirring speed of 150 rpm, 0.1 g of Na5V12O32 was added to a solution of heavy metal ions with a concentration of 4,000 mg·L-1. The equilibrium adsorption capacity was calculated by the following equation (Eq. 1):
where,
The Na5V12O32 nanowire arrays of 0.1 g which reacted with the desired solution (the concentration of Cd2+ or Cr3+ was 4,000 mg·L-1) under stirring at 25°C were tested. The X-ray diffraction spectrum of the products before and after adsorption is shown in Figure 2. In Figure 2A, the prepared Na5V12O32 nanowire arrays had low crystallinity at room temperature, and the peaks near 15.2°, 22.8°, 29.8°, 38.8°, and 50.3° correspond to (200), (300), (−211), (−303), and (020) crystal faces of Na5V12O32 (JCPDS No.24-1156), respectively. In order to analyze the structure and composition of the adsorbed products more accurately, the adsorbed products were heated at different temperatures, and named Na5V12O32-Cd-300°C, Na5V12O32-Cd-500°C, Na5V12O32-Cr-300°C, and Na5V12O32-Cr-500°C, respectively, and are shown in Figure 2B. With the increase in temperature, the crystallinity of Na5V12O32-Cd and Na5V12O32-Cr improved, the diffraction peak became more acute, and the signals of many other peaks were displayed. In Figure 2C, the peaks of the sample belong to CdV2O6 (JCPDS No.73-0187), V2O5(JCPDS No.73-0187), and Na0.76V6O15 (JCPDS No.75-1653) which showed that an ion exchange reaction occurred between Cd2+ and Na5V12O32 to produce CdV2O6. Furthermore, the incompleteness of the chemical reaction was confirmed by the appearance of V2O5 and Na0.76V6O15. Similar results can be seen in Figure 2D as CrCl3 was converted to Cr2V4O13 due to ion exchange. The peaks of the sample belong to V2O5(JCPDS No.73-0187) and Na0.76V6O15 (JCPDS No.75-1653), which could also be ascribed to an incomplete ion exchange.
FIGURE 2. XRD patterns of products before and after adsorption: (A) Na5V12O32, (B) Na5V12O32-Cd-500°C, (C) Na5V12O32-Cd and Na5V12O32-Cr at different temperatures, (D) Na5V12O32-Cr-500°C.
The surface morphology of the samples was further studied using an SEM and the results are shown in Figure 3. As can be seen from Figure 3A, the surface of Na5V12O32 nanowire arrays was relatively smooth and showed an irregular grass-like structure which could increase the number of active sites, and the assays achieved efficient adsorption of Cd2+ and Cr3+ through ion exchange reaction. Supplementary Figure S1 shows the prepared Na5V12O32 nanowire arrays on a nanometer scale and the diameter was 157 nm.
FIGURE 3. SEM images of (A) Na5V12O32, (B) Na5V12O32-Cd, (C) Na5V12O32-Cr, (D) and (E) are the element mapping images of Na5V12O32-Cd and Na5V12O32-Cr, respectively.
The SEM images of the adsorbed product are shown in Figures 3B, C. The results showed that the structure of the Na5V12O32 nanowire arrays was stable, and there was no obvious change before and after adsorption. The volume of sodium vanadate after adsorption was slightly larger, and a small number of particles were attached to the surface. The element mapping images of Na5V12O32-Cd and Na5V12O32-Cr are shown in Figures 3D, E. The appearance of Cd and Cr proved the successful adsorption of Na5V12O32, and the sodium element indicated that the ion exchange reaction was incomplete, which was consistent with the results shown in Supplementary Figure S2.
In order to further verify the adsorption mechanism, the FTIR of Na5V12O32, Na5V12O32-Cd, and Na5V12O32-Cr were investigated and compared, and are shown in Figure 4. The characteristic adsorption peaks of the Na5V12O32 nanowire arrays (Vatanpour et al., 2021) were located at 1635.41 cm-1 and 1400.56 cm-1 (V-OH symmetrical tensile vibration and anti-symmetrical tensile vibration), 988.12 cm-1 (V=O tensile vibration), and 534.15 cm-1 (V-O-V symmetrical tensile vibration). After Cr3+ adsorption, the peaks mainly existed at 1629.68, 1393.39, 989.12, and 529.86 cm-1, and after Cd2+ adsorption, the peaks mainly existed at 1625.39, 1383.37, 992.42, and 532.73 cm-1. This indicated that before and after adsorption, the peaks had a small shift, which could be attributed to the stability of the vanadate structure and no change of functional group in the reaction. The characteristic sorption peaks at 1635.41 cm-1 were strong after the adsorption. This could be explained by the fact that V-OH vibration was strengthened after the ion exchange of Na+ to Cd2+ and Cr3+, making the corresponding bands strong (Harbi et al., 2016). The change in the peak at 1383.37 cm-1 was more obvious than that at 1393.39 cm-1, which could be due to the higher adsorption capacity. During the ion exchange process (Fang et al., 2021), the reaction equation (Eqs 2, 3) could be described as follows:
In order to explore the effect of adsorbent on the experiment, a series of adsorption experiments were conducted with different adsorbents with mass and an initial concentration of 100 mg·L-1. Figure 5 shows the effect of adsorbent dosage on the removal of Cd2+ and Cr3+. The results showed that with the increase in the dosage of Na5V12O32, the removal rate of Cd2+ and Cr3+ also increased, but the adsorption rate gradually slowed down. When the amount of adsorbent was 0.1 g, the removal rate of Cd2+ was increased by 90.1%, and when the amount of adsorbent was increased to 0.3 g, the removal rate of Cd2+ was 99.1%, which was only an increase of 9 percentage points. The same situation could also be found in the removal of Cr3+, as when the amount of adsorbent was 0.1 g, the removal rate of Cr3+ was increased by 70.4%, and when the amount of adsorbent increased to 0.3 g, the removal rate was 82.6%, which was only an increase of 12.6 percentage points. Therefore, 0.1 g was the best amount of adsorbent.
In order to explore the effect of adsorption time on the experiment, a series of adsorption experiments were conducted with different contact times and an initial concentration of 4,000 mg·L-1.
Figure 6 shows the influence of contact time on adsorption capacity. It indicates that the adsorption capacity of Na5V12O32 on Cd2+ and Cr3+ increased rapidly with the increase of contact time, and then tended to be stable. When the contact time was 36 h, the maximum adsorption capacity was 541.2 and 251.8 mg·g-1, respectively. This can be attributed to the gradual reduction of the ion exchange site of Na5V12O32 with the progress of the reaction, which makes the adsorption rate gradually decrease and finally tends to the adsorption equilibrium.
At the same time, the adsorption capacity of sodium vanadate was compared and is shown in Table 1. Compared with other adsorbents, the Na5V12O32 nanowire arrays in this study had a higher maximum adsorption capacity and were adsorbent with excellent adsorption performance to remove heavy metal ions from aqueous solution.
The analysis of adsorption kinetics was used to determine the adsorption rate and the equilibrium time during ion exchange. The adsorption of Cd2+ and Cr3+ by Na5V12O32 is a complex process, and the main behavior and mechanism of the adsorption process can be analyzed by certain models. In this experiment, a pseudo-first-order dynamic model (Dao et al., 2022), pseudo-second-order dynamic model (Khamizov, 2020), and Elovich dynamic model (Kaki et al., 2019) were established to find the most suitable model for the experimental data, and the results are shown in Figure 7. The kinetic parameters and correlation coefficients are shown in Table 2. The results show that the R2 values of the pseudo-second-order kinetic model are 0.9945 and 0.9949, respectively, which are much higher than those of the pseudo-first-order kinetic model and Elovich kinetic model. Therefore, the pseudo-second-order kinetic model is more suitable to describe the adsorption behavior of Cd2+ and Cr3+ by Na5V12O32.
FIGURE 7. Kinetic simulation of Na5V12O32 adsorption: Pseudo first order kinetic diagram of Cd2+ (A) and (B) Cr3+ adsorbed on Na5V12O32; Pseudo second order kinetic diagram of (C) Cd2+ and (D) Cr3+ adsorbed on Na5V12O32; Elovich kinetics diagram of (E) Cd2+ and (F) Cr3+ adsorbed on Na5V12O32.
In this experiment, sodium vanadate nanowire arrays (Na5V12O32) were synthesized by a simple hydrothermal method and used as an adsorbent to remove Cd2+ and Cr3+ from the simulated solution. The adsorption experiment results showed that the Na5V12O32 nanowire arrays had great adsorption potential, and the maximum adsorption capacity of Cd2+ and Cr3+ was 541.2 and 251.8 mg·g-1, respectively. The adsorption behavior was more consistent with the pseudo-second-order kinetic model. In summary, Na5V12O32 nanowire arrays are a promising candidate for heavy metal ion wastewater treatment, and the high adsorption performance is worthy of further exploration and research.
The original contributions presented in the study are included in the article/Supplementary Material, further inquiries can be directed to the corresponding authors.
DP: Writing–original draft. XF: Supervision, Visualization, Writing–review and editing. DF: Supervision, Writing–review and editing.
The author(s) declare financial support was received for the research, authorship, and/or publication of this article. This work was supported by the fund of the Key Special Projects of the Ministry of Science and Technology (China: 2021YFE0104300, Uzbekistan: MUK-2021-45), Major Special Projects in Yunnan Province (No. 202002AB080001), Science Research Project of Yunnan Province (No. 202001AT070082), Basic Research Project of Yunnan Province/General Project (No. 202001AT070002), and Kunming Academician Expert Workstation of Yunnan Province.
The authors declare that the research was conducted in the absence of any commercial or financial relationships that could be construed as a potential conflict of interest.
All claims expressed in this article are solely those of the authors and do not necessarily represent those of their affiliated organizations, or those of the publisher, the editors and the reviewers. Any product that may be evaluated in this article, or claim that may be made by its manufacturer, is not guaranteed or endorsed by the publisher.
The Supplementary Material for this article can be found online at: https://www.frontiersin.org/articles/10.3389/fmats.2023.1302072/full#supplementary-material
Arim, A. L., Quina, M. J., and Gando-Ferreira, L. M. (2018). Insights into the sorption mechanisms of Cr(III) by chemically modified pine bark. Chem. Eng. Technol. 41 (7), 1378–1389. doi:10.1002/ceat.201800034
Bensalah, J., Idrissi, A., Faydy, M. E., Doumane, G., Staoui, A., Hsissou, R., et al. (2023). Investigation of the cationic resin as a potential adsorbent to remove MR and CV dyes: kinetic, equilibrium isotherms studies and DFT calculations. J. Mol. Struct. 1278, 134849. doi:10.1016/j.molstruc.2022.134849
Bentouami, A., and Oulai, M. S. (2006). Cadmium removal from aqueous solutions by hydroxy-8 quinoleine intercalated bentonite. J. Colloid Interface Sci. 293 (2), 270–277. doi:10.1016/j.jcis.2005.06.040
Cao, Y., Fang, D., Wang, C., Xu, W., Luo, Z., et al. (2015). Novel aligned sodium vanadate nanowire arrays for high-performance lithium-ion battery electrodes. Rsc Adv. 5 (53), 42955–42960. doi:10.1039/c5ra01102g
Dao, P. T., Tran, N. Y. T., Tran, Q. N., Bach, G. L., and Lam, T. V. (2022). Kinetics of pilot-scale essential oil extraction from pomelo (Citrus maxima) peels: Comparison between linear and nonlinear models. Alexandria Eng. J. 61 (3), 2564–2572. doi:10.1016/j.aej.2021.07.002
el Amri, A., Kadiri, L., Hsissou, R., Lebkiri, A., Wardighi, Z., Rifi, E. H., et al. (2023). Investigation of Typha Latifolia (TL) as potential biosorbent for removal of the methyl orange anionic dye in the aqueous solution. Kinetic and DFT approaches. J. Mol. Struct. 1272, 134098. doi:10.1016/j.molstruc.2022.134098
Elfeghe, S., Anwar, S., and Zhang, Y. H. (2022). Adsorption and removal studies of cadmium ion onto sulphonic/phosphonic acid functionalization resins. Can. J. Chem. Eng. 100 (10), 3006–3014. doi:10.1002/cjce.24400
el Gaayda, J., Rachid, Y., Titchou, F. E., Barra, I., Hsini, A., Yap, P. S., et al. (2023). Optimizing removal of chromium (VI) ions from water by coagulation process using central composite design: effectiveness of grape seed as a green coagulant. Sep. Purif. Technol. 307, 122805. doi:10.1016/j.seppur.2022.122805
Elkady, M. F., Abu-Saied, M. A., Rahman, A. M. A., Soliman, E., Elzatahry, A., Elsayed Yossef, M., et al. (2011). Nano-sulphonated poly (glycidyl methacrylate) cations exchanger for cadmium ions removal: effects of operating parameters. Desalination 279 (1-3), 152–162. doi:10.1016/j.desal.2011.06.002
Fang, D., Xu, X., Bao, R., Wan, R., Yang, F., Yi, J., et al. (2021). Layered Na5V12O32 nanowires with enhanced properties of removing Pb2+ in aqueous solution by chemical reaction. J. Environ. Chem. Eng. 9 (2), 104765. doi:10.1016/j.jece.2020.104765
Harbi, S., Guesmi, F., Tabassi, D., Hannachi, C., and Hamrouni, B. (2016). Application of response surface methodology and artificial neural network: modeling and optimization of Cr(VI) adsorption process using Dowex 1X8 anion exchange resin. Water Sci. Technol. 73 (10), 2402–2412. doi:10.2166/wst.2016.091
Ishfaq, A., Ilyas, S., Yaseen, A., and Farhan, M. (2019). Hydrometallurgical valorization of chromium, iron, and zinc from an electroplating effluent. Sep. Purif. Technol. 209, 964–971. doi:10.1016/j.seppur.2018.09.050
Jebli, A., Amri, A. E., Hsissou, R., Lebkiri, A., Zarrik, B., Bouhassane, F. Z., et al. (2023). Synthesis of a chitosan@hydroxyapatite composite hybrid using a new approach for high-performance removal of crystal violet dye in aqueous solution, equilibrium isotherms and process optimization. J. Taiwan Inst. Chem. Eng. 149, 105006. doi:10.1016/j.jtice.2023.105006
Kadiri, L., Ouass, A., Hsissou, R., Safi, Z., Wazzan, N., Essaadaoui, Y., et al. (2021). Adsorption properties of coriander seeds: spectroscopic kinetic thermodynamic and computational approaches. J. Mol. Liq. 343, 116971. doi:10.1016/j.molliq.2021.116971
Kaki, E., Gogsu, N., Altindal, A., Salih, B., and Bekaroğlu, Ö. (2019). Synthesis, characterization and VOCs adsorption kinetics of diethylstilbestrol-substituted metallophthalocyanines. J. Porphyr. Phthalocyanines 23 (1-2), 166–174. doi:10.1142/s1088424619500196
Khamizov, R. K. A. (2020). A pseudo-second order kinetic equation for sorption processes. Russ. J. Phys. Chem. A 94 (1), 171–176. doi:10.1134/s0036024420010148
Kocaoba, S., Cetin, G., and Akcin, G. (2022). Chromium removal from tannery wastewaters with a strong cation exchange resin and species analysis of chromium by MINEQL+. Sci. Rep. 12 (1), 9618. doi:10.1038/s41598-022-14423-3
Lebkiri, I., Abbou, B., Hsissou, R., Safi, Z., Sadiku, M., Berisha, A., et al. (2023). Investigation of the anionic polyacrylamide as a potential adsorbent of crystal violet dye from aqueous solution: equilibrium, kinetic, thermodynamic, DFT, MC and MD approaches. J. Mol. Liq. 372, 121220. doi:10.1016/j.molliq.2023.121220
Lebkiri, I., Abbou, B., Kadiri, L., Ouass, A., Essaadaoui, Y., Ouaddari, H., et al. (2022). Polyacrylamide hydrogel an effective adsorbent for the removal of heavy metal from aqueous solution: isotherm, kinetic, and thermodynamic studies. Russ. J. Phys. Chem. A 96 (7), 1484–1492. doi:10.1134/s0036024422070159
Lingamdinne, L. P., Godlaveeti, S. K., Angaru, G. K. R., Chang, Y. Y., Nagireddy, R. R., Somala, A. R., et al. (2022). Highly efficient surface sequestration of Pb2+ and Cr3+ from water using a Mn3O4 anchored reduced graphene oxide: selective removal of Pb2+ from real water. Chemosphere 299, 134457. doi:10.1016/j.chemosphere.2022.134457
Liu, C., Wang, P., Liu, X. K., Yi, X., Liu, D., and Zhou, Z. (2019b). Ultrafast removal of Cadmium(II) by green cyclodextrin metal-organic-framework-based nanoporous carbon: adsorption mechanism and application. Chemistry-an Asian J. 14 (2), 261–268. doi:10.1002/asia.201801431
Liu, C., Wu, T., Hsu, P. C., Xie, J., Zhao, J., Liu, K., et al. (2019a). Direct/alternating current electrochemical method for removing and recovering heavy metal from water using graphene oxide electrode. ACS Nano 13 (6), 6431–6437. doi:10.1021/acsnano.8b09301
Makarenko, N. V., Arefieva, O. D., Kovekhova, A. V., and Zemnukhova, L. A. (2019). Removal of Cr3+ ions by phytic acid derivatives from rice bran. Bioresources 14 (2), 4866–4872. doi:10.15376/biores.14.2.4866-4872
Priastomo, Y., Setiawan, H. R., Mutmainah, , Kurniawan, Y. S., and Ohto, K. (2020). Simultaneous removal of lead(II), chromium(III), and copper(II) heavy metal ions through an adsorption process using C-phenylcalix 4 pyrogallolarene material. J. Environ. Chem. Eng. 8 (4), 103971. doi:10.1016/j.jece.2020.103971
Rengaraj, S., Yeon, K. H., Kang, S. Y., Lee, J. U., Kim, K. W., and Moon, S. H. (2002). Studies on adsorptive removal of Co(II), Cr(III) and Ni(II) by IRN77 cation-exchange resin. J. Hazard. Mater. 92 (2), 185–198. doi:10.1016/s0304-3894(02)00018-3
Shahryari, T., Mostafavi, A., Afzali, D., and Rahmati, M. (2019). Enhancing cadmium removal by low-cost nanocomposite adsorbents from aqueous solutions; a continuous system. Compos. Part B-Engineering 173, 106963. doi:10.1016/j.compositesb.2019.106963
Song, C. Y., Jiao, Y. Y., Tian, Z. H., Qin, Q., Qin, S., Zhang, J., et al. (2022). Fabrication of hollow-fiber nanofiltration membrane with negative-positive dual-charged separation layer to remove low concentration CuSO4. Sep. Purif. Technol. 296, 121352. doi:10.1016/j.seppur.2022.121352
Vatanpour, V., Mansourpanah, Y., Khadem, S. S. M., Zinadini, S., Dizge, N., Ganjali, M. R., et al. (2021). Nanostructured polyethersulfone nanocomposite membranes for dual protein and dye separation: lower antifouling with lanthanum (III) vanadate nanosheets as a novel nanofiller. Polym. Test. 94, 107040. doi:10.1016/j.polymertesting.2020.107040
Wang, M., Ye, H., Zheng, X., Chen, S., Xing, H., Tao, X., et al. (2023). Adsorption behaviors and mechanisms of simultaneous cadmium and fluoride removal on waste bovine bone from aqueous solution. J. Environ. Chem. Eng. 11 (1), 109035. doi:10.1016/j.jece.2022.109035
Wen, Y., Xie, Z., Xue, S., Long, J., Shi, W., and Liu, Y. (2022). Preparation of novel polymethacryloyl hydrazone modified sodium alginate porous adsorbent with good stability and selective adsorption capacity towards metal ions. Sep. Purif. Technol. 303, 122184. doi:10.1016/j.seppur.2022.122184
Wu, R. P. (2019). Removal of heavy metal ions from industrial wastewater based on chemical precipitation method. Ekoloji 28 (107), 2443–2452.
Zhang, J., Xue, C. H., Ma, H. R., Ding, Y. R., and Jia, S. T. (2020). Fabrication of PAN electrospun nanofibers modified by tannin for effective removal of trace Cr(III) in organic complex from wastewater. Polymers 12 (1), 210. doi:10.3390/polym12010210
Keywords: Na5V12O32, nanowire array, adsorption, heavy metal ions, kinetic study
Citation: Peng D, Fan X and Fang D (2023) High-efficiency adsorption of Cd2+ and Cr3+ by sodium vanadate nanowire arrays. Front. Mater. 10:1302072. doi: 10.3389/fmats.2023.1302072
Received: 26 September 2023; Accepted: 13 November 2023;
Published: 13 December 2023.
Edited by:
Osman Ahmed Zelekew, Adama Science and Technology University, EthiopiaReviewed by:
Alemayehu Dubale Duma, Ethiopian Biotechnology Institute (EBTi), EthiopiaCopyright © 2023 Peng, Fan and Fang. This is an open-access article distributed under the terms of the Creative Commons Attribution License (CC BY). The use, distribution or reproduction in other forums is permitted, provided the original author(s) and the copyright owner(s) are credited and that the original publication in this journal is cited, in accordance with accepted academic practice. No use, distribution or reproduction is permitted which does not comply with these terms.
*Correspondence: Xin Fan, eGZhbkBnbHV0LmVkdS5jbg==; Dong Fang, ZmFuZ2RvbmdAa211c3QuZWR1LmNu
Disclaimer: All claims expressed in this article are solely those of the authors and do not necessarily represent those of their affiliated organizations, or those of the publisher, the editors and the reviewers. Any product that may be evaluated in this article or claim that may be made by its manufacturer is not guaranteed or endorsed by the publisher.
Research integrity at Frontiers
Learn more about the work of our research integrity team to safeguard the quality of each article we publish.