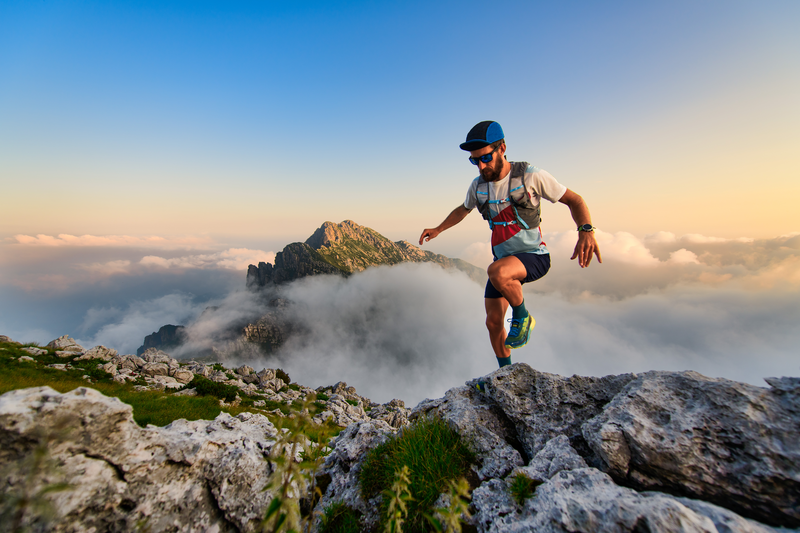
95% of researchers rate our articles as excellent or good
Learn more about the work of our research integrity team to safeguard the quality of each article we publish.
Find out more
ORIGINAL RESEARCH article
Front. Mater. , 05 June 2023
Sec. Biomaterials and Bio-Inspired Materials
Volume 10 - 2023 | https://doi.org/10.3389/fmats.2023.1210728
A correction has been applied to this article in:
Corrigendum: A cytocompatible microporous Sr-doped titanium dioxide coating fabricated by plasma electrolytic oxidation
Titanium has broad application prospects in the field of hard bone tissue repair and replacement due to its low density, an elastic modulus similar to that of human bone, and better biocompatibility than stainless steel and cobalt-based alloys. However, titanium is a biologically inert material with low biological activity. After implantation, it only physically bonds with bone instead of chemically bonding, which causes complications such as implant loosening. In this study, a strontium-doped titanium dioxide coating was prepared on a titanium surface with micro-arc oxidation. Based on a systematic study of the surface properties of the strontium-doped titanium dioxide coating, the biocompatibility and bioactivity of the coating were evaluated with in vitro cell experiments. The results showed that the strontium-doped titanium dioxide coating was successfully prepared on the titanium surface and exhibited strong bonding with the substrate. The coating surface had a porous structure, and the strontium was dispersed on the surface and inside the coating. The strontium ions were released slowly, which improved the corrosion and wear resistance of the titanium. In vitro cell experiments showed that the coating had good cell compatibility; it promoted the adhesion, proliferation and differentiation of MC3T3-E1 cells and exhibited good biological activity. These results showed that preparation of a strontium-doped titanium dioxide coating by micro-arc oxidation is feasible. The coating had a good surface morphology, improved the biological activity of the titanium and has good potential for clinical application.
Titanium and its alloys have excellent mechanical properties, elastic moduli and specific gravities close to those of bone tissue, and good deformation and fatigue resistance. In addition, they also exhibit good biocompatibility; for example, they are not carcinogenic, do not cause allergic reactions, are nontoxic, and resist corrosion by bodily fluids. Therefore, titanium and its alloys are widely used as hard tissue substitutes (for teeth and bone tissue) in medicine (Guillemot et al., 2004; Xue et al., 2020). However, titanium and its alloys are biologically inert and have poor biological activity. After implantation into the human body, they induce foreign body reactions that generate surface layers of encapsulated fibers and hinder new bone generation. This prolongs the cycle of the titanium implant replacement and reduces the success rate of titanium implants (Kaur and Singh, 2019; Attarilar et al., 2020).
To solve the above problems and improve the success rates and repair effects of titanium implants, researchers have tested a variety of surface activation technologies, such as plasma spraying, laser cladding, electrophoretic electrochemical deposition, ion implantation, and magnetron sputtering, to biologically activate the surfaces of titanium implants (Kato et al., 2000; Ong and Chan, 2000; Milella et al., 2001; Ding, 2003; Manivasagam et al., 2021). The purpose of surface activation is to form an ideal interface between the implant and the bone tissue after implantation. Research has shown that the bonding interfaces between titanium implants and bone tissue are mainly divided into two types, namely, fiber bonding systems and osseous bonding systems, and the osseous bonding systems are preferable. At present, plasma spraying of hydroxyapatite (HA) on the surfaces of titanium alloy implants is the most widely used clinical surface activation technique. The resulting coating facilitates direct physical and chemical bone bonding and generates a reliable interface strength with the surrounding bone tissue (Ong and Chan, 2000). However, the coating also has some defects, including poor adhesion between the coating and the metal substrate and the risk of coating detachment after implantation (Ong and Chan, 2017). Moreover, the HA and other active ingredients are quickly degraded by body fluids, and their geometric shapes are not conducive to bonding with complex surfaces.
A new electrochemical technology, micro-arc oxidation (MAO), which is also known as plasma electrolytic oxidation, generates oxide coatings on the surfaces of metals such as aluminum, magnesium, zirconium, and titanium. Compared to other surface modification technologies, MAO has exceptional advantages and has attracted much attention. By applying this process, porous ceramic films with nanoscale roughness are generated in situ on the metal surfaces, and the thicknesses, roughnesses, pore sizes, and chemical compositions of the film layer can be controlled by adjusting the experimental parameters. Therefore, the micromorphology and chemical properties of the metal surface can be modified in one process step (Ribeiro et al., 2015). Research showed that the ceramic membrane can be divided into three layers according to its structural characteristics: the inner layer is a transition layer, which is uneven and conforms with the metal matrix, which is the key factor in achieving high bonding strength between the ceramic layer and the matrix; the middle dense layer is the main body of the ceramic membrane, with a high hardness and wear resistance; and the outermost layer is a loose layer with increased surface roughness (He et al., 2019). The ceramic membrane forms on the substrate by in situ growth in an interlocking manner and exhibits strong bonding with the substrate, so it effectively overcomes the risk of early coating detachment caused by the different coefficients of thermal expansion for the coating and titanium implant and increases the wear resistance and corrosion resistance of the material.
Adjustments of the composition and concentration of the micro-arc oxidation electrolyte also affect the surface performance of the coating, as during the micro-arc oxidation process, the electrolyte participates in the main chemical reaction and contains ions that are deposited and doped into the titanium oxide film through discharge channels. Research has shown that the addition of some inorganic or organic substances to the electrolyte correspondingly improves the biological activity of the oxide film. If Ca and P are added to the electrolyte and undergo micro-arc oxidation reactions, the oxide film promotes cell proliferation and bone integration on the titanium surfaces (Kozelskaya et al., 2022). Adjustments to the micro-arc oxidation voltage can improve the thickness of the film layer and enhance its corrosion resistance and wear resistance.
In recent years, many scholars have proposed surface biofunctionalizations of metal biomedical materials, which are both innovative and challenging. The core idea of this concept is to endow the metal biomedical materials with special biomedical functions while utilizing their excellent mechanical properties to enable the dual functions of mechanical load-bearing and biomedical functioning. Strontium is an important trace element in the human body, and it exists in almost all tissues. The strontium contents in bones and teeth account for 99% of the total amount of strontium in the human body. The effect of strontium on the human body is similar to that of calcium, with a dual effect on bone cells and strong osteotactic properties (Marx et al., 2020). Research has shown that low doses of strontium are beneficial for bones, while low concentrations of strontium inhibit bone resorption and promote bone formation (Lourenço et al., 2019). In vitro studies on cell and tissue cultures showed that strontium enhanced the replication of osteoblasts, increase the number of osteoblasts, improve the differentiation and mineralization abilities of osteoblasts, promote bone formation, stimulate bone tissue formation, reduce the activity of osteoclasts, reduce the number of osteoclasts, and reduce the rate of bone absorption (Offermanns et al., 2018). Due to the osteogenic and anti-osteoporotic effects of strontium, scholars have studied the incorporation of strontium into different bone repair materials and found that strontium played a positive role in the bone repair process (Geng et al., 2018; Geng et al., 2021; Lu et al., 2022).
The preparation of bioactive coatings on the surfaces of titanium implants through surface modifications maintains the mechanical properties of titanium and endows the titanium with bioactivity, which has been an important research topic in recent years. Hydroxyapatite-coated titanium implants are currently used as bioactive materials in clinical practice, but the crystallinities of the coatings are low, and early degradation by bodily fluids occurs readily after implantation. Moreover, the bonding strength between the HA coating and the titanium substrate is low, and there is often a risk of coating peeling after implantation into the body. In addition, the raw material requires high-purity HA powder, which is expensive. These shortcomings seriously restrict widespread application of this coating material in clinical practice, and other coating materials also have similar shortcomings. Therefore, research and development of bioactive coating materials with excellent biological activities and high binding strengths to replace HA coating materials has become a key focus of research in this field.
The main goals for surface modifications of titanium implants are to enhance the surface biological activity of the titanium, improve the bone-promoting performance of the titanium implants, shorten the bone integration time between the orthopedic implants and the surrounding bone tissues, and achieve immediate or early loading of the implant. Strontium has numerous biological activities, and titanium dioxide coatings prepared with MAO have special microporous structures. We envision the introduction of strontium into the titanium surface through MAO so that the titanium surface has integrated structural/biomedical functions. With dual induction of bone formation through microporous structure and strontium release, early bone integration can be achieved between the titanium implant and the bone interface. The aim of this study is to deposit a strontium-doped titanium dioxide active coating on the surface of titanium through micro-arc oxidation and conduct a detailed biological evaluation.
The titanium contents in commercial pure titanium sheets are at least 99.5%. According to the amount of impurities they contain, pure titanium sheets are divided into levels such as TA1, TA2, and TA3. In this study, a TA2 pure titanium sheet, which has moderate mechanical and corrosion resistance, was used. The commercial TA2 pure titanium material was cut into 10 mm pieces with a wire cutting machine tool. A 1 mm × 10 mm small piece was gradually polished with 200–1,200 grit sandpaper and then cleaned with acetone, ethanol, and deionized water during ultrasonic vibration for 10 min. It was then dried at room temperature, sterilized, and disinfected before use.
Calcium glycerophosphate (0.02 mmol/L) and calcium acetate (0.2 mmol/L) were used as the electrolyte solutions, and the prepared samples were labeled MT. Then, 0.05 mmol/L strontium acetate solution was added to the electrolyte, and the prepared samples were labeled MT-Sr. The Ti group was the blank control group. With a self-developed micro-arc oxidation power supply, the electrical parameters for oxidation were set as follows: the frequency was 50 Hz, the time was 6 min, and the voltage was 450 V. Before the micro-arc oxidation discharge process, the cooling system was started, the electrolyte in the electrolytic tank was stirred to maintain a uniform electrolyte concentration, and the temperature was controlled at below 30°C. After the micro-arc oxidation treatment, the titanium sheets were cleaned with deionized water, dried, and disinfected for future use.
Scanning electron microscopy was used to observe the surface morphologies of the samples, while energy spectrum analyses, X-ray diffraction, and X-ray photoelectron spectroscopy were used to observe the elemental compositions, phases, and chemical states of the coatings. Contact angle meter and profiler was used to test the wettability and roughness, and a nanomechanical property testing system was used to test the bonding strength of the coating.
The corrosion resistances of the samples were tested with an electrochemical workstation. A three-electrode system was used. A saturated calomel electrode was the reference electrode, a platinum electrode was the auxiliary electrode, and the test sample was the working electrode. The corrosion solution contained 0.9 wt% NaCl. The sample was immersed in 30 mL of the corrosion solution, stabilized for 60 min, and then subjected to impedance measurements. The scanning speed was 1 mV/s. Three parallel samples were selected from each group to test their corrosion resistances. After each sample was tested, the corrosion solution was replaced before proceeding to test the next sample.
In this study, the round-trip friction function of a nanomechanical performance test system (equipment model NanoTest, Vantage, MML, British) was adopted in the friction and wear experiments. The loads were 50 mN, the 5 Hz tests were conducted for 10 min, and the stroke lengths were 3 mm. The sample was preprocessed in simulated body fluid for 30 min before testing. After the test, the surface was observed with a microscope, and the depths and widths of the scratches were measured with a three-dimensional optical profilometer (Contour GT, Brooke, Germany).
The data for multiple positions were measured separately, the average value was used to calculate the wear volume Wv, and the calculations were performed with Eqs. 1, and 2:
where Wv is the wear volume (mm3), L is the length of the wear scar (mm), h is the depth of the wear scar (mm), and b is the width of the wear scar (mm).
MC3T3-E1 cells from the Shanghai Cell Bank of the Chinese Academy of Science were cultured in a medium containing α-MEM, 1% bispecific antibody and 10% fetal bovine serum. The cells were cultured in a 37°C cell culture chamber containing 5% CO2, and the medium was changed every 2 days.
Live/dead fluorescence staining was used to qualitatively evaluate the cytotoxicities of each group of samples. One milliliter of the cell suspension (2 × 104 cells/cm2) was transferred onto the surfaces of each group of samples, incubated for 3 days, rinsed with sterile PBS, and tested with the Live/Dead Viability/Cytotoxicity test kit. The cytotoxicity of the material was determined through fluorescence microscopy.
To determine the adhesion of cells on the surfaces of each group of materials, fluorescence staining was used to detect the number of adherent cells on the surfaces of the materials after 2 h. The cell suspension (2 × 104 cells/cm2) was inoculated in 24-well plates with samples, incubated for 2 h, and fixed with 4% paraformaldehyde for 30 min. After rinsing with PBS, a DAPI staining agent was added dropwise to the surfaces of the samples to avoid light staining for 5 min. Fluorescence microscopy was used to observe and take photographs, and the number of cells was counted with Image-Pro Plus software.
The cell culture and inoculation methods were the same as before. After 1, 3, and 10 days of culture at 37°C, the nonadherent parietal cells were washed with PBS, and the parietal cells were digested and adhered with trypsin in 96-well plates. After overnight culture, CCK8 solution was added to each well, and the absorbance at 450 nm was detected with a microplate reader.
The cell inoculation density and culture method were the same as above. MC3T3-E1 cells were collected at three time points, 1, 3, and 10 days after inoculation, and real-time quantitative PCR was used to detect the mRNA levels of the osteogenic differentiation-related genes (including ALP, BMP-2, OCN, and COL-I).
The data are presented as the means ± standard deviations and were analyzed using SPSS 16.0 software. One-way ANOVA and SNK tests were used to compare the different groups. A value of p < 0.05 indicates a significant difference, while p < 0.01 indicates a significant difference.
Figure 1 shows the SEM surface morphologies of different samples, and significant morphological differences were observed for the Ti group and the other groups. The surface of Ti was relatively flat, without micropores and residual polishing scratches. The MT group had a typical micro-arc oxidation morphology, and the surfaces were covered with micropores of varying sizes interconnected with large and small pores nested within each other. The micropores were also irregularly shaped, with localized burning marks. Similar to the surfaces of the MT group, the surfaces of the MT-Sr group were covered by pores with irregular shapes and varying sizes.
Figure 1. SEM surface morphologies of different samples under low magnification and high magnification. The oxidation time was 5 min, the voltage was 450 V, and the reactions were run at room temperature.
Figure 2 is the elemental analysis diagram of the MT-Sr. MT-Sr was composed of Ti, Sr, Ca, P, and O, and Sr was doped into the oxidation coating. The Sr, Ca and P came from the electrolyte solution; that is, during the oxidation process, the elements in the electrolyte entered the coating.
Figure 3 shows the XPS spectrum of MT-Sr, while Figure 3A shows the full X-ray photoelectron spectrum of the MT-Sr microporous coating, including the characteristic peaks for Sr, Ti, O, Ca, and P. Similar to the EDS results, strontium was successfully introduced into the coating during the micro-arc oxidation process. The peaks in the Ti 2p spectrum corresponded to titanium dioxide, while Ca 2p and P 2p data indicated the presence of Ca3(PO4)2.
Figure 3. X-ray photoelectron spectroscopy data for MT-Sr; (A) complete XPS scan, (B) Ti 2p, (C) Ca 2p, (D) P 2p, and (E) O 1s spectra.
Figure 4 shows the XRD phases found on the surfaces of different samples. It can be seen from the figure that the coating was mainly composed of rutile-type TiO2, anatase-type TiO2 and pure titanium phases. No diffraction peaks for Sr-containing compounds were detected. We speculate that this may be related to the small amount of Sr incorporated or the uniform distribution in the phase.
Figure 5 shows the contour surface morphologies of the samples. It is obvious that the surface of the Ti control group sample was flat and had a smaller roughness value. Compared with the surface of the Ti control group sample, the surfaces of the MT and MT-Sr samples were more uneven, and their roughness values were significantly increased. The Ra values of the samples were quantitatively analyzed, and the results showed that the roughnesses for each group of samples decreased in the order MT-Sr ≥ MT>Ti. Micro-arc oxidation increased the surface roughnesses of the samples.
Figure 6 shows the contact angles of the samples. The contact angle is one of the indicators used to evaluate the wettabilities of materials. Generally, the larger the surface roughness of a material is, the smaller the contact angle, and the greater the hydrophilicity. In this study, compared with that of the Ti control group, the contact angle for the MT-Sr group was smaller, indicating that micro-arc oxidation increased the hydrophilicity of Ti.
Figure 6. Static contact angles for the Ti, MT and MT-Sr groups and bar chart for the contact angles.
Figure 7 shows the acoustic emission spectrum of the coating measured with a nanoscratch instrument (NanoTest Vantage). In acoustic emission testing of coating adhesion, a load results in the emission of a weak acoustic signal when the coating is scratched or peeled off due to insufficient adhesion between the coating and the substrate. As shown in the acoustic emission spectrum, it can be seen that when the load reaches 129.6N, the acoustic emission signal suddenly increases, indicating that the MT-Sr coating fails under this pressure, and the coating exhibited good bonding with the titanium matrix.
Figure 7. Acoustic emission spectrum for MT-Sr (the initial load was 0 N, the final load was 150 N, the scratch speed was 2 mm/min, the loading rate was 50 N/min and n = 3).
Figure 8 shows wear performance images for the samples. In our study, the size method was adopted to measure the depths and widths of wear marks with a white light interferometer, and then the wear volume Wv and wear rate K were calculated. The results showed that the wear volume Wv of the MT-Sr coating was 0.578 and the wear rate was 4.63×103, while the wear volume Wv of the Ti coating was 0.773 and the wear rate was 6.19×103, which indicated that the MT-Sr coating had excellent wear resistance.
Figure 8. Morphology of the wear scars on Ti, MT and MT-Sr (The loads were 50 mN, the 5 Hz tests were conducted for 10 min, and the stroke lengths were 3 mm).
Figure 9 shows the electrochemical impedance data for each group of samples. Figure 9A shows the Nyquist curves, and all three graphs presented typical semicircular capacitive arcs. The capacitive arcs are related to charge transfer, and the diameters of the capacitive arcs reflect the corrosiveness to a certain extent. The larger the diameter of the capacitive arc is, the greater the hindrance to charge transfer, and the stronger the corrosion resistance of the coating. It is obvious that the capacitance arc diameter for MT-Sr was significantly higher than those for Ti and MT, indicating that MT-Sr had strong corrosion resistance. Figure 9B shows the Bode-phase curves, and the MT-Sr surface exhibited a typical pure capacitive behavior response. Figure 9C shows the Bode-impedance curves. Compared with those for Ti and MT, the low-frequency impedance value for MT-Sr was significantly higher, indicating that MT-Sr had fewer surface defects, did not suffer serious damage in the SBF solution and exhibited strong corrosion resistance. Figure 9D shows typical potential dynamic polarization curves, and the corrosion resistances of the samples improved after the micro-arc oxidation treatments, which was related to the formation of dense TiO2 coatings during the micro-arc oxidation processes.
Figure 9. Electrochemical impedance spectroscopy. Nyquist curves (A), Bode-phase curves (B), Bode-impedance curves (C) and typical potential dynamic polarization curves (D) for Ti, MT and MT-Sr.
Figure 10 shows the live/dead results for staining of the MC3T3-E1 cells on the various sample surfaces. After the cells were inoculated onto the surfaces of the four groups of samples for 24 h, live and dead cell staining was performed. The cells exhibiting green fluorescence were living cells, while the cells exhibiting red fluorescence were dead cells. There were many live cells on the surfaces of each group of samples, with only a small number of dead cells visible. There were no significant differences among the three groups, indicating that none of the sample groups exhibited significant cytotoxicity and that the MT-Sr coating was not significantly cytotoxic and had good cell compatibility.
Figure 10. Live/dead staining results for MC3T3-E1 cells on the different sample surfaces. The data are expressed as the mean ± SD (n = 3). **p < 0.01.
Figure 11 shows the adhesion of MC3T3-E1 cells on the different sample surfaces. The numbers of adherent cells on the surfaces of the different groups decreased in the order MT-Sr > MT>Ti. Compared with those of the Ti and MT groups, the number of adherent cells in the MT-Sr group was significantly higher, indicating that the MT-Sr coating effectively promoted adhesion of the MC3T3-E1 cells.
Figure 11. Adhesion of MC3T3-E1 cells on the different sample surfaces. The data are expressed as the mean ± SD (n = 3). *p < 0.05 and **p < 0.01.
Figure 12 shows the proliferation of MC3T3-E1 cells on the different sample surfaces. It is evident that, similar to cell adhesion, proliferation of the sample cells in each group gradually increased over time. Compared with the Ti and MT groups, the MT-Sr group showed statistically significant higher cell proliferation, which may be closely related to the porous structure and the sustained release of strontium ions.
Figure 12. Proliferation of MC3T3-E1 cells on the different sample surfaces. The data are expressed as the mean ± SD (n = 3). **p < 0.01.
Figure 13 shows the expression levels of osteogenic differentiation-related genes (ALP, BMP-2, OCN, and COL-I) on the surfaces of each group of samples at different time points. The expression of osteogenic differentiation genes on the surfaces of each group of samples gradually increased with time, and the expression of genes in each group decreased in the order MT-Sr > MT>Ti. Compared with those of the Ti and MT groups, the expression of osteogenic differentiation genes in the MT-Sr group was significantly higher, indicating that the MT-Sr coating promoted osteogenic differentiation of the MC3T3-E1 cells.
Figure 13. Expression levels for the osteogenic differentiation-related genes [(A): ALP; (B):BMP; (C): OCN; (D):Col-1]. The data are expressed as the mean ± SD (n = 3). *p < 0.05 and **p < 0.01.
Fracture, wear, and corrosion are the three most common causes of metal failure. After implanting an implant into the human body, the complex physiological environment in the bodily fluid usually accelerates corrosion of the implanted metal. This reduces the mechanical performance of the implant, which leads to the dissociation of metal ions, and it damages the microstructure and biological activity of the implant surface and increases the probability of implant failure. Polarization curves are commonly used in corrosion electrochemical research. In this study, compared with that of Ti, the self-corrosion potentials of MT and MT Sr were shifted positively, indicating that the MT and MT Sr exhibited better chemical stability in the corrosive solution and less corrosion. The corrosion current density is an important parameter used in evaluating corrosion reaction kinetics, and the corrosion current densities and self-corrosion rates of MT and MT-Sr were lower than those of Ti. This explains the self-corrosion potentials, corrosion current densities, and self-corrosion rates showing that MT and MT-Sr were more corrosion resistant than Ti. To determine the corrosion resistances of MT and MT-Sr, the electrochemical impedance spectra of the different samples were measured at the self-corrosion potentials. We found that the impedance values for MT and MT-Sr were greater than that for Ti and presented capacitive arcs. Moreover, the diameters of the capacitive arcs in the MT and MT-Sr impedance spectra were significantly larger than that for Ti, demonstrating good corrosion resistance.
We believe there are two possible mechanisms for the improved corrosion resistance seen after titanium micro-arc oxidation. First, the corrosion reaction rate of the titanium substrate was determined by the electrochemical control step, and the corrosion reaction rate for the titanium micro-arc oxidation film layer was determined by the diffusion controlled step. Based on the polarization curve and impedance test results, it is believed that the micro-arc oxidation film layer on the titanium substrate surface hindered ion transfer from the solution to the electrode surface or corrosion products to the solution, thereby improving the corrosion resistance. Second, the main product formed by titanium micro-arc oxidation was titanium dioxide, which is a ceramic material. The biggest advantage of ceramics is better corrosion resistance. In addition, after micro-arc oxidation, the significant increase in the thickness of the oxide film on the titanium surface also reduced the contact between the titanium substrate and the bodily fluids, which improved the corrosion resistance. In this study, we found that micro-arc oxidation improved the wear resistance of titanium, significantly improved the friction coefficient compared to that of the titanium matrix, and doping with the strontium in the electrolyte increased the friction coefficient further. We believe that the main reason was that the micro-arc oxidation increased the hardness of titanium, which led to an increase in the wear resistance. In addition, the strontium-doped micro-arc oxidation coatings increased the surface roughness of titanium and indirectly improved its wear resistance. Therefore, the application of micro-arc oxidation on the surface of titanium improved the surface morphology and enhanced the corrosion resistance and wear resistance.
The interactions between the surfaces of orthopedic titanium implants and the host tissues are crucial for the long-term success of the implants. The surface characteristics of implants depend on multiple factors, including the surface morphology, chemical energy, roughness, hydrophilicity and chemical composition. These surface characteristics directly affect the interfacial interactions between the implant surface and the bone tissue, thereby affecting the quality and speed of bone integration (Albrektsson and Wennerberg, 2019). There are many methods with which to modify implant surfaces, such as acid etching, hydrothermal treatment, plasma spraying, and anodic oxidation. Micro-arc oxidation is a simple and effective chemical method used to prepare high-strength oxide film coatings bonded to metal substrates. In recent years, it has been a popular research topic in the field of orthopedic implant modifications. It has been reported in the literature that the coatings obtained after micro-arc oxidation treatments of titanium surfaces increased the biocompatibilities and biological activities of osteoblasts (Pan et al., 2019). It has proven effective to adjust the elemental composition and morphology of the coating by adjusting the micro-arc oxidation parameters, such as the voltage, time, duty cycle, and electrolyte composition. In this study, micro-arc oxidation with the addition of strontium to the electrolyte was utilized to form a strontium-doped coating on the surface of titanium through an oxidation reaction. By studying the surface morphology and properties of this coating, a new method was identified for improving the biological activities of orthopedic titanium implants.
The surface of the titanium implant was modified by micro-arc oxidation, and a rough and porous titanium surface was observed after the oxidation reaction. The surface pores acted as discharge channels for the micro-arc oxidation. A plasma oxide film was formed during the plasma treatment, and in a short time, the discharge channels reached high temperatures and high pressures, and the reactants entered the channels through diffusion and underwent oxidation. The oxides sprayed from the discharge channels reached the surface of the coating, which rapidly solidified due to the “cold quenching” effect of the electrolyte, and this increased the coating thicknesses in the local areas near the discharge channels. As the discharge channels cooled, the reaction products were deposited on the inner walls of the channels, and this deposition was accompanied by rapid exothermic volume expansion. As the oxidation reaction continued, the entire coating underwent uniform thickening (Li et al., 2018). Additionally, during the oxidation process, large amounts of gas escaped to form a loose and porous surface structure, which enabled adhesion and proliferation space for binding of the osteoblasts, which enabled the growth of bone tissue toward the pores and integration of the bone formed between the implant and the bone tissue.
Adhesion between the coating and substrate affects the stabilities of implants. Good adhesion with the surrounding bone tissue stabilizes the implant, which is the foundation of implant bone integration and the key to success of the implant. Research has shown that there are many factors affecting the adhesion between the coating and the substrate, including the properties of the substrate, the bonding method for the coating and the substrate, the physical and chemical properties of the coating material, and the method of coating preparation (Ottria et al., 2018). In this study, a strontium-doped titanium dioxide coating was prepared on a titanium surface with micro-arc oxidation. Scratch experiments and cross-sectional observations showed that the coating and the titanium matrix had bonded strongly, which met the requirements for orthopedic implant materials.
Research has confirmed that compared to smooth surfaces, rough surfaces exhibit higher levels of short-term osteoblast attachment, and the cell morphology is directly related to the properties of the material surface. Cells are more likely to adhere, stretch, and proliferate on rough, porous surfaces (Fandridis and Papadopoulos, 2008). Only through adhesion of more cells on the titanium surfaces can these cells promote bone tissue proliferation and aging, accelerate bone integration, and shorten the bone integration cycles of implants (Lewallen et al., 2021). Therefore, transforming the surfaces of orthopedic titanium implants from smooth to rough is beneficial for cell adhesion and growth and for dispersing stress at the implant–bone interface, which increases the initial stability. A prior study found that the alkaline phosphatase activities of cells on rough implant surfaces were higher, which enabled cell diffusion and proliferation on titanium surfaces and prevented implant loosening and sinking (Mistry et al., 2021). In this study, the roughnesses of three groups of samples were compared with a profilometer, and the results showed that compared with that of the Ti coating control group, the roughness of the strontium-doped titanium dioxide coating was higher and exhibited a statistically significant difference. This was mainly because during the micro-arc oxidation process, the film layer was broken down by spark discharge and formed a porous structure, resulting in increased roughness of the coating.
Whether studying cell proliferation or differentiation, initial attachment of the cells is a crucial first step and the foundation for subsequent biological activity of the cells. The adhesion behaviors of cells are influenced by the physical and chemical properties of the material surface, such as the surface roughness, and the surface elemental composition, porosity, and pore size (Anselme, 2000). The MT-Sr coating prepared by micro-arc oxidation in this study exhibited a porous surface structure, and more importantly, strontium was introduced into the coating during the micro-arc oxidation process. Research showed that strontium induced MC3T3-E1 cells to differentiate into osteoblasts, which enables proliferation of the osteoblasts, and inhibits the proliferation of osteoclasts and bone absorption, so strontium plays an important role in the process of bone formation (Zhou et al., 2022). The results of this study showed that on the first day after cell inoculation on the material surface, the amounts of cells adhered to the surfaces of each group of samples were different. The number of cells adhered to the surfaces of the MT-Sr coating group was significantly higher. With longer times, the number of cells adhered to the surfaces of the samples of each group gradually increased, but the number of cells adhered to the MT-Sr coating group was significantly higher than those of the other two groups. The difference was statistically significant and indicated that the porous, rough surface of the MT-Sr coating doped with strontium ions was most conducive to cell adhesion. Similar to cell adhesion, the extension of cells on the surfaces of each group of materials also showed similar results.
After adhesion and extension of the bone cells on the surfaces of titanium implants, they immediately entered the proliferation stage. The proliferation of cells on the material surface is the foundation for subsequent cell differentiation and bone integration. There are many factors affecting the proliferation of cells on the surfaces of materials, such as implant roughness, surface morphology, chemical composition, surface wettability, charge, and disinfection methods used for the implant surfaces. Zhang et al. (2016) showed that strontium was incorporated into the nanoporous structures of titanium implants via micro-arc oxidation and found that the coating induced faster bone formation. Similarly, Wang et al. (2023) found that strontium-doped coatings on titanium surfaces improved their osteogenic capabilities. In this study, the proliferation rate for the strontium-doped titanium dioxide coating was higher than that of the blank control group on days 1, 3, and 5, with statistically significant differences. This indicated that the strontium-doped titanium dioxide coating promoted the proliferation of MC3T3-E1 cells. The good biological activity of the strontium-doped titanium dioxide coating was related to the porous structure of the coating and to the slow release of strontium ions from the coating. Our research results are consistent with the results reported in the literature (Yuan et al., 2017; Geng et al., 2022).
Some osteogenic marker genes can be expressed during cell differentiation and proliferation. The genes BMP2, RunX2, ALP, and OCN are related to osteogenic differentiation by cells. ALP is a protein gene encoding extracellular maturation, and it is expressed in the early stages of osteoblast differentiation; expression is increased until the mineralization stage, and then the activity decreases. The OCN gene is one of the indicators of osteoblast activity and differentiation. BMP2 plays a significant role in the differentiation of osteoblasts and the synthesis and secretion of the bone matrix and is one of the most important extracellular signaling molecules promoting bone tissue formation and inducing bone differentiation. RunX2 is a specific osteoblast transcription factor expressed in the osteoblasts of developing bone, and it plays an irreplaceable role. In this study, expression of the aforementioned osteogenic-related genes was detected with qRT‒PCR. The results showed that compared with that in the Ti coating control group, the expression levels for BMP2, RunX2, ALP, and OCN in the strontium-doped titanium dioxide coating group were higher, and the differences were statistically significant. This indicated that the strontium-doped titanium dioxide coating promoted osteoblast differentiation.
Strontium is a trace element in the human body, and it elicits cytotoxic effects when present in excess (Attarilar et al., 2020). Therefore, the cytotoxicities of strontium-doped titanium dioxide coatings on the surfaces of implants also require special attention. In this study, the cytotoxicity of a strontium-doped titanium dioxide coating was evaluated through live cell staining. The results showed that there was no significant difference in the number of dead cells on the surface of the strontium-doped titanium dioxide coating compared to that on the surface of the Ti coating control group, indicating that the strontium-doped titanium dioxide coating exhibited good cell compatibility but no significant cytotoxicity. In this study, the strontium-doped titanium dioxide coating had low strontium contents and exhibited slow strontium ion release, so the coating did not exhibit significant cytotoxicity.
In summary, strontium was introduced into a titanium dioxide coating through micro-arc oxidation, and a strontium-doped titanium dioxide coating was successfully prepared on the surface of titanium. The coating was firmly bonded to the titanium substrate and had high surface porosity and good wear and corrosion resistance. In vitro cell experiments showed that the strontium-doped titanium dioxide coating promoted adhesion, proliferation and differentiation of MC3T3-E1 cells and exhibited good biocompatibility and biological activity. This study provides a new method for improving the biological activity of titanium, which has potential clinical applications.
The original contributions presented in the study are included in the article/supplementary material, further inquiries can be directed to the corresponding author.
HZ and SH were responsible for conceptualization of the study; QZ provided the guidance and supervision for the execution of experiments; LZ, JY, and BJ carried out the cellular studies; LZ, JY, and XN carried out preparation and characterization evaluation of materials, KL and PZ carried out the imaging and data analysis; HZ, SH, and LZ prepared the draft of the manuscript; QZ reviewed and edited the draft of the manuscript. All authors contributed to the article and approved the submitted version.
This work was supported by Guizhou Province Excellent Young Science and Technology Talents Project (YQK [2023] 022), Key Research and Development (Key R&D) plan of Jiangsu Province—social development (BE2021683), Guiyang Science and Technology Plan Project (grant number [2023] 48-23), Guizhou provincial people’s hospital fund (grant numbers GZSYBS [2022] 01 and GZSYQN [2022] 03).
The authors declare that the research was conducted in the absence of any commercial or financial relationships that could be construed as a potential conflict of interest.
All claims expressed in this article are solely those of the authors and do not necessarily represent those of their affiliated organizations, or those of the publisher, the editors and the reviewers. Any product that may be evaluated in this article, or claim that may be made by its manufacturer, is not guaranteed or endorsed by the publisher.
Albrektsson, T., and Wennerberg, A. (2019). On osseointegration in relation to implant surfaces. Clin. Implant. Dent. Relat. Res. 21 (1), 4–7. doi:10.1111/cid.12742
Anselme, K. (2000). Osteoblast adhesion on biomaterials. Biomaterials 21 (7), 667–681. doi:10.1016/s0142-9612(99)00242-2
Attarilar, S., Yang, J., Ebrahimi, M., Wang, Q., Liu, J., Tang, Y., et al. (2020). The toxicity phenomenon and the related occurrence in metal and metal oxide nanoparticles: A brief review from the biomedical perspective. Front. Bioeng. Biotechnol. 8, 822. doi:10.3389/fbioe.2020.00822
Ding, S. J. (2003). Properties and immersion behavior of magnetron-sputtered multi-layered hydroxyapatite/titanium composite coatings. Biomaterials 24 (23), 4233–4238. doi:10.1016/s0142-9612(03)00315-6
Fandridis, J., and Papadopoulos, T. (2008). Surface characterization of three titanium dental implants. Implant. Dent. 17 (1), 91–99. doi:10.1097/ID.0b013e318166d9ac
Geng, Z., Li, X., Ji, L., Li, Z., Zhu, S., Cui, Z., et al. (2021). A novel snail-inspired bionic design of titanium with strontium-substituted hydroxyapatite coating for promoting osseointegration. J. Mater. Sci. Technol. 79, 35–45. doi:10.1016/j.jmst.2020.11.041
Geng, Z., Sang, S., Wang, S., Meng, F., Li, Z., Zhu, S., et al. (2022). Optimizing the strontium content to achieve an ideal osseointegration through balancing apatite-forming ability and osteogenic activity. Biomater. Adv. 133, 112647. doi:10.1016/j.msec.2022.112647
Geng, Z., Wang, X., Zhao, J., Li, Z., Ma, L., Zhu, S., et al. (2018). The synergistic effect of strontium-substituted hydroxyapatite and microRNA-21 on improving bone remodeling and osseointegration. Biomater. Sci. 6 (10), 2694–2703. doi:10.1039/c8bm00716k
Guillemot, F., Prima, F., Bareille, R., Gordin, D., Gloriant, T., Porté-Durrieu, M. C., et al. (2004). Design of new titanium alloys for orthopaedic applications. Med. Biol. Eng. Comput. 42 (1), 137–141. doi:10.1007/bf02351023
He, W., Yin, X., Xie, L., Liu, Z., Li, J., Zou, S., et al. (2019). Enhancing osseointegration of titanium implants through large-grit sandblasting combined with micro-arc oxidation surface modification. J. Mater. Sci. Mater. Med. 30 (6), 73. doi:10.1007/s10856-019-6276-0
Kato, H., Nakamura, T., Nishiguchi, S., Matsusue, Y., Kobayashi, M., Miyazaki, T., et al. (2000). Bonding of alkali- and heat-treated tantalum implants to bone. J. Biomed. Mater. Res. 53 (1), 28–35. doi:10.1002/(sici)1097-4636(2000)53:1<28::aid-jbm4>3.0.co;2-f
Kaur, M., and Singh, K. (2019). Review on titanium and titanium based alloys as biomaterials for orthopaedic applications. Mater. Sci. Eng. C Mater. Biol. Appl. 102, 844–862. doi:10.1016/j.msec.2019.04.064
Kozelskaya, A. I., Rutkowski, S., Frueh, J., Gogolev, A. S., Chistyakov, S. G., Gnedenkov, S. V., et al. (2022). Surface modification of additively fabricated titanium-based implants by means of bioactive micro-arc oxidation coatings for bone replacement. J. Funct. Biomater. 13 (4), 285. doi:10.3390/jfb13040285
Lewallen, E. A., Trousdale, W. H., Thaler, R., Yao, J. J., Xu, W., Denbeigh, J. M., et al. (2021). Surface roughness of titanium orthopedic implants alters the biological phenotype of human mesenchymal stromal cells. Tissue Eng. - Part a. 27 (23-24), 1503–1516. doi:10.1089/ten.TEA.2020.0369
Li, Y., Wang, W., Liu, H., Lei, J., Zhang, J., Zhou, H., et al. (2018). Formation and in vitro/in vivo performance of "cortex-like" micro/nano-structured TiO2 coatings on titanium by micro-arc oxidation. Mater. Sci. Eng. C Mater. Biol. Appl. 87, 90–103. doi:10.1016/j.msec.2018.02.023
Lourenço, A. H., Torres, A. L., Vasconcelos, D. P., Ribeiro-Machado, C., Barbosa, J. N., Barbosa, M. A., et al. (2019). Osteogenic, anti-osteoclastogenic and immunomodulatory properties of a strontium-releasing hybrid scaffold for bone repair. Mater. Sci. Eng. C Mater. Biol. Appl. 99, 1289–1303. doi:10.1016/j.msec.2019.02.053
Lu, W., Zhou, C., Ma, Y., Li, J., Jiang, J., Chen, Y., et al. (2022). Improved osseointegration of strontium-modified titanium implants by regulating angiogenesis and macrophage polarization. Biomater. Sci. 10 (9), 2198–2214. doi:10.1039/d1bm01488a
Manivasagam, V. K., Sabino, R. M., Kantam, P., and Popat, K. C. (2021). Surface modification strategies to improve titanium hemocompatibility: A comprehensive review. Mater. Adv. 2 (18), 5824–5842. doi:10.1039/d1ma00367d
Marx, D., Rahimnejad Yazdi, A., Papini, M., and Towler, M. (2020). A review of the latest insights into the mechanism of action of strontium in bone. Bone Rep. 12, 100273. doi:10.1016/j.bonr.2020.100273
Milella, E., Cosentino, F., Licciulli, A., and Massaro, C. (2001). Preparation and characterisation of titania/hydroxyapatite composite coatings obtained by sol-gel process. Biomaterials 22 (11), 1425–1431. doi:10.1016/s0142-9612(00)00300-8
Mistry, S., Burman, S., Roy, S., Maitra, N. J., Roy, R., and Chanda, A. (2021). Surface characteristics of titanium dental implants with improved microdesigns: An in vivo study of their osseointegration performance in goat mandible. J. Biomater. Appl. 35 (7), 799–813. doi:10.1177/0885328220947345
Offermanns, V., Andersen, O. Z., Riede, G., Sillassen, M., Jeppesen, C. S., Almtoft, K. P., et al. (2018). Effect of strontium surface-functionalized implants on early and late osseointegration: A histological, spectrometric and tomographic evaluation. Acta Biomater. 69, 385–394. doi:10.1016/j.actbio.2018.01.049
Ong, J. L., and Chan, D. C. (2000). Hydroxyapatite and their use as coatings in dental implants: A review. Crit. Rev. Biomed. Eng. 28 (5-6), 667–707. doi:10.1615/critrevbiomedeng.v28.i56.10
Ong, J. L., and Chan, D. C. N. (2017). A review of hydroxapatite and its use as a coating in dental implants. Crit. Rev. Biomed. Eng. 45 (1-6), 411–451. doi:10.1615/CritRevBiomedEng.v45.i1-6.160
Ottria, L., Lauritano, D., Andreasi Bassi, M., Palmieri, A., Candotto, V., Tagliabue, A., et al. (2018). Mechanical, chemical and biological aspects of titanium and titanium alloys in implant dentistry. J. Biol. Regul. Homeost. Agents 32 (1), 81–90.
Pan, X., Li, Y., Abdullah, A. O., Wang, W., Qi, M., and Liu, Y. (2019). Micro/nano-hierarchical structured TiO2 coating on titanium by micro-arc oxidation enhances osteoblast adhesion and differentiation. R. Soc. Open Sci. 6 (4), 182031. doi:10.1098/rsos.182031
Ribeiro, A. R., Oliveira, F., Boldrini, L. C., Leite, P. E., Falagan-Lotsch, P., Linhares, A. B., et al. (2015). Micro-arc oxidation as a tool to develop multifunctional calcium-rich surfaces for dental implant applications. Mater. Sci. Eng. C Mater. Biol. Appl. 54, 196–206. doi:10.1016/j.msec.2015.05.012
Wang, Y. R., Yang, N. Y., Sun, H., Dong, W., Deng, J. P., Zheng, T. X., et al. (2023). The effect of strontium content on physicochemical and osteogenic property of Sr/Ag-containing TiO2 microporous coatings. J. Biomed. Mater. Res. B Appl. Biomater. 111 (4), 846–857. doi:10.1002/jbm.b.35195
Xue, T., Attarilar, S., Liu, S., Liu, J., Song, X., Li, L., et al. (2020). Surface modification techniques of titanium and its alloys to functionally optimize their biomedical properties: Thematic review. Front. Bioeng. Biotechnol. 8, 603072. doi:10.3389/fbioe.2020.603072
Yuan, N., Jia, L., Geng, Z., Wang, R., Li, Z., Yang, X., et al. (2017). The incorporation of strontium in a sodium alginate coating on titanium surfaces for improved biological properties. Biomed. Res. Int. 2017, 1–11. doi:10.1155/2017/9867819
Zhang, W., Cao, H., Zhang, X., Li, G., Chang, Q., Zhao, J., et al. (2016). A strontium-incorporated nanoporous titanium implant surface for rapid osseointegration. Nanoscale 8 (9), 5291–5301. doi:10.1039/c5nr08580b
Keywords: titanium, plasma electrolytic oxidation, surface characterization, coating, biological activity
Citation: Zheng H, He S, Zhou L, Yuan J, Jiang B, Ni X, Lu K, Zhang P and Zhao Q (2023) A cytocompatible microporous Sr-doped titanium dioxide coating fabricated by plasma electrolytic oxidation. Front. Mater. 10:1210728. doi: 10.3389/fmats.2023.1210728
Received: 23 April 2023; Accepted: 26 May 2023;
Published: 05 June 2023.
Edited by:
Arun Prabhu Rameshbabu, Harvard Medical School, United StatesReviewed by:
Shokouh Attarilar, Shanghai Jiao Tong University, ChinaCopyright © 2023 Zheng, He, Zhou, Yuan, Jiang, Ni, Lu, Zhang and Zhao. This is an open-access article distributed under the terms of the Creative Commons Attribution License (CC BY). The use, distribution or reproduction in other forums is permitted, provided the original author(s) and the copyright owner(s) are credited and that the original publication in this journal is cited, in accordance with accepted academic practice. No use, distribution or reproduction is permitted which does not comply with these terms.
*Correspondence: Quanming Zhao, ZG9jdG9yMTEwNkAxNjMuY29t
†These authors have contributed equally to this work and share first authorship
Disclaimer: All claims expressed in this article are solely those of the authors and do not necessarily represent those of their affiliated organizations, or those of the publisher, the editors and the reviewers. Any product that may be evaluated in this article or claim that may be made by its manufacturer is not guaranteed or endorsed by the publisher.
Research integrity at Frontiers
Learn more about the work of our research integrity team to safeguard the quality of each article we publish.