- 1Department of Biology, College of Science, Imam Mohammad Ibn Saud Islamic University (IMSIU), Riyadh, Saudi Arabia
- 2Department of Zoology, College of Science, King Saud University, Riyadh, Saudi Arabia
- 3Department of Biology, College of Science, Princess Nourah bint Abdulrahman University, Riyadh, Saudi Arabia
- 4Department of Chemistry, College of Science, Imam Mohammad Ibn Saud Islamic University (IMSIU), Riyadh, Saudi Arabia
- 5Department of Production Technology, Faculty of Technology and Education, Helwan University, Cairo, Egypt
Introduction: Increased mortality owing to breast and lung cancer is an urgent global medical issue. Nanotherapy is one of the most widely used and recent procedures for combating cancer. Therefore, this study aimed to assess the beneficial effects of multi-walled carbon nanotubes (MWCNTs) to precisely target breast (MCF-7) and lung cancer (A549) cell lines. Multi-walled carbon nanomaterials are considered as a typical biocompatible nanomaterial that can be used in drug delivery without causing side effects. On the contrary, the metallic nanoparticles, even that of noble metals, are reported to have side effects that are still unavoidable.
Methods: The MWCNTs were purified and stabilized using Arabic gum in an aqueous solution under ultrasonic radiation. Scanning and transmission electron microscopy (SEM and TEM, respectively) were employed to characterize the morphology of the MWCNTs, and elemental analysis was performed using SEM to determine their chemical composition.
Results: The investigated MWCNTs had an average diameter of 10–12 nm and were mainly composed of 99.4% carbon and other trace elements. The Arabic gum emulsifying agent was found a powerful stabilizing agent for the investigated MWCNTs in aqueous solutions. The cytotoxic effect of the MWCNT/ Arabic gum colloidal solution on MCF-7 and A549 was evaluated by 3-(4,5- dimethylthiazol-2-yl)-2,5-diphenyltetrazolium bromide and lactate dehydrogenase assays, and the expression of apoptosis-related genes was assessed using quantitative real-time polymerase chain reaction and a human apoptosis signaling antibody array. This analysis aimed to clarify additional pathways involved, including those mediated by interleukin-1, tumor necrosis factor-α, and ceramide signaling pathways enrichments. Concerning the inhibition of cell growth, the efficacy of MWCNT/Arabic gum IC50 of MCF-7 (48.23 μg/mL) was higher than IC50 of A549 (100.1 μg/mL). MWCNT/Arabic gum enhanced the mRNA expression of apoptosis-associated genes encoding P53, Bax, and Caspases (9, 8, and 3). Upregulation of the apoptotic signaling pathway in the MCF-7 cell lysates was observed before and after exposure to the MWCNT/Arabic gum colloidal solution.
Conclusion: The physicochemical properties of MWCNT/Arabic gum exhibited remarkable apoptotic activity in breast and lung cancer cell lines. However, further in vivo investigations are recommended to clarify the other molecular aspects related to the use of MWCNT/Arabic gum.
1 Introduction
Cancer is a fatal disease and considered a critical global medical health challenge (Kirtonia et al., 2021). In 2020, approximately 19.3 million new cases of cancer were diagnosed worldwide, with over 10.0 million cancer deaths. With an anticipated 2.3 million new cases, female breast cancer has exceeded lung cancer as the most often diagnosed disease, although lung cancer remains the leading cause of cancer-related deaths, with an estimated 1.8 million fatalities (Sung et al., 2021). Various strategies and treatments, such as chemotherapy, surgery, and radiation, are currently being used to treat cancer. However, these choices have serious adverse effects (Schirrmacher, 2019). Therefore, lowering harmful side effects is desirable to enhance the performance of drugs and improve the quality of life of cancer patients.
Nanotechnology represents a recent substitute for the layout and composition of nanomaterials (Sani et al., 2023) that can be applied as advanced tool for cancer diagnosis (Pourmadadi et al., 2023), immunotherapy of cancer cells (Chen, 2023) and is presently a very promising tool to treat different types of tumors (Casais-Molina, 2018). Nanoparticles are small and have large surface areas; hence, considerable amounts of drugs can be loaded onto their surfaces to reach the intended targets. Drug-conjugated AuNPs significantly affect diseased cells. These effects are attributed to the fact that NP-drug conjugates can disrupt cell membrane permeability and eventually provoke cytolysis (Habib and Akbar, 2021).
Some nanomaterials, including nanotubes, exhibit various physical, chemical, and biocompatibility properties, which has led to recent scientific evidence that qualifies for the possibility of using carbon nanomaterials in the remediation and release of eclectic drugs, as well as cofactors in the diagnosis and limitation of tumors (Chen et al., 2015).
The native forms of carbon nanomaterials have low solubility in biological media. This insolubility is related to the exaggerated accumulation of classical drugs and high stabilization of oxidative enzymes, rendering the elimination process difficult (Ke, 2007). Thus, the enhanced activity of carbon nanomaterials promotes their solubility, lowers an organism’s toxicity, and enhances the bioadministration of drugs (Lucafo et al., 2013). In addition, a study (Cirillo et al., 2019) reported that multi-walled carbon nanotube (MWCNT) hybrid materials have comprehensive physical and chemical characteristics that enable them to synergize drug efficacy in lung cancer cells while reducing toxicity to healthy cells. Nanomaterials have attracted attention in nanomedical applications as useful tools for cancer treatment and for optimizing therapeutic results (Kumar et al., 2023) Carbon nanotubes (CNTs) have been extensively studied to explore their potential implementation in cancer remediation because of their prominent thermal, electrical, optical, and chemical characteristics (Dizaji BF et al., 2020).
CNTs, with their unique features, are considered a suitable option for avoiding the undesirable effects correlated with cancer using conventional methods. Therefore, this study explored the impact of CNTs on breast cancer cells (MCF-7) and lung cancer cells (A549).
CNTs, a promising category of nanomaterials, have recently emerged and possess unique properties such as a large surface-area-to-volume ratio owing to the ability of graphene structures to capture small molecules on their surfaces. The graphene structure is suitable for various applications in electronic devices, drug delivery systems, and biosensors (Zheng et al., 2006; Daoush and Imae., 2015). Furthermore, CNTs have been successfully utilized in different medical and pharmaceutical fields because of their high surface-area-to-volume ratio, as they can absorb and bond with multiple diagnostic and therapeutic agents, such as vaccines, antibodies, and drugs (Akbar et al., 2022).
An important step during the fabrication of nanomaterials is to stabilize the particles and prevent their agglomeration in solution. Notably, the stabilization of nanoparticles through surface treatment is a key parameter in ensuring the activity of nanoparticles in the environment. In this regard, the benefits of nanotubes can be maximized by proper dispersion in a solution (Liu et al., 2005; Batool et al., 2021). The electroosmotic- or peristaltic-controlled flow of CNTs through ionic solutions has been found to be an alternative method for promoting the diffusion of CNTs in solution (Akram et al., 2022; Abo-Dahab, 2023). This method of enhanced diffusion is beneficial for controlled therapeutic trials concerning the delivery of drugs to targeted cancer cells (Butt et al., 2023). As integrated molecules, CNTs have high chemical stability and low binding energies because of the covalent bonds between the carbon atoms connected to the sp2 hybrid (Tans et al., 1998; Durgun et al., 2003). Therefore, it is necessary to modify the graphene surface structure of CNTs to create the desired functional groups that can link with metal clusters. Previous reports have revealed that introducing specific functional groups onto the surface of CNTs can help address this issue. Many studies have focused on the surface functionalization of CNTs (e.g., by HNO3, H2SO4/HNO3, and HCl/HNO3), which has been shown to improve their dispersion in solution (Chen et al., 2005; Daoush, 2012). In addition, the binding of organic molecules to the surface of CNTs renders them more soluble, thereby extending their applications in different fields (White, 2005; Daoush and Imae, 2015). In this respect, dendrimers (a type of polymer) have been found to be suitable for attachment to CNT surfaces (Garcia-Martinez et al., 2003).
Conversely, previous studies have focused on the stabilization of nanoparticles against agglomerations and the reduction of deposition in solutions through the utilization of electrostatic stabilizing agents or emulsifiers. Electrolytes composed of organic polymers, including polyaspartate and polystyrene sulfonate, polymethacrylic acid, butyl methacrylate, carboxymethyl cellulose, and potato starch and garlic extract have been demonstrated to be effective surface modifiers that resist aggregation in solution and control the size of nanoparticles and the distribution of final dispersion (Tedeschi, 2007). In another study, cactus leaf extract contained carboxymethyl (-O-CH2-COO-) and sulfoxy (-OCH2-CHOH-CH2-O-CH2-CH2SO3-) functional groups, which can interact with the surface of the nanoparticles and enhance their stabilization in solution (Ijaz et al., 2017).
Arabic gum is a dried gummy exudate obtained from the stems of Acacia senegal (colorless) and Acacia seyal (reddish) of the Acacia Family Leguminosae. Its molecular weight is approximately 240,000–850,000 and comprises a high molecular weight glycoprotein containing 90% carbohydrate (D-galactose, L-rhamnose, L-arabinose, and D-glucuronic acid) and protein content, as well as a lower molecular weight heterogeneous polysaccharide. Arabic gum has a waddle-blossom structure composed of several polysaccharide block units linked to a common polypeptide. It also contains the calcium, magnesium, and potassium salts of Arabic acid, a polysaccharide acid. It is predominantly a carbohydrate with approximately 2% protein-rich in hydroxypropyl, prolyl, and seryl residues that are responsible for its emulsifying activity (Johnson, 2005).
Carbon nanomaterials are known to have limited solubility in the body fluids, and their hydrophilic properties can be considerably enhanced by employing Gum Arabic that is consisted of high molecular weight polymers of polysaccharides (90%) and glycoprotein (10%) (Song, 2011). Therefore, Arabic gum is considered as a powerful stabilizing and dispersing agent that provides a biocompatible shell for nanostructures. The current study aimed to evaluate the effect of MWCNTs dispersed in an aqueous solution of Arabic gum (procured from local source) on breast and lung cancer cell lines. SEM, EDS, TEM and XRD were used to investigate the prepared and stabilized MWCNTs. The cytotoxic effects of MWCNTs on MCF-7 and A549 cell lines were evaluated by the aid of 3-(4,5-dimethylthiazol-2-yl)-2,5-diphenyltetrazolium bromide and lactate dehydrogenase assays.
2 Materials and methods
2.1 Preparation and investigation of MWCNTs/Arabic gum colloidal solution
Multiwalled carbon nanotubes with surface area of ∼200 m2/g were purchased from Iljin Nanotech Co., Ltd. (Seoul, South Korea). Arabian gum (Acacia senegal) tree exudates were collected elsewhere in Saudi Arabia. The raw powder of the MWCNTs was subjected to a purification process involving heat treatments at 450°C for 30 min to evaporate and burn any organic binder that may have contaminated its surface. Additionally, it was washed with acetone several times to remove any soluble organic impurities. The heat-treated MWCNTs were then chemically treated with 30 vol% HCl solution for 15 min to dissolve any metallic impurities that may have contaminated the nanotubes during manufacturing. The obtained MWNTs were washed extensively with distilled water to remove any acid or soluble inorganic impurities from their surfaces. An aqueous solution of the stabilizing agent of the investigated MWCNTs was prepared by boiling 1 g of previously ground Arabic gum in 100 mL of distilled water with continuous magnetic stirring until complete dissolution. The resultant solution was cooled and stored as a stock solution at room temperature (24⸰C). A calculated amount of 100 mg of MWCNTs was ultrasonicated for 15 min with freshly prepared Arabic gum stock solution, which was used to prepare 0.1 wt% MWCNTs/Arabic gum colloidal solution. The obtained colloidal solution was stored for further characterization and investigation. Figure 1 illustrates a schematic flowchart of the step-by-step synthesis process from the preparation of the Arabic gum colloidal solution to the final product of the MWCNT/Arabic gum colloidal solution. After the purification process, carbon, sulfur, hydrogen, and nitrogen elemental analyses of the investigated MWCNT/Arabic gum colloidal solution were performed (VarioEL III CHNS Elementar GmbH).
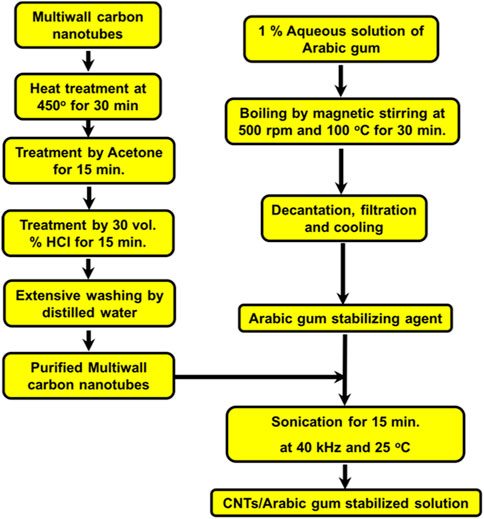
FIGURE 1. Purification and stabilization of multi-wall carbon nanotubes in Arabic gum colloidal solution.
In addition, the particle shape and size were investigated using field-emission scanning electron microscopy (FE-SEM) (Philips XL 30SFEG). Furthermore, the surface morphology of the prepared nanoparticles was investigated using the high-resolution transmission electron microscope of the model (HITACHI H-7000).
2.2 In vitro studies
2.2.1 Cytotoxic 3-(4,5-dimethylthiazol-2-yl)-2,5-diphenyltetrazolium bromide (MTT) assay
MTT cell viability assay was utilized in the current investigation to assess the antiproliferative effect of MWCNT/Arabic gum colloidal solution. Briefly, MCF-7 and A549 cells were cultured in DMEM (Gibco, ThermoFisher Scientific, CA, United States) each containing 10% v/v FBS. Cells were seeded at a 5 × 104 per 1 mL density in 24-well cell culture plates and incubated at 37°C and 5% CO2 for 24 h. Cells were then treated for 48 h with the MWCNT/Arabic gum colloidal solution under investigation, and after the incubation period, 100 μL of MTT reagent (Invitrogen Life Technologies, CA, United States) (5 mg/mL in PBS) was added to all wells and further incubated at 37°C for 2 h. Subsequently, 1 mL of isopropanol-HCl was added to dissolve the crystalline formazan, and reduced MTT formation was measured at a wavelength of 540 nm using a microplate reader (Thermo Fisher Scientific, Waltham, MA, United States). The wells with untreated cells were used as controls. The dose-response curve was used to calculate the 0.5 IC50 (concentration needed to inhibit cell growth by 50%). Cell viability was calculated using the following equation:
2.2.2 Measurement of released lactate dehydrogenase (LDH)
In order to confirm the cytotoxic action of MWCNT/Arabic gum colloidal solution, cell viability and membrane integrity were determined using an LDH assay kit (Cayman CHEMICAL, Item No. 601170, MI, United States). The procedure was performed as follows: MCF-7 and A549 cells were seeded in 24-well (5 × 104 per 1 mL) followed by 24 h incubation at 37°C in 5% CO2 chamber. The cells were then exposed to IC50 of the MWCNT/Arabic gum colloidal solution and incubated for 48 h. Later, 100 μL from the cell supernatant was transferred to a 96-well assay plate and 100 μL of LDH Reaction Solution (prepared #3, see Cayman) was added to each well. The plate was placed on a shaker for 30 min in an incubator at 37°C. The absorbance was measured at 490 nm. Finally, the cytotoxicity of each fraction was calculated as follows:
2.2.3 Quantitative real-time polymerase chain reaction (qRT-PCR)
To clarify the cell death mode mediated by MWCNT/Arabic gum colloidal solution, the expression levels of proapoptotic genes (Bax, Caspases-3, 8 and 9) were explored. In brief, MCF-7 and A549 cells (5 × 104 cells/well) were cultured in 25 cm flask culture plates and treated with the prepared MWCNT/Arabic gum colloidal solution at 0.5 IC50 for 24 h. After incubation, the total RNA was prepared using QIAzol Lysis Reagent (Qiagen, Cat. No. 79306, United States), and cDNA was synthesized with oligo dT primers using the Superscript II Reverse Transcriptase cDNA Synthesis Kit (Applied Biosystems, Thermo Fisher Scientific, Lithuania) according to the manufacturer’s instructions. Polymerase chain reaction (PCR) was performed using a Rotor-Gene machine (PrimeQ, China) with gene specific primers as listed in Table 1. Evaluation of mRNA expression using use 2−ΔΔCt with GAPDH as the internal reference wad estimated by the following equation;
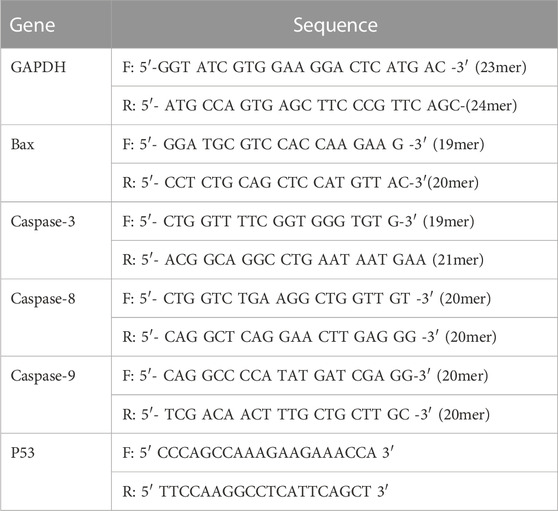
TABLE 1. Primer sequence (forward and reverse) targeting GAPDH, P53 mRNA, Bax, and Caspases 3, 8, 9.
2.2.4 Human apoptosis signaling array
To further understanding the effectiveness of MWCNT/Arabic gum colloidal solution, the upregulation of apoptosis signaling markers was studied on the protein levels. Treated cell lysates were prepared according to the manufacturer’s instructions and analyzed using a Human Apoptosis Signaling Antibody Array (RayBiotech, Peachtree Corners, GA, United States).
2.2.5 Statistical analysis of data
The results were expressed as means ± SD of the three replicates. Student’s t-test was used to compare data between groups (p- < 0.05).
3 Results
3.1 Characterizations of MWCNTs/Arabic gum colloidal solution
The produced Arabic gum and the fabricated MWCNT/Arabic gum aquous colloidal solution are shown in Figures 2A, B. The results indicate that the prepared MWCNT/Arabic gum colloidal solution was completely homogeneous and dispersed for a prolonged period owing to the continuous use of the stabilized MWCNTs for subsequent investigations and experimental procedures. The Arabic gum solution composed of 1 g of Arabic gum dissolved in 100 mL of distilled water was observed as a suitable stabilizing agent for purified MWCNTs in an aqueous solution.
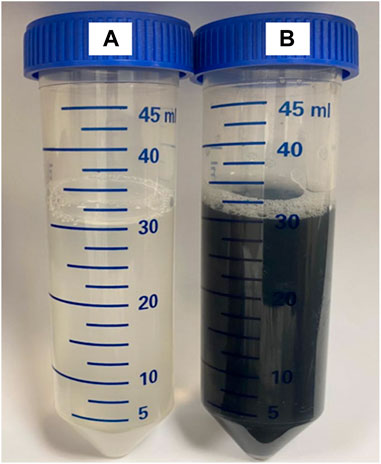
FIGURE 2. The produced Arabic gum and the fabricated stabilized MWCNTs/Arabic gum aquous colloidal solution. (A) Arabic gum stabilizing agent, (B) the prepared colloidal solution of the investigated multi-wall carbon nanotubes dispersed in 1 wt% Arabic gum stabilizing agent.
The chemical composition of the MWCNTs was investigated to analyze the elements content of the MWCNTs under investigation. Table 2 lists the typical chemical compositions of the MWCNTs investigated after purification. The results showed that the carbon nanotube powder was highly pure and mainly composed of approximately 99.4 wt%. In addition, the fabrication process of the nanotubes resulted in the presence of carbon and trace elements such as nitrogen, hydrogen, and sulfur, as indicated by their respective percentages.
High-resolution SEM and TEM images of the untreated MWCNTs were shown in Figures 3A, B. The untreated MWCNTs had the texture of agglomerated filaments with capped ending. It was also abserved that the MWCNTs were composed of multiwalled graphitic carbon structures containing hollow core in the center. In addition, high-resolution TEM images of the stabilized MWCNTs in solution were presented in Figures 3C, D. It was revealed that the stabilized MWCNTs in solutions had core-multiwalled graphitic structure. The SEM investigation showed that the MWNTs have an outer diameter of 36 nm approximately, however the TEM investigation revealed a median outer diameter of ∼ 10–15 nm which is a more accurate estimation due to higher resolution of the transmission electron beam than the scanning beam.
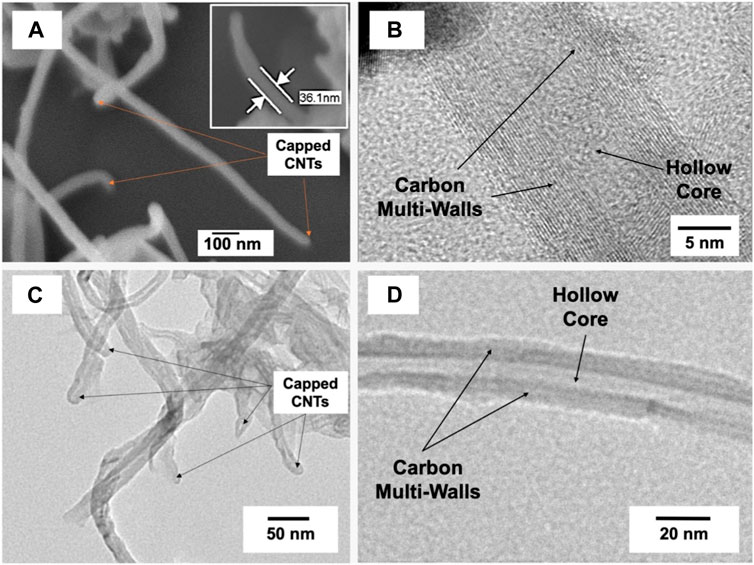
FIGURE 3. (A) FESEM electron micrograph, (B–D) HRTEM electron micrographs with different magnifications of the investigated multi-walls carbon nanotubes.
3.2 In vitro study
3.2.1 MTT proliferation assay
Figure 4A shows the cytotoxic effects of MWCNT/Arabic gum colloidal solution on MCF-7 and A549 cells. Our findings showed that the investigated MWCNT/Arabic gum colloidal solution inhibited cell growth in a dose-dependent manner and were more effective against MCF-7 cells than against A549 cells, with IC50 values of 48.23 and 100.1 μg/mL, respectively.
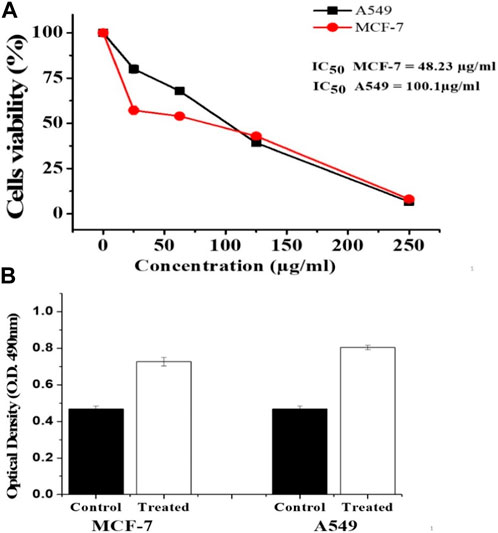
FIGURE 4. Effect of the investigated MWCNT/Arabic gum colloidal solution on MCF 7 and A549 using MTT and LDH assays. (A) MTT formazan absorbance was estimated at a wavelength of 540 nm in an ELISA reader. (B) MCF-7 and A549 cells were treated with MWCNT/Arabic gum colloidal solution for 48 h at 48.23 μg/mL and 100.1 μg/mL, respectively. LDH activity was determined using ELISA reader at a wavelength of 490 nm. The obtained data were represented as means ± SD (∗p < 0.05 was considered significant compared to control) of three independent experiments carried out in triplicates.
3.2.2 LDH assay
The effects of CNTs on MCF-7 and A549 cells were investigated using an LDH release assay. As shown in Figure 4B, the investigated MWCNT/Arabic gum colloidal solution showed remarkable cytotoxic activity (p ˂ 0.05) 48 h after exposure to the IC50 concentration.
3.2.3 RT-PCR (real-time polymerase chain reaction)
Apoptosis-related genes were significantly upregulated in the MCF-7 and A549 cells treated with MWCNT/Arabic gum colloidal solution. Fold changes were as follows: 1.42 ± 0.39, 5.34 ± 0.22 for P53; 1.69 ± 0.73, 2.50 ± 0.69 for BAX; 1.63 ± 0.11, 1.57 ± 0.32 for caspase 3; 2.58 ± 0.76, 1.53 ± 0.09 for caspase 8; and 1.66 ± 0.34, 1.79 ± 0.30 for caspase 9 in MCF-7 and A549 cells, respectively. For the untreated control groups of MCF-7 and A549 cells, the mean ± standard deviations were 1.0 ± 0.05 and 1.0 ± 0.03, respectively. MWCNT/Arabic gum colloidal solution positively affected apoptotic gene expression in MCF-7 and A549 cells, but to different degrees. The expression of P53 gene in MCF-7 cells showed statistically significant differences (p ≤ 0.05), while in A549 cells, the expression was highly significant (p < 0.001). The BAX genes in both MCF-7 and A549 exhibited statistically significant expression (p ≤ 0.05). Additionally, the expression of caspase 8 in MCF-7 was highly significant (p ≤ 0.005), while in A549 cells, a slight increment in expression was observed. Moreover, in MCF-7 cells there was a significant increase in the expression of caspases 3 and 9. However, in A549 cells, these caspases showed only a slight elevation, as shown in Figures 5, 6.
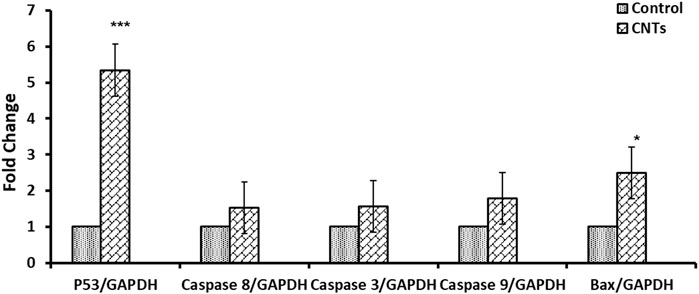
FIGURE 5. Effect of the investigated MWCNT/Arabic gum colloidal solution on the expression of apoptosis-associated genes (mRNA expression). Fold change of the genes encoding Bax, p53, and caspases 3, 8, and 9 in MCF-7 cancer cells exposed to IC50 concentration (48.23 μg/mL) for 24 h compared to (DMSO/control). The obtained data were represented as means ± SD (∗p < 0.05, ∗∗p < 0.005, and ∗∗∗p < 0.0005 compared to control) of three independent experiments conducted in triplicates.
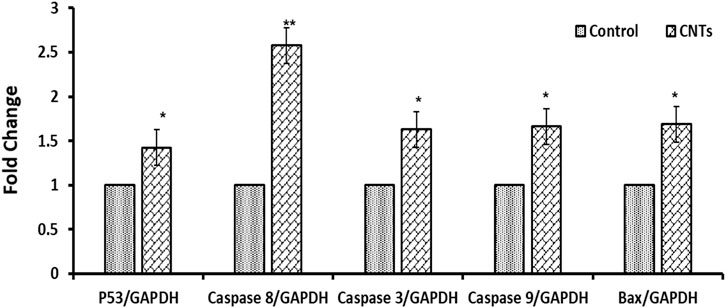
FIGURE 6. Effect of the investigated MWCNT/Arabic gum colloidal solution on expression of apoptosis-associated genes (mRNA expression). Fold change of the genes encoding Bax, p53, and caspases 3, 8, and 9 in A549 cancer cells exposed to a concentration of 100.1 μg/mL (IC50) for 24 h. The obtained data were represented as means ± SD (∗p < 0.05, ∗∗p < 0.005, and ∗∗∗p < 0.0005 compared to control) of three experiments independently conducted in triplicates.
3.2.4 Human apoptosis signaling array
The total protein lysate of MCF-7 was treated with 48.23 μg/mL MWCNT/Arabic gum colloidal solution for 24 h compared to the control, as illustrated in Figure 7. The chemiluminescence was then measured using the ChemiDoc system and quantified by ImageJ software (Gomez-Perez et al, 2020) Figures 7A, B. The image and semi-quantification confirmed the mRNA expression for p53 and Caspase 3, shown in Figure 5. In addition, the analysis identified an upregulation in 17 antigen-specific antibodies, which represent proteins expressed in response to cell stress and apoptosis signaling pathways. The list of proteins elevated in Figure 7A and uploaded in Gene enrichment set Analysis (GESA) were used to identify all the possible pathways, including interleukin-1 (IL-1), Tumor necrosis factor-α (TNF-α), and ceramide signaling pathways, that could be activated by MWCNT/Arabic gum colloidal solution, as presented in Table 3 and Figure 7C (Mootha et al., 2003; Subramanian et al., 2005).
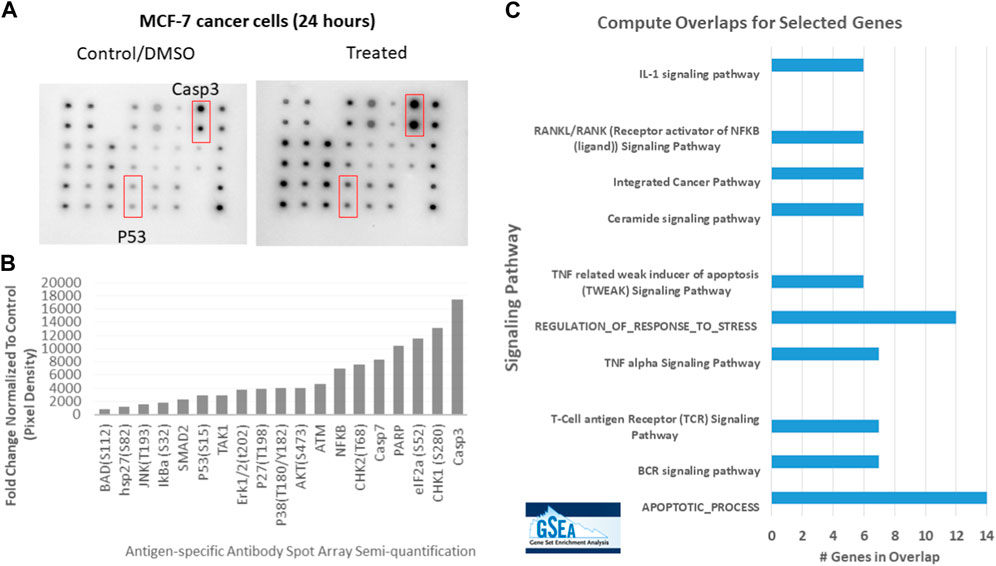
FIGURE 7. Upregulation of apoptosis signaling pathway in MCF-7 cell lysates treated with MWCNT/Arabic gum colloidal solution. (A) An apoptosis signaling pathways array of human samples was used to detect the signaling pathways in MCF-7 cells. The control group consisted of MCF-7 cells treated with DMSO (control), while the experimental group was treated with the IC50 concentration (48.23 μg/mL) for 24 h (B) Semi-quantitative detection of 19 antigen-specific antibody spot arrays in fold change after normalizing the data to the control. (C) The list of proteins elevated in (B) and uploaded in Gene enrichment set Analysis (GESA) were used to identify all the possible pathways that MWCNT/Arabic gum colloidal solution activate.
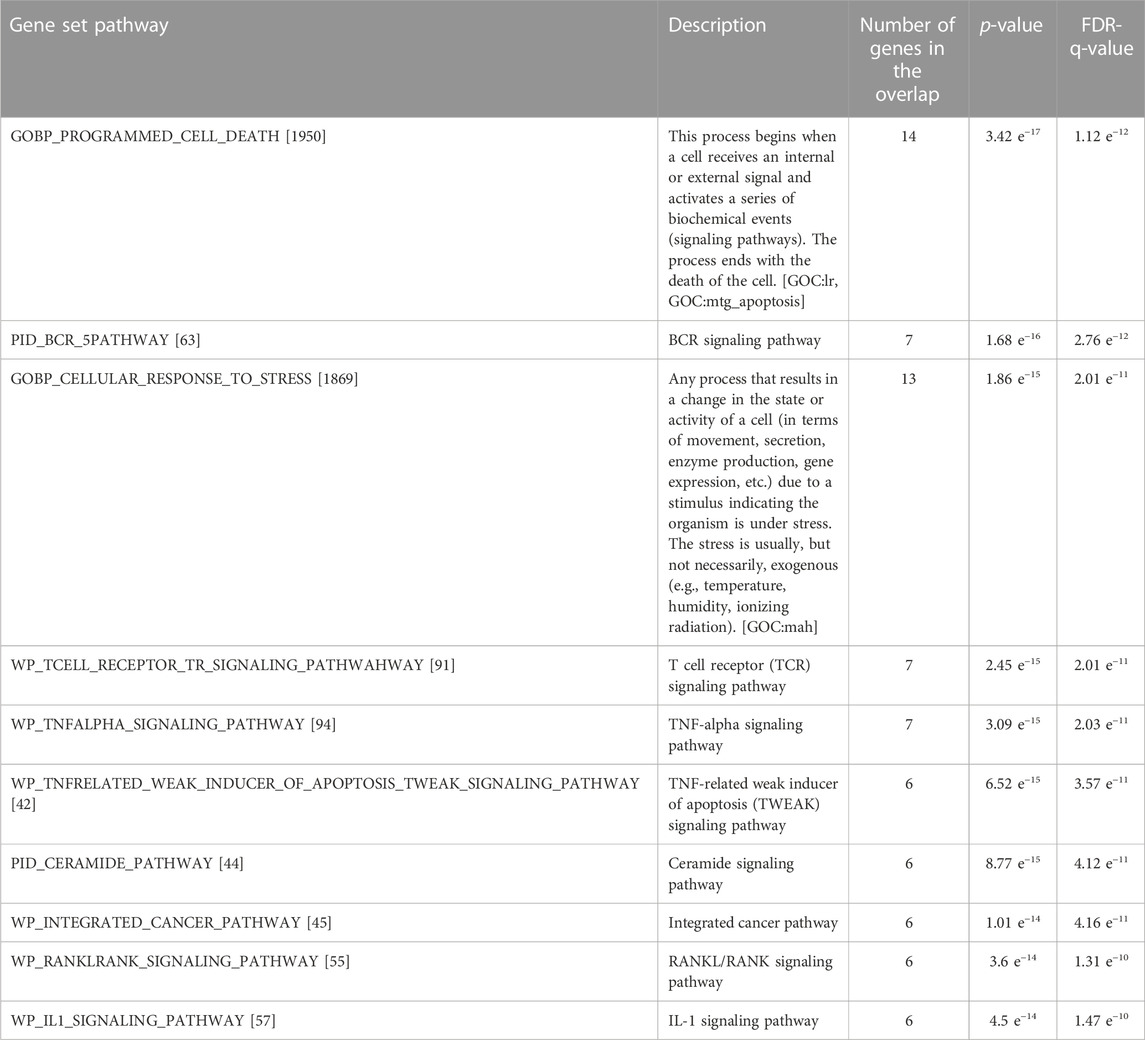
TABLE 3. Detailed list of the pathways and significance compared to gene enrichment set analysis (GESA).
4 Discussion
The physicochemical properties of nanoparticles, including their size and surface area, greatly affect their applications (Mahmoudi et al., 2011). Nanoparticles possess a large surface-area-to-volume ratio that determines their interactions with cellular proteins (Li and Sarah, 2009). The adsorption and binding of proteins to nanoparticles are controlled by their size, shape, surface area, and surface charge, as well as the type of nanoparticles, for example, metals, nonmetals, organic polymers, or carbon nanomaterials (Li and Sarah, 2009; Monopoli et al., 2011; Verma et al., 2018). Binding of the protein surface to nanoparticles has been the focus of several studies. Therefore, the formation of a protein surface layer with nanoparticles is an important issue. The surface protein-bound layer is either soft (weakly bound) or hard (strongly bound) (Kihara et al., 2019). The formation of soft or hard bonding layers is determined by the surface morphology, biological environment surrounding the nanoparticles, exposure time, and other physicochemical properties.
In this study, MWCNTs were dispersed by ultrasonic emulsification in an aqueous solution of Arabic gum. The MWCNTs in the aqueous solutions tended to aggregate and form clusters. However, the Arabic gum emulsifier has powerful stabilizing and emulsifying effects. Freshly prepared 1 wt% Arabic gum solution is used to stabilize 0.1 wt% MWCNTs dispersed in an aqueous solution under 40 kHz ultrasonic radiation. Many attempts (Xu, et al., 2018) have been reported in previous work to disperse MWCNTs in different suitable solvents. Dispersion methods include both chemical and physical methods. Chemical modification involves the formation of covalent bonds and the introduction of functional groups (–COOH) on the surface of MWCNTs. An oxidation process has been used to functionalize the MWCNTs under ultrasonic radiation with a mixture of sulfuric and nitric acids (Daoush, 2012). In addition, ultrasonic radiation can enhance the fission of the H-OH bonds in the water molecules forming OH and H radicals. This reaction is reversible or shifts to produce H2 and H2O2. These redox activities are beneficial in aqueous solutions where sonochemical reactions take place (Riesz et al., 1985). The hyperoxidant H2O2 is subsequently released to initiate the oxidation of the surface of the MWCNTs and introduce some functional groups to enhance the stabilization of the MWCNTs by the electrostatic attraction in the aqueous solutions (Savun-Hekimoğlu, 2020). H2O2 oxidation under neutral conditions can impact the morphologies and structures of MWCNTs by forming functional groups on their surface. (Peng, 2006). Reactive groups, including hydroxyl (−OH), carboxyl (−COOH), and carbonyl (=C=O) groups, have been developed; however, the prevalent group on MWCNTs’ surfaces is the hydroxyl group (Peng, 2006).
Physical methods used to disperse MWCNTs in solution include the addition of various dispersants, surfactants, polymers, and aromatic compounds during the synthesis process. When surfactants are added to MWCNTs dispersions, the surfactant molecules adsorb at the interface, followed by self-accumulation into supramolecular structures, which helps to retain a stable colloidal state in the MWCNT dispersion. Coulombic or hydrophobic attraction plays a key role in achieving stable colloidal systems using ionic or nonionic surfactants. There are three basic principles for dispersing fine particles in water: 1) repulsion between the particles and their zeta potentials, 2) steric hindrance of the adsorption layer, and 3) reduction of hydrophobic linkages among the dispersed particles (Xu, et al., 2018).
Arabic gum was used in the present research work as an emulsifying agent. The polysaccharide and glycoprotein macromolecules of the Arabic gum have the ability to surround the MWCNTs and prevent their agglomeration in the aqueous solutions without the need to a surface functionalization process. It was reported that the use of a surface functionalization process can break C=C bonds of the graphitic structure of the carbon nanotubes and consequently affects the basic properties of carbon nanotubes (Rashmi et al., 2011). Arabic gum as stabilizing agent can disperse the carbon nanotubes in the solution without any change in their properties. This is ascribed to the formation of an electrostatic double layer by the interaction between the free electrons in the Pz orbitals of C=C atoms in the MWCNTs and the different active groups of polysaccharide and glycoproteins constituents of the Arabic gum. This interaction greatly facilitates the suspension of MWCNTs in aqueous solutions (Rashmi et al., 2011; Kumar and Kumaresan, 2017). Figure 8 shows a schematic representation of the interaction between the polysaccharide and glycoproteins macromolecules composing the Arabic gum and the carbon nanotubes in aqueous media. The amino, carboxyl and hydroxyl groups on the surface of polysaccharide and glycoprotein macromolecules of Arabic gum form hydrogen bonds with the water molecules on one side, and with Pz electrons of C=C bonds of the graphitic structure of MWCNTs on the other side (Mzwd, et al., 2022). The preliminary trial of the current study revealed that 1 wt% aqueous solution of Arabic gum is a powerful stabilizing agent with sustaining of the Arabic gum ionization at isoelectric point of pH 4.5. The ionization of the functional groups of Arabic gum help interact with the carbon nanotubes. It was also reported that Arabic gum can form a relatively thick coating layer of high molecular weight on the surface of nanoparticles, hence classified as “soft particles” that comprise spherical cover of polymers. In addition, Arabic Gum has small surface potential that implies dominant steric or electrosteric repulsive interaction between the Arabic gum coat and the stabilized carbon nanotubes surfaces (Song, 2011).
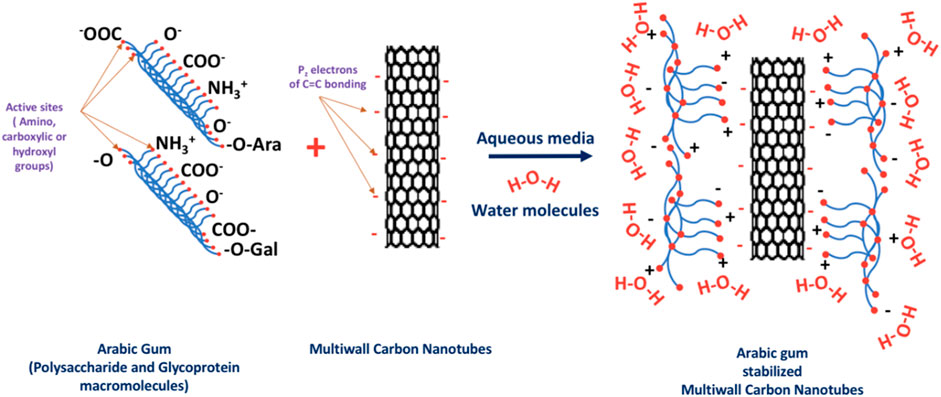
FIGURE 8. Schematic representation of the stabilization of multiwalled carbon nanotubes by the Arabic gum stabilizing agent.
A previous study revealed that cellular proteins can be adsorbed on the surface of nanoparticles based on protein electrostatistics and their capability to provoke structural deformities on the nanoparticles surface (Shemetov, et al., 2012). Therefore, electrostatic interactions (ionic interactions and hydrogen bonds) are crucial for the initial reaction, and essential amino acids, including arginine and lysine, are particularly critical for these electrostatic reactions on the surfaces of stabilized nanoparticles. This initial step is reversible, whereas the later step of adsorption depends on the hardness of the proteins. Rigid (hard) proteins, such as lysosomal proteins, are not deformed on the nanoparticle surface and thus are not expected to be adsorbed.
In contrast, soft proteins are prone to deformities and are structurally rearranged on the surface to increase the number of interaction sites on the nanoparticle surface. During these protein deformation changes, conformational changes occur, and interactions take place between the surfaces of nanoparticles surfaces and exposed proteins. This subsequent step is often considered quasi-irreversible (Norde, 2008).
Application of the cancer cells with the CNTs are a promising formularization technique that display an auspicious influence on tumor cells, particularly with better cellular internalization. This study reports the robust cytotoxic effects of CNTs on MCF-7 and A549 cells. To estimate the cytotoxic effect on cell viability, MTT and LDH tests are widely used as toxicological techniques and can be applied to cytotoxicity studies in vitro (Xia et al., 2007). Cell viability assays, such as the MTT assay, measure viable cells with active metabolism and convert MTT into a purple-colored formazan product, whereas the LDH leakage assay is based on the measurement of lactate dehydrogenase activity in the extracellular medium. As reported in the literature, another assay should be used to confirm cytotoxicity in in vitro studies to increase the reliability of the results obtained from one assay. Therefore, LDH and MTT assays were employed in the current study to confirm the results obtained (Fotakis, 2006). Both assays clearly show that the investigated MWCNTs exerted cytotoxic effects against both cell types tested in this study. These results are in line with those of our previous study that demonstrated the anticancer capability of CuNPs against both MCF-7 and LoVo tumor cell lines (Al-Zahrani et al., 2021).
A similar cytotoxic effect involving LDH release has been reported for QCuNPs previously (Mukhopadhyay, et al., 2018). Furthermore, a study (Wang et al., 2015) demonstrated that CuNPs can pass through cell membranes via diffusion or endocytosis, enhancing their activity. Previous studies have shown that CNTs can block cell proliferation and induce apoptosis in several ways. Overall, CNTs can also cause membrane deterioration, minimize the capability of cellular adherence, increase intracellular reactive oxygen species leading to oxidative stress, invigorate stressed endoplasmic reticulum, and trigger cell death by autophagy (Dizaji BF et al., 2020).
The apoptotic pathway has emerged as a prominent target in the discovery and development of novel anticancer therapies. Most antitumor drugs currently used in clinical oncology exploit apoptotic signaling pathways to initiate cancer cell death (Pistritto et al., 2016). A previous study reported that the functionalization moieties of CNTs can significantly influence the cytotoxic effect and apoptosis induction. The inhibition of A549 cell lines treated with MWCNT-OH and MWCNT-COOH (pristine and functionalized MWCNTs) has been reported. It was found that the original MWCNTs caused lower cell viability than functionalized MWCNTs; however, functionalized MWCNTs were more genotoxic than pristine shape (Zhou L, et al., 2017). These previously reported findings are in accordance with the results of the present investigation concerning the positive effect of the prepared MWCNT/Arabic gum colloidal solution on the expression of apoptotic genes (Bax, P53, and caspases 3, 8, and 9) in both types of the investigated cells (MCF-7 and A549).
The molecular mechanisms underlying apoptosis contribute to establishing the basis for novel cancer therapies (Pfeffer, 2018). In both intrinsic (mitochondria-mediated) and extrinsic apoptosis, caspases (proteolytic enzymes) are activated to induce organelle damage (Danial, 2007). Above all this, P53 activates pro-apoptotic genes (BAX) in the intrinsic pathway and catalyzes various caspases, eventually leading to programmed cell death (Cheng, et al., 2015). This study demonstrated that the reaction of MCF-7 and A549 cells with the prepared MWCNTs increases the expression of P53 which in turn enhances MWCNT-induced apoptosis. Caspases are activated in all apoptosis pathways, and caspases 8 and 9 are the main initiators of the active caspases employed in the extrinsic and intrinsic apoptotic pathways, respectively. A study by Srivastava et al. (Srivastava, et al., 2013) is in accordance with our results and shows that p53 is among the major contributors in organizing cellular reduction-oxidation reactions, which may create oxidative stress and provoke DNA damage, leading to the development of apoptosis.
Protein array analysis also showed a high upregulation of the investigated MWCNTs treated cell lysis protein expression that allied with p53 and caspase 3 gene expression. In addition, 17 critical proapoptotic proteins, such as BAD, dimerize with BAX to induce cell death. Interestingly, BAX was significantly elevated during RT-PCR (Attri et al, 2021).
Using the GESA database to overlap with the high protein expression list (genes) revealed enrichment in cell damage and stress response pathways, as expected. However, it also intersects with TNF-α, TNF-related weak inducer of apoptosis, T cell receptor, and IL-1 signaling pathways (Mootha, et al., 2003; Subramanian, et al., 2005). In addition, the ceramide signaling pathway, a key player in cancer proliferation, autophagy, and drug resistance regulated by p53, is triggered (Jeffries, and Krupenko, 2018).
This investigation illustrates the fundamental role of MWCNT/Arabic gum colloidal solution in initiating apoptosis pathways at both the RNA and protein expression levels and other fundamental signals recognized as pro-apoptotic activators that would enhance the effectiveness of MWCNT/Arabic gum colloidal solution application on cancer cells and minimize drug resistance.
5 Conclusion
This study demonstrated the cytotoxic effect of MWCNTs on MCF-7 and A549 cells, as evaluated through MTT and LDH assays. Additionally, the expression of apoptosis-related genes was assessed using qRT-PCR, and the Human apoptosis signaling antibody array was employed to examine the involvement of TNF-α, IL-1, and ceramide signaling pathways in terms of enrichments. The MWCNTs were dispersed in an emulsifying agent composed of Arabic gum to handle and enhance the effectiveness of the investigated cells. By using the Arabic gum stabilizing agent as a natural and biocompatible polymer of low cost; the synthesis process is less expensive, more ecofriendly, and safe than the other acid or alkaline functionalization processes. We recommend the use of Arabic gum as a good emulsifying agent for medical applications as well as a good drug carrier agent for cancer therapy. The future studies will focus on the dynamic employ of MWCNTs and its metallic nanocomposites with an adequate in vivo dose. Furthermore, investigation will bem carried out to assess their potential adverse effects in vivo.
Data availability statement
The original contributions presented in the study are included in the article/Supplementary Material, further inquiries can be directed to the corresponding author.
Author contributions
Conceptualization; MA, NA, AQ, NA, SA, and WD; Methodology; MA, NA, AQ, NA, SA, and WD; formal analysis; MA, NA, AQ, NA, SA, and WD; investigation; MA, NA, AQ, NA, SA, and WD; resources; MA, NA, AQ, NA, SA, and WD; writing original draft preparation; MA, NA, AQ, NA, SA, and WD; writing review and editing; MA, NA, AQ, NA, SA, WD, and MM. All authors contributed to the article and approved the submitted version.
Funding
This work was funded by Princess Nourah bint Abdulrahman University Researchers Supporting Project number (PNURSP 2023R62), Princess Nourah bint Abdulrahman University, Riyadh, Saudi Arabia. Researchers Supporting Project number (RSP2023R26), King Saud University, Riyadh, Saudi Arabia.
Conflict of interest
The authors declare that the research was conducted in the absence of any commercial or financial relationships that could be construed as a potential conflict of interest.
Publisher’s note
All claims expressed in this article are solely those of the authors and do not necessarily represent those of their affiliated organizations, or those of the publisher, the editors and the reviewers. Any product that may be evaluated in this article, or claim that may be made by its manufacturer, is not guaranteed or endorsed by the publisher.
References
Abo-Dahab, S. M., Mohamed, R. A., Abd-Alla, A. M., and Soliman, M. S. (2023). Double-diffusive peristaltic MHD Sisko nanofluid flow through a porous medium in presence of non-linear thermal radiation, heat generation/absorption, and Joule heating. Sci. Rep. 13, 1432. doi:10.1038/s41598-023-27818-7
Akbar, N. S., Maraj, E. N., Noor, N. F., and Habib, M. B. (2022). Exact solutions of an unsteady thermal conductive pressure driven peristaltic transport with temperature-dependent nanofluid viscosity. Case Stud. Therm. Eng. 35, 102124. doi:10.1016/j.csite.2022.102124
Akram, J., Akbar, N. S., and Tripathi, D. (2022). Entropy generation in electroosmotically aided peristaltic pumping of MoS2 Rabinowitsch nanofluid. Fluid Dyn. Res. 54, 015507. doi:10.1088/1873-7005/ac4e7b
Al-zharani, M., Qurtam, A., Daoush, W., Eisa, M. H., Aljarba, N. H., Alkahtani, S., et al. (2021). Antitumor effect of copper nanoparticles on human breast and colon malignancies. Environ. Sci. Pollut. Res. 28, 1587–1595. doi:10.1007/s11356-020-09843-5
Attri, A., Thakur, D., Kaur, T., Sensale, S., Peng, Z., Kumar, D., et al. (2021). Nanoparticles incorporating a fluorescence turn-on reporter for real-time drug release monitoring, a chemoenhancer and a stealth agent: Poseidon’s trident against cancer. Mol. Pharm. 18 (1), 124–147. doi:10.1021/acs.molpharmaceut.0c00730
Batool, M., Khurshid, S., Qureshi, Z., and Daoush, W. M. (2021). Adsorption, antimicrobial and wound healing activities of biosynthesised zinc oxide nanoparticles. Chem. Pap. 75 (3), 893–907. doi:10.1007/s11696-020-01343-7
Butt, A. W., Akbar, N. S., Tripathi, D., and Akram, J. (2023). Analytical investigation of electroosmotically regulated peristaltic propulsion of Cu-water nanofluid through a microtube. Iraqi J. Sci. 64 (5), 2354–2367. doi:10.24996/ijs.2023.64.5.21
Casais-Molina, M. L., Cab, C., Canto, G., Medina, J., and Tapia, A. (2018). Carbon nanomaterials for breast cancer treatment. J. Nanomater. 2018. Article ID 2058613. doi:10.1155/2018/2058613
Chen, D., Dougherty, C. A., Zhu, K., and Hong, H. (2015). Theranostic applications of carbon nanomaterials in cancer: Focus on imaging and cargo delivery. J. Control. Release 210, 230–245. doi:10.1016/j.jconrel.2015.04.021
Chen, W., Zhao, J., Lee, J. Y. Z., and Liu, Z. (2005). Microwave heated polyol synthesis of carbon nanotubes supported Pt nanoparticles for methanol electrooxidation. Mat. Chem. Phys. 91 (1), 124–129. doi:10.1016/j.matchemphys.2004.11.003
Chen, Y. (2023). Nanotechnology for next-generation cancer immunotherapy: State of the art and future perspectives. J. Control. Release 356, 14–25. doi:10.1016/j.jconrel.2023.02.016
Cheng, C. H., Yang, F. F., Ling, R. Z., Liao, S. A., Miao, Y. T., Ye, C. X., et al. (2015). Effects of ammonia exposure on apoptosis, oxidative stress and immune response in pufferfish (Takifugu obscurus). Aquat. Toxicol. 164, 61–71. doi:10.1016/j.aquatox.2015.04.004
Cirillo, G., Vittorio, O., Kunhardt, D., Valli, E., Voli, F., Farfalla, A., et al. (2019). Combining carbon nanotubes and chitosan for the vectorization of methotrexate to lung cancer cells. Materials 12, 2889. doi:10.3390/ma12182889
Danial, N. N. (2007). BCL-2 family proteins: Critical checkpoints of apoptotic cell death. Clin. Cancer Res. 13, 7254–7263. doi:10.1158/1078-0432.ccr-07-1598
Daoush, W. M., and Imae, T. (2015). Fabrication of PtNi bimetallic nanoparticles supported on multi-walled carbon nanotubes. J. Exp. Nanosci. 10 (5), 392–406. doi:10.1080/17458080.2013.838703
Daoush, W. M., and Imae, T. (2012). Syntheses and characterizations of multi-walled carbon nanotubes-supported palladium nanocomposites. J. Mater. Res. 27 (13), 1680–1687. doi:10.1557/jmr.2012.123
Durgun, E., Dag, S., Bagci, V. M. K., Gülseren, O., Yildirim, T., and Ciraci, S. (2003). Systematic study of adsorption of single atoms on a carbon nanotube. Phys. Rev. B 67, 201401. doi:10.1103/physrevb.67.201401
Fotakis, G., and Timbrell, J. A. (2006). In vitro cytotoxicity assays: Comparison of LDH, neutral red, MTT and protein assay in hepatoma cell lines following exposure to cadmium chloride. Toxicol. Lett. 160, 171–177. doi:10.1016/j.toxlet.2005.07.001
Garcia-Martinez, G. C., Scott, R. W. J., and Crooks, R. M. (2003). Extraction of monodisperse palladium nanoparticles from dendrimer templates. J. Am. Chem. Soc. 125, 11190–11191. doi:10.1021/ja037196y
Gomez-Perez, S., McKeever, L., and Sheean, P. (2020). Tutorial: A step-by-step guide (version 2.0) for measuring abdominal circumference and skeletal muscle from a single cross-sectional computed-tomography image using the national institutes of health image J. JPEN J. Parenter. Enter. Nutr. 44 (3), 419–424. doi:10.1002/jpen.1721
Habib, M. B., and Akbar, N. S. (2021). New trends of nanofluids to combat Staphylococcus aureus in clinical isolates. J. Therm. Anal. Calorim. 143, 1893–1899. doi:10.1007/s10973-020-09502-4
Ijaz, F. S., Shahid, S. A., Khan, W., and Ahmad, Z. S (2017). Green synthesis of copper oxide nanoparticles using leaf extract: Antimicrobial, antioxidant and photocatalytic dye degradation activitie. J. Pharm. Res. 16, 743. doi:10.4314/tjpr.v16i4.2
Jeffries, K. A., and Krupenko, N. I. (2018). Ceramide signaling and p53 pathways. Adv. Cancer Res. 140, 191–215. doi:10.1016/bs.acr.2018.04.011
Johnson, W. (2005). Final report of the safety assessment of Acacia catechu gum, Acacia concinna fruit extract, Acacia dealbata leaf extract, Acacia dealbata leaf wax, Acacia decurrens extract, Acacia farnesiana extract, Acacia farnesiana flower wax, Acacia farnesiana gum, Acacia Senegal extract, Acacia Senegal gum, and Acacia Senegal gum extract 1. Int. J. Toxicol. 24 (3), 75–118. doi:10.1080/10915810500257170
Ke, P. C., and Qiao, R. (2007). Carbon nanomaterials in biological systems. J. Phys. Condens. Matter 19 (37), 373101. doi:10.1088/0953-8984/19/37/373101
Kihara, S., van der Heijden, N. J., Seal, C. K., Seal, C. K., Mata, J. P., Whitten, A. E., et al. (2019). Soft and hard interactions between polystyrene nanoplastics and human serum albumin protein corona. Bioconjug. Chem. 30 (4), 1067–1076. doi:10.1021/acs.bioconjchem.9b00015
Kirtonia, A., Gala, K., Fernandes, S. G., Pandya, G., Pandey, A. K., Sethi, G., et al. (2021). Repurposing of drugs: An attractive pharmacological strategy for cancer therapeutics. Seminars Cancer Biol. 68, 258–278. doi:10.1016/j.semcancer.2020.04.006
Kumar, L., Bisen, M., Harjai, K., Chhibber, S., Azizov, S., Lalhlenmawia, H., et al. (2023). Advances in nanotechnology for biofilm inhibition. ACS Omega 8 (24), 21391–21409. doi:10.1021/acsomega.3c02239
Kumar, P. G., Kumaresan, V., and Velraj, R. (2017). Stability, viscosity, thermal conductivity, and electrical conductivity enhancement of multi-walled carbon nanotube nanofluid using gum Arabic. Nanostructures 25 (4), 230–240. doi:10.1080/1536383x.2017.1283615
Li, F., and Sarah, P. (2009). Effect of nanoparticles on protein folding and fibrillogenesis. Int. J. Mol. Sci. 10, 646–655. doi:10.3390/ijms10020646
Liu, Z., Gan, L. M., Hong, L., Chen, W., and Lee, J. Y. (2005). Carbon-supported Pt nanoparticles as catalysts for proton exchange mem-brane fuel cells. J. Power Sources 139 (1–2), 73–78. doi:10.1016/j.jpowsour.2004.07.012
Lucafo, M., Gerdol, M., Pallavicini, A., Pacor, S., Zorzet, S., Da Ros, T., et al. (2013). Profiling the molecular mechanism of fullerene cytotoxicity on tumor cells by RNA-seq. Toxicology 314 (1), 183–192. doi:10.1016/j.tox.2013.10.001
Mahmoudi, M., Lynch, I., Ejtehadi, M. R., Monopoli, M. P., Bombelli, F. B., and Laurent, S. (2011). Protein-nanoparticle interactions: Opportunities and challenges. Chem. Rev. 111 (9), 5610–5637. doi:10.1021/cr100440g
Monopoli, M. P., Walczyk, D., Campbell, A., Elia, G., Lynch, I., Bombelli, F. B., et al. (2011). Physical−chemical aspects of protein corona: Relevance to in vitro and in vivo biolo- gical impacts of nanoparticles. J. Am. Chem. Soc. 133 (8), 2525–2534. doi:10.1021/ja107583h
Mootha, V. K., Lindgren, C. M., Eriksson, K. F., Subramanian, A., Sihag, S., Lehar, J., et al. (2003). PGC-1α-responsive genes involved in oxidative phosphorylation are coordinately downregulated in human diabetes. Nat. Genet. 34 (3), 267–273. doi:10.1038/ng1180
Mukhopadhyay, R., Kazi, J., and Debnath, M. C. (2018). Synthesis and characterization of copper nanoparticles stabilized with Quisqualis indica extract: Evaluation of its cytotoxicity and apoptosis in B16F10 melanoma cells. Biomed. Pharmacother. 97, 1373–1385. doi:10.1016/j.biopha.2017.10.167
Mzwd, E., Ahmed, N. M., Alsaee, S. K., Altowyan, A. S., Almessiere, M. A., Munirah, A., et al. (2022). Green synthesis of gold nanoparticles in Gum Arabic using pulsed laser ablation for CT imaging. Sci. Rep. 12, 10549. doi:10.1038/s41598-022-14339-y
Norde, W. (2008). My voyage of discovery to proteins in flatland and beyond. B Biointerfaces 61, 1–9. PubMed: 18023976. doi:10.1016/j.colsurfb.2007.09.029
Peng, Y., and Liu, H. (2006). Effects of oxidation by hydrogen peroxide on the structures of multi-walled carbon nanotubes. Ind. Eng. Chem. Res. 45 (19), 6483–6488. doi:10.1021/ie0604627
Pfeffer, C. M., and Singh, A. T. (2018). Apoptosis: A target for anti-cancer therapy. Int. J. Mol. Sci. 19 (2), 448. doi:10.3390/ijms19020448
Pistritto, G., Trisciuoglio, D., Ceci, C., Garufi, A., and D'Orazi, G. (2016). Apoptosis as anti-cancer mechanism: Function and dysfunction of its modulators and targeted therapeutic strategies. Aging (Albany NY) 8 (4), 603–619. doi:10.18632/aging.100934
Pourmadadi, M., Ghaemi, A., Shamsabadipour, A., Rajabzadeh-Khosroshahi, M., Shaghaghi, M., Rahdar, A., et al. (2023). Nanoparticles loaded with Daunorubicin as an advanced tool for cancer therapy. Eur. J. Med. Chem. 258, 115547. doi:10.1016/j.ejmech.2023.115547
RashmiIsmail, W. Af, SopyanJameelYusofKhalid, I. AtF. M., and Mubarak, N. M. (2011). Stability and thermal conductivity enhancement of carbon nanotube nanofluid using gum Arabic. J. Exp. Nanosci. 6 (6), 567–579. doi:10.1080/17458080.2010.487229
Riesz, P., BerdahI, D., and Christman, C. L. (1985). Free radical generation by ultrasound in aqueous and nonaqueous solutions. Environ. Health Perspect. 64, 233–252. doi:10.1289/ehp.8564233
Sani, A., Pourmadadi, M., Shaghaghi, M., Eshaghi, M., Shahmollaghamsary, S., Arshad, R., et al. (2023). Revolutionizing anticancer drug delivery: Exploring the potential of tamoxifen-loaded nanoformulations. J. Drug Deliv. Sci. Technol. 86, 104642. doi:10.1016/j.jddst.2023.104642
Savun-Hekimoğlu, B. (2020). A review on sonochemistry and its environmental applications. Acoustics 2, 766–775. doi:10.3390/acoustics2040042
Schirrmacher, V. (2018). From chemotherapy to biological therapy: A review of novel concepts to reduce the side effects of systemic cancer treatment (review). Int. J. Oncol. 54 (2), 407–419. doi:10.3892/ijo.2018.4661
Shemetov, A. A., Nabiev, I., and Sukhanova, A. (2012). Molecular interaction of proteins and peptides with nanoparticles. ACS Nano 6, 4585–4602. PubMed: 22621430. doi:10.1021/nn300415x
Song, J. E., Phenrat, T., Marinakos, S., Xiao, Y., Liu, J., Wiesner, M. R., et al. (2011). Hydrophobic interactions increase attachment of gum Arabic- and PVP-coated Ag nanoparticles to hydrophobic surfaces. Environ. Sci. Technol. 45 (14), 5988–5995. doi:10.1021/es200547c
Srivastava, R. K., Rahman, Q., Kashyap, M. P., Singh, A., Jain, G., Jahan, S., et al. (2013). Nano-titanium dioxide induces genotoxicity and apoptosis in human lung cancer cell line, A549. Hum. Exp. Toxicol. 32 (2), 153–166. doi:10.1177/0960327112462725
Subramanian, A., Tamayo, P., Mooth, V. K., Mukherjee, S., Ebert, B. L., Gillette, M. A., et al. (2005). Gene set enrichment analysis: A knowledge-based approach for interpreting genome-wide expression profiles. Proc. Natl. Acad. Sci. 102 (43), 15545–15550. doi:10.1073/pnas.0506580102
Sung, H., Ferlay, J., Siegel, R. L., Laversanne, M., Soerjomataram, I., Jemal, A., et al. (2021). Global cancer statistics 2020: GLOBOCAN estimates of incidence and mortality worldwide for 36 cancers in 185 countries. CA Cancer J. Clin. 71 (3), 209–249. doi:10.3322/caac.21660
Tans, S. K., Verschueren, A. R. M., and Dekker, C. (1998). Room-temperature transistor based on a single carbon nanotube. Nature 49, 49–52. doi:10.1038/29954
Tedeschi, P., Maietti, A., Boggian, M., Vecchiati, G., and Brandolini, V. J. (2007). Fungitoxicity of lyophilized and spray-dried garlic extracts. Sci. Health, Part B 42, 795–799. doi:10.1080/03601230701551459
Verma, S. K., Jha, E., Panda, P. K., Thirumurugan, A., Parashar, S. K. S., Patro, S., et al. (2018). Mechanistic insight into size-dependent enhanced cytotoxicity of industrial antibacterial titanium oxide nanoparticles on colon cells because of reactive oxygen species quenching and neutral lipid alteration. ACS Omega 3 (1), 1244–1262. doi:10.1021/acsomega.7b01522
Wang, T., Chen, X., Long, X., Liu, Z., and Yan, S. (2016). Copper nanoparticles and copper sulphate induced cytotoxicity in hepatocyte primary cultures of Epinephelus coioides”. PLoS ONE 11 (2), e0149484. doi:10.1371/journal.pone.0149484
White, C. T., and Mintmire, J. W. (2005). Fundamental properties of single wall carbon nanotubes. J. Phys. Chem. B 109, 52–65. doi:10.1021/jp047416+
Xia, G. H., Chen, B. A., Shao, Z. Y., Lu, H. X., Konstanze, D., and Hartmut, D. (2007). Mechanism of 2-methoxyestradiol-induced apoptosis in myelodysplastic syndrome MUTZ-1 cell line. Zhongguo Shi Yan Xue Ye Xue Za Zhi 15, 296–301.
Xu, P., Shi, L., Huang, J., Wang, C., Zhang, Y., and Xu, H. (2018). Methods of dispersion and stabilization of several nanomaterials in water. Ferroelectrics 527 (1), 133–148. doi:10.1080/00150193.2018.1450560
Zheng, H. T., Li, Y., Chen, S., and Shen, P. K. (2006). Effect of supportonthe activity of Pd electrocatalyst for ethanol oxidation. J. Power Sources 163, 371–375. doi:10.1016/j.jpowsour.2006.09.062
Keywords: multi-walled carbon nanotubes, Arabic gum, breast cancer, lung cancer, cell line, apoptosis genes
Citation: Al-zharani M, Alyami NM, Qurtam AA, Aljarba NH, Alkahtani S, Mubarak M and Daoush WM (2023) Use of multi-walled carbon nanotubes (MWCNTs) stabilized in Arabic gum colloidal solution to induce genotoxicity and apoptosis of human breast and lung cancer cell lines. Front. Mater. 10:1229637. doi: 10.3389/fmats.2023.1229637
Received: 26 May 2023; Accepted: 24 July 2023;
Published: 10 August 2023.
Edited by:
Sadanand Pandey, Yeungnam University, Republic of KoreaReviewed by:
Deepak Kumar, Shoolini University, IndiaAbbas Rahdar, Zabol University, Iran
Elvis Fosso Kankeu, University of South Africa, South Africa
Copyright © 2023 Al-zharani, Alyami, Qurtam, Aljarba, Alkahtani, Mubarak and Daoush. This is an open-access article distributed under the terms of the Creative Commons Attribution License (CC BY). The use, distribution or reproduction in other forums is permitted, provided the original author(s) and the copyright owner(s) are credited and that the original publication in this journal is cited, in accordance with accepted academic practice. No use, distribution or reproduction is permitted which does not comply with these terms.
*Correspondence: Mohammed Al-zharani, bW15YWx6YWhyYW5pQGltYW11LmVkdS5zYQ==
‡These authors have contributed equally to this work