Corrigendum: Fabrication, properties and biological activity of a titanium surface modified with zinc via plasma electrolytic oxidation
- 1Department of Orthopedics, Guizhou Provincial People’s Hospital, Guiyang, Guizhou, China
- 2Department of Stomatology, the Seventh Medical Center of PLA General Hospital, Beijing, China
- 3Department of Orthopaedics, Affiliated Hospital of Jiangnan University, Wuxi, Jiangsu, China
- 4Department of Orthopedics, Dafeng People’s Hospital, Yancheng, Jiangsu, China
To improve the biological activities of titanium implant surfaces and promote efficient early bone formation, the formation of bioactive coatings that promote bone formation on titanium implant surfaces is currently an important area of research. In this study, we prepared a zinc-doped porous coating (Zn-PEO) on a titanium alloy surface via plasma electrolytic oxidation. The surface characteristics of the samples were evaluated with field emission scanning electron microscopy (FE-SEM), X-ray photoelectron spectroscopy (XPS), profilometry, static contact angles and nanoscratch studies. The slow release of zinc from the coating was detected with inductively coupled plasma‒mass spectrometry (ICP‒MS). The corrosion resistances of the samples were evaluated via electrochemical studies. On this basis, we evaluated the biocompatibility and biological activity of the sample through in vitro cell experiments. The results confirmed that a Zn-PEO coating was successfully prepared on the surface of the titanium alloy; it exhibited a porous micro/nanomorphology, and the coating and the substrate were tightly bound. The coating increased the roughness of the titanium surface and improved the wear and corrosion resistance. More importantly, the coating promoted adhesion, proliferation, differentiation and mineralization of bone marrow mesenchymal stem cells and had good biological activity. Therefore, Zn-PEO coatings with porous structures were prepared on the surfaces of titanium implants through plasma electrolytic oxidation. The Zn-PEO coating exhibited good surface properties and biological activity and has good application prospects.
1 Introduction
Titanium and its alloys are widely used as hard tissue repair and replacement materials in orthopedics and stomatology due to their excellent mechanical properties, corrosion resistance and biocompatibility. However, there are problems with titanium and its alloys, such as biological inertness of the surface, poor biological activity, lack of osteogenic inducibility, high elastic moduli and limited corrosion resistance, which affect bonding with the surrounding bone tissue and significantly limit their use in orthopedic and dental implants (Donley and Gillette, 1991; Ottria et al., 2018). Due to the interactions between the titanium implants and the surrounding bone tissue that initially occur at the surfaces of the titanium alloys, it is necessary to modify these surfaces to solve the clinical problems.
Many surface modification technologies have been used to improve the surface properties of titanium and its alloys, including hydrothermal treatment, hydrogen peroxide treatment, alkali heat treatment, acid treatment, chemical vapor deposition, plasma spraying, ion implantation, anodic oxidation and plasma electrolytic oxidation (PEO) (Ripamonti et al., 2012; Gasik et al., 2015; Chouirfa et al., 2019; Li et al., 2019; Stein et al., 2020; Van den Borre et al., 2022). PEO is a relatively simple and efficient technique that generates oxide ceramic coatings that grow in situ and exhibit strong bonding and micro/nanoporous structures on the surfaces of titanium, magnesium, aluminum, tantalum and other metals (Sikdar et al., 2021). Moreover, it is widely used in surface modifications of metal materials. In a classic electrolyte mixture containing Ca and P, PEO forms micro/nano porous coatings containing Ca and P on the surface of titanium. Research has shown that the micro/nanoporous structures formed by plasma electrolytic oxidation of titanium enhances cell adhesion and proliferation and promotes the formation of bone tissue on the surfaces of titanium implants (Marques Ida et al., 2015). In addition, previous studies have shown that the porous structures on the titanium surfaces significantly improve integration between the titanium implants and the bone tissue (Wang et al., 2022). Therefore, the porous micro/nanostructures prepared with PEO activate the titanium surface and enhance its biological activity.
The micro/nanostructure increases the surface area of the titanium implant and increases the space between the implant and the host bone. More importantly, the micro/nanostructure provides a suitable microenvironment for the growth of bone marrow mesenchymal stem cells (BMSCs) and other cells (Li K. et al., 2020). PEO can provide a range of coating pore sizes if the appropriate parameters (voltage, current, duty ratio and time) are chosen. Prior results showed that the pore sizes of the coating gradually increased with increasing PEO treatment time, whereas the number of pores gradually decreased. More importantly, PEO can also introduce bioactive elements such as Cu, Si, Co, Mn, and Sr into the coating (Zhou and Zhao, 2016; Sedelnikova et al., 2019; Li Y. et al., 2020; Shimabukuro, 2020), control the contents of the elements in the coating by controlling the concentrations of the electrolyte and other parameters and improve the bioactivity of titanium implant through slow release of these bioactive ions. Due to these advantages, PEO has received increasing attention in clinical practice.
As an essential trace element for the human body, zinc is essential for bone growth and development in humans and various animals. Zinc is a very effective contributor to bone metabolism. Specifically, zinc affects the functions of osteoclasts, whereby it reduces bone resorption by inhibiting the osteoclasts. By inhibiting the expression of RANK, the activities of TRAP and cathepsin K are inhibited, and bone resorption is reduced (Sutthavas et al., 2022). In addition, zinc affects the function of osteoblasts. By regulating the expression of Runx2, increasing the syntheses and activities of matrix proteins, type I collagen and ALP, and increasing the accumulation of calcium and phosphorus, zinc promotes calcification of the extracellular matrixes of the osteoblasts and promotes bone formation. In addition, zinc may also affect the bone formation process by influencing the expression of osteoprotegerin and regulating insulin growth factor (Toledano et al., 2021). Furthermore, zinc affects the formation of bone in the human body through various channels, and osteoblasts are important functional cells in the process of osteogenesis.
The interface between the titanium surface and the bone is the focal point for material and cellular biological response events, but the biological inertness of the titanium surface inhibits effective integration of the material with the surrounding bone tissue. The need to “biologically activate” the inert titanium surface interface constitutes an important challenge in this field. The interactions occurring at the interface between the titanium surface and the bone tissue depend on the surface characteristics of titanium, including the surface morphology, chemical composition, roughness, hydrophilicity/hydrophobicity and surface charge. Due to the porous micro- and nanomorphologies of the coatings prepared via plasma electrolytic oxidation, it is important to introduce zinc with its various biological functions into the coating through plasma electrolytic oxidation. When considering this scenario, this study was designed to use plasma electrolytic oxidation to prepare a Zn-PEO coating exhibiting porous structures on the surface of a titanium alloy; stainless steel was chosen as the cathode and titanium alloy as the anode in a zinc-containing electrolyte solution. Zinc was introduced into the titanium surface coating through plasma electrolytic oxidation, which combined the desired titanium surface structure and biological activity. With this combination of porous structures and zinc, the titanium surface was biologically activated. This study will provide new ideas for improving and optimizing the biological activities of titanium surfaces, and the method has clinical application prospects.
2 Materials and methods
2.1 Preparation of coating
The titanium alloy was processed into titanium sheets with diameters of 12 mm and thicknesses of 1 mm with wire electrical discharge machining (EDM) technology. Abrasive paper (200 #, 400 #, 600 #, 1,000 #, 1,500 # and 3,000 #) was used to polish the titanium sheet to remove the oil stains on the surface until it was smooth and free of obvious scratches. Finally, acetone, anhydrous ethanol and deionized water were used for ultrasonic cleaning, and the sheet was dried in an oven for later use.
The power supply for plasma electrolytic oxidation was a MAO-600-11A. The voltage was 400 V, the time was 6 min, and the frequency was 1,000 Hz. The electrolyte temperature was consistently maintained at approximately 25°C. After the plasma electrolytic oxidation was completed, the sample was washed with deionized water and dried for later use.
To prepare the PEO coating, we dissolved 0.2 mol/L calcium acetate and 0.02 mol/L calcium glycerophosphate in 1 L of deionized water, stirred to dissolve the reagents and allowed the solution to stand at room temperature for 1 h. A stainless steel plate was used as the cathode, and a titanium alloy plate was used as the anode for plasma electrolytic oxidation.
In the preparation of the Zn-PEO coating, zinc gluconate (0.02 mol/L) was added to the abovementioned electrolyte mixture, and plasma electrolytic oxidation was performed with the same parameters.
2.2 Evaluation of the surface coating properties
Field emission scanning electron microscopy (FE-SEM) and profilometry were used to observe the surface morphologies of the samples; in addition, energy dispersive spectroscopy (EDS) and X-ray photoelectron spectroscopy (XPS) were used to observe the elemental compositions and chemical states of the coatings, and The surface roughness profilometer is used to test the roughness of the coating. The adhesion strengths and friction properties of the coatings were tested with nanomechanics testing system.
2.3 Zinc ion release experiment
The entire Zn-PEO sample was immersed in 10 mL α-MEM solution and sealed for storage, and samples were removed after heating at 37°C in an incubator for 1, 3, 5, 7, and 10 days successively. The samples were tested three times, and the regularity of zinc ion release from the Zn-PEO coating into the solution was analyzed with inductively coupled plasma-mass spectrometry (ICP‒MS).
2.4 Corrosion resistance tests
An electrochemical workstation was used to detect the corrosion resistances of the samples and generate Nyquist curves, Bode-impedance curves and Bode-phase curves. A three electrode system was used, with a saturated calomel electrode (SCE) as the reference electrode (RE), a platinum electrode as the auxiliary electrode (CE) and the workpiece as the working electrode. The corrosion solution contained 0.9 wt% NaCl. The sample was immersed in 30 mL of the corrosion solution and stabilized for 60 min. The frequency test range was 90 kHz–10 mHz, and the sinusoidal AC signal amplitude was 10 mV. After completion, the corresponding impedance parameters were calculated with the appropriate software.
2.5 Wear resistance evaluation
In this study, we used the nanomechanics testing system to evaluate the friction performance of the samples. The conditions for friction and wear are: a loading load of 50 mN, a wear scar length of 3 mm, a wear time of 10 min and a reciprocating frequency of 5 Hz. To better simulate the wear behavior in the human body environment, this test was conducted in a simulated body fluid environment. After the friction and wear test is completed, the samples are sequentially cleaned with alcohol and deionized water, and the surface morphology of the grinding crack is observed using a three-dimensional profilometer. The depth and width of the grinding crack are measured, and the wear rate of each group of samples is calculated (Wangcai et al., 2018).
2.6 In vitro cell research
2.6.1 Cell culture
Studies involving animals were reviewed and approved by the Ethics Committees of Guizhou Provincial People’s Hospital. The tibias and femurs of SD rats were the sources of the BMSCs. The rats were euthanized via cervical dislocation, and both femurs and tibiae were removed. The bone marrow was washed out with DMEM culture medium containing 1% double antibody and 10% fetal bovine serum. The cells were cultured in a 37°C cell incubator containing 5% CO2, and the fluid was changed every 3 days. Two to three generations of cells were used for the subsequent experiments.
2.6.2 Live/dead staining
Cells were inoculated on the surface of each sample at a density of 2 × 104 cells/well and cultured in a 37°C and 5% CO2 constant temperature incubator for 48 h. After rinsing with PBS, the cells were transferred to a new 24-well plate and treated with live/dead viability/cytotoxicity kits, after which fluorescence microscopy was used to determine the cell toxicities.
2.6.3 Adhesion of BMSCs
The cell inoculation density and culture method were the same as above. After incubation at 37°C for 120 min. Rinse with PBS to remove non adherent cells. The cells adhered to the samples were fixed with 75% alcohol and the nucleus was labeled with DAPI. Take photos under a fluorescence microscope and count the number of cells using Image J software.
2.6.4 Proliferation of BMSCs
The cell inoculation density and culture method were the same as those described above. After 72 h of culture, 200 μL EdU medium was added and the samples were incubated. Then, 100 µL of cell fixative was added to each well for 30 min, and 200 μL of 1X Apollo @ dye reaction solution was added for 30 min. Finally, Hoechst labeled the nucleus, washed the samples, and photographed under a fluorescence microscope.
2.6.5 ALP staining
The cell inoculation density and culture method were the same as those described above. The culture was terminated on the 14th day after inoculation, washed with PBS and fixed. ALP was stained with the BCIP/NBT kit and photographed under a stereomicroscope.
2.6.6 ECM mineralization
The culture was terminated on the 21st day after inoculation, rinsed with distilled water, fixed with paraformaldehyde solution, added to a alizarin red staining solution and stained at 37°C for 30 min. After thorough cleaning with distilled water, stereomicroscope photographs were taken.
2.7 Statistical analyses
All of the data were expressed as the mean ± standard deviation and were analyzed with SPSS 18.0 software. One-way ANOVA and the SNK test were used to compare the differences between the groups. Moreover, p < 0.05 indicated a statistically significant difference.
3 Results
Figure 1 shows the morphologies of different samples determined with FE-SEM. The surface of the Ti was smooth, with traces of sandpaper grinding and no micro/nanostructures. The surface of the PEO coating was covered with micropores of varying sizes and irregular shapes; several pores penetrated each other and several pore gaps remained in the cracks. The morphology of the Zn-PEO coating determined with SEM was similar to that of PEO, its surface was covered with micro holes of different sizes, and there were a few cracks remaining between the surfaces. This was caused by discharge ablation during the plasma electrolytic oxidation.
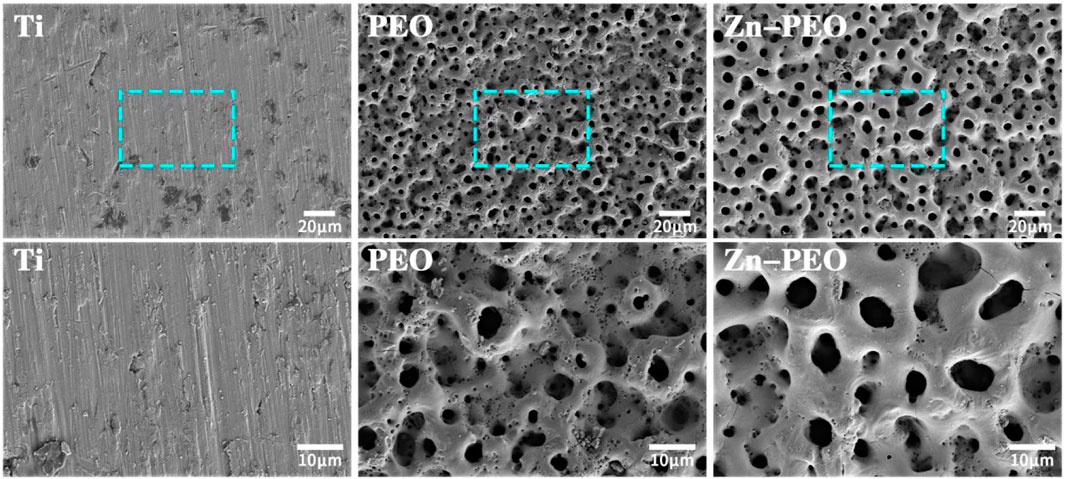
Figure 1. SEM surface morphologies of different samples under low and high magnification (500× and 1,500×).
Figure 2 shows the XPS data for the Zn-PEO coating, and Figure 2A shows the full X-ray photoelectron spectrum of the Zn-PEO microporous coating. In addition to the characteristic peaks for titanium, oxygen, calcium and phosphorus, there were characteristic peaks of Zn. The peak in the Ti 2p spectrum corresponded to titanium dioxide, and the P-O bonds of PO43− were evident in the P 2p data, thus indicating that P was present as PO43−. The Ca 2p binding energies were 347.5 eV and 351.7 eV, and the Ca 2p and P 2p data indicated the presence Ca3(PO4)2. The Zn 2p binding energy at 1,022.2 eV corresponded to ZnO.
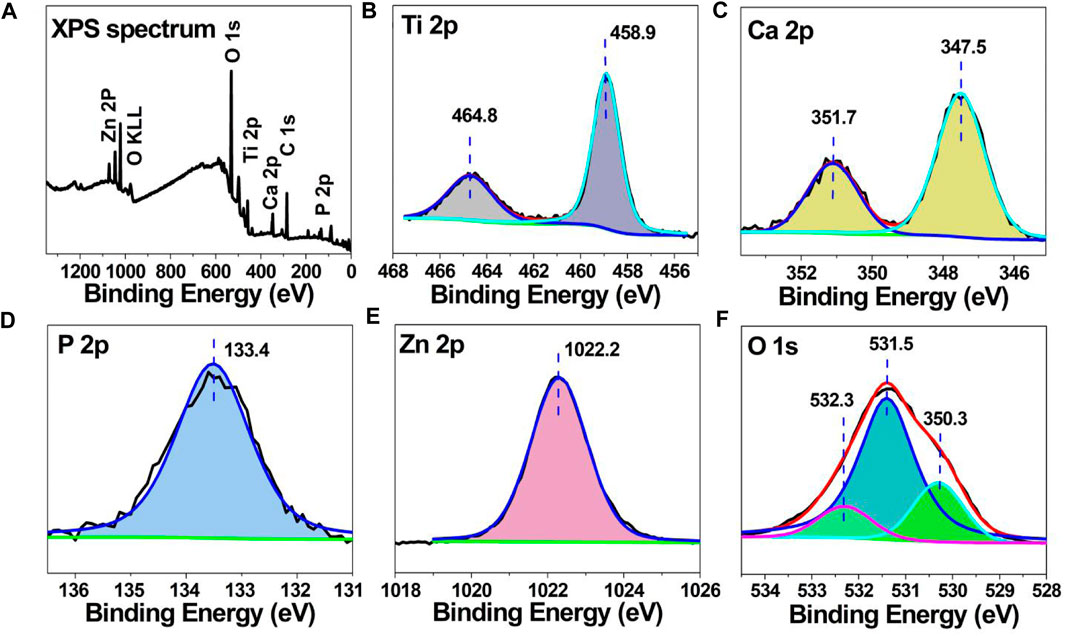
Figure 2. (A) XPS full spectrum for the Zn-PEO coating and (B) Ti 2p, (C) Ca 2p, (D) P 2p, (E) Zn 2p, and (F) O 1s XPS data.
Figure 3 contains electron microscopy images showing the cross-sectional morphology of the Zn-PEO coating. The coating consisted of a dense inner layer and a loose outer layer. The coating was grown from the titanium substrate and inlayed with the substrate. Moreover, the coating and the titanium substrate were strongly bound.
Figure 4 shows the profilometry surface topography of the sample. The Ti surface was relatively flat, and the PEO coating and Zn-PEO surface exhibited crater-like structures, thus resulting in a relatively high degree of roughness. Quantitative analyses of the surface roughness, Ra, for each sample showed that Zn-PEO and PEO coatings were rougher than the Ti surface, and the differences were statistically significant (p < 0.01). However, there were no significant differences in the roughnesses of the Zn-PEO and PEO coatings. Obviously, PEO increased the roughness of the titanium surface, which enabled cell adhesion and extension.
The bonding strength between the coating and the substrate is one of the important standards for evaluating the quality of the coating. The scratch method is one of the most commonly used methods for testing the adhesion between coatings and substrates. Its experimental principle is to keep the indenter running at a constant speed until the coating is scratched under the condition of increasing load. The critical load value for coating failure is determined by detecting the acoustic emission signal curve and the changes in tangential friction force throughout the entire process. During this process, a conical indenter is used to slide on the coating, and with the continuous increase of load, when the critical load is reached, the coating and substrate begin to peel off. At this point, a damage signal will appear on the acoustic emission curve, and a turning point will appear on the corresponding position of the friction curve to determine the bonding force between the coating and substrate. In this study, the adhesion between Zn-PEO coating and titanium substrate was tested by the nanomechanics testing system equipped with a diamond indenter. Figure 5 shows the acoustic emission spectrum of the Zn-PEO coating. Based on the acoustic emission spectrum of the coating, the adhesive force between the Zn-PEO coating and the substrate was greater than 180 N, indicating that the Zn-PEO coating was strongly bonded to the titanium substrate.
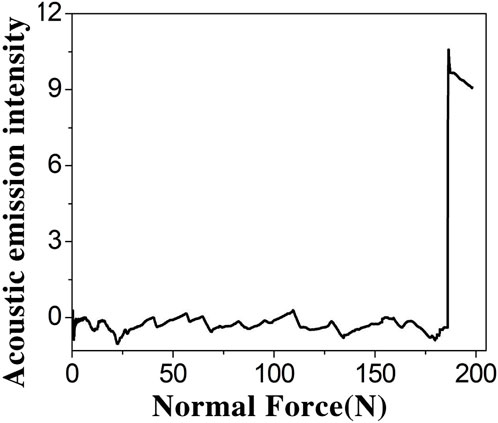
Figure 5. Acoustic emission spectrum of Zn-PEO (The initial load is 0 N, the final load is 150 N, the scratch speed is 2 mm/min, and the load loading rate is 50 N/min).
Figure 6 shows the surface morphology of the three-dimensional profilometer for each group of samples. It is obvious that the surface of Ti is severely worn, with deep and uniform grinding crack. Compared with Ti, the depth of wear marks on PEO and Zn-PEO is significantly reduced, and the wear marks are rougher. By calculating the wear rate, the wear rate of each group of samples follows the following trend: Ti > PEO > Zn-PEO.
Figure 7 shows the slow release curve for the zinc ions. With increasing time, the zinc ions underwent slow release into the solution. During the first 7 days, the zinc ions exhibited rapid release, the rate reached a peak on the seventh day and then stabilized, and then slow release followed. It was concluded that the zinc ions in the Zn-PEO coating were released slowly.
Figure 8 shows the electrochemical impedance spectra of each group of samples. Figure 8A shows the Nyquist curves of the samples, which clearly showed that the impedance curve shapes of the three samples were basically the same and contained capacitive arcs. The diameters of the capacitive arcs for Ti, PEO, and the Zn-PEO coating gradually increased, and the Zn-PEO coating had a significantly larger arc diameter than the other two samples, which indicated good corrosion resistance. Figure 8B shows the Bode-impedance curves of the samples. The higher the IZI value in the low-frequency region is, the better the corrosion resistance of the sample, and this again confirmed the excellent corrosion resistance of the Zn-PEO coating. Figure 8C shows the Bode-phase curves of the samples; there were no significant differences among the samples, indicating that there were no significant changes in the coating structure of the sample doped with Zn. In summary, the PEO coating formed by plasma oxidation improved the corrosion resistance of titanium, and the addition of zinc to the coating further enhanced the corrosion resistance of the coating.
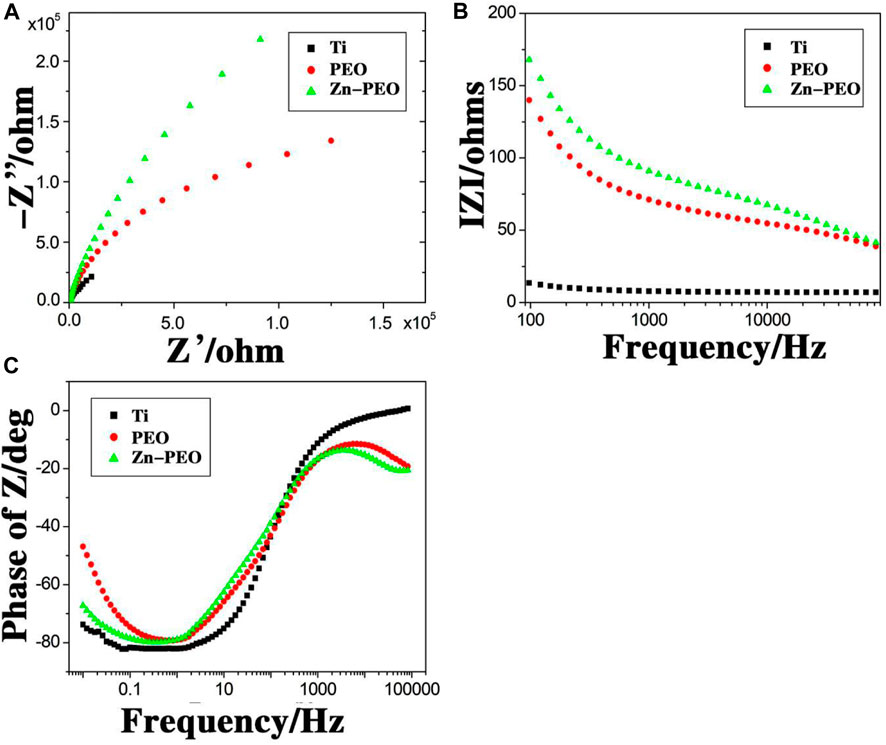
Figure 8. Electrochemical impedance spectroscopy. Nyquist curves (A), Bode-impedance curves (B) and Bode-phase curves (C) for Ti, PEO and Zn-PEO.
Figure 9 shows the results for fluorescence staining of living/dead BMSCs on different sample surfaces, with green indicating living cells and red indicating dead cells. The surfaces of all samples exhibited mainly green living cells with small amounts of red dead cells. Quantitative analyses showed that there were no significant differences in the number of dead cells among the groups, thus indicating that the Zn-PEO coating had no significant cytotoxicity and had good biocompatibility.
Figure 10 shows the number of BMSCs adhered to the different sample surfaces. At the 120 min time point, the number of cells adhering to the Zn-PEO surface was significantly higher than those of the Ti and PEO groups. Thus, the introduction of Zn into the coating promoted cell adhesion.
EdU staining is a new detection method for evaluating cell proliferation. Figure 11 shows the EdU staining results. The red fluorescence-labeled cells are EdU-positive, and the more EdU-positive cells in red, the more vigorous the cell proliferation. Obviously, compared with the Ti and PEO groups, there were significantly more EdU-positive cells on the Zn-PEO surface due to the microstructure of the coating and the slow release of zinc ions.
Figure 12A shows the ALP staining results for BMSCs cultured on the sample surface for 14 days. ALP is an indicator of early osteogenic differentiation of cells. ALP nodules were expressed on the surfaces of samples from all groups. Compared with those of the Ti and PEO groups, the color of the Zn-PEO stain was deeper and has more ALP nodules. Quantitative analysis of ALP staining showed that the difference was statistically significant compared to the Ti group (Figure 12C). It was obvious that Zn-PEO promoted osteogenic differentiation of the BMSCs. Figure 12B shows the alizarin red staining results for BMSCs cultured on the sample surface for 21 days. Alizarin red staining reflects mineralization of the extracellular matrix. We observed mineralized nodules on the surfaces of each group of samples. At 21 days, the Zn-PEO group had more mineralized nodules than the Ti and PEO groups, and quantitative analysis of alizarin red staining also showed that the difference was statistically significant compared to the Ti group (Figure 12D), thus indicating that the Zn-PEO surface promoted extracellular matrix mineralization, which was closely related to the porous structure of the surface and the slow release of zinc ions.
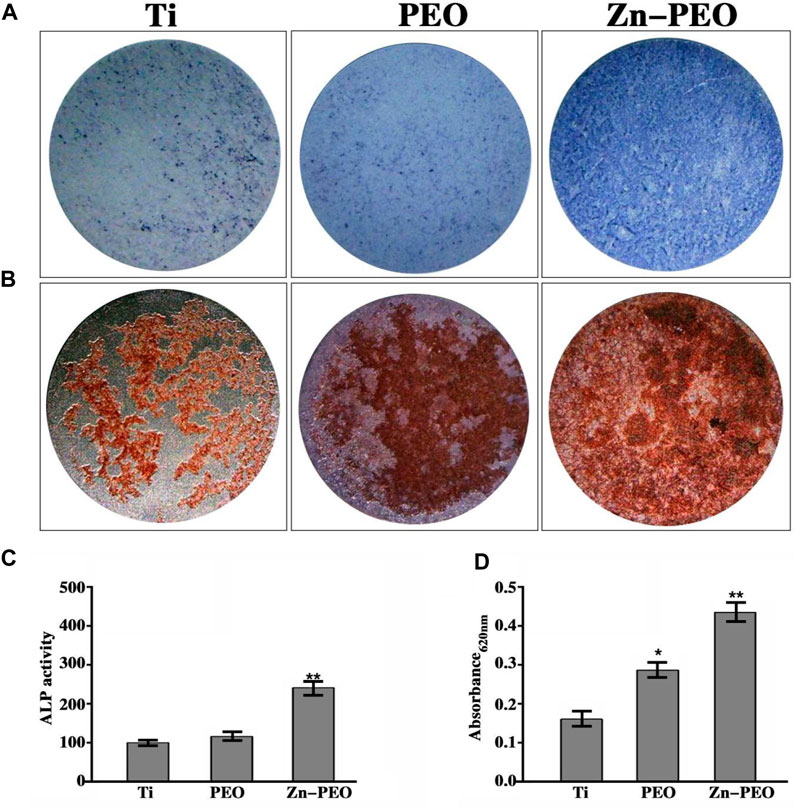
Figure 12. ALP staining of BMSCs after 7 days of culture and ECM mineralization of BMSCs after 21 days of culture [(A): ALP staining; (B): ECM mineralization; (C): Quantitative analysis of ALP staining and (D): Quantitative analysis of alizarin red staining].
4 Discussion
Due to the poor surface bioactivity and bone integration performance of titanium and its alloys, implant loosening is a difficult problem experienced by orthopedists and stomatologists. Improvement of the titanium surface bioactivity is effective in preventing the loosening of titanium implants. Prior results showed that the morphology and chemical composition of a titanium surface are closely related to its surface biological activity (Rupp et al., 2018). In terms of surface morphology, micro/nanomorphologies better simulate the microenvironments enabling natural cell growth and promote good bone integration between titanium implants and bone tissues. Moreover, the chemical composition of the titanium surface can directly improve the biological activity of titanium by releasing bioactive substances. In this study, zinc acetate was used as the main component of the electrolyte, and a Zn-PEO coating was prepared on the surface of a titanium alloy through plasma electrolytic oxidation. The micro/nanomorphology exhibited a good bionic effect when combined with zinc with good biological activity to improve the biological activity of the titanium. Therefore, this study provides new ideas for improving the biological activity of titanium.
The surface morphology has an important effect on the biocompatibility and bioactivity of an implant (Ogle, 2015). The Zn-PEO coating prepared in this study contained micro/nanopores, which were formed because the partial discharge in the plasma electrolytic oxidation process caused current breakdown of the titanium surface. The sizes of the micro/nanopores can be adjusted by adjusting the plasma-related parameters, such as the voltage and time. Generally, a higher voltage corresponds to larger pore diameters. Moreover, longer PEO times generate rougher surfaces. It has been confirmed that the micro- and nanostructures produced by plasma electrolytic oxidation can enhance cell adhesion and proliferation (Feller et al., 2015). The results of this were consistent with those reports. Compared with those of the Ti group, the cell adhesion and proliferation were significantly increased for the PEO and Zn-PEO groups, which we believe was related to the micro/nanostructures generated by plasma electrolytic oxidation.
The surface roughnesses of materials are also important factors affecting their biological activities (Barfeie et al., 2015). The definition of surface roughness is not completely consistent for titanium implants. Generally, a roughness Ra < 1 µm indicates a smooth surface, and a roughness Ra > 1 µm indicates a rough surface. In this study, the surface roughness of the Zn-PEO coating formed by plasma electrolytic oxidation of titanium exhibited Ra > 1 μm, which indicated a rough surface that enables cell adhesion and proliferation. Moreover, a rougher implant surface corresponds to a larger specific surface area and greater hydrophilicity, which facilitate adhesion and proliferation of osteoblasts on the implant surface (Feller et al., 2014).
In studies of the surface modifications of titanium implants, it is relative easy to dope biologically active inorganic ions exhibiting controllable release into the coating. The advantages of this are that the method is inexpensive and the coating is durable. Zn is an indispensable trace element in the human body. Although its content is very small, it plays a very important role in the growth of bone tissue and is a necessary trace element for osteoblastic activity, ALP activity and collagen synthesis. In recent years, with the successful addition of zinc to bioceramics, hydroxyapatite, tricalcium β-phosphate and magnesium alloys, some researchers have attempted to use zinc to modify the surfaces of titanium alloys (Xue et al., 2008; Garbo et al., 2020; Batool et al., 2022). Yu et al. (2023) introduced zinc doping into the surface of titanium through micro arc oxidation and found that the zinc ions exhibited stable release, as well as good angiogenesis and osteogenesis potential. Cerqueira et al. (2021) prepared a zinc-doped bioactive coating on a titanium surface with the sol-gel method. Further research showed that the coating exhibited no cytotoxicity and caused ALP and TGF in osteoblasts. Moreover, the expression of β and RUNX2 genes increased. Wang et al. (2019) fixed zinc ions on the surfaces of titanium implants through polydopamine (PDA) chemical surface modifications to prepare Ti-PDA-Zn coatings. Further research showed that the Ti-PDA-Zn coating exhibited excellent antibacterial activity against both Staphylococcus aureus and Escherichia coli in vitro. In vivo experiments confirmed that the Ti-PDA-Zn coating showed excellent antibacterial performance and improved bone integration ability in the presence of Staphylococcus aureus. In this study, we used plasma electrolytic oxidation to introduce zinc into porous micro/nanocoatings. In vitro studies confirmed that this coating promotes the adhesion and proliferation of bone marrow mesenchymal stem cells. Our results are consistent with the abovementioned results, thus indicating that the introduction of zinc into the surfaces of titanium implants improves the biocompatibility and biological activity of titanium and has potential clinical application value.
After contact with materials, cells typically undergo three processes: attachment, proliferation and differentiation. Proliferation and differentiation are two major functions of cell life. ALP expression begins in the late stage of cell proliferation and is often widely used as a marker of cell osteogenesis and early osteogenesis. Increased ALP expression corresponds to a higher probability that the cells will differentiate into osteoblasts. In this study, three different material surfaces were cultured with cells for 7 and 14 days, and the results showed that the level of ALP expression on the Zn-PEO surface was the highest, with a statistically significant difference compared to the control group. This indicated that the Zn-PEO surface promoted cell differentiation into osteoblasts and promoted bone formation. Alizarin red staining was used to detect the degree of mineralization for the cells cultured on the different samples. The results showed that the Zn-PEO surface had the highest degree of mineralization, thus indicating that the Zn-PEO surface promoted cell mineralization. This result was consistent with the results from ALP detection. Therefore, it can be speculated that Zn-PEO on the titanium surface promoted osteoblastic differentiation and extracellular matrix mineralization of cells. Our research and the results of literature reports are consistent. Jin et al. (2014) implanted zinc and silver onto the surfaces of titanium implants through ion implantation to form a zinc-containing film with Ag nanoparticles (Ag NP). In vitro studies confirmed that this biologically active film promoted osteogenic differentiation and mineralization of the bone marrow mesenchymal stem cells. Chen et al. (2020) prepared zinc-doped TiO2 nanotubes (TNTs) on a pure titanium surface through anodic oxidation. Further research showed that zinc-doped TiO2 nanotubes promoted the proliferation of M2-type macrophages and showed stronger cell proliferation, adhesion, osteogenic-related gene expression, alkaline phosphatase activity and extracellular mineralization in osteoblasts cultured on zinc-doped TNTs. In a similar study, He et al. (2018) prepared TiO2 nanorod membranes doped with zinc-containing bioactive glass (TiO2/Zn-BG) on tantalum substrates. Cell experiments showed that, compared to membranes without Zn (TiO2/BG), the expression of osteogenic-related genes and ALP and ECM mineralization on TiO2/Zn-BG membranes were significantly upregulated. Further research showed that the enhanced osteoblast differentiation and mineralization seen with this bioactive film were closely related to the Zn2+ microenvironments around the TiO2 nanorods. The Zn-PEO coatings on the surfaces of the titanium implants used in this study promoted bone formation, which was related to the micro- and nanomorphology of the Zn-PEO coating and may be related to the release of Zn2+ from the Zn-PEO coating. However, the specific mechanism for bone formation with Zn2+ still requires further research.
In this study, although the surface roughness and porosity of the sample was increased by the plasma electrolytic oxidation treatment, the corrosion resistance of the Zn-PEO and PEO surfaces were significantly better than that of titanium, which was closely related to the crystalline titanium dioxide in the coating after the plasma electrolytic oxidation. Titanium dioxide is a ceramic, and the biggest advantage of ceramics is their excellent corrosion resistance. Because plasma electrolytic oxidation is carried out at high temperatures, the coating is formed directly on the surface of titanium, ensuring the tightness and integrity of the coating and effective bonding to the titanium substrate. Furthermore, although SEM cross-sectional images of the coating revealed micrometer-sized pores on the surface leading to the titanium substrate, the pores were not in direct contact with the metal substrate but only reached the middle of the coating. The closer the pores were to the coating interface, the smaller the pore sizes. Therefore, the pores did not damage the integrity of the ceramic coating. In addition, the increased thickness of the titanium surface coating after plasma electrolytic oxidation also reduced the contact between the titanium substrate and the liquid and improved its corrosion resistance. Therefore, the coating formed on the titanium surface via plasma electrolytic oxidation improved the titanium surface morphology and enhanced its corrosion resistance. In addition, the corrosion resistance of the Zn-PEO coating prepared in this study was higher than that of the PEO coating. We believe that because the Zn-PEO coating contains zinc, the release of zinc ions from the coating improved the corrosion resistance. However, further research is needed to elucidate the specific mechanism.
Cell compatibility is a basic requirement for implants. In this study, the biocompatibility of the Zn-PEO coating was evaluated with live/dead cell staining. There were no significant differences in the number of dead cells on the surfaces of each sample group, thus indicating that Zn-PEO had good biocompatibility but no significant cytotoxicity. Although zinc is a necessary trace element, excess local concentrations may lead to toxic reactions in nearby cells or tissues. Due to the specific mechanism of plasma electrolytic oxidation, an excessive concentration of zinc in the electrolyte solution during plasma electrolytic oxidation will prevent the plasma electrolytic oxidation from striking an arc, thus resulting in failure of the plasma electrolytic oxidation. Therefore, in this study, the Zn-PEO coatings prepared on the titanium surfaces through plasma electrolytic oxidation had a very low zinc concentrations, and the zinc was slowly released; therefore, there was no significant cytotoxicity. Our results are similar to those of previous reports. The Zn-modified TiO2 coatings prepared by Lv et al. (2021) on the surfaces of titanium implants via ultrasonic micro arc oxidation showed no significant cytotoxicity and good biocompatibility. Li et al. (2021) prepared a layer of hydroxyapatite with different concentrations of zinc ions on a titanium surface by using the sol gel process combined with sol gel and micro arc oxidation and coated it on the micro arc oxidized titanium substrate. In vitro experiments confirmed that the zinc ion-doped composite coating had good biocompatibility.
In summary, we added zinc gluconate to an electrolyte solution composed of calcium acetate and calcium glycerophosphate and prepared a Zn-PEO coating on a titanium surface with plasma electrolytic oxidation. The electrolyte solution was stable, and the prepared coating had good biocompatibility. The Zn-PEO surface had a microporous structure, and the zinc in the coating was released slowly. The coating enhanced the wear and corrosion resistance of the titanium and provided suitable roughness. In vitro experiments confirmed that the Zn-PEO process promoted the adhesion, proliferation, differentiation and mineralization of BMSCs and generated good biological activity. This study provides a new method for improving the biological activity of titanium and has potential for clinical application.
Data availability statement
The original contributions presented in the study are included in the article/Supplementary Material, further inquiries can be directed to the corresponding author.
Author contributions
QZ designed the study, SZ, JW, and PZ carried out the evaluations of the surface coating properties, RL, YJ, and XW performed the cell culture and cell experiments. RL, YJ, and KL carried out the data analyses, RL and JY wrote the draft of the manuscript, XN, SZ, and JW prepared the figures. KL and PZ helped in performing the analyses with constructive discussions. QZ provided guidance and supervision for the execution of experiments. All authors read and approved the final manuscript.
Funding
This work was supported by the Key Research and Development (Key R & D) plan of Jiangsu Province—social development (BE2021683), National Science Foundation of China(82160415), Guizhou Province Excellent Young Science and Technology Talents Project (YQK[2023] 022) and Guizhou Provincial Science and Technology Projects (ZK [2024] general 475), and Guizhou Provincial People’s Hospital fund [grant numbers GZSYBS (2022) 01].
Conflict of interest
The authors declare that the research was conducted in the absence of any commercial or financial relationships that could be construed as a potential conflict of interest.
Publisher’s note
All claims expressed in this article are solely those of the authors and do not necessarily represent those of their affiliated organizations, or those of the publisher, the editors and the reviewers. Any product that may be evaluated in this article, or claim that may be made by its manufacturer, is not guaranteed or endorsed by the publisher.
References
Barfeie, A., Wilson, J., and Rees, J. (2015). Implant surface characteristics and their effect on osseointegration. Br. Dent. J. 218, E9. doi:10.1038/sj.bdj.2015.171
Batool, S. A., Ahmad, K., Irfan, M., and Ur Rehman, M. A. (2022). Zn-Mn-doped mesoporous bioactive glass nanoparticle-loaded zein coatings for bioactive and antibacterial orthopedic implants. J. Funct. Biomater. 13, 97. doi:10.3390/jfb13030097
Cerqueira, A., Romero-Gavilán, F., García-Arnáez, I., Martinez-Ramos, C., Ozturan, S., Iloro, I., et al. (2021). Bioactive zinc-doped sol-gel coating modulates protein adsorption patterns and in vitro cell responses. Mat. Sci. Eng. C Mat. Biol. Appl. 121, 111839. doi:10.1016/j.msec.2020.111839
Chen, B., You, Y., Ma, A., Song, Y., Jiao, J., Song, L., et al. (2020). Zn-incorporated TiO2 nanotube surface improves osteogenesis ability through influencing immunomodulatory function of macrophages. Int. J. Nanomedicine 15, 2095–2118. doi:10.2147/ijn.S244349
Chouirfa, H., Bouloussa, H., Migonney, V., and Falentin-Daudré, C. (2019). Review of titanium surface modification techniques and coatings for antibacterial applications. Acta Biomater. 83, 37–54. doi:10.1016/j.actbio.2018.10.036
Donley, T. G., and Gillette, W. B. (1991). Titanium endosseous implant-soft tissue interface: A literature review. J. Periodontol. 62, 153–160. doi:10.1902/jop.1991.62.2.153
Feller, L., Chandran, R., Khammissa, R. A., Meyerov, R., Jadwat, Y., Bouckaert, M., et al. (2014). Osseointegration: Biological events in relation to characteristics of the implant surface, South African dent. J 69 (112), 114–117.
Feller, L., Jadwat, Y., Khammissa, R. A., Meyerov, R., Schechter, I., and Lemmer, J. (2015). Cellular responses evoked by different surface characteristics of intraosseous titanium implants. Biomed. Res. Int. 2015, 1–8. doi:10.1155/2015/171945
Garbo, C., Locs, J., D'Este, M., Demazeau, G., Mocanu, A., Roman, C., et al. (2020). Advanced Mg, Zn, Sr, Si Multi-Substituted Hydroxyapatites for Bone Regeneration. Int. J. Nanomedicine 15, 1037–1058. doi:10.2147/ijn.S226630
Gasik, M., Braem, A., Chaudhari, A., Duyck, J., and Vleugels, J. (2015). Titanium implants with modified surfaces: meta-analysis of in vivo osteointegration. Mat. Sci. Eng. C Mat. Biol. Appl. 49, 152–158. doi:10.1016/j.msec.2014.12.074
He, M., Chen, X., Cheng, K., Dong, L., Weng, W., and Wang, H. (2018). Enhanced cellular osteogenic differentiation on Zn-containing bioglass incorporated TiO2 nanorod films. J. Mat. Sci. Mat. Med. 29, 136. doi:10.1007/s10856-018-6141-6
Jin, G., Qin, H., Cao, H., Qian, S., Zhao, Y., Peng, X., et al. (2014). Synergistic effects of dual Zn/Ag ion implantation in osteogenic activity and antibacterial ability of titanium. Biomaterials 35, 7699–7713. doi:10.1016/j.biomaterials.2014.05.074
Li, B., Yang, T., Sun, R., and Ma, P. (2021). Biological and antibacterial properties of composite coatings on titanium surfaces modified by microarc oxidation and sol-gel processing. Dent. Mat. J. 40, 455–463. doi:10.4012/dmj.2020-034
Li, K., Liu, S., Hu, T., Razanau, I., Wu, X., Ao, H., et al. (2020). Optimized nanointerface engineering of micro/nanostructured titanium implants to enhance cell-nanotopography interactions and osseointegration. ACS Biomater. Sci. Eng. 6, 969–983. doi:10.1021/acsbiomaterials.9b01717
Li, X., Qi, M., Sun, X., Weir, M. D., Tay, F. R., Oates, T. W., et al. (2019). Surface treatments on titanium implants via nanostructured ceria for antibacterial and anti-inflammatory capabilities. Acta Biomater. 94, 627–643. doi:10.1016/j.actbio.2019.06.023
Li, Y., Wang, W., Yu, F., Wang, D., Guan, S., Li, Y., et al. (2020). Characterization and cytocompatibility of hierarchical porous TiO2 coatings incorporated with calcium and strontium by one-step micro-arc oxidation. Mat. Sci. Eng. C Mat. Biol. Appl. 109, 110610. doi:10.1016/j.msec.2019.110610
Lv, Y., Sun, S., Zhang, X., Lu, X., and Dong, Z. (2021). Construction of multi-layered zn-modified TiO2 coating by ultrasound-auxiliary micro-arc oxidation: Microstructure and biological property. Mat. Sci. Eng. C Mat. Biol. Appl. 131, 112487. doi:10.1016/j.msec.2021.112487
Marques Ida, S., da Cruz, N. C., Landers, R., Yuan, J. C., Mesquita, M. F., Sukotjo, C., et al. (2015). Incorporation of Ca, P, and Si on bioactive coatings produced by plasma electrolytic oxidation: The role of electrolyte concentration and treatment duration. Biointerphases 10, 041002. doi:10.1116/1.4932579
Ogle, O. E. (2015). Implant surface material, design, and osseointegration. Dent. Clin. North. Am. 59, 505–520. doi:10.1016/j.cden.2014.12.003
Ottria, L., Lauritano, D., Andreasi Bassi, M., Palmieri, A., Candotto, V., Tagliabue, A., et al. (2018). Mechanical, chemical and biological aspects of titanium and titanium alloys in implant dentistry. J. Biol. Regul. Homeost. Agents 32, 81–90.
Ripamonti, U., Roden, L. C., and Renton, L. F. (2012). Osteoinductive hydroxyapatite-coated titanium implants. Biomaterials 33, 3813–3823. doi:10.1016/j.biomaterials.2012.01.050
Rupp, F., Liang, L., Geis-Gerstorfer, J., Scheideler, L., and Hüttig, F. (2018). Surface characteristics of dental implants: A review. Dent. Mat. 34, 40–57. doi:10.1016/j.dental.2017.09.007
Sedelnikova, M. B., Komarova, E. G., Sharkeev, Y. P., Ugodchikova, A. V., Tolkacheva, T. V., Rau, J. V., et al. (2019). Modification of titanium surface via Ag-Sr- and Si-containing micro-arc calcium phosphate coating. Bioact. Mat. 4, 224–235. doi:10.1016/j.bioactmat.2019.07.001
Shimabukuro, M. (2020). Antibacterial property and biocompatibility of silver, copper, and zinc in titanium dioxide layers incorporated by one-step micro-arc oxidation: A review. Antibiotics 9, 716. doi:10.3390/antibiotics9100716
Sikdar, S., Menezes, P. V., Maccione, R., Jacob, T., and Menezes, P. L. (2021). Plasma electrolytic oxidation (PEO) process-processing, properties, and applications. Nanomaterials 11, 1375. doi:10.3390/nano11061375
Stein, S., Kruck, L., Warnecke, D., Seitz, A., Dürselen, L., and Ignatius, A. (2020). Osseointegration of titanium implants with a novel silver coating under dynamic loading. Eur. Cell Mat. 39, 249–259. doi:10.22203/eCM.v039a16
Sutthavas, P., Schumacher, M., Zheng, K., Habibović, P., Boccaccini, A. R., and van Rijt, S. (2022). Zn-loaded and calcium phosphate-coated degradable silica nanoparticles can effectively promote osteogenesis in human mesenchymal stem cells. Nanomaterials 12, 2918. doi:10.3390/nano12172918
Toledano, M., Vallecillo-Rivas, M., Osorio, M. T., Muñoz-Soto, E., Toledano-Osorio, M., Vallecillo, C., et al. (2021). Zn-containing membranes for guided bone regeneration in dentistry. Polymers 13, 1797. doi:10.3390/polym13111797
Van den Borre, C. E., Zigterman, B. G. R., Mommaerts, M. Y., and Braem, A. (2022). How surface coatings on titanium implants affect keratinized tissue: A systematic review. J. Biomed. Mat. Res. B Appl. Biomater. 110, 1713–1723. doi:10.1002/jbm.b.35025
WangCai, Z. W., Wu, H., Lin, N., Yao, X., He, Z., and Liu, X. (2018). Friction and wear behaviors of Ti6Al 4V alloy treated by plasma Ni alloying. Surf. Rev. Lett. J. 25, 1850096. doi:10.1142/S0218625X18500968
Wang, L., Shang, X., Hao, Y., Wan, G., Dong, L., Huang, D., et al. (2019). Bi-functional titanium-polydopamine-zinc coatings for infection inhibition and enhanced osseointegration. RSC Adv. 9, 2892–2905. doi:10.1039/c8ra09112a
Wang, R., Ni, S., Ma, L., and Li, M. (2022). Porous construction and surface modification of titanium-based materials for osteogenesis: A review. Front. Bioeng. Biotechnol. 10, 973297. doi:10.3389/fbioe.2022.973297
Xue, W., Dahlquist, K., Banerjee, A., Bandyopadhyay, A., and Bose, S. (2008). Synthesis and characterization of tricalcium phosphate with Zn and Mg based dopants. J. Mat. Sci. Mat. Med. 19, 2669–2677. doi:10.1007/s10856-008-3395-4
Yu, L., Yin, Y., Guo, Z., Fei, Y., Wen, X., Wang, J., et al. (2023). A functional study of zinc-titanium coatings and exploration of the intrinsic correlation between angiogenesis and osteogenesis. J. Mat. Chem. B 11, 3236–3251. doi:10.1039/d3tb00119a
Keywords: plasma electrolytic oxidation, titanium, surface properties, zinc-doped porous coating, biological activity
Citation: Luo R, Jiao Y, Zhang S, Wu J, Wu X, Lu K, Zhang P, Li Y, Ni X and Zhao Q (2023) Fabrication, properties and biological activity of a titanium surface modified with zinc via plasma electrolytic oxidation. Front. Mater. 10:1202110. doi: 10.3389/fmats.2023.1202110
Received: 07 April 2023; Accepted: 21 June 2023;
Published: 04 July 2023.
Edited by:
Ri Qiu, Shandong University of Science and Technology, ChinaReviewed by:
Jing Qiu, Nanjing Medical University, ChinaTzer-Min Lee, National Cheng Kung University, Taiwan
Copyright © 2023 Luo, Jiao, Zhang, Wu, Wu, Lu, Zhang, Li, Ni and Zhao. This is an open-access article distributed under the terms of the Creative Commons Attribution License (CC BY). The use, distribution or reproduction in other forums is permitted, provided the original author(s) and the copyright owner(s) are credited and that the original publication in this journal is cited, in accordance with accepted academic practice. No use, distribution or reproduction is permitted which does not comply with these terms.
*Correspondence: Quanming Zhao, doctor8583@163.com
†These authors have contributed equally to this work and share first authorship