- 1Department of Transportation and Geotechnical Engineering, Balochistan Campus, National University of Sciences and Technology (NUST), Quetta, Pakistan
- 2Department of Civil Engineering, Universiti Tenaga Nasional, Kajang, Malaysia
- 3Department of Civil Engineering, Universiti Malaya, Kuala Lumpur, Malaysia
- 4Departement of Civil and Natural Resources Engineering, Lulea University of Technology, Lulea, Sweden
- 5Department of Civil Engineering, School of Engineering, Monash University Malaysia, Selangor, Malaysia
- 6School of Engineering, Edith Cowan University, Perth, WA, Australia
The addition of polymer to a base binder has been documented as a successful approach in terms of improving physical and rheological properties of the base bitumen. However, the main drawbacks of polymer-modified bitumen are incompatibility and degradation of polymer due to aging. This article aims to introduce a bibliometric analysis and review on modifying bitumen with polymers. Additionally, this article intent to highlight the significant gaps and recommendations for future work. Furthermore, another objective of this article is to provide a worth attempt regrading reducing the negative impact of polymer’s drawbacks on the performance of polymer-modified base binder. The findings of this article demonstrated that the test of storage stability for polymer-amended bitumen should be modified, in which the softening point of aluminum tube centerpiece should be measured to introduce a better evaluation for the storage stability of polymer-amended bitumen. In addition, the effects of kinetic factors (mixing sequence, viscosity of blend, shear rate, and time of mixing) on the compatibility of polymer-amended bitumen should be investigated. Moreover, the addition of compatibilizers and stabilizers to polymer-modified binder is recommended to improve compatibility and reduce the effect of aging on degradation of polymer.
1 Introduction
Bitumen is a by-product of crude oil, which is obtained from crude oil distillation. The distillation procedures are started by removing the lighter fractions through heating crude oil at temperature of 300°C–350°C in the distillation column. Subsequently, residue is further distilled in a vacuum distillation column (Waheed and Oni, 2015; Boczkaj et al., 2017). However, bitumen is widely used in the applications of pavement due to its adhesion property. In other words, bitumen works as an adhesive material that binds coarse aggregates, fine aggregates, and filler to form the layer of asphalt on the roads (Pyshyev et al., 2016; Oliviero Rossi et al., 2017; Nizamuddin et al., 2021; Alnadish et al., 2020b). On the other hand, bitumen is a viscoelastic material that behaves as an elastic material at high temperature and viscous at low temperature. The elastic property indicates the ability of bitumen to recover after deformation, while the viscous property implies the inability of bitumen to recover after deformation (Behzadfar and Hatzikiriakos, 2013; Alnadish et al., 2020a; Alnadish et al., 2021). Moreover, the physical and rheological properties of bitumen play a major role in terms of identifying the lifespan of the asphalt layer. The better the physical and rheological properties of bitumen, the longer the lifespan of the asphalt layer. Otherwise, the main distresses that significantly affect the performance of bitumen are rutting, fatigue, low-temperature cracking, and aging. The damage of rutting occurs at high temperature, in which the bitumen becomes soft and susceptible to the vertical deformation due to the traffic loads. While at low/intermediate temperatures the distresses of thermal cracking and fatigue cracking (horizontal cracks) occur due the stiff behaviour of bitumen at low/intermediate temperatures due to aging. However, these cracks rapidly decrease the life span of the asphalt layer due to the water seepage to the pavement layers. Therefore, the bitumen should be hard enough at high temperatures to resist the vertical deformation and elastic at low/intermediate temperatures to resist the occurrence of horizontal cracks (Subhy, 2017; Teltayev and Radovskiy, 2018; Alnadish et al., 2022; Ishaq et al., 2022).
Accordingly, vigorous efforts by researchers have been made to improve performance of bitumen in terms of hardness and elasticity. Modifying bitumen with polymers is one of the well-known approaches in enhancing performance of bitumen (Brasileiro et al., 2019; Nizamuddin et al., 2021; Martinho et al., 2022). It is documented that introducing polymer to a base binder significantly improves the hardness of bitumen at high temperatures and low-temperature performance through enhancing elasticity of the base binder. Thus, the service life of the asphalt layer incorporated modified bitumen with polymer is notably improved (Pérez-Lepe et al., 2005; Sengoz and Isikyakar, 2008; Bulatović et al., 2012; Zhu et al., 2014; Nizamuddin et al., 2020). Moreover, the main drawbacks of utilizing polymers as bitumen modifiers are incompatibility and degradation. Incompatibility occurs due to the differences in polarity, densities, and high interfacial tension, while the degradation of polymer-amended bitumen is attributed to subjecting bitumen to short-term and long-term aging (Kalantar et al., 2012; Munera and Ossa, 2014; Behnood and Gharehveran, 2019; Porto et al., 2019; Zhou et al., 2020).
The objective of this article is to introduce a bibliometric analysis in order to discover the trend of modifying bitumen with polymers. Another aim of this article is to conduct a review on the performance of polymer-modified bitumen. However, the drawbacks of polymer blend/polymer-amended binder in terms of immiscibility/separation phase and degradation were highlighted in this article. In addition, the common methods regarding evaluating and enhancing the phase separation of polymer blend have been introduced. Lastly, the research gaps and recommendations on the polymer-modified bitumen were highlighted. Additionally, strategies for reducing the influence of polymers’ drawbacks were proposed.
2 Chemical composition of bitumen
Bitumen can be separated into the chemical groups of asphaltenes and maltenes. However, maltenes can be sub-divided into aromatics, resins, and saturates. Asphaltenes are insoluble solids characterized by the color of black/brown and the molecular weight of 800–3,500 g/mol. Asphaltenes represents 5%–20% by bitumen weight. However, asphaltenes components have direct effect on the physical properties of bitumen. The higher the content of asphaltenes, the higher the viscosity, softening point and the lower the penetration of bitumen. Resins are soluble solids with drack brown color. The range of resins molecular weight is 780–1400 g/mol. Bitumen weight consisting of resins by about 30%–45%. Resins are responsible for ductility and adhesion properties of bitumen. Aromatics are non-polar carbon viscous liquids characterized by dark brown color and the molecular weight of 570–980 g/mol. However, 30%–45% of bitumen weight composed of aromatics. The molecular weight range of saturates is 470–880 g/mol, which are white in color, and non-polar viscous oils. Saturates represents 5%–15% by bitumen weight. Aromatics and saturates are responsible for viscosity of bitumen (Read et al., 2003; Lesueur, 2009; Redelius and Soenen, 2015). Table 1 summarizes the chemical components of asphaltenes, resins, aromatics, and saturates.

TABLE 1. Chemical composition of bitumen (Read et al., 2003; Lesueur, 2009; Redelius and Soenen, 2015).
2.1 Methods of bitumen separation into four fractions
Methods of bitumen separation play a vital role in identifying asphaltenes, resins, aromatics, and saturates components in bitumen. Thus, the method of separation has a significant impact on the chemical evaluation of bitumen. There are different methods have been utilized in separating the components of bitumen. However, the most utilized methods in separating bitumen are Rostler and Sternberg (ASTM D2006), the clay-gel method (ASTM D2007), and Corbett-Swarbrick (ASTM D4124). According to the method developed by Rostler and Sternberg, the separation process starts by removing asphaltenes components from bitumen using n-pentane. Thereafter, the other fractions are separated in sequence using the solution of sulfuric acid. Figure 1A shows the procedures of separation (Goodrich et al., 1986). However, this method has been discounted in 1976 due to the hazardous work environment and the exotic acidic conditions that prevent the recovery of frictions for additional analysis. Therefore, the method of clay-gel has been developed. Figure 1C summarizes the separation processes of bitumen frictions. In this method, the asphaltenes components are removed firstly using n-pentane. Thereafter, the other frictions are introduced into two columns, the upper and lower column are filled with clay and activated silica gel. Then, the sample is filtered using n-pentane. This method is characterized by its simplicity, lower cost, and safe work environment. On the other hand, Corbett-Swarbrick method is an adsorption chromatography technique used to separate bitumen into frictions. The procedures of this method is illustrated in Figure 1B. In this method, the processes starts by removing asphaltenes using n-pentane, followed by adsorbing the remaining fractions on a chromatographic column with solvents of increasing polarity. This method is characterized by separating bitumen into frictions with homogeneity and less complex. In addition, the remaining frictions in this method can be used for further separations and analysis (Claine, 1984; Thenoux, 1987).
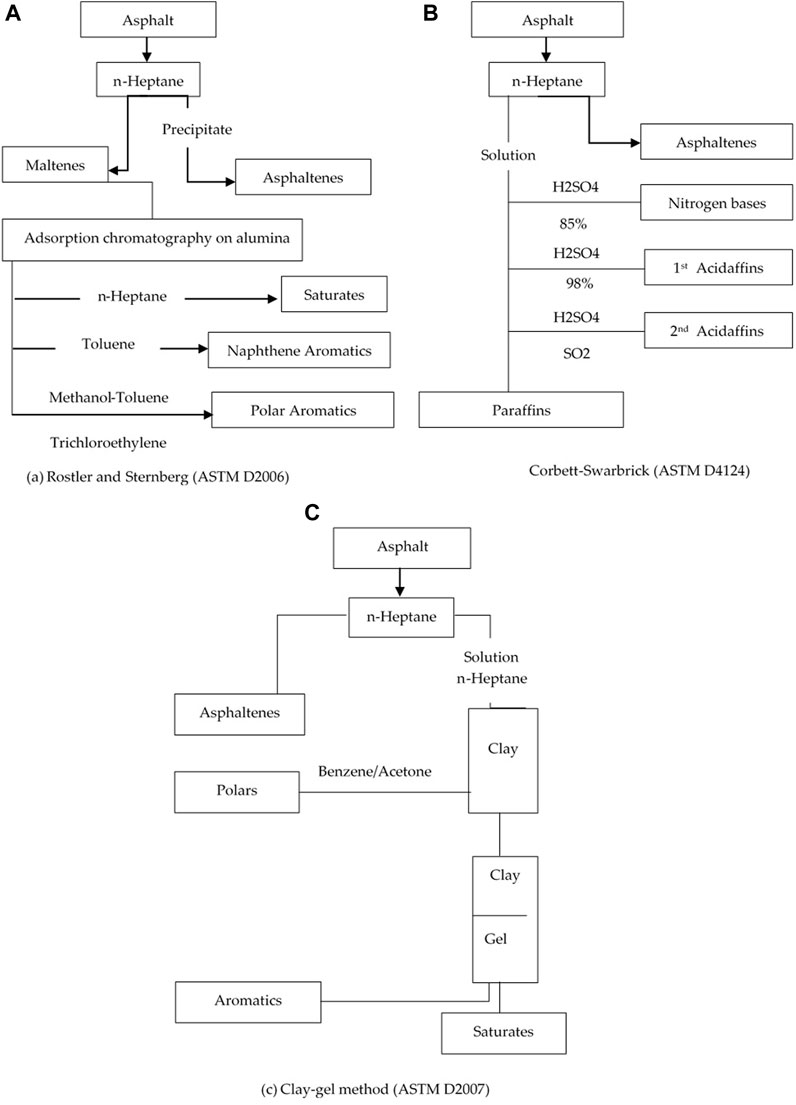
FIGURE 1. Methods of bitumen separation (Claine, 1984; Goodrich et al., 1986; Thenoux, 1987).
2.1.1 Physical properties of bitumen
2.1.1.1 Hardness
Harness refers to the ability of bitumen to resist deformation. The hardness of bitumen is assessed using the test of penetration (Tarefder et al., 2010). Penetration test aims to determine the penetrated depth by a needle with a load of 100 g at a temperature of 25°C. The lower the depth of a needle, the better the hardness of bitumen (Jia et al., 2005).
2.1.1.2 Ductility
Ductility of bitumen indicates the capability of bitumen to elongate without failure under traffic loads (Pereira et al., 2018). The empirical experiment of ductility is used to measure bitumen ductility through stretching a bitumen sample at a temperature of 25°C until breaking point. The longer the stretching, the higher ductile and elastic behavior of bitumen (Jia et al., 2005).
2.1.1.3 Viscosity
The ability of bitumen to be mixed with aggregates and desirable coating refers to viscosity. The viscosity of bitumen is determined using the device of rotational viscometer (Redelius and Soenen, 2015). This test aims to measure the required torque to maintain a constant speed of 20 RPM at testing temperature of 135°C (Jia et al., 2005).
2.1.1.4 Durability
Durability refers to the ability of bitumen to resist environment condition. In other words, durability indicates the resistance of bitumen to aging. The high bitumen resistance to aging implies the longer life of bitumen in situ. Aging of bitumen result in a significant change of the physical and chemical properties. However, bitumen aging occurs due to the reaction between bitumen and oxygen, evaporation of bitumen lightweight components during mixing and placing, and strict hardening. Aging of bitumen can be divided into short-term aging and long-term aging. Short-term aging occurs during mixing and placing of asphalt mix, while long-term aging is occurred during the service life of asphalt layer by the reactions between environment conditions and bitumen (Sirin et al., 2018; Prosperi and Bocci, 2021). However, the test of rolling thin film oven (RTFO) is used to simulate the short-term aging of binder. In this test, an eight glass bottles are filled with 35 g of bitumen. Thereafter, the bottles are placed in a heated oven at a temperature of 163°C and rotated for 85 min at 85 RPM. The test of pressure aging vessel is used to simulate the long-term aging of bitumen. In this test, the subjected samples of bitumen to short-term aging are placed in steel pans. Subsequently, subjecting the samples of binder to aging for 20 h in a heated vessel pressurized at temperature 90°C–110°C to 2.10 MPa. Then, the physical, rheological, and chemical properties of binder are determined to study the effect of aging on the properties (Jia et al., 2005).
2.1.2 Rheological properties of bitumen
The viscoelastic property of bitumen is measured using dynamic shear rheometer (DSR). However, elastic property refers to the bitumen ability to recover after deformation, while the viscous property indicates the inability of binder to recover after removing load (Pszczola et al., 2018). DSR aims to determine the performance of bitumen at high, intermediate, and low temperatures through identifying the complex shear modulus (G*) and phase angle (δ). In other words, DSR test measures the resistance of binder to rutting and fatigue. However, G* refers to the rutting resistance of binder, while δ indicates the elastic behavior of bitumen. The increase in G* implies the better resistance to rutting, while the decrease in the δvalue implies the better elastic behavior of binder. In other words, higher G* and lower δ refers to the desirable performance of bitumen. However, the rutting factor of G*/sinδ is used to assess the rutting resistance of bitumen sample (Zeng et al., 2022; Gu et al., 2019; Liu et al., 2022). According to Superpave specifications, the value of rutting factor must be at least 1 kPa for unaged binder, while the rutting factor of short-term aged (RTFO) bitumen should be higher than 2.20 kPa.On the other hand, the factor of G*sinδ refers to the resistance of bitumen to fatigue cracking. The lower the factor of G*sinδ, the better the resistance to fatigue. According to Superpave specifications, the value of G*sinδ for long-term (PAV) aged binder should be less than 5,000 kPa. Additionally, the value of G*sin for long-term (PAV) aged binder at low temperature must be less than 5,000 kPa (Jia et al., 2005).
2.1.3 Low-temperature properties of bitumen
The decrease in the surrounding temperature leads to shrinkage of bitumen, which, in turn, generates stresses leading to thermal cracks due to inability of bitumen to relax stresses. However, the tests of bending beam rheometer (BBR) and direct tension tester (DTT) are used to measure the stiffness and relaxation properties at low temperatures. In the test of BBR, the bitumen specimen is submerged in a cold liquid bath, followed by subjecting the center of the sample to a specific load. Thereafter, the generated deflection by the load is identified. However, the stiffness and relaxation of bitumen is measured by the test of DTT through subjecting the sample to a constant rate of elongation, followed by the determination of sample stress and strain at failure (Jia et al., 2005).
3 Polymers
3.1 Types of polymers
Modifying bitumen with polymers is the most used approach to enhance the physical and rheological properties of bitumen. However, bitumen modifiers can be divided into thermoplastic elastomers, thermoplastic polymers, and thermosetting resins. Thermoplastic elastomers is also called rubber, while thermoplastic polymers called plastics. However, thermoplastic elastomers/rubbers can be classified into Styrene-butadiene- styrene (SBS), Styrene-butadiene- rubber (SBR), Styrene-isoprene- Styrene (SIS), Styrene-ethylene-butylene -Styrene (SEBS), natural rubber, while thermoplastic polymers/plastics can be categorized into Ethylene-vinyl-acetate (EVA), Polyethylene (PE), ethylene butyl acrylate (EBA), polyvinyl chloride (PVC), polypropylene (PP), polystyrene (PS) (Fakirov, 2006; Shanks, 2012). Thermoplastic elastomers are produced naturally from trees such as latex rubber or industrially from petroleum oil, while thermoplastic polymers are obtained from crude oil. However, thermoplastic elastomers and thermoplastics are widely used in modifying bitumen due to their abilities to enhance physical and rheological properties of bitumen. Furthermore, the main drawbacks of using polymers in binder are incompatibility and degradation of polymers due to aging (Nizamuddin et al., 2021; Xu et al., 2022). However, asphalt layer is subjected to low and high temperature during its service life. Therefore, the glass transition (Tg) of polymer play a vital role in changing the properties of polymers. Glass transition refers to the temperature at which polymer becomes stiff (glassy state) when subjected to a temperature below glass transition temperature, while the polymer becomes elastic (rubbery state) when exposed to a temperature higher than the glass transition temperature (Beyler and Hirschler, 2002; Fred-Ahmadu et al., 2020). Table 2 summarizes the glass transition temperature (Tg), melting temperature (Tm), and density for some polymers.
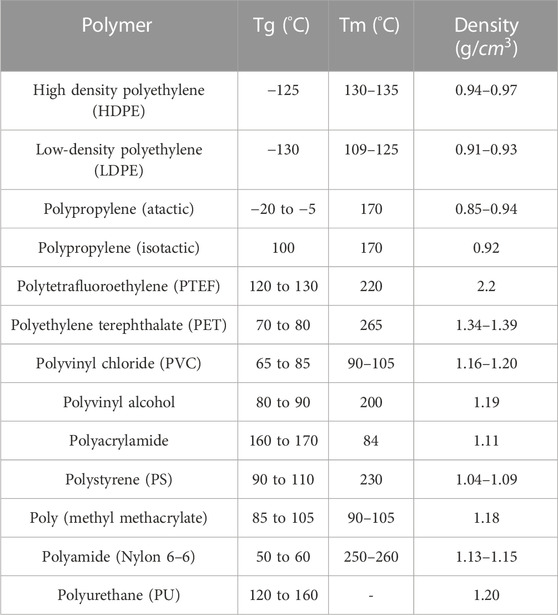
TABLE 2. Glass transition temperature (Tg), melting temperature (Tm), and density of polymers (Beyler and Hirschler, 2002; Fred-Ahmadu et al., 2020).
3.2 Polymer blend
Polymer blend refers to the blending of one or more polymer to produce a new type of polymer characterized by its desired performance and low cost. However, different methods are used to blend polymers, which are solution mixing, melt blending, latex blending, partial block/graft copolymerization, and interpenetrating polymer networks (IPN) (Mao et al., 2020).
3.3 Drawbacks of polymer blend
3.3.1 Immiscibility/separation phase
The blend of polymers maybe miscible (homogeneous) or immiscible (heterogeneous). The main factors that affect miscibility of polymer blend are temperature, polarity, molecular weight, pressure, interfacial tension, and composition of polymer. The higher the temperature of blend, the better the miscibility of polymer blend, while the difference in polarity of polymers refers to the immiscibility of blend. This is because polar materials are attractive to polar materials and polar solvent, while the non-polar materials are attractive to the non-polar materials and non-polar solvent. In other words, there is no attraction between the polar materials and non-polar materials. Additionally, the increase in the weight of molecular significantly decrease the miscibility of blend. The high interfacial tension between the components of polymers leads to immiscibility/separation phase in the blend of polymer. Furthermore, the blend of polymer can be classified into miscible polymer blend, partially miscible blend (compatible blend), and immiscible polymer blend (Robeson, 2014; Ajitha and Thomas, 2020a; Shanks, 2020). However, the thermodynamic parameter of Gibbs free energy is used to evaluate the separation phase of polymer blend. Gibbs free energy (ΔGm) is calculated through the difference between enthalpy (ΔHm) and entropy (TΔSm) of mixing (ΔGm = ΔHm- TΔSm). Polymer blend is considered miscible if the Gibbs free energy is negative, while the positive value of Gibbs free energy implies the immiscibility of polymer blend. In other words, the negative Gibbs free energy indicates the superior interaction between the components of polymers in the blend.
Moreover, the miscible blend shows negative Gibbs free energy, single-phase morphology, and single glass transition, while the compatible blend shows negative Gibbs free energy, single/two-phase morphology, and single/two glass transition. Otherwise, the immiscible blend displays positive Gibbs free energy, two-phase morphology, and two-glass transition. Furthermore, the degree of miscibility of polymer blend can be identified through the diagram of phase. Figure 2 shows the phase diagram of polymer blend. It can be seen in the figure that there are three regions, which are stable/miscible region, unstable/immiscible region, and metastable region. The miscible region is located between the two binodal, while the immiscible region is surrounded by the curve of spinodal. Otherwise, the region area between the curve of binodal and spinodal refers to the metastable region. However, the binodal separation phase occurs when the temperature of miscible polymer blend brought to the temperature of immiscible/unstable region. The phase of binodal separation produces a fine two-phase. The separation phase of spinodal occurs when the miscible blend at the stable region is forced to the immiscible/unstable region. This separation phase generates an interconnected structures in both minor and major phases (Utracki and Wilkie, 2002; Sarath et al., 2014; Shanks, 2020). However, incompatibility/separation of polymer-modified bitumen is occurred by the same mechanism of separation phase/immiscibility of polymer blend, in which the occurrence of incompatibility and uneven poorly structure/spherical droplets between polymer and bitumen is attributed to the difference in polarity, high interfacial tension, and different in density between the components of blend. Therefore, the addition of compatibilizer to polymer-modified bitumen is required to improve homogeneity between polymer and bitumen. However, the occurrence of separation due to difference in densities of blend components is slightly different from the separation phase that occurs at the interface of the blend due to the differences in polarity, high interfacial tension, and temperature. However, the change in densities results in a significant change in the physical properties and rheological properties of polymer-modified bitumen. This is because the addition of polymer/nanomaterials with low density as compared to that of bitumen will float, while the introduction of polymer/nanomaterials with a density higher than the density of bitumen will sink. The sinking/floating of bitumen modifiers depends on the diameter and viscosity of polymer-modified blend. According to the principles of the Stokes’ law, the higher the viscosity of blend, the lower the sinking/floating (velocity of separation) of bitumen modifiers, while the lower the diameter of nanomaterials/droplet of blend, the lower the velocity of separation. The velocity of separation is identified through the equation below (Munera and Ossa, 2014; Porto et al., 2019; Behnood and Gharehveran, 2019; Brûlé, 1996).
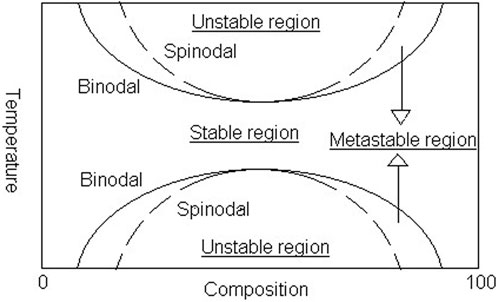
FIGURE 2. Phase diagram of polymer blend of polymer blend (Robeson, 2014).
Where V is velocity of separation, g is the acceleration of gravity, r is the radius of droplet, d1is the density of minor phase (nanomaterials/polymer), d2is the density of major phase (bitumen), and
Understandably, the separation between water and oil is occurred by the difference in density, in which oil floats due to its low density as compared to density of water. In addition, separation phase/immiscibility at interface is attributed to the difference in polarity, in which water is polar and oil is non-polar, thus there are no attraction between molecules. Furthermore, the difference in polarity leads to the increase of interfacial tension, which, in turn, leads to the immiscibility between water and oil (separation phase at interface). The sepration phase at interface leads to uneven poorly structure/spherical droplets. Thus, the addition of emulsifier/surfactant is required to stabilize the blend of water and oil through coating droplets and eliminate forming of coalescing. In other words, the blend of water and oil becomes miscible by the addition of emulsifier/surfactant, this is because the molecules of emulsifier has one end attracted to water (polar) and one end attracted to oil (non-polar). Therefore, the addition of emulsifier reduces the interfacial tension and links between molecules of water and oil, which, in turn, improve morphology at interface and separation due to the difference in densities (Lu et al., 1999; Pérez-Lepe et al., 2006; Pérez-Lepe et al., 2006; Xue et al., 2014; Padaki et al., 2015; Gupta et al., 2017; Pérez-Lepe et al., 2007).
3.3.1.1 Methods to evaluate the separation phase/immiscibility of polymer blend
3.3.1.1.1 Morphology (microscopy)
The immiscibility/separation phase of polymer blend at interface can be evaluated by means of morphology. Morphology is used to describe the minor dispersion phase (domain/droplet) on major continuous phase, fibers phase on the major phase, degree of miscibility through identifying the domain size, shape, distribution of polymer blend. Figure 3 shows the minor phase (domain/dispersed phase) of polymer (B) and the major phase of polymer (A) for polymer blend (A/B) (Utracki and Wilkie, 2002).
However, the formation of morphology depends on viscosity, interfacial tension, interfacial adhesion, and blending protocol. The domain size (dispersed phase) distribution depends on the concentration of polymer (B) composition in the blend. The increase in the concentration of polymer (B) in the blend leads to the increase in the number of domain particles and size of domain due to coalescence. The high rate of coalescence between domain particles occurs at the high interfacial tension, low shear rate, and long mixing time. Otherwise, the viscosity of polymer blend (A/B) play a major role in terms of the coalescence rate. If the viscosity of minor phase is lower than that of major phase the rate of coalescence is dramatically reduced due to the restricted diffusion of minor phase on the high viscous of major phase. Moreover, the size of domain has a significant effect on the mechanical properties of blend. The higher the size of domain, the poorer the performance of polymer blend. On the other hand, the common techniques in analyzing the morphology of polymer blend are transmission electron microscopy (TEM), scanning electronic microscopy (SEM), atomic force microscopy (AFM), optical microscopy, fluorescence microscopy, confocal microscopy. These techniques aim to identify the phase separation, domain size, distribution of separated phase, and surface roughness of polymer blend (Utracki and Wilkie, 2002; Sarath et al., 2014; Ajitha and Thomas, 2020b; Shanks, 2020).
3.3.1.1.2 Glass transition temperature
The most accepted approach in evaluating the immiscibility/phase separation is by identifying the glass transition temperature of polymer blend. The single glass transition of polymer blend indicates the miscibility of blend, while the multiple glass transition glass implies the partially miscible/immiscibility of blend. However, the crosslinking degree, average mass of molar, flexibility of chain, and crystallinity are the main factors that affect the glass transition temperature. Otherwise, the most common technique in determining the glass transition temperature of polymer blend is differential scanning calorimetry (DSC). Furthermore, the techniques of dynamic mechanical analysis (DMA), dielectric relaxation spectroscopy (DRS), thermogravimetric Analysis (TGA), and Solid-state nuclear magnetic resonance (NMR) are utilized to determine the glass transition temperature of polymer blend (Utracki and Wilkie, 2002; Sarath et al., 2014; Ajitha and Thomas, 2020a; Shanks, 2020).
3.3.2 Degradation of polymers
Most polymers are susceptible to degradation over time when exposed to heat, light, and oxygen. These conditions make a significant change in the physical and mechanical properties of polymers. However, the mechanism of polymers degradation can be divided into initiation, propagation, and termination. In the initiation mechanism, when the polymer is exposed to heat and light, the oxidative degradation is started by forming radical chains/free radical (R) through the hydrogen abstraction (He et al., 2021; Visan et al., 2021). The reactions of initiation are shown below.
The propagation mechanism refers to the form of peroxy radical (ROO*) by the reaction between free radical (R) and Oxygen (O2), which then produce new radical to react with more chains and obtain more base radicals. The reactions are described as follow:
In the termination, the coupling termination is occurred by the reactions between radicals with each other. The reactions of termination are demonstrated below.
3.3.3 Methods of enhancing miscibility/compatibility, stabilization, and performance of polymer blend
3.3.3.1 Approaches of polymer blend compatibilization
Compatibilization refers to the addition of compatibilizers into polymer blend to improve the behavior of polymer blend through reducing the interfacial tension, morphology stabilization, and improve the adhesion between phases in the solid state. This in turn results in the fine distribution of polymer composition and decreases the conglomeration. However, the common approaches in improving the compatibility of polymer blend can be classified into.
3.3.3.1.1 Non-reactive copolymers
Block/graft copolymers are used as non-reactive copolymers (compatibilizers) to improve compatibility of polymer blend. However, the addition of block/graft copolymers to polymer blend is able to create a stable morphology of blend through reducing the interfacial tension and this leads to the reduction in domain size and better distribution of dispersed phase. This is because the addition of compatibilizers to the blend of polymer (A/B) works as emulsifiers/surfactants, which means one end of block/graft copolymers is attractive/miscible to one part of the blend (A), while the another end is attractive to the second part of the blend (B). In other words, the interaction between compatibilizers and polymer blend (A/B) produces the fine distribution of polymer blend composition through breaking up the domain size into small sizes. However, the efficiency of compatibilizers is highly affected by the molecular weight, which means that if the molecular weight of homopolymer is much higher than that of arm copolymer, the compatibilizers cannot behave as surfactant. Therefore, the increase in the molecular weight and concentration of copolymer reduces the interfacial tension. The common used compatibilizers for the blend of polymer are block copolymers, this is attributed to the greater interfacial adhesion as compared to graft copolymers. Moreover, the main difference between graft copolymers and block copolymers is that the repeating units of graft copolymers are chain, while repeating units of the block copolymers are linear (Koning et al., 1998; Ajitha and Thomas, 2020b). Table 3 demonstrates some of compatibilizers for polymer blend.
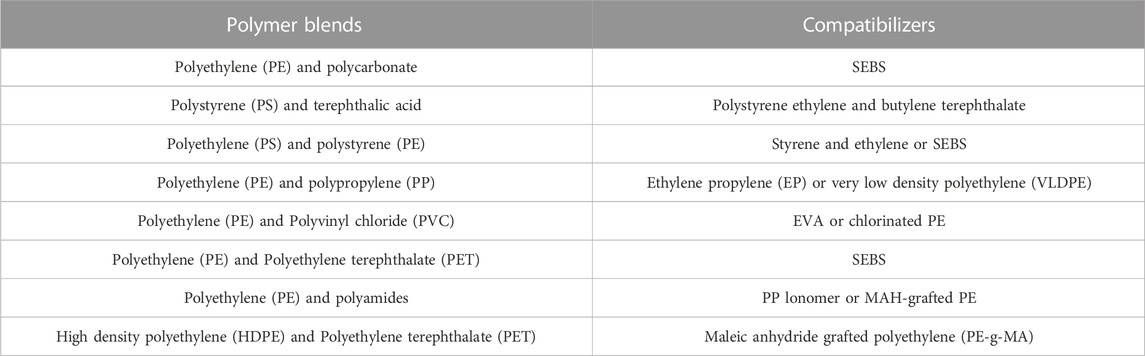
TABLE 3. Compatibilizers of polymer blend (Chen and White, 1993; Oliphant et al., 1993; Jarukumjorn, 2007).
3.3.3.1.2 Reactive copolymers
The use of graft/block copolymers as compatibilizers depends on the miscibility between compatibilizers and components of blend. In some cases, it is difficult to obtain a miscible compatibilizer with components of blend. Therefore, the use of reactive copolymers is worthwhile. The compatibilization of polymer blend by reactive copolymers is done by adding a copolymer with at least one component incorporating functional groups. However, the copolymer component that consisted of functional groups is attractive/miscible with one component of polymer blend, i.e., (A) through the addition of copolymer to the polymer blend (A/B) during preparation of blend (melt mixing). Thereafter, the melt preparation is followed by the in situ-formed graft/block copolymer, in which the component of added copolymer is attractive/miscible with the another component of polymer blend, i.e., (B) (Utracki and Wilkie, 2002; Sarath et al., 2014; Shanks, 2020).
3.3.3.1.3 Nanomaterials
Nanomaterials with size in the range of 1–100 nm are considered nanofiller. Nanofillers/nanomaterials can be categorized based on the nanoscale into zero-dimensional (0D), one-dimensional (1D), two-dimensional (2D), and three-dimensional (3D). Zero-dimensional indicates that all dimensions of particles are less than 100 nm such as carbon nanocapsules, and quantum dots, while one-dimensional nanomaterials implies that one dimension of the particle is higher than 100 nm and the another dimension is lower than 100 nm such as nanotubes, nanorods, and nanowires. Additionally, the two-dimensional nanomaterials refers to the materials that all their dimensions are higher than 100 nm such as silicates, clays, graphene, while the materials that are not confined to the nanoscale such as silica and nanoparticles metal are considered 3D nanomaterials. However, the addition of nanomaterials to an immiscible polymer blend significantly improves compatibility/miscibility and performance of polymer blend. This is because introducing nanomaterials to the polymer blend reduces the size of dispersed phase and suppress the coalescence between dispersed phases through reducing the interfacial tension. Furthermore, the compatibilization mechanism of polymer blend by means of nanomaterials depends on the selective localization of nanomaterials (Mochane et al., 2020; Munera and Ossa, 2014; Porto et al., 2019; Behnood and Gharehveran, 2019; Brûlé, 1996). Localization of nanomaterials in polymer blend can be at major/minor phase or at the interface of polymer blend. The uniform distribution of nanomaterials in the major/minor phase of polymer blend improves compatibility through increasing the viscosity of blend and this in turn prevent the coarsening of dispersed phase. In other words, the addition of nanomaterials into a polymer blend significantly improves the consistency between the viscosity of minor phase and viscosity of major phase. However, at the interface localization of nanomaterials the domains (dispersed phases) are surrounded by the nanomaterials. The localization of nanomaterials at the interface of blend enhances compatibility through reducing coalescence of domains. This is because the localization of nanomaterials at interface prevents coalescence between domains. Figures 4A–C display the localization of nanomaterials at the minor phase, major phase, and at the interface of blend, respectively. However, the main factors that affect the selective localization are thermodynamic (polarity and interaction between component of blend) and kinetic (mixing sequence, viscosity of blend, shear rate, and time of mixing). Regarding the thermodynamic factors, if the nanomaterials are attractive to the component of minor phase, the localization of nanomaterials will be at the minor phase. While if the nanomaterials are attractive to the major phase, the localization of nanomaterials will be at the major phase. The localization of nanomaterials can be predicted based on the wetting coefficient expressed in Eq. 2.
Where
The localization of nanomaterials will be in the phase of A (major phase), if the value of wetting coefficient is higher than 1, while if the value of wetting coefficient lower than—1, the localization of nanomaterials will be in the phase of B (minor phase). However, the localization of nanomaterials will be at the interface, if
Moreover, the addition of nanoparticles (nanosilica, nanoclay, carbon nanotubes, and nanofibers) has been documented as a successful approach in terms of enhancing compatibility, thermal stability and mechanical properties of polymers such as naylon 6, polypropylene (PP), polyethylene terephthalate (PET), epoxy, polyethylene (PE) (Loste et al., 2019). Regarding improving mechanical and physical properties of polymers by modifying polymer with nanoclay/nanoparticles, Soleimani et al. (2012), stated that introducing nanoclay of 1%–5% by weigh to polypropylene matrix improved the Young’s modulus by about 36.4% and 17.6% at the temperatures of 25°C and 196°C, respectively. Chan et al. (2011), confirmed that the incorporation of 5% nanoclay to polymer composite enhanced Young’s modulus and tensile strength by about 28% and 25%, respectively. Furthermore, it is reported that the size and density of nanoparticles play a vital role in enhancing properties of polymers. The smaller the diameter of nanoparticles, the higher the surface area, and the better the performance and interface properties of polymer (Ashraf et al., 2018).
On the other hand, processing (mixing) method play an essential role on influencing the mechanical properties of polymers. This is attributed to the heterogeneity between nanocaly and polymers. The common processing of nanoclay/polymer can be classified into solution method, melt mixing, and in situ polymerization. In the solution method, polymer is dissolved using a solvent, then nanoclay are added and mixed with dissolved polymer. Thereafter, the solvent is evaporated. However, in the method of in situ polymerization the nanoclay are mixed within a liquid monomer subjected to heating, followed by the polymerization. In the melt method, polymer is melted, followed by mixing nanoclay with the melted polymer without the use of solvents. The method of melt mixing became interesting due to the high production (Albdiry et al., 2013; Guo et al., 2018; Rafiee and Shahzadi, 2019). Figures 5A–C show the procedures of solution-mixing, melt-mixing, and In-situ polymerization, respectively.
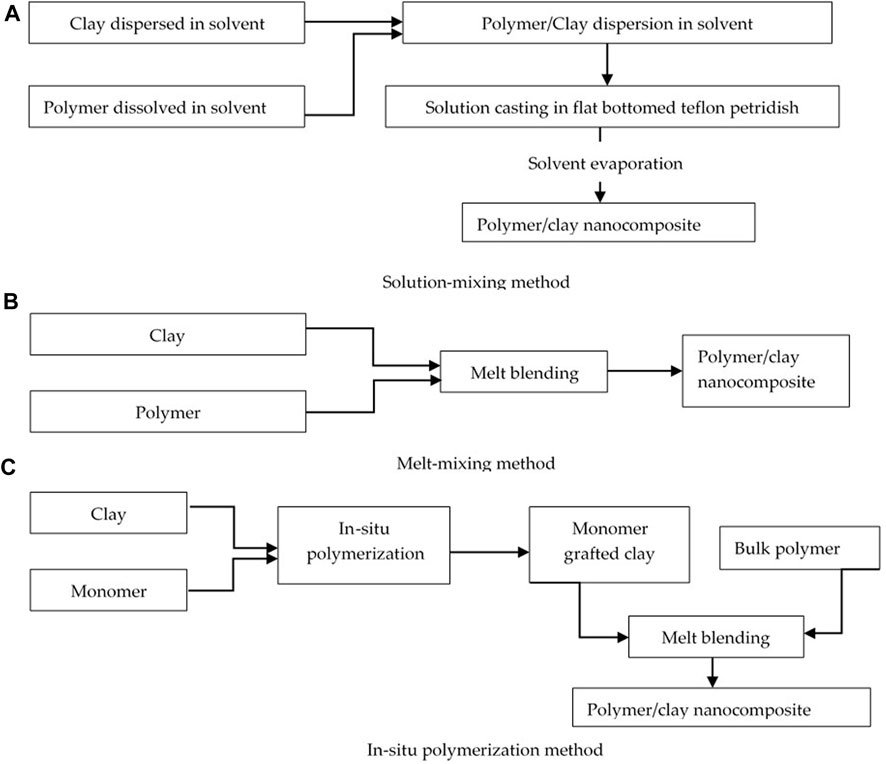
FIGURE 5. Processing Methods of modifying polymer with nanoclay (Guo et al., 2018).
On the other hand, it is reported that the use of nanoclay/nanoparticles in polymer-modified bitumen significantly improves compatibility and homogeneity between polymers and binder (Munera and Ossa, 2014; Porto et al., 2019; Behnood and Gharehveran, 2019; Brûlé, 1996). This is because nanoparticles decreases the interfacial tension between polymer and bitumen, in which the addition of nanoparticles leads to break up the size of separated particles into small sizes, this in turn decrease the interfacial tension (Ashraf et al., 2018).
3.3.3.2 Cross-linking agents
A unit of polymer containing hundreds/thousands of monomers. However, through the process of polymerization the structure of polymer can be classified into linear polymers, cross-linked polymers, branched polymers, and networked polymers (Damiati et al., 2022). Figure 6 displays the types of polymerization. Linear polymers such as thermoplastic are characterized by their long chains, these long chains are held together by hydrogen bonding. However, bonding of linear polymers is susceptible to break by heat. Branched polymers such as thermoplastic are created by the addition of short chains through replacing monomers by covalently-bonded. However, branched polymers chains distinguished by the low density as compared to linear polymers and the susceptibility to break by heating. Otherwise, cross-linked polymers such as thermosetting are characterized by the strong bond in comparison with linear and branched polymers. This is because the monomers of polymers are held together by covalent bond through the addition of cross-linking agent. Networked polymers are represented by the complex form of network, in which monomers are heavily linked to each other. Networked polymers are characterized by the high resistance to heat (Biesenberger and Sebastian, 1983; Daniels, 1989; Hirao et al., 2005).
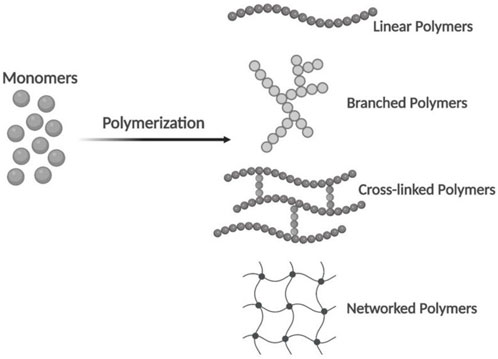
FIGURE 6. Types of polymerization (Damiati et al., 2022).
However, the addition of crosslinking agents to create cross-linked/bonding monomers of polymers are the most common type of polymerization in the applications of polymers. Chemical bond between monomers occurs by the covalent bond, in which the electron pairs between atoms are formed by sharing electrons. Furthermore, the most use of cross-linking agent approach is in the applications of rubber vulcanization, in which the addition of cross-linking agent to rubber leads to improve rigidity (glassy state) of rubber (Bacskay et al., 1997; Huang et al., 2020). In addition, it is proven that adding cross-linking agent to polymers improves thermal stability, physical properties, and mechanical properties. This is because adding cross-linking agent aims to create chemical bonds between monomers/molecules of polymers by covalent bond. Additionally, the content of cross-linking agent play a vital role in the physical, mechanical, thermal properties of polymers (Mane et al., 2015; Guo et al., 2019). The higher the content of cross-linking agent, the higher the density of crosslinking, weight of molecular, viscosity, and glassy state (less flexibility) of polymer (Mane et al., 2015; Hoti et al., 2021). Therefore, the addition of cross-linking agent to a miscible blend leads to the separation due to the increase in the molecular weight of blend (Sarath et al., 2014). However, sulfur is the most common type of cross-linking agents. Sulfur is characterized by its density of 2.07 g/
Otherwise, it is documented that the addition of sulfur to polymer-modified bitumen significantly enhances compatibility. This enhancement may attributed to the increase in density of the polymer component, in which the addition of sulfur creates a chemical bonds/links between molecules of polymer and bitumen. However, the increase in the density of polymer leads to the decrease of separation phase that occurs by the difference in densities between bitumen and polymer.
3.3.3.3 Polymer stabilizers
Thermal and mechanical properties of polymers can be enhanced using chemical additive. Stabilizers are utilized to prevent degradation of polymers during manufacturing or service life of polymers. However, antioxidants are well-known additives that have been utilized in enhancing thermal stability of polymers. Antioxidants can be classified into primary antioxidants and secondary antioxidants (Atta et al., 2017; Zafar et al., 2019; Brito et al., 2021). Primary antioxidants is aimed to remove propagation radicals, free radicals, and peroxy radical (ROO*). This can be done by means of chain breaking donor antioxidant (CB-D) such as BHT, Topanol O, Topanol C, Irganox 1076, Irganox 1010, and Cyanox 2246. However, CB-D is used to react with AH and ROO* in order to produce ROOH and A* (non-radicals products) (Al-Malaika, 1998). The reaction is described below.
Secondary antioxidants are used to prohibit the generation of free radicals (R). This can be done by using decompose hydroperoxides stoichiometrically (PD-S) or decompose hydroperoxides catalytically (PD-C). The reaction below describes how PD-S prevent the generation of free radicals.
Furthermore, sulfur/nanoparticles can be used to enhance thermal stability of polymers because reinforcing polymers with nanoparticles significantly improves the properties of polymers (Chrissafis and Bikiaris, 2011; Senthil Kumar et al., 2018; Terada et al., 2020).
4 Materials and methods
4.1 Approach of study
In this article, the bibliometric analysis was conducted to identify the most contributing authors, organizations, sources, and countries on the polymer-modified bitumen. Furthermore, the trend of published articles of polymer-amended binder was also determined. However, bibliometric analysis was carried out by means of VOSviewer tool (version 1.6.18). The data of bibliometric analysis can be obtained from different sources such as Scopus, Web of Science, PubMed, google scholar, and Dimensions. However, obtaining the data from Scopus and Web of Science requires subscription, while the data from Dimensions, google scholar, and PubMed are obtained free (Alnadish et al., 2023).
4.2 Source of data
In this article, the data of bibliometric analysis were obtained in the form of CSV from Dimensions by searching in the title and abstract for the keywords of “Polymer And bitumen”. The keywords were researched from 1 January 2000 to 31 December 2022.
4.3 Eligibility criteria
In this study, articles such as original articles, review, and letters were considered for bibliometric analysis, while chapters, proceeding, book, monograph and preprint were excluded. Figure 7 displays the approach of selecting documents for bibliometric analysis.
4.4 Data analysis
In this study, the analysis between bibliometric coupling, authors, sources, organizations, and countries was carried out. However, the minimum number of published articles was set to 1, while the resolution parameter and size of cluster was set to 1.
5 Results and discussions
5.1 Bibliometric analysis
5.1.1 Annual publishing
Figure 8 shows the trend of annual publications of utilizing polymers as bitumen modifier. It can be seen in the figure that the published articles on polymer-modified bitumen has been notably increased from 2011 onwards. The highest number of publications was in the year of 2021 with 235 articles, followed by the year of 2022 with 205 articles. However, the number of published articles slightly decreased in the year of 2022 as compared to the published articles in the year of 2011. It can be said that the interest in the use of polymer as binder modifier is increasing exponentially. This is attributed to the benefits of polymer-modified binder in terms of enhancing the physical and rheological properties that enhances the lifespan of modified binder.
5.1.2 Top contributing authors
The outputs of authors, number of documents, citations, and total link strength for the bibliometric analysis of bibliographic coupling versus authors are summarized in Table 4. In addition, the outputs of network visualization is used to highlight the top authors through size of node and link. The larger the size of node and the thicker the links indicate the significant contributing of an author. As highlighted in Table 4; Figure 9, the most contributing authors are Gallegos, C, Giustozzi, Filippo, and Partal, P. In addition, the highest link strength is between Gallegos, C and Partal, P, followed by the link strength between García-Morales, M and Partal, P. However, the link strength indicates the co-occurrence/co-authorship between studies. The higher the strength of link, the more the connections between the content/keywords of studies.
5.1.3 Top contributing sources
The bibliometric analysis for bibliographic coupling versus sources is used to identify the most contributing sources in a specific field. However, the name of sources, number of documents, citations, and total link strength are listed in Table 4, while the network visualization is shown in Figure 10. As can be seen in the table, the sources of Construction and Building Materials, Road Materials and Pavement Design, and Iop Conference Series Materials Science and Engineering are the top three in terms of number of published documents. While the sources of Construction and Building Materials, Fuel, and Road Materials and Pavement Design are the most contributing in terms of number of citations. In addition, the most contributing sources in terms of total link strength are Construction and Building Materials, Road Materials and Pavement Design, and International Journal of Pavement Engineering. However, the large size of node in the network visualization shown in Figure 10 indicates the most contributing sources in terms of number of published articles. The thicker link strength presented in the figure implies the strong connection between the documents of sources.
5.1.4 Top contributing organizations
The most contributing organizations in modifying base binder with polymers is identified by the bibliometric analysis between bibliographic couplings versus organizations. Table 4 and Figure 11 shows the outputs of the bibliometric analysis. As observed in Table 4 and network visualization shown in Figure 11 the most contributing organizations in terms of number of documents are University of Alberta, University of Huelva, and University of Nottingham, while the top organizations in terms of citations are Royal Institute of Technology, University of Alberta, and University of Huelva. However, the top contributing organizations in terms of total link strength are University of Huelva, Rmit University, and Royal Institute of Technology. In addition, the link strength between organizations shown in Figure 11 implies the co-occurrence/co-authorship between published documents from organizations. The thicker the strength of link, the more the relatedness between published documents in terms of content/keywords. Otherwise, it is observed that the most contributing organizations are from the developed countries. Therefore, there should be a salient support for the organizations in developing countries in terms of publishing articles and case studies related to the modification of base bitumen with polymer.
5.1.5 Top contributing countries
The most contributing countries in modifying bitumen with polymer is determined through the bibliometric analysis between bibliographic couplings versus countries. The findings of bibliometric analysis are displayed in Table 4; Figure 12. It can be concluded from Table 4; Figure 12 that the top contributing countries in terms of published documents are Russia, China, and Iran, while the most contributing countries in terms of citations are United Kingdom, Spain, and Canada. The higher the number of citations, the more the impact of documents. However, China, Italy, and Iran are the top contributing countries in terms of total link strength. It can be concluded that the published articles from developing countries still limited as compared to the published documents from developed countries.
5.2 Performance of polymer-modified bitumen
5.2.1 Physical properties of polymer-modified bitumen
The incorporation of polymers with bitumen significantly increases the physical properties of binder in terms of softening point and viscosity. On the contrary, the addition of polymers notably decreases penetration and ductility. It is documented that the addition of SBS to a base binder increased viscosity and softening point, while the penetration and ductility are reduced (Bai, 2017; Hao et al., 2017; Shah and Mir, 2020; Tang et al., 2021; Kong et al., 2021). Similarly, various studied (Zhang et al., 2012; Liang et al., 2017; Li et al., 2021), concluded that modifying a base bitumen with SBR significantly improved the physical properties of aged and unaged binder. However, introducing SIS to a bitumen notably enhanced penetration, softening point, viscosity, and ductility (Kim et al., 2019; Ji et al., 2020; Mazumder et al., 2020). According to the findings of studies (Bachir et al., 2016; Zapién-Castillo et al., 2016; Zhang et al., 2018), SEBS amended-bitumen improved physical properties of unaged and aged modified binder. It is confirmed that the physical properties of bitumen subjected to short-term aging and long-term aging could be enhanced by the addition of EVA (Al-Mansob et al., 2014; Jiang et al., 2017; Keymanesh et al., 2017). Furthermore, adding PE to a base binder produces a modified bitumen with superior physical properties (Yan et al., 2020; Liang et al., 2021; Yan et al., 2021).
5.2.2 Morphological properties of polymer-modified bitumen
Morphology properties are used to identify the size, shape, and structure of polymer-modified bitumen. In addition, the compatibility between polymer and binder is evaluated through morphology properties. Fluorescence microscopy is the common test utilized in the evaluation of morphology properties. It is reported that the addition of polymers to a binder negatively affects the homogeneity between bitumen and polymer due to the difference in the densities. Tang et al. (2021) noticed the incompatibility of 9% SBS-amended binder, in which the structure of polymers was like honeycomb. Furthermore, the concertation of polymers remarkably decreased after subjecting modified binder to short-term aging (RTFO) and long-term aging (PAV). However, authors observed the enhancement in the compatibility and aging resistance of 9% SBS-modified bitumen by introducing 0.15% sulfur. Similarly, Hao et al. (2017) concluded that adding 0.15% sulfur to 4.5% SBS-modified binder significantly enhanced compatibility and decreased degradation of polymer. On the contrary; Zhang et al. (2012) pointed out that modifying base binder with 4% SBR caused incompatibility and subjecting SBR-amended binder to aging significantly decreased the existence of polymers due to degradation. In addition, researchers stated that the addition of 0.03% sulfur improved compatibility, but sulfur is more susceptible to degradation due to aging; Li et al. (2021) confirmed that the incorporation of 4% Sasobit and 4% epoxidized soybean pol (ESO) to 4% SBS-modified binder significantly enhanced homogeneity and resistance to aging. Authors claimed that the addition of Sasobit and ESO maintained structure and concertation of polymers after aging. Liang et al. (2017) confirmed that the addition of 1.5% polyphosphoric acid (PPA) to 3% SBR-modified bitumen notably improved the compatibility. Mazumder et al. (2020) noticed that the addition of 15% SIS to a base binder significantly reduced the honeycomb structure of binder. Researchers stated that the reduction in bee structure is attributed to the dissolution of waxy particles by the addition of SIS. Zapién-Castillo et al. (2016) assured that modifying bitumen with 6% SEBS displayed poor compatibility. However, researchers observed the remarkable enhancement in compatibility after adding 6% nanoclay to SEBS-amended bitumen. Authors stated that the addition of nanoclay balanced the difference in the densities of polymer and bitumen. Al-Mansob et al. (2014) demonstrated that the addition of 6% natural rubber to a base binder showed satisfactory compatibility. Jiang et al. (2017) evaluated the compatibility of bitumen-modified with 5% EVA, 2% SBS, and 0.05% sulfur. The morphological evaluation results exhibited that before aging the modified binder with EVA showed incompatibility between bitumen and polymers, in which the interface of polymer was like honeycomb. However, the addition of SBS to EVA-modified binder improved the compatibility through the appearance of the polymer particles in the form of a continuous filament network. Furthermore, the addition of sulfur to modified bitumen with EVA and SBS displayed the best compatibility. After aging, researchers noticed that the concentration of polymer particles significantly decreased for all modified bitumen. Authors stated that the crosslinked of polymers were destroyed due to aging processes. Yan et al. (2021) indicated that the addition of 4% PE to a base binder displayed poor compatibility. However, authors declared that introducing 4% EVA to PE-modified binder enhanced the compatibility.
5.2.3 Chemicals properties of polymer-modified bitumen
In a study conducted by Hao et al. (2017), the chemical composition of 4.5% SBS-modified bitumen was investigated by means of gel permeation chromatography (GPC). The findings of study demonstrated that subjecting modified binder to short-term aging (RTFTO) and long-term aging (PAV) decreased the molecular weight of polymer. Additionally, the asphaltenes components notably increased, while the maltenes composition decreased after subjecting modified bitumen to RTFTO and PAV. Authors indicated that the decrease in molecular weight is attributed to the degrading of polymer. However, researchers concluded that the addition of 0.15% sulfur to 4.5% SBS-modified binder further reduced the molecular weight of polymers. In similar content, Hu et al. (2020) noticed that subjecting 4.5% SBS-modified binder to PAV decreased percentage molecular weight of polymers from 3.5% to 0.8%, while asphaltenes content increased by about 30%; Tang et al. (2021) observed that subjecting 9% SBS-modified bitumen to PAV significantly decreased the average molecular weight of polymers and increased the average molecular weight of asphaltenes. However, researchers found that the addition of 0.15% sulfur to 9% SBS-modified binder further decreased molecular weight of polymers and increased the average molecular weight of asphaltenes. Authors attributed this change in the average molecular weight to filtration of gel permeation chromatography (GPC) specimens. Bachir et al. (2016) obtained similar findings, in which the addition of 5% SEBS to base bitumen increased the content of asphaltenes before aging and after aging.
5.2.4 Rheological properties of polymer-modified bitumen
The aim of adding polymers to a base binder is to improve the rheological properties. The rheological properties are evaluated through complex shear modulus (G*) and phase angle (δ). Complex shear modulus refers to the resistance of binder to rutting, while phase angle implies the elastic behavior of binder. The higher the complex shear modulus, the better the resistance to rutting. On the contrary, the low phase angle indicates the better elastic behavior. Furthermore, rutting factor (G*/sinδ) and fatigue factor (G*sinδ) are used to assess the resistance of bitumen to rutting and fatigue cracking. The higher the rutting factor the better the resistance to rutting, while the low value of fatigue factor indicates the better resistance of binder to fatigue cracking. However, numerous studies have investigated of modifying a base binder with polymers. In a comparative study conducted by Hu et al. (2020), a base binder was modified with 4.5% SBS, 12% high viscosity modified asphalt (HVMA), and 16% HVMA. However, the rheological properties of modified bitumen subjected to RTFO and PAV were investigated. The findings of study demonstrated that the unaged and aged of 12% and 16% HVMA-modified bitumen showed better complex shear modulus and phase angle than the modified bitumen with 4.5% SBS. Hao et al. (2017) concluded that subjecting 4.5% SBS-modified bitumen to RTFTO and PVA significantly increased complex modulus and reduced phase angle. Authors noticed that the addition of 0.15% sulfur improved complex shear modulus and phase angle. According to Shah and Mir. (2020), the addition of 2% montmorillonite clay (OMMT) to 4% SBS-amended binder notably enhanced rutting factor and fatigue factor. However, the authors noticed that subjecting 4% SBS modified-bitumen decreased rutting and fatigue factor. Researchers confirmed that the addition of OMMT improved rutting and fatigue factor of aged bitumen. Similarly, Zhang et al. (2012) indicated that the phase angle of 4% SBR modified-binder significantly decreased after modified bitumen exposed to PAV; Li et al. (2021) observed that the addition of 4% Sasobit and 6% epoxidized soybean pol (ESO) to 4% SBR amended-bitumen notably enhanced complex shear modulus and phase angle of subjected modified binder to short and long-term aging. Mazumder et al. (2020) confirmed that modifying base binder with 15% SIS significantly improved rutting and fatigue factor as compared to unmodified binder. Bachir et al. (2016) concluded that adding 5% SEBS to a base binder improved complex shear modulus and phase angle before and after aging. In a research conducted by Al-Mansob et al. (2014), natural rubber was introduced to a base binder at different contents 0%–12% in 3% increment by bitumen weight. The study findings displayed that adding natural rubber at content of 6% improved unaged bitumen performance to rutting and fatigue cracking in comparison with unmodified binder. In a comparative study conducted by Jiang et al. (2017) a base binder was modified with EVA, SBS, and sulfur to investigate the impact of utilizing these polymers on the rheological, properties of modified binder. Three types of modified binder were prepared, the first type was prepared by modifying a base bitumen with 5% EVA, the second type was fabricated by amending base binder with 5% EVA and 2% SBS, the third type was equipped by introducing 0.05% sulfur to a bitumen incorporated 5% EVA and 2% SBS. According to dynamic shear rheometer evaluation, the short-term aged modified bitumen with EVA exhibited the highest complex shear modulus and phase angle, followed by the modified bitumen with EVA and SBS, while the modified bitumen with EVA, SBS, and sulfur showed the lowest complex shear modulus and phase angle. However, the higher complex shear modulus indicates the better resistance to rutting, while the lowest phase angle implies the better elastic behavior. Thus, the addition of sulfurificantly improved aging resistance of bitumen. Yan et al. (2021) assessed the performance of modified bitumen with EVA and low-density polyethylene (LDPE). In the study, EVA was introduced to a base binder at different proportions 0, 4, 6, 8% by binder weight. Furthermore, EVA modified-binder was also amended with 4% of LDPE. Researches noticed that the unaged modified bitumen with 4% LDPE showed the best complex shear modulus, phase angle, followed by the modified bitumen with 4% LDPE and 8% EVA. Senise et al. (2017) evaluated the performance of different polymers modified-bitumen (3% SBS/0.05% sulfur, 3% EVA/0.05% sulfur, and 3% EBA/0.05% sulfur). The findings of study demonstrated that SBS/sulfur showed the best complex shear modulus and phase angle, followed by EBA/sulfur, and EVA/sulfur modified bitumen.
5.2.5 Low-temperature performance of polymer-modified bitumen
The low-temperature performance of bitumen are evaluated by means of bending beam rheometer (BBR) and direct tension tester (DTT). The parameters of relaxation ratio (m-value) and elongation are used to identify the performance of bitumen at low temperature. The higher the m-value and elongation, the better the resistance of binder to cracking at low temperature. However, several studies investigated the performance of polymer-modified bitumen at low temperature. Liang et al. (2021) investigated low-temperature performance of bitumen modified with 3% SBR and different proportions of polyphosphoric acid (PPA) i.e. 0%–2% in 0.5% increment. The findings of bending beam rheometer test showed that the base binder at low temperature exhibited higher stiffens and lower relaxation rate (m-value) than the modified binder, while the addition of 3% improved the performance of binder at low-temperature, in which relaxation rate (m-value) was increased. Researchers stated that the optimum content of PPA is 1.5% by binder weight. Mazumder et al. (2020) indicated that modifying a base binder with 15% SIS displayed superior performance at low-temperature, in which the relaxation ratio (m-value) of 15% SIS modified-bitumen remarkably increased. Zhang et al. (2018) noticed that adding 0.8% PPA to 4% SEBES significantly enhanced m-value of aged and unaged bitumen. In a comparative study conducted by Jiang et al. (2017), a base binder was modified with EVA, SBS, and sulfur to investigate the impact of utilizing these polymers on the low-temperature performance of binder. Three types of modified binder were prepared. The first type was prepared by modifying base bitumen with 5% EVA, the second type was fabricated by amending base binder with 5% EVA and 2% SBS, the third type was equipped with introducing 0.05% sulfur to bitumen incorporated 5% EVA and 2% SBS. The low-temperature assessment findings demonstrated that the unaged and aged modified binder with EVA and SBS displayed the highest m-value, followed by the modified bitumen with EVA, SBS, and sulfur. Yan et al. (2021) assessed the low-temperature performance of modified bitumen with EVA and low-density polyethylene (LDPE). In the study, EVA was introduced to a base binder at different proportions, i.e. 0, 4, 6, 8% by binder weight. Thereafter, EVA modified binder was also amended with 4% of LDPE. Authors observed that unaged modified bitumen with 4% LDPE showed the best m-value, followed by the modified bitumen with 4% LDPE and 8% EVA. Table 5 summarizes the findings of the literature review.
5.2.6 Compatibilization of polymer-modified bitumen
Incompatibility is considered as the main drawback of polymer-amended bitumen However; different strategies have been used to decrease the incompatibility of polymer-modified bitumen such as adding waste oil, nanomaterials/nanoclay, sulfur, wax, polymers, and compatibilizers (reactive elastomeric terpolymer, PE-g-MA). It is documented that the addition of waste oil into polymer-amended bitumen significantly improves compatibility, aging resistance, and low-temperature performance. This is because adding oil reduces the viscosity of the major phase (bitumen) in the blend, which, in turn, decreases the phase separation. In addition, introducing oil to a modified binder with polymers reduces viscosity, softening point, rutting factor, and increases penetration, elastic recovery, and ductility. This is attributed to the improvement of elastic behavior of blend, which, in turn, increases the resistance of polymer blend to aging and improves low-temperature performance (Nie et al., 2020; Ren et al., 2021). Otherwise, the decrease in the performance of polymer-amended bitumen incorporating oil is attributed to the fact that the introduction of oil softens the blend. Furthermore, it is proven that adding nanomaterials such as (nanoclay, nanosilica, nano-ZnO, nano-TiO2, and nano-CaCO3) to a polymer-modified binder improves compatibility, aging resistance, physical properties, and rheological properties. The improvement in the compatibility of polymer-amended binder is attributed to the reduction in dispersed phase by decreasing the interfacial tension (Luo et al., 2017; Peng et al. 202; Zhang et al., 2016). Moreover, introducing EVA to SBS/GTR/PE-amended base binder significantly enhances compatibility, physical properties, and rheological properties of the modified binder.
5.3 Discussions
This study aimed to conduct a bibliometric analysis and review on the applications of polymers as bitumen modifiers. However, the purpose of bibliometric analysis is to discover the trend and evolutionary of scientific research in a specific field. According to the trend of published documents, there is a notable increase in the number of published articles since the year of 2010 and forward. The remarkable increase in the published articles in terms of polymer-modified bitumen indicates the growing interest in modifying bitumen with polymer. This is because the addition of polymer into a base binder significantly improves the performance of the asphalt layer, and this in turn leads to protect environment and minimize the cost of repairing. Furthermore, according to the outputs of the bibliometric analysis, the top three contributing sources in terms of number of published documents are Construction and Building Material, Road Materials and Pavement Design, and Iop Conference Series Materials Science and Engineering, with the number of 162, 71, and 65, respectively. However, there should be an inspirational encouragement in terms of publishing documents related to the performance of polymer-amended bitumen through launching special issues. On the other hand, the outputs of the bibliometric analysis showed that the top contributing organizations and countries based on the number of published documents are University of Huelva, University of Alberta, University of Nottingham, China, Russia, and Iran, respectively. This indicates that the developed countries are the most contributing in terms of the published articles. The low number of published researches from developing countries as compared to developed countries may be due to a lack of interest in publishing findings or modifying bitumen with polymer. Thus, there should be a constant stimulation for universities in developing to impose the culture of modifying bitumen with polymers through clarification the benefits of polymer-amended bitumen with regard to saving natural sources and minimizing costs of repairing/rehabilitation of the asphalt layer. Furthermore, there should be a premium support for the universities that suffer from a lack of equipment in developing countries. In addition, strenuous efforts should be made in promoting universities in developing countries to publish articles and case studies with regard to performance of polymer-modified bitumen such as organizing scientific conferences and launch special issues with premium offers.
On the other hand, another objective of this study is to introduce a review on the applications of polymers in bitumen. According to the review studies, the addition of polymer into a base binder notably enhances physical properties, rutting resistance, and fatigue resistance of modified binder. This is because the addition of polymer stiffs the bitumen, which, in turn, improve the performance of modified bitumen at high temperatures. Otherwise, the main drawbacks of amending bitumen with polymers are incompatibility, degradation, and low-temperature performance. The incompatibility of polymer-amended bitumen is occurred by the difference in polarity and densities, in which the component of polymer is not attractive to bitumen, while the difference in densities may make polymer compositions floats or sinks in the liquid of bitumen. However, the degradation of polymer-modified bitumen is attributed to the phenomenon of aging. The increase in temperature of mixing/storing and the exposure of polymer-modified bitumen to oxygen, light of Sun, and environmental factors lead to aging/degradation of polymer-amended bitumen. Furthermore, the addition of polymer to a base binder may negatively affects the low-temperature performance of modified bitumen; this is because modifying bitumen with polymer stiffs the bitumen, which, in turn, decreases the elastic behavior of amended bitumen (Zhang et al., 2012; Bai, 2017; Hao et al., 2017; Liang et al., 2017; Kim et al., 2019; Ji et al., 2020; Mazumder et al., 2020; Shah and Mir. 2020; Li et al., 2021; Tang et al., 2021). Otherwise, it is proven that the addition of waste oil to polymer-modified binder significantly enhances compatibility and resistance to aging. This is because the introduction of oil to polymer-amended bitumen decreases the viscosity of the blend and improves elasticity of blend. This in turn notably reduces the phase separation of the blend. In addition, the addition of nanomaterials to polymer-modified improves compatibility, resistance to aging, and resistance to degradation. This is because the addition of nanomaterials reduces the domain/dispersed phase through decreasing interfacial tension between components of blend. Additionally, nanomaterials work as reinforced materials, which, in turn, improve the performance of polymer in terms of resistance to degradation (Luo et al., 2017; Peng et al. 202; Zhang et al., 2016; Nie et al., 2020; Ren et al., 2021). However, Table 5 summarizes the findings of the review studies.
6 Research gaps and recommendations
The factors of processing/mixing protocol (mixing sequence, viscosity of blend, shear rate, and time of mixing) play a major role on quality of compatibility and performance of nanoparticles-modified polymer/bitumen. The proper the sequence of blending, longer time of mixing, lower viscosity of blend, and higher shear rate, the better the compatibility of blend. However, in accordance with the review studies, the addition of nanomaterials/sulfur to polymer-modified bitumen contributes toward the improvement of compatibility. In most studies, modifying bitumen with polymers and nanomaterials is started by blending bitumen with polymer, and then nanomaterials are introduced to the blend of polymer-amended bitumen. However, the preparation of polymer-modified bitumen is done by mixing bitumen with polymer at high mixing temperature 170°C–180°C by means of high shear mixer at a shear rate of 500–5000 RPM for 30–90 min. Thereafter, nanomaterials are added to the blend of polymer and bitumen, followed by blending with the same conditions of mixing polymer with bitumen in terms of mixing temperature, shear rate, and blending time. Therefore, there is a lack of information in terms of studying the effect of preparation protocol of the addition of nanomaterials into polymer-amended bitumen. This is because homogeneity/compatibility is highly affected by mixing sequence, viscosity of blend, shear rate, and time of mixing.
On the other hand, incompatibility/separation between polymer and bitumen occurs by the differences in the densities, in which the lighter additive floats to the top of stored bitumen at elevated temperature and denser modifier sinks to the bottom of stored bitumen. However, according to ASTM D7173, the compatibility/separation in polymer-modified bitumen is assessed through placing a filled aluminum tube (25 mm in diameter and 125–140 mm in length) with 50 g of bitumen vertically in an oven at a temperature of 163°C ± 5°C for 48 h, followed by placing the aluminum tube in a freezer at a temperature of 10°C ± 10°C for 4 h. Subsequently, the aluminum tube is then cut into three equal pieces. Thereafter, the difference between softening point of the top piece and the bottom piece is measured, while the centerpiece is discarded. It is recommended that the difference in softening point should be less than 2.2°C, in which polymer-modified binder is considered compatible. However, it is reported that the addition of polymer to a base binder shows poor compatibility because polymer floats to the top of aluminum tube. Therefore, the softening point of the top aluminum tube piece is much higher than the softening point of the bottom piece. Otherwise, it is reported that introducing nanoclay to polymer-modified bitumen significantly increase the softening point of the aluminum tube bottom as compared to the softening aluminum tube top (Zou et al., 2017). This is because the sinks of nanocaly enhances the compatibility. This enhancement may attributed to the dives of nanoclay to bottom of aluminum tube because nanoclay are denser than binder, while polymer floats to the top of aluminum tube because of its low density. Thus, the softening point at the bottom of aluminum tube is enhanced due to nanoclay, and the difference between softening point between the top and bottom of aluminum is minimized. Therefore, it can be said that the softening point of aluminum tube centerpiece should be evaluated and compared with softening point of top and bottom of aluminum tube.
Additionally, density of modifier play a vital role in terms of compatibility. In other words, the modifier will float if its density less than that of bitumen, while the modifier will sink if the modifier density is higher than that of bitumen, and this will make significant changes in the performance of polymer-modified bitumen. Therefore, the density of polymer should be equal to that of bitumen to avoid the separation by the change in densities. However, it is difficult to obtain a polymer with a density similar to that of binder. Therefore, the density of polymer can be obtained by means of blending polymer with another polymer or nanomaterials. The density of polymer blend can be predicted using the equation expressed below. This equation aims to identify the density of composite materials.
Where Gsb is specific gravity of composite material,
Clearly, if bitumen needs to be amended with the polymer of LDPE and nanomaterials, in which the density of LDPE is 0.92 g/
Similarly, the density of a polymer can be amended by adding another type of polymer, i.e., density of LDPE (0.92 g/
Furthermore, it should be noted that mixing protocols (mixing sequence, temperature of blending, time of mixing, and share rate) play a vital role in the quality of compatibility of blend. Regarding the sequence of mixing, the less attractive components of blend should be blended together first, followed by blending the premixed components with the attractive components.
Based on the gaps mentioned above, the following recommendations are proposed.
• Effects of mixing sequence on the compatibility of blend should be investigated. In other words, the first blend should be started by modifying bitumen with nanomaterials, followed by the addition of polymer to the blend. Similarly, the second blend should be started by the mixing polymer with nanomaterials, followed by introducing bitumen to the blend. The third blend should be started by mixing bitumen with polymer, followed by the addition of nanomaterials. Thereafter, a comparative analysis should be done for the three blends to identify the suitable mixing sequence that achieve the best compatibility.
• Different mixing temperature for the blend should be studied to identify the appropriate mixing temperature that produce a compatible blend. In addition, the effects of the share rate and mixing time on the compatibility of polymer-amended bitumen should be investigated.
• Density of additive should be taking into account to avoid separation by the difference in densities. However, the density of modifier should be amended to be similar to that of bitumen by adding polymers/nanomaterials. Moreover, the softening point of aluminum tube centerpiece should be measured to introduce a better evaluation for compatibility between polymer-modified binder and nanomaterials/nanoclay.
• The use of antioxidants in polymer-modified binder should be studied. Furthermore, the performance of modified bitumen with high thermal stable property of plastics such as polyetherimide (PEI), polyether ether ketone (PEEK), polybenzimidazole (PBI), and polytetrafluoroethylene (PTFE) should be evaluated.
7 Conclusion
This study aimed to introduce a bibliometric analysis and review on the polymer-modified bitumen. However, the conclusions of the article can be stated as follows.
• The trend of annual publishing in a steady increase, in particular for the last 7 years. Additionally, the most contributing organizations and countries are from the developed countries.
• The addition of polymer to a base binder notably enhance physical and rheological properties of binder. Otherwise, introducing polymer to the base binder negatively affects compatibility.
• Subjecting polymer-modified bitumen to aging leads to the degradation of polymer. In addition, elastic behavior of amended bitumen with polymers decreases by aging.
• The addition of waste oil into polymer-amended binder significantly improves compatibility and aging resistance of modified binder. Additionally, introducing nanoparticles/sulfur to polymer-amended binder significantly enhances compatibility.
Author contributions
AA: conceptualizing, analysis, investigation, and writing—original draft. HK: editing and reviewing. MI and NM, editing, and reviewing. YG: editing, reviewing, and funding. All authors contributed to the article and approved the submitted version.
Acknowledgments
The authors extend their appreciation to Lulea University of Technology for the financial and technical support.
Conflict of interest
The authors declare that the research was conducted in the absence of any commercial or financial relationships that could be construed as a potential conflict of interest.
Publisher’s note
All claims expressed in this article are solely those of the authors and do not necessarily represent those of their affiliated organizations, or those of the publisher, the editors and the reviewers. Any product that may be evaluated in this article, or claim that may be made by its manufacturer, is not guaranteed or endorsed by the publisher.
References
Ajitha, A. R., Mathew, L. P., and Thomas, S. (2020). “Compatibilization of polymer blends by micro and nanofillers,” in Compatibilization of polymer blends (Amsterdam, Netherlands: Elsevier), 179–203.
Ajitha, A. R., and Thomas, S. (2020a). Compatibilization of polymer blends. Amsterdam, Netherlands: Elsevier, 640.
Ajitha, A. R., and Thomas, S. (2020b). “Introduction: Polymer blends, thermodynamics, miscibility, phase separation, and compatibilization,” in Compatibilization of polymer blends (Amsterdam, Netherlands: Elsevier), 1–29.
Albdiry, M. T., Yousif, B. F., Ku, H., and Lau, K. T. (2013). A critical review on the manufacturing processes in relation to the properties of nanoclay/polymer composites. J. Compos. Mater. 47 (9), 1093–115.
Al-Malaika, S. (1998). “Antioxidants: An overview,” in Plastics additives: An AZ reference. United Kingdom: Springer, 55–72.
Al-Mansob, R. A., Ismail, A., Alduri, A. N., Azhari, C. H., Karim, M. R., and Yusoff, N. I. M. (2014). Physical and rheological properties of epoxidized natural rubber modified bitumens. Constr. Build. Mater. 63, 242–248. doi:10.1016/j.conbuildmat.2014.04.026
Alnadish, A. M., Aman, M. Y., Katman, H. Y. B., and Ibrahim, M. R. (2020b). Laboratory assessment of the performance and elastic behavior of asphalt mixtures containing steel slag aggregate and synthetic fibers. Int. J. Pavement Res. Technol. 14, 473–481. doi:10.1007/s42947-020-1149-y
Alnadish, A. M., Aman, M. Y., Katman, H. Y. B., and Ibrahim, M. R. (2021). Characteristics of warm mix asphalt incorporating coarse steel slag aggregates. Appl. Sci. 11 (8), 3708. doi:10.3390/app11083708
Alnadish, A. M., Aman, M. Y., Katman, H. Y. B., and Ibrahim, M. R. (2020a). Influence of the long-term oven aging on the performance of the reinforced asphalt mixtures. Coatings 10 (10), 953. doi:10.3390/coatings10100953
Alnadish, A. M., Aman, M. Y., Katman, H. Y. B., and Ibrahim, M. R. (2022). Laboratory evaluation of fiber-modified asphalt mixtures incorporating steel slag aggregates. CMC-Computers Mater. Continua 70 (3), 5967–5990. doi:10.32604/cmc.2022.017387
Alnadish, A. M., Singh, N. S. S., and Alawag, A. M. (2023). Applications of synthetic, natural, and waste fibers in asphalt mixtures: A citation-based review. Polymers 15 (4), 1004. doi:10.3390/polym15041004
Ashraf, M. A., Peng, W., Zare, Y., and Rhee, K. Y. (2018). Effects of size and aggregation/agglomeration of nanoparticles on the interfacial/interphase properties and tensile strength of polymer nanocomposites. Nanoscale Res. Lett. 13 (1), 214–217. doi:10.1186/s11671-018-2624-0
Atta, E. M., Mohamed, N. H., and Silaev, A. A. A. (2017). Antioxidants: An overview on the natural and synthetic types. Eur. Chem. Bull. 6 (8), 365–375. doi:10.17628/ecb.2017.6.365-375
Bachir, D. S., Dekhli, S., and Mokhtar, K. A. (2016). Rheological evaluation of ageing properties of SEBS polymer modified bitumens. Period. Polytech. Civ. Eng. 60 (3), 397–404. doi:10.3311/ppci.7853
Bacskay, G. B., Reimers, J. R., and Nordholm, S. (1997). The mechanism of covalent bonding. J. Chem. Educ. 74 (12), 1494. doi:10.1021/ed074p1494
Bai, M. (2017). Investigation of low-temperature properties of recycling of aged SBS modified asphalt binder. Constr. Build. Mater. 150, 766–773. doi:10.1016/j.conbuildmat.2017.05.206
Behnood, A., and Gharehveran, M. M. (2019). Morphology, rheology, and physical properties of polymer-modified asphalt binders. Eur. Polym. J. 112, 766–791. doi:10.1016/j.eurpolymj.2018.10.049
Behzadfar, E., and Hatzikiriakos, S. G. (2013). Viscoelastic properties and constitutive modelling of bitumen. Fuel 108, 391–399. doi:10.1016/j.fuel.2012.12.035
Beyler, C. L., and Hirschler, M. M. (2002). Thermal decomposition of polymers. SFPE Handb. fire Prot. Eng. 2 (7), 111–131.
Biesenberger, J. A., and Sebastian, D. H. (1983). “Principles of polymerization engineering,” in Princ of polym eng (Hoboken, NJ: John Wiley and Sons).
Boczkaj, G., Fernandes, A., and Gągol, M. (2017). “Studies on treatment of bitumen effluents by means of advanced oxidation processes (AOPs) in basic pH conditions,” in Frontiers in wastewater treatment and modelling: Ficwtm 2017 (Cham: Springer International Publishing), 1, 331–336.
Brasileiro, L., Moreno-Navarro, F., Tauste-Martínez, R., Matos, J., and Rubio-Gámez, M. D. C. (2019). Reclaimed polymers as asphalt binder modifiers for more sustainable roads: A review. Sustainability 11 (3), 646. doi:10.3390/su11030646
Brito, J., Hlushko, H., Abbott, A., Aliakseyeu, A., Hlushko, R., and Sukhishvili, S. A. (2021). Integrating antioxidant functionality into polymer materials: Fundamentals, strategies, and applications. ACS Appl. Mater. Interfaces 13 (35), 41372–41395. doi:10.1021/acsami.1c08061
Brûlé, B. (1996). Polymer-modified asphalt cements used in the road construction industry: basic principles. Transp. Res. Rec. 1535 (1), 48–53.
Bulatović, V. O., Rek, V., and Marković, K. J. (2012). Polymer modified bitumen. Mater. Res. innovations 16 (1), 1–6. doi:10.1179/1433075x11y.0000000021
Chan, M. L., Lau, K. T., Wong, T. T., Ho, M. P., and Hui, D. (2011). Mechanism of reinforcement in a nanoclay/polymer composite. Compos. Part B Eng. 42 (6), 1708–1712. doi:10.1016/j.compositesb.2011.03.011
Chen, C. C., and White, J. L. (1993). Compatibilizing agents in polymer blends: Interfacial tension, phase morphology, and mechanical properties. Polym. Eng. Sci. 33 (14), 923–930. doi:10.1002/pen.760331409
Chrissafis, K., and Bikiaris, D. (2011). Can nanoparticles really enhance thermal stability of polymers? Part I: An overview on thermal decomposition of addition polymers. Thermochim. Acta 523 (1-2), 1–24. doi:10.1016/j.tca.2011.06.010
Claine, P. J. (1984). Chemical composition of asphalt as related to asphalt durability: State of the Art. Transp. Res. Rec. 999, 13–30.
Damiati, L. A., El-Yaagoubi, M., Damiati, S. A., Kodzius, R., Sefat, F., and Damiati, S. (2022). Role of polymers in microfluidic devices. Polymers 14 (23), 5132. doi:10.3390/polym14235132
Fakirov, S. (2006). Handbook of condensation thermoplastic elastomers. Hoboken, NJ: John Wiley and Sons.
Fred-Ahmadu, O. H., Bhagwat, G., Oluyoye, I., Benson, N. U., Ayejuyo, O. O., and Palanisami, T. (2020). Interaction of chemical contaminants with microplastics: Principles and perspectives. Sci. Total Environ. 706, 135978. doi:10.1016/j.scitotenv.2019.135978
Goodrich, J. L., Goodrich, J. E., and Kari, W. J. (1986). “Asphalt composition tests: Their application and relation to field performance,” in 65th Annual Meeting of the Transportation Research Board, Washington, January 13-16, 1986.
Gu, L., Chen, L., Zhang, W., Ma, H., and Ma, T. (2019). Mesostructural modeling of dynamic modulus and phase angle master curves of rubber modified asphalt mixture. Materials 12 (10), 1667. doi:10.3390/ma12101667
Guo, F., Aryana, S., Han, Y., and Jiao, Y. (2018). A review of the synthesis and applications of polymer–nanoclay composites. Appl. Sci. 8 (9), 1696. doi:10.3390/app8091696
Guo, H., Yue, L., Rui, G., and Manas-Zloczower, I. (2019). Recycling poly (ethylene-vinyl acetate) with improved properties through dynamic cross-linking. Macromolecules 53 (1), 458–464. doi:10.1021/acs.macromol.9b02281
Gupta, R. K., Dunderdale, G. J., England, M. W., and Hozumi, A. (2017). Oil/water separation techniques: A review of recent progresses and future directions. J. Mater. Chem. A 5 (31), 16025–16058. doi:10.1039/c7ta02070h
Hao, G., Huang, W., Yuan, J., Tang, N., and Xiao, F. (2017). Effect of aging on chemical and rheological properties of SBS modified asphalt with different compositions. Constr. Build. Mater. 156, 902–910. doi:10.1016/j.conbuildmat.2017.06.146
He, Y., Li, H., Xiao, X., and Zhao, X. (2021). Polymer degradation: Category, mechanism and development prospect. E3S Web Conf. 290, 01012. doi:10.1051/e3sconf/202129001012
Hirao, A., Hayashi, M., Loykulnant, S., Sugiyama, K., Ryu, S. W., Haraguchi, N., et al. (2005). Precise syntheses of chain-multi-functionalized polymers, star-branched polymers, star-linear block polymers, densely branched polymers, and dendritic branched polymers based on iterative approach using functionalized 1, 1-diphenylethylene derivatives. Prog. Polym. Sci. 30 (2), 111–182. doi:10.1016/j.progpolymsci.2004.12.002
Hoti, G., Caldera, F., Cecone, C., Rubin Pedrazzo, A., Anceschi, A., Appleton, S. L., et al. (2021). Effect of the cross-linking density on the swelling and rheological behavior of ester-bridged β-cyclodextrin nanosponges. Materials 14 (3), 478. doi:10.3390/ma14030478
Hu, M., Sun, G., Sun, D., Zhang, Y., Ma, J., and Lu, T. (2020). Effect of thermal aging on high viscosity modified asphalt binder: Rheological property, chemical composition and phase morphology. Constr. Build. Mater. 241, 118023. doi:10.1016/j.conbuildmat.2020.118023
Huang, S., Kong, X., Xiong, Y., Zhang, X., Chen, H., Jiang, W., et al. (2020). An overview of dynamic covalent bonds in polymer material and their applications. Eur. Polym. J. 141, 110094. doi:10.1016/j.eurpolymj.2020.110094
Ishaq, M. A., Venturini, L., and Giustozzi, F. (2022). Correlation between rheological rutting tests on bitumen and asphalt mix flow number. Int. J. Pavement Res. Technol. 15 (6), 1297–1316. doi:10.1007/s42947-021-00089-z
Jarukumjorn, K. (2007). Compatibilization of recycled high density polyethylene (HDPE)/polyethylene terephthalate (PET) blends. Suranari, Thailand: Suranaree University of Technology.
Ji, B., Lee, S. J., Mazumder, M., Lee, M. S., and Kim, H. H. (2020). Deep regression prediction of rheological properties of SIS-modified asphalt binders. Materials 13 (24), 5738. doi:10.3390/ma13245738
Jia, Y., Cao, R. J., and Li, B. (2005). “Superpave fundamentals reference manual,” in NHI course. United States: Federal Highway Administration.
Jiang, Z., Hu, C., Easa, S. M., Zheng, X., and Zhang, Y. (2017). Evaluation of physical, rheological, and structural properties of vulcanized EVA/SBS modified bitumen. J. Appl. Polym. Sci. 134 (21), 44850. doi:10.1002/app.44850
Kalantar, Z. N., Karim, M. R., and Mahrez, A. (2012). A review of using waste and virgin polymer in pavement. Constr. Build. Mater. 33, 55–62. doi:10.1016/j.conbuildmat.2012.01.009
Keymanesh, M. R., Ziari, H., Damyar, B., and Shahriari, N. (2017). Effect of waste EVA (ethylene vinyl acetate) and waste CR (crumb rubber) on characteristics of bitumen. Petroleum Sci. Technol. 35 (22), 2121–2126. doi:10.1080/10916466.2017.1384840
Kim, H. H., Mazumder, M., Lee, M. S., and Lee, S. J. (2019). Evaluation of high-performance asphalt binders modified with SBS, SIS, and GTR. Adv. Civ. Eng. 2019, 1–11. doi:10.1155/2019/2035954
Kong, P., Xu, G., Chen, X., Shi, X., and Zhou, J. (2021). Effect of different high viscosity modifiers on rheological properties of high viscosity asphalt. Front. Struct. Civ. Eng. 15 (6), 1390–1399. doi:10.1007/s11709-021-0775-z
Koning, C., Van Duin, M., Pagnoulle, C., and Jerome, R. (1998). Strategies for compatibilization of polymer blends. Prog. Polym. Sci. 23 (4), 707–757. doi:10.1016/s0079-6700(97)00054-3
Lesueur, D. (2009). The colloidal structure of bitumen: Consequences on the rheology and on the mechanisms of bitumen modification. Adv. colloid interface Sci. 145 (1-2), 42–82. doi:10.1016/j.cis.2008.08.011
Li, Q., Zhang, H., and Chen, Z. (2021). Improvement of short-term aging resistance of styrene-butadiene rubber modified asphalt by Sasobit and epoxidized soybean oil. Constr. Build. Mater. 271, 121870. doi:10.1016/j.conbuildmat.2020.121870
Liang, M., Xin, X., Fan, W., Zhang, J., Jiang, H., and Yao, Z. (2021). Comparison of rheological properties and compatibility of asphalt modified with various polyethylene. Int. J. Pavement Eng. 22 (1), 11–20. doi:10.1080/10298436.2019.1575968
Liang, P., Liang, M., Fan, W., Zhang, Y., Qian, C., and Ren, S. (2017). Improving thermo-rheological behavior and compatibility of SBR modified asphalt by addition of polyphosphoric acid (PPA). Constr. Build. Mater. 139, 183–192. doi:10.1016/j.conbuildmat.2017.02.065
Liu, F., Zhou, Z., and Zhang, X. (2022). Construction of complex shear modulus and phase angle master curves for aging asphalt binders. Int. J. Pavement Eng. 23 (3), 536–544. doi:10.1080/10298436.2020.1758934
Loste, J., Lopez-Cuesta, J. M., Billon, L., Garay, H., and Save, M. (2019). Transparent polymer nanocomposites: An overview on their synthesis and advanced properties. Prog. Polym. Sci. 89, 133–158. doi:10.1016/j.progpolymsci.2018.10.003
Lu, X., Isacsson, U., and Ekblad, J. (1999). Phase separation of SBS polymer modified bitumens. J. Mater. Civ. Eng. 11 (1), 51–57. doi:10.1061/(asce)0899-1561(1999)11:1(51)
Luo, W., Zhang, Y., and Cong, P. (2017). Investigation on physical and high temperature rheology properties of asphalt binder adding waste oil and polymers. Constr. Build. Mater. 144, 13–24. doi:10.1016/j.conbuildmat.2017.03.105
Mane, S., Ponrathnam, S., and Chavan, N. (2015). Effect of chemical cross-linking on properties of polymer microbeads: A review. Can. Chem. Trans. 3 (4), 473–485. doi:10.13179/canchemtrans.2015.03.04.0245
Mao, H., Yang, Y., Zhang, H., Zhang, J., and Huang, Y. (2020). A critical review of the possible effects of physical and chemical properties of subcritical water on the performance of water-based drilling fluids designed for ultra-high temperature and ultra-high pressure drilling applications. J. Petroleum Sci. Eng. 187, 106795. doi:10.1016/j.petrol.2019.106795
Martinho, F. C., Picado-Santos, L. G., Lemos, F. M., Lemos, M. A. N., and Santos, E. R. (2022). Using plastic waste in a circular economy approach to improve the properties of bituminous binders. Appl. Sci. 12 (5), 2526. doi:10.3390/app12052526
Mazumder, M., Siddique, A., Ahmed, R., Lee, S. J., and Lee, M. S. (2020). Rheological and morphological characterization of Styrene-Isoprene-Styrene (SIS) modified asphalt binder. Adv. Civ. Eng. 2020, 1–13. doi:10.1155/2020/8877371
Mochane, M. J., Sefadi, J. S., Motsoeneng, T. S., Mokoena, T. E., Mofokeng, T. G., and Mokhena, T. C. (2020). The effect of filler localization on the properties of biopolymer blends, recent advances: A review. Polym. Compos. 41 (7), 2958–2979. doi:10.1002/pc.25590
Munera, J. C., and Ossa, E. A. (2014). Polymer modified bitumen: Optimization and selection. Mater. Des. 62, 91–97. doi:10.1016/j.matdes.2014.05.009
Nie, X., Li, Z., Yao, H., Hou, T., Zhou, X., and Li, C. (2020). Waste bio-oil as a compatibilizer for high content SBS modified asphalt. Petroleum Sci. Technol. 38 (4), 316–322. doi:10.1080/10916466.2019.1703741
Nizamuddin, S., Boom, Y. J., and Giustozzi, F. (2021). Sustainable polymers from recycled waste plastics and their virgin counterparts as bitumen modifiers: A comprehensive review. Polymers 13 (19), 3242. doi:10.3390/polym13193242
Nizamuddin, S., Jamal, M., Gravina, R., and Giustozzi, F. (2020). Recycled plastic as bitumen modifier: The role of recycled linear low-density polyethylene in the modification of physical, chemical and rheological properties of bitumen. J. Clean. Prod. 266, 121988. doi:10.1016/j.jclepro.2020.121988
Oliphant, K., Rajalingam, P., Baker, W. E., and La Mantia, F. P. (1993). “Chapter 11—ground rubber tire-polymer composites,” in Recycling of plastic materials. Editor F. P. La Mantia (Toronto, ON, Canada: ChemTec Publishing), 153–170.
Oliviero Rossi, C., Teltayev, B., and Angelico, R. (2017). Adhesion promoters in bituminous road materials: A review. Appl. Sci. 7 (5), 524. doi:10.3390/app7050524
Padaki, M., Murali, R. S., Abdullah, M. S., Misdan, N., Moslehyani, A., Kassim, M. A., et al. (2015). Membrane technology enhancement in oil–water separation. A review. Desalination 357, 197–207. doi:10.1016/j.desal.2014.11.023
Peng, C., Guo, C., You, Z., Xu, F., Ma, W., You, L., et al. (2020). The effect of waste engine oil and waste polyethylene on UV aging resistance of asphalt. Polymers 12 (3), 602. doi:10.3390/polym12030602
Pereira, L., Freire, A. C., da Costa, M. S., Antunes, V., Quaresma, L., and Micaelo, R. (2018). Experimental study of the effect of filler on the ductility of filler-bitumen mastics. Constr. Build. Mater. 189, 1045–1053. doi:10.1016/j.conbuildmat.2018.09.063
Pérez-Lepe, A., Martínez-Boza, F. J., and Gallegos, C. (2005). Influence of polymer concentration on the microstructure and rheological properties of high-density polyethylene (HDPE)-modified bitumen. Energy and fuels 19 (3), 1148–1152. doi:10.1021/ef0497513
Pérez-Lepe, A., Martínez-Boza, F. J., Attané, P., and Gallegos, C. (2006). Destabilization mechanism of polyethylene-modified bitumen. J. Appl. Polym. Sci. 100 (1), 260–267. doi:10.1002/app.23091
Pérez-Lepe, A., Martínez-Boza, F. J., and Gallegos, C. (2007). High temperature stability of different polymer-modified bitumens: A rheological evaluation. J. Appl. Polym. Sci. 103 (2), 1166–1174. doi:10.1002/app.25336
Porto, M., Caputo, P., Loise, V., Eskandarsefat, S., Teltayev, B., and Oliviero Rossi, C. (2019). Bitumen and bitumen modification: A review on latest advances. Appl. Sci. 9 (4), 742. doi:10.3390/app9040742
Prosperi, E., and Bocci, E. (2021). A review on bitumen aging and rejuvenation chemistry: Processes, materials and analyses. Sustainability 13 (12), 6523. doi:10.3390/su13126523
Pszczola, M., Jaczewski, M., Rys, D., Jaskula, P., and Szydlowski, C. (2018). Evaluation of asphalt mixture low-temperature performance in bending beam creep test. Materials 11 (1), 100. doi:10.3390/ma11010100
Pyshyev, S., Gunka, V., Grytsenko, Y., and Bratychak, M. (2016). Polymer modified bitumen. Ukraine: National Aviation University.
Read, J., Whiteoak, D., and Hunter, R. N. (2003). The shell bitumen handbook. Westerkirk, UK: Thomas Telford.
Rafiee, R., and Shahzadi, R. (2019). Mechanical properties of nanoclay and nanoclay reinforced polymers: a review. Polym. Compos. 40 (2), 431–445.
Redelius, P., and Soenen, H. (2015). Relation between bitumen chemistry and performance. Fuel 140, 34–43. doi:10.1016/j.fuel.2014.09.044
Ren, S., Liu, X., Fan, W., Qian, C., Nan, G., and Erkens, S. (2021). Investigating the effects of waste oil and styrene-butadiene rubber on restoring and improving the viscoelastic, compatibility, and aging properties of aged asphalt. Constr. Build. Mater. 269, 121338. doi:10.1016/j.conbuildmat.2020.121338
Robeson, L. (2014). Historical perspective of advances in the science and technology of polymer blends. Polymers 6 (5), 1251–1265. doi:10.3390/polym6051251
Sarath, C. C., Shanks, R. A., and Thomas, S. (2014). “Polymer blends,” in Nanostructured polymer blends (Norwich, NY: William Andrew Publishing), 1–14.
Sengoz, B., and Isikyakar, G. (2008). Evaluation of the properties and microstructure of SBS and EVA polymer modified bitumen. Constr. Build. Mater. 22 (9), 1897–1905. doi:10.1016/j.conbuildmat.2007.07.013
Senise, S., Carrera, V., Navarro, F. J., and Partal, P. (2017). Thermomechanical and microstructural evaluation of hybrid rubberised bitumen containing a thermoplastic polymer. Constr. Build. Mater. 157, 873–884. doi:10.1016/j.conbuildmat.2017.09.126
Senthil Kumar, M. S., Mohana Sundara Raju, N., Sampath, P. S., and Chithirai Pon Selvan, M. (2018). Influence of nanoclay on mechanical and thermal properties of glass fiber reinforced polymer nanocomposites. Polym. Compos. 39 (6), 1861–1868. doi:10.1002/pc.24139
Shah, P. M., and Mir, M. S. (2020). Performance of OMMT/SBS on the rheological properties of asphalt binder. Korea-Australia Rheology J. 32, 235–242. doi:10.1007/s13367-020-0022-5
Shanks, R. A. (2020). “Concepts and classification of compatibilization processes,” in Compatibilization of polymer blends (Amsterdam, Netherlands: Elsevier), 31–56.
Shanks, R. (2012). “Thermoplastic elastomers,” in Thermoplastic elastomers (London, UK: InTech), 137–154.
Sirin, O., Paul, D. K., and Kassem, E. (2018). State of the art study on aging of asphalt mixtures and use of antioxidant additives. Adv. Civ. Eng. 2018, 1–18. doi:10.1155/2018/3428961
Soleimani, N., Khalili, S. M., Farsani, R. E., and Hedayatnasab, Z. (2012). Mechanical properties of nanoclay reinforced polypropylene composites at cryogenic temperature. J. Reinf. Plastics Compos. 31 (14), 967–976. doi:10.1177/0731684412450349
Subhy, A. (2017). Advanced analytical techniques in fatigue and rutting related characterisations of modified bitumen: Literature review. Constr. Build. Mater. 156, 28–45. doi:10.1016/j.conbuildmat.2017.08.147
Tang, N., Huang, W., and Hao, G. (2021). Effect of aging on morphology, rheology, and chemical properties of highly polymer modified asphalt binders. Constr. Build. Mater. 281, 122595. doi:10.1016/j.conbuildmat.2021.122595
Tarefder, R. A., Zaman, A. M., and Uddin, W. (2010). Determining hardness and elastic modulus of asphalt by nanoindentation. Int. J. Geomechanics 10 (3), 106–116. doi:10.1061/(asce)gm.1943-5622.0000048
Teltayev, B., and Radovskiy, B. (2018). Predicting thermal cracking of asphalt pavements from bitumen and mix properties. Road Mater. Pavement Des. 19 (8), 1832–1847. doi:10.1080/14680629.2017.1350598
Terada, N., Kouge, K., Komaguchi, K., Hayakawa, S., and Tsutsumi, H. (2020). Thermal stability change of insoluble sulfur by a heat treatment and its mechanism study. Anal. Sci. 36 (1), 75–79. doi:10.2116/analsci.19sap05
Thenoux, G. (1987). Chemical characterization of asphalt cements using chromatographic techniques: Relation to rheological properties and field performance. Available at: https://ir.library.oregonstate.edu/downloads/sf268776w (Accessed April 30, 1987).
Utracki, L. A., and Wilkie, C. A. (2002). Polymer blends handbook, 1. Dordrecht: Kluwer academic publishers, 2.
Visan, A. I., Popescu-Pelin, G., and Socol, G. (2021). Degradation behavior of polymers used as coating materials for drug delivery—A basic review. Polymers 13 (8), 1272. doi:10.3390/polym13081272
Waheed, M. A., and Oni, A. O. (2015). Performance improvement of a crude oil distillation unit. Appl. Therm. Eng. 75, 315–324. doi:10.1016/j.applthermaleng.2014.10.078
Xie, X., Hui, T., Luo, Y., Li, H., Li, G., and Wang, Z. (2020). Research on the properties of low temperature and anti-UV of asphalt with nano-ZnO/nano-TiO2/copolymer SBS composite modified in high-altitude areas. Adv. Mater. Sci. Eng. 2020, 1–15. doi:10.1155/2020/9078731
Xu, P., Zhu, Z., Wang, Y., Cong, P., Li, D., Hui, J., et al. (2022). Phase structure characterization and compatibilization mechanism of epoxy asphalt modified by thermoplastic elastomer (SBS). Constr. Build. Mater. 320, 126262. doi:10.1016/j.conbuildmat.2021.126262
Xue, Z., Cao, Y., Liu, N., Feng, L., and Jiang, L. (2014). Special wettable materials for oil/water separation. J. Mater. Chem. A 2 (8), 2445–2460. doi:10.1039/c3ta13397d
Yan, K., Chen, J., You, L., and Tian, S. (2020). Characteristics of compound asphalt modified by waste tire rubber (WTR) and ethylene vinyl acetate (EVA): Conventional, rheological, and microstructural properties. J. Clean. Prod. 258, 120732. doi:10.1016/j.jclepro.2020.120732
Yan, K., Hong, Z., You, L., Ou, J., and Miljković, M. (2021). Influence of ethylene-vinyl acetate on the performance improvements of low-density polyethylene-modified bitumen. J. Clean. Prod. 278, 123865. doi:10.1016/j.jclepro.2020.123865
Zafar, M. S., Quarta, A., Marradi, M., and Ragusa, A. (2019). Recent developments in the reduction of oxidative stress through antioxidant polymeric formulations. Pharmaceutics 11 (10), 505. doi:10.3390/pharmaceutics11100505
Zapién-Castillo, S., Rivera-Armenta, J. L., Chávez-Cinco, M. Y., Salazar-Cruz, B. A., and Mendoza-Martínez, A. M. (2016). Physical and rheological properties of asphalt modified with SEBS/montmorillonite nanocomposite. Constr. Build. Mater. 106, 349–356. doi:10.1016/j.conbuildmat.2015.12.099
Zeng, Z. A., Underwood, B. S., and Castorena, C. (2022). Low-temperature performance grade characterisation of asphalt binder using the dynamic shear rheometer. Int. J. Pavement Eng. 23 (3), 811–823. doi:10.1080/10298436.2020.1774766
Zhang, F., Hu, C., and Zhang, Y. (2018). Research for SEBS/PPA compound-modified asphalt. J. Appl. Polym. Sci. 135 (14), 46085. doi:10.1002/app.46085
Zhang, F., Yu, J., and Wu, S. (2012). Influence of ageing on rheology of SBR/sulfur-modified asphalts. Polym. Eng. Sci. 52 (1), 71–79. doi:10.1002/pen.22047
Zhang, H. L., Su, M. M., Zhao, S. F., Zhang, Y. P., and Zhang, Z. P. (2016). High and low temperature properties of nano-particles/polymer modified asphalt. Constr. Build. Mater. 114, 323–332. doi:10.1016/j.conbuildmat.2016.03.118
Zhao, H., Zhang, Q., Wen, X., Wang, G., Gong, X., and Shi, X. (2021). Dual covalent cross-linking networks in polynorbornene: Comparison of shape memory performance. Materials 14 (12), 3249. doi:10.3390/ma14123249
Zhou, T., Zhou, J., Li, Q., and Li, B. (2020). Aging properties and mechanism of microwave-activated crumb rubber modified asphalt binder. Front. Mater. 7, 603938. doi:10.3389/fmats.2020.603938
Zhu, J., Birgisson, B., and Kringos, N. (2014). Polymer modification of bitumen: Advances and challenges. Eur. Polym. J. 54, 18–38. doi:10.1016/j.eurpolymj.2014.02.005
Keywords: polymer-modified bitumen, compatibility, degradation, aging, compatibility improvement methods
Citation: Alnadish AM, Katman HYB, Ibrahim MR, Gamil Y and Mashaan NS (2023) A bibliometric analysis and review on the performance of polymer-modified bitumen. Front. Mater. 10:1225830. doi: 10.3389/fmats.2023.1225830
Received: 19 May 2023; Accepted: 20 June 2023;
Published: 03 July 2023.
Edited by:
Hui Yao, Beijing University of Technology, ChinaReviewed by:
Xinxing Zhou, Shanxi Transportation Technology Research & Development Co., Ltd., ChinaPeiwen Hao, Chang’an University, China
Copyright © 2023 Alnadish, Katman, Ibrahim, Gamil and Mashaan. This is an open-access article distributed under the terms of the Creative Commons Attribution License (CC BY). The use, distribution or reproduction in other forums is permitted, provided the original author(s) and the copyright owner(s) are credited and that the original publication in this journal is cited, in accordance with accepted academic practice. No use, distribution or reproduction is permitted which does not comply with these terms.
*Correspondence: Adham Mohammed Alnadish, adhmalnadish@gmail.com; Yaser Gamil, yaser.gamil@ltu.se