- 1State Key Laboratory of Geomechanics and Geotechnical Engineering, Institute of Rock and Soil Mechanics, Chinese Academy of Sciences, Wuhan, China
- 2University of Chinese Academy of Sciences, Beijing, China
- 3Department of Civil Engineering, Khwaja Fareed University of Engineering and Information Technology, Rahim Yar Khan, Pakistan
- 4Civil Engineering Department, College of Engineering, King Khalid University, Abha, Saudi Arabia
- 5Geomechanics and Geotechnics Group, Kiel University, Kiel, Germany
- 6Department of Civil Engineering, Zakir Husain College of Engineering and Technology, Aligarh Muslim University, Aligarh, India
- 7Department of Civil Engineering, Red Sea University, Port-Sudan, Sudan
- 8China Medical University Hospital, China Medical University (Taiwan), Taichung, Taiwan
Soil stabilization is a practical approach for enhancing the suitability of problematic soil in construction projects. This study focusses on analyzing the impact of the bio-enzyme Terrazyme on the engineering properties of Mirpur soil, which exhibits inadequate performance as subgrade soil, particularly in moist conditions. The study investigates key engineering characteristics, including unconfined compressive strength (UCS), California Bearing Ratio (CBR), maximum dry density (MDD), Atterberg’s Limits, and compressibility index. Additionally, X-Ray Diffraction and SEM analysis were conducted to identify the mineral composition and particle structure of Mirpur soil. It is demonstrated that the incorporation of Terrazyme enhanced the engineering properties of the soil. The findings will contribute to a better understanding of the efficacy of bio-mediated soil stabilization techniques.
1 Introduction
Clayey sand soil is a type of soil commonly encountered in construction projects. It is characterized by a combination of sand and clay particles, with the sand particles making up the majority of the soil composition. The advantage of clayey sand soil is its improved load-bearing capacity compared to pure clay soils. The presence of sand particles adds strength to the soil, making it more capable of supporting heavy loads (Koohpeyma et al., 2013). This characteristic is valuable in various construction scenarios, including the construction of buildings, bridges, and other structures that require stable foundations. Clayey sand soil’s ability to withstand greater loads can contribute to the overall stability and safety of the constructed infrastructure. However, clayey sand soil is not without its challenges. One of the main problems associated with this soil type is its susceptibility to changes in moisture content. Clayey sand tends to undergo volume changes as the moisture content fluctuates, which can lead to soil settlement or expansion (Monkul and Ozden, 2007). These volume changes can potentially affect the structural integrity of the construction, causing cracks,shifts, or even failures. Additionally, clayey sand soil can exhibit poor cohesion due to the dominance of sand particles. With less cohesive properties, clayey sand soil may be more prone to erosion, especially when exposed to flowing water or heavy rainfall (Gu et al., 2019). Erosion can undermine the stability of slopes, embankments, and other earthworks. Proper moisture control and stabilization techniques, such as compacting and adding stabilizing agents, may be necessary to mitigate these issues and ensure the long-term stability of the project. Soil stabilization offers an efficient and practical solution for making problematic soils suitable for construction. Conventional additives such as lime (Zhou et al., 2023), rice husk ash (Canakci et al., 2015), bagasse ash (Osinubi et al., 2009), fly ash (Rao and Subbarao, 2012), and granulated blast slag (Thomas et al., 2018) have been widely used. However, non-conventional stabilizers, known as biological stabilizers, have gained attention in recent years. These biological products, including microbes (Ahmad et al., 2023; Li et al., 2023), Terrazyme (Saini and Vaishnava, 2015), and other enzymatic stabilizers (Ganapathy et al., 2017), are composed of extracts from vegetables and sugar cane. The use of biological soil stabilization has been growing worldwide due to its economic feasibility and effectiveness in stabilizing expansive soils.
Enzymatic soil stabilization originated from the utilization of biological by-products in horticulture, and its adaptation has led to the development of a material suitable for soil stabilization in construction. These bio-enzymes enhance the bonding capacity and wetting of the soil particles, transforming them into easily workable and densely compacted structures, resulting in a more durable and robust structure (Thomas and Rangaswamy, 2020). Enzymes act as catalysts, accelerating chemical reactions without becoming part of the end product. In soil stabilization, enzymes prevent loss of density and excessive water absorption by promoting tight bonding between particles and reducing the thickness of the water layer adsorbed by the soil (Pooni et al., 2021). The reaction between soil and bio-enzymes primarily involves an interaction between the clay content of the soil sample and the bio-enzyme. Clayey soils have a net negative charge and attract positively charged ions, known as replaceable cations. These cations can be replaced or exchanged by cations of another type. The presence of negatively charged clay facets hinders the free movement of cations within the clay structure, leading to changes in osmotic pressure and attempts to balance the cation concentration (Naik et al., 2016). The exchange of cations in clayey soils results in swelling and shrinkage. The effectiveness of a stabilizing agent mixed into a soil specimen depends on the valence and size of the cation. Smaller cations have greater mobility and can pull the soil structure together, removing water trapped in the soil particles and enhancing the molecular structure of clay particles (Mitikie and Lee, 2019). The swelling potential of soil poses a challenge, as it arises from the presence of adsorbed water on fine soil particles. This layer of adsorbed water governs the swelling and shrinkage potential of soils. Pure mechanical means cannot eliminate this film of absorbed water; however, variations in water content through temperature effects or mechanical means can alter the amount of water held (Prost et al., 1998). This provides an ideal environment for biological enzymes to operate. The action of bio-enzymes reduces the dipole moment of water molecules, causing their dissociation into positive hydrogen ions and hydroxyl ions. Hydroxyl ions separate into hydrogen and oxygen, while hydrogen ions convert into hydronium ions that can donate or accept charges. Most colloidal particles in soils have a negative charge, and the thin layer of water absorbed around these particles contains positively charged ions for electrical charge balance. The negatively charged hydroxyl ions or positive charges of the hydronium ions attract metal cations, adhering to the particle surface. Enzyme formulation reduces the electrical conduction of water molecules, allowing anions to apply sufficient force on cations in the adsorbed water film. This breaks the electrostatic potential barrier and transfers metal ions from the absorbed water to free water. As the film of absorbed water acting on soil particles reduces in thickness, soil particles lose their swelling potential, resulting in a more friable and workable structure (Thomas and Rangaswamy, 2021), as shown in Figure 1. The liberated hydrogen ions can react with hydroxyl ions to form water and gaseous hydrogen, initiating the process again.
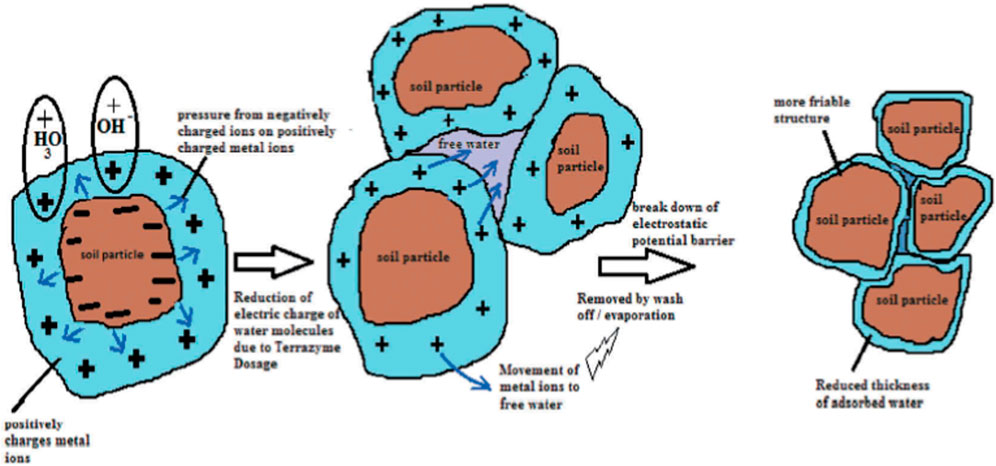
FIGURE 1. Mechanism underlying the impact of Terrazyme on the reduction of the thickness of the adsorbed water layer (Thomas and Rangaswamy, 2021).
A number of studies have provided evidence on the positive impact of introducing specific enzymes into soil (Thomas et al., 2018; Hoang et al., 2019; Renjith et al., 2020; Yan et al., 2022). Some examples of bio-enzymes available in the commercial market include Permazyme (Peng et al., 2011), Renolith (Singh and Garg, 2015), Fujibeton (Rajoria and Kaur, 2014), and Terrazyme (Saini and Vaishnava, 2015). These enzymes have been found to bring about significant improvements in both the chemical and physical properties of the soil within a relatively short period, a process that would normally require several years to occur naturally. Laboratory experiments conducted by these researchers have demonstrated that commercial enzyme products, such as Terrazyme, can effectively reduce the liquid limit of the soil, lower the optimum moisture content, decrease the soil hydraulic conductivity, and enhance the bearing capacity as determined by the CBR test (Bara and Tiwary, 2023). Terrazyme, among other enzymes, has been extensively employed by researchers, where notable enhancements and alterations in soil properties have been observed. Notably, Saini and Vaishnava (2015) and Shankar et al. (2009) found that soil-aggregate mixtures treated with enzymes require less compaction effort to achieve the same level of compaction compared to untreated soils. This reduced compaction effort can be attributed to the enzymes’ ability to diminish the thickness of the water layer surrounding clay particles, reduce water surface tension, and facilitate easier water flow out of the soil in comparison to untreated soil. In a comparative study by (Li et al., 2011) examining the effectiveness of Terrazyme for soil stabilization compared to cement stabilization, the authors concluded that Terrazyme offers greater environmental friendliness. Additionally, they found that utilizing Terrazyme can result in cost savings of approximately 25%–30% in the construction of a pavement layer stabilized with cement. Parsons and Milburn (2003) also reported that enzyme treatment enhances the cohesion between soil particles and increases the unit weight of the soil by improving the wetting action of water. Once the enzyme interacts with the soil, these changes become permanent (Saini and Vaishnava, 2015). The manufacturers of Terrazyme have determined different specifications for soils that are feasible for treatment with Terrazyme. The main effect of Terrazyme is on the plasticity index (PI) of soil. Manufacturers claim that the plasticity index of soil stabilized with Terrazyme can be reduced by up to 10% (Eujine et al., 2014). Terrazyme makes the soil more friable and easier to compact. Terrazyme increases the MDD and reduces the OMC of the soil up to a certain limit depending upon the soil characteristics and conditions. The MDD can be increased by 4%–8% while OMC can be reduced by 2%–7% (He, 2019). A research study by Bergmann (2000) concluded that at least 2% fine content of soil is necessary for bio-enzymes to stabilize the soil. However, to achieve considerable results, the clay content must be 10%–15% of the soil specimen. Soil stabilized with Terrazyme shows more resistance against weathering and wear than soils treated with other conventional stabilizing agents. Malko et al. (2016) studied the impact of Terrazyme on two different soils containing gibbsite and hematite. The author concluded that bio-mediated soils exhibit excellent properties under different prevailing conditions. Furthermore, those soils showed no affinity to water. Ramesh and Sagar (2015) treated black cotton soil and red soil containing 21% and 18% clay content, respectively, with Terrazyme. An 8% and 15% improvement in the CBR of black cotton soil and red soil, respectively, was reported under desiccator curing, while a 12% and 25% improvement in the CBR, respectively, was recorded under air dried conditions. Similar increments in the UCS of both soils were observed.
Despite the growing interest and application of bio-enzymes in soil stabilization, there are still some gaps in the existing literature. Firstly, while previous studies have shown the positive impact of bio-enzymes on soil properties, there is a need for more comprehensive research to explore the effects and advantages of different bio-enzymes, including Terrazyme, on various soil types. Secondly, limited studies have focused on the engineering properties of clayey sand stabilized with Terrazyme. Therefore, there is a lack of understanding regarding the specific effects of Terrazyme on the key engineering characteristics, such as the UCS, CBR, MDD, Atterberg’s Limits, and compressibility index of clayey sand. Addressing these gaps will contribute to a better understanding of the efficacy of Terrazyme and enhance its potential for wide-scale application in soil stabilization projects. Therefore, the objective of this research is to evaluate the impact of Terrazyme on the engineering properties of Mirpur soil, a type of clayey sand soil with inadequate performance as a subgrade soil. We analyze changes in the UCS, CBR, MDD, Atterberg’s Limits, and compressibility index of Mirpur soil after treatment with Terrazyme. We also investigate the mineral compositions and particle structure of Mirpur soil using XRD, Fourier-transform infrared spectroscopy (FTIR), and SEM analysis. By conducting these experiments and analyzing the results, this study aims to provide insights into the effectiveness of Terrazyme as a bio-enzyme for clayey sand soil stabilization and contribute to the existing knowledge in this field.
2 Materials and methods
2.1 Soil properties
The soil used in this research study was obtained from the sideways of the road opposite the New Mirpur City housing complex, located in the Mirpur District of Azad Jammu and Kashmir, as shown in Figure 2. This particular clayey sand soil was chosen due to its observed delayed response under moist conditions. Table 1 presents the characterization of the untreated soil. Before conducting the definitive laboratory tests, preliminary trials were conducted. To identify the mineral composition present in the soil and analyze the structure of soil particles, XRD and SEM analyses were performed. These analyses provided valuable insights into the mineral composition and particle structure of the soil.
2.2 Enzyme type
The bio-enzyme known as “Terrazyme” was used in this research work. Terrazyme is a biological compound composed of alcohols, vegetables, and sugarcane extracts. This specific product was originally procured from Nature Plus, Inc. Stratford, United States. According to the manufacturers, this product is non-toxic, non-hazardous, eco-friendly, and biodegradable. FTIR analysis was carried out primarily to analyze the presence of different chemical groups and the chemical bonding between those groups. The secondary objective of this analysis was to check whether the ingredients indicated by the manufacturer are present in this bio-enzyme or not. The physical and chemical characteristics of Terrazyme are given in Table 2.
2.3 Modified proctor test
In this study, the Modified Proctor test was conducted to evaluate the compaction characteristics of soil samples. Representative soil samples were collected from the site and prepared by removing organic materials and debris. The samples were then air-dried or adjusted to the desired moisture content. The test was performed using a cylindrical proctor mold and a rammer. The compacted soil specimens were prepared in three layers, with each layer compacted uniformly with 25 blows. The moisture content and dry density of each specimen were measured, and the data points were used to construct a compaction curve. The maximum dry density and optimum moisture content were determined from the curve, providing valuable information about the soil’s compaction properties.
2.4 Atterberg’s limit
The soil samples were then prepared by removing any large particles or debris and were subjected to a specific treatment process. The Atterberg’s limit tests, including the liquid limit and plastic limit, were conducted according to standardized procedures. The liquid limit test involved determining the moisture content at which the soil transitions from a liquid to a plastic state, while the plastic limit test determines the moisture content at which the soil can no longer be molded without crumbling. The results of these tests provide important information about the treated soil’s behavior, such as its plasticity, compressibility, and potential for volume change, which are crucial for engineering and construction applications.
2.5 California bearing ratio (CBR) test
The treated soil samples were prepared and compacted in a CBR mold at a specified moisture content and compaction energy. The CBR test involved subjecting the compacted soil specimen to incremental vertical loads and measuring the corresponding penetration resistance. The ratio of the penetration resistance of the treated soil to that of a standard material at the same moisture content and compaction energy yields the CBR value. The CBR values provide an indication of the treated soil’s load-bearing capacity and its suitability for various engineering applications, such as pavement design and subgrade evaluation.
2.6 Unconfined compressive strength (UCS)
The UCS test was conducted to assess the strength characteristics of treated soil samples. The UCS test involved applying a compressive load to a cylindrical soil specimen in an unconfined condition, without the application of any lateral pressure. The load was increased gradually until failure occurred, and the maximum load at failure was recorded. The UCS value represents the maximum compressive stress that the treated soil can withstand before failure. This information is crucial in assessing the stability and load-bearing capacity of the treated soil in engineering applications such as slope stability analysis, foundation design, and earthworks construction.
2.7 Soil mineral identification using X-ray diffraction (XRD)
A detailed X-ray diffraction test was carried out on an oven-dried soil sample. The minerals identified by the interpolation of XRD results were illite, mica, calcite, quartz, quartz low, and magnesium calcite. “MATCH” software was used to identify the minerals from the XRD results. This software contains a database of organic and inorganic materials, matches the peaks of XRD results with the database, and gives a description of minerals present in the soil.
A graphical representation of the XRD results is shown in Figure 3.
2.8 Fourier-transform infrared spectroscopy [FTIR] of terrazyme
The primary intention in conducting this test was to produce a likely biological product in Pakistan and the secondary objective was to check whether the ingredients listed by the manufacturer are present in the product or not. The manufacturers claim that this specific product is made up of vegetable and sugarcane extracts; these constituents are abundantly available in Pakistan. The primary principle of FTIR analysis is that a source of infrared rays produces rays of different wavenumbers and these rays are transmitted through a material. After this, a graphical representation of the peaks produced, plotting the wavenumber against transmittance, is obtained. Then, these peaks are interpolated by using the datasheet of the infrared rays. The results of the FTIR analysis are shown in Figure 4; Table 3.
3 Experimental methodology
The soil samples collected were allowed to air dry at room temperature (25°C ± 5°C) and humidity (45% ± 10%) for the purpose of determining their index and strength properties. To prepare the soil for testing, the dried soil lumps were crushed into powder using a wooden hammer and passed through a sieve with a 4.75 mm opening size. All the tests conducted to determine the index and engineering properties of the soil were carried out in accordance with the prescribed test procedures outlined in ASTM standards. It was observed that different dosages of enzymes have varying effects on the same soil. Inadequate amounts of enzymes may not result in effective stabilization, while higher quantities can have adverse effects on the soil (Shankar et al., 2009). Therefore, determining the optimal dosage of TerraZyme would be advantageous in order to enhance the effectiveness of enzyme stabilization. Four different dosages of Terrazyme were selected on the basis of initial laboratory investigation of the soil. The criteria for the selection of dosages are given in Tables 4, 5.
4 Results and discussion
Initial soil investigation was carried out according to ASTM standards. Samples were cured in a moisture-controlled environment. Before placement of the treated soil samples in testing machines for different strength tests such as the UCS, CBR and Modified Proctor tests, samples were kept in open air at atmospheric pressure to provide some oxygen for the chemical reactions between the soil and Terrazyme. This is because a certain amount of oxygen is required for enzyme activity to occur.
4.1 Moisture-density relationship of soil at different terrazyme dosages
The influence of moisture content (%) on the dry density was investigated at four different dosages of Terrazyme: D1, D2, D3, and D4 using Modified Proctor tests following the ASTM D1557-12 standard (D1557-12, 2021). Interestingly, the results consistently showed a similar trend for all dosages, with dry density initially increasing with increasing moisture content, reaching a maximum value, and then decreasing. According to Figure 5, Dosage-3 of Terrazyme resulted in the most significant improvement in dry density compared to the other dosages considered. Previous studies on soil stabilization agents, including enzymatic additives such as Terrazyme, have reported improvements in the moisture-density relationship of soil (Eujine et al., 2014; Bara and Tiwary, 2023). These improvements are attributed to various factors such as increased compaction efficiency, improved soil particle bonding, and enhanced water absorption characteristics. In some cases, researchers (Hinojosa et al., 2004; Baldrian et al., 2010; Steinweg et al., 2012; Tomar and Baishya, 2020) have found that higher dosages of enzymatic additives can lead to a more significant increase in the MDD and OMC, similar to the observed results at Dosage-3 in this study. Additionally, other studies (Rajoria and Kaur, 2014; Canakci et al., 2015; Ganapathy et al., 2017; Pooni et al., 2021) have highlighted the importance of considering the specific soil type, composition, and moisture content range when evaluating the effectiveness of soil stabilization additives. The response to Terrazyme or similar enzymatic additives may vary depending on these factors. Understanding the moisture-density relationship and identifying the optimum moisture content are crucial for soil compaction and engineering applications. The knowledge of the maximum dry density enables engineers and construction professionals to determine the ideal moisture range during construction processes to achieve the desired level of soil compaction and stability.
4.2 Atterberg’s limits of treated soil
The results of these tests indicated moderate improvements in the Atterberg’s limits of the treated soil. Table 6 presents the changes observed in the Atterberg’s limits of the soil at varying dosages of Terrazyme. For the LL values, it can be observed that the treated soil samples had similar values to the untreated soil (29%), indicating that the liquid limit did not experience significant changes due to Terrazyme application. This suggests that the liquid limit of the soil was not significantly affected by the enzymatic treatment. Regarding the PL values, all the treated soil samples had the same plastic limit as the untreated soil (16%). This implies that the plastic limit of the soil remained constant despite the application of Terrazyme. Therefore, the enzymatic treatment did not have a noticeable impact on the plastic limit of the soil. Examining the PI values, which are obtained by subtracting the PL from the LL, it can be observed that there were slight reductions in the plasticity index as the Terrazyme dosage increased. The untreated soil had a PI of 13%, while the treated soil samples ranged from 11% (at D-2 and D-3 dosages) to 8% (at D-4 dosage). These reductions indicate a moderate decrease in the plasticity of the soil due to the enzymatic treatment.
Previous studies (Eujine et al., 2014; Rajoria and Kaur, 2014; Khan and Taha, 2015; Patel et al., 2018; Renjith et al., 2020) investigating the impact of enzymatic additives on the Atterberg’s limits have reported varying results depending on the specific enzyme used, soil type, and treatment conditions. Some studies (Khan and Taha, 2015; Ramesh and Sagar, 2015; He, 2019) have demonstrated significant improvements in the Atterberg’s limits, including reductions in the liquid limit, plastic limit, and plasticity index. These improvements are attributed to the enzymatic treatment’s ability to modify the soil’s particle size distribution and enhance its workability. In contrast, other studies (Patel et al., 2018) have reported limited or negligible effects of enzymatic additives on the Atterberg’s limits of soil. Factors such as the enzyme dosage, soil composition, and initial soil properties can influence the effectiveness of the treatment. It is worth noting that different enzymes may have varying affinities for different soil types, which can lead to variations in their effectiveness. In the present study, the results showed moderate improvements in the Atterberg’s limits of the treated soil. The liquid limit (LL) and plastic limit (PL) remained the same as those for the untreated soil for all the Terrazyme dosages. However, there was a slight reduction in the plasticity index (PI) with increasing Terrazyme dosage, indicating a decrease in the soil’s plasticity. Comparing these findings with previous research, it is important to consider the specific enzymatic additive used in each study. Different enzymes may have different mechanisms of action and may interact with soil particles differently, leading to varying results. Additionally, variations in the soil composition and initial soil properties can influence the response to enzymatic treatment. It is also worth mentioning that the dosage of Terrazyme used in this study may have influenced the observed results. The moderate improvements in the Atterberg’s limits suggest that the dosage range employed may not have been optimized to induce significant changes. Further investigations could explore a wider range of dosages to determine if there exists an optimal dosage that yields more pronounced effects.
4.3 CBR of treated soil
The study also investigated the CBR of both treated and untreated soils under soaked and unsoaked conditions. Additionally, the swell potential of the treated soil was determined at each dosage of Terrazyme. The results indicated a significant increase in the CBR for the treated soil under both soaked and unsoaked conditions, while the swell potential decreased from 4.91% to 0.76%, which falls within the acceptable limit of less than 1%. Figure 6 illustrates the variations in the CBR (soaked and unsoaked) with different dosages of Terrazyme. The increase in the CBR for the treated soil suggests an improvement in its load-bearing capacity and resistance to deformation. The enhanced wetting and bonding capabilities of the soil particles due to the Terrazyme treatment likely contributed to the observed increase in the CBR. This improvement can have significant implications for the engineering properties of the soil, indicating its enhanced stability and suitability for construction applications. The reduction in swell potential from 4.91% to 0.76% is also noteworthy. Swell potential refers to the tendency of soil to expand when subjected to moisture. The decrease in swell potential indicates that the treated soil experienced reduced volumetric changes and improved resistance to swelling. This reduction is beneficial as excessive swelling can cause damage to structures built on expansive soils. Figure 6 demonstrates the variations in the CBR (soaked and unsoaked) with different dosages of Terrazyme. By analyzing the graph, it is possible to observe a trend of increasing CBR with higher Terrazyme dosages. The increase in the CBR is noticeable under both soaked and unsoaked conditions, indicating the consistent positive impact of Terrazyme on the load-bearing capacity of the soil. The findings of this study regarding the increase in the CBR and the decrease in swell potential are in line with previous research on the effects of enzymatic additives on soil engineering properties (Mekonnen et al., 2020; Kushwaha et al., 2018). Enzymatic treatments have been reported to improve the CBR of soils by enhancing its compaction characteristics, particle bonding, and shear strength. Additionally, the reduction in swell potential aligns with studies showcasing the ability of enzymatic additives to mitigate the swelling behavior of expansive soils. To gain a comprehensive understanding, further investigations could focus on evaluating the long-term performance of the treated soil, considering factors such as durability, cyclic loading, and environmental conditions.
4.4 Stress-strain behavior of treated soil
In this study, unconfined compression tests were conducted to analyze the stress-strain behavior of both untreated and treated soil. Different values of ultimate stress are observed, corresponding to the variation in dosages of Terrazyme. The ultimate strain is the strain that corresponds to the maximum stress point on the stress-strain curve, as shown in Figure 7; the soil sample fails after reaching this point. The application of Terrazyme led to significant changes in the behavior of the soil, as evidenced by the stress-strain curves presented in Figure 7. From the stress-strain curves for untreated soil and soil treated with Terrazyme at four different dosages (D1, D2, D3, and D4), it is observed that the stress-strain behavior of the treated soil varied depending on the dosage applied. Among the different dosages, D2 exhibited the highest stress values of 1,200 KPa at 1.8% strain. This suggests that the application of Terrazyme at the D2 dosage enhanced the strength and load-bearing capacity of the soil. However, it is noteworthy that the stress-strain curve for the D3 dosage showed stress values that were similar to those of the untreated soil. This implies that treatment with Terrazyme at the D3 dosage did not significantly alter the ultimate strength of the soil.
4.5 Microstructure analysis of soil (SEM)
The study employed SEM to analyze the microstructure of soil particles. The analysis involved using an oven-dried soil specimen. Initially, SEM images of the untreated soil sample were captured, revealing the presence of cavities, porous soil particles, and some particles wrapped in a membrane. These characteristics suggest the potential for volumetric changes when the soil is exposed to moisture. Figure 8 shows detailed SEM images of the untreated soil microstructure. Subsequently, the treated soil samples were also analyzed using SEM. The images show significant improvements in the soil structure in terms of particle arrangement and bonding between particles. This suggests that the Terrazyme treatment positively influenced the microstructure of the soil. Figure 8 depicts SEM images of the untreated soil, while Figure 9 displays SEM images of the soil treated with Terrazyme, at a higher magnification level. The SEM images provide visual evidence of the changes in the microstructure of the treated soil, supporting the findings of the other tests and analyses conducted in the study. The improved soil structure seen in the SEM images further validates the positive effects of Terrazyme on the soil’s engineering properties.
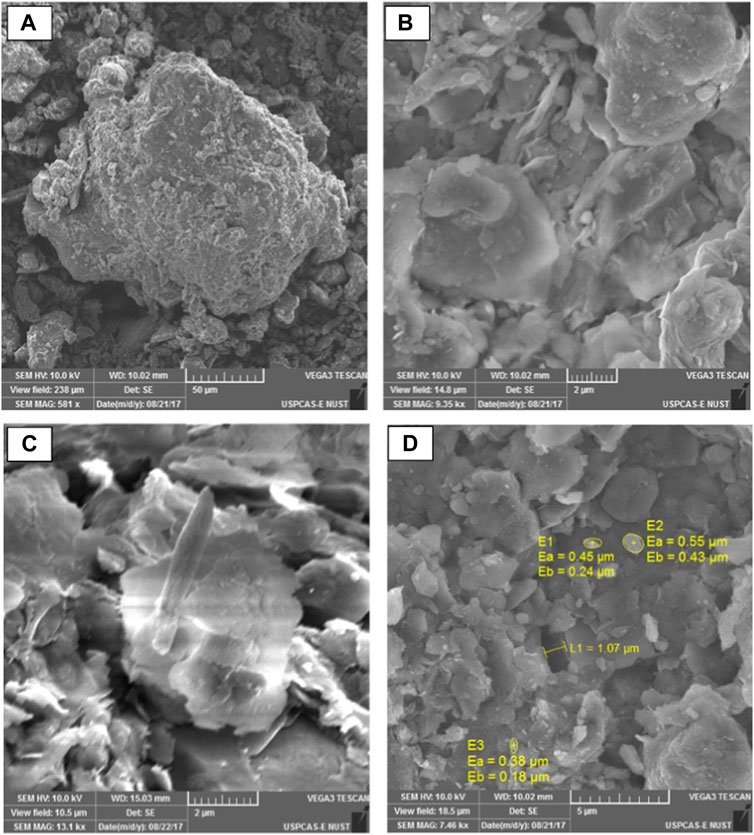
FIGURE 8. SEM micrographs of untreated soil irregular particles with a double diffused water layer: (A) ×581 magnification; (B) ×9,350 magnification; (C) ×13,100 magnification; and (D) pores size with ×7,460 magnification.
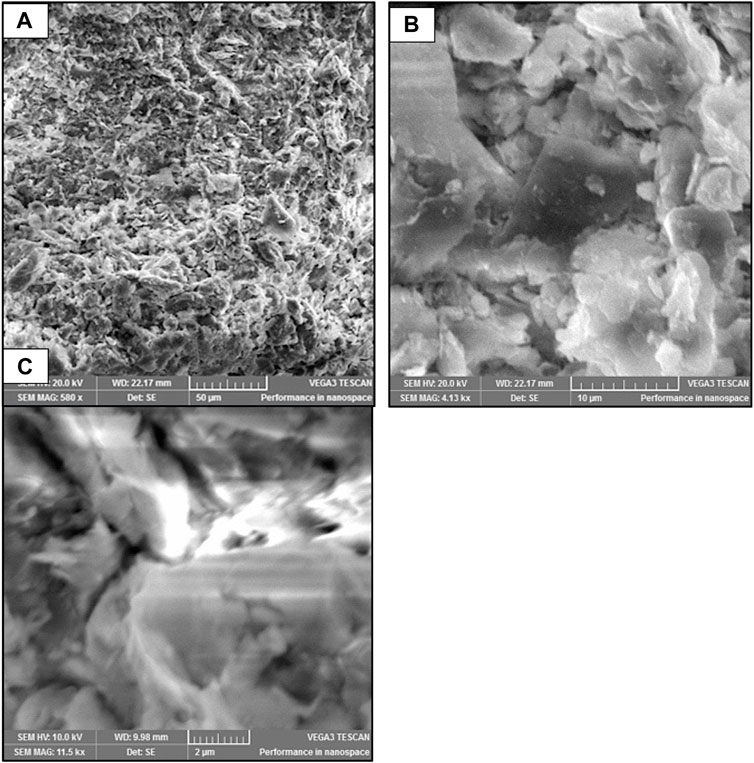
FIGURE 9. SEM micrographs of the typical arrangement of Terrazyme-treated soil particles: (A) ×580 magnification; (B) ×4,130 magnification; and (C) ×11,500 magnification.
4.6 pH value and changes in cation exchange capacity (CEC) of treated soil
The study also investigated the pH of the untreated soil, which was initially recorded as 8.02. However, after treatment with Terrazyme, a decrease in the pH of the soil was observed. This decrease in pH can be attributed to the fact that Terrazyme has a lower pH value compared to the untreated soil. The pH values of both the soil and Terrazyme were measured using an automatic pH meter. Figure 10 illustrates the variations in the pH of the treated soil at different dosages of Terrazyme. The decrease in pH after Terrazyme treatment suggests a shift towards a more acidic environment in the soil. This change can be attributed to the introduction of Terrazyme, which has an acidic pH. The low pH of Terrazyme could be attributed to the composition and characteristics of the enzymatic additives used in its formulation.
The study evaluated the CEC of both untreated and treated soil using the Yilmaz (2004). According to Yilmaz, the CEC is a function of the liquid limit of the soil. The results indicated a reduction in the CEC for the treated soil, suggesting a decrease in the concentration of exchangeable cations. The application of Terrazyme created a favorable environment for the stabilization of exchangeable cations in the soil, leading to the observed reduction in the CEC. Figure 11 illustrates the variations in the CEC of the treated soil. The decrease in the CEC of the treated soil has implications for the soil swelling behavior. The CEC is a measure of the soil’s ability to retain and exchange cations, and it plays a significant role in determining the soil’s response to moisture. A higher CEC is generally associated with greater swelling potential, as the exchangeable cations can attract water molecules and cause the soil to expand. By reducing the CEC, the Terrazyme treatment potentially mitigated the soil’s swelling behavior. The stabilization of exchangeable cations in the treated soil likely contributed to the reduction in swelling potential. This is beneficial in engineering and construction applications, as excessive swelling can lead to soil instability, structural damage, and reduced load-bearing capacity. The findings of this study align with the Yilmaz correlation and support previous research that has demonstrated the influence of enzymatic additives on the CEC and soil swelling behavior. It is important to note that the specific enzymatic additives used in previous studies may vary, and the effects on the CEC can differ. However, the general trend of reducing CEC and mitigating swelling potential with enzymatic treatments has been observed in multiple studies (Taha et al., 2013; Renjith et al., 2020).
4.7 Cost effectiveness analysis of terrazyme
The cost impact of a soil stabilizer is a crucial factor in determining the budget of a construction project, particularly when dealing with problematic soils. Conducting a cost-effectiveness study helps in selecting a soil stabilizer that is economically efficient. Table 7 provides a comprehensive cost-effective analysis of Terrazyme, comparing it with common subgrade preparation and an improved subgrade. The estimated cost of preparing a 10 km stretch of subgrade, with dimensions of 7.3 m width and 1 m height of fill, is presented in the table in PKR (million). The estimated cost of using the common subgrade is 37.689 million PKR. However, when using the improved subgrade by the removal and replacement method, the estimated cost increases to 51.859 million PKR. On the other hand, by employing Terrazyme to improve the common subgrade, the estimated cost reduces to 42.945 million PKR. The cost effectiveness of using Terrazyme to improve the common subgrade into an improved subgrade is calculated as 17%. This indicates the cost savings achieved by using Terrazyme compared to the increased cost of the improved subgrade.
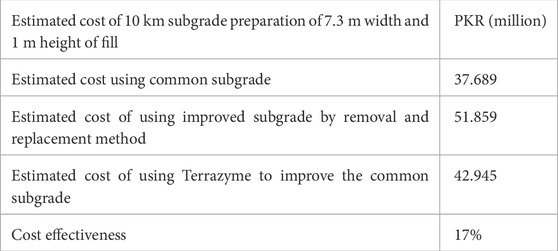
TABLE 7. Rough cost estimation as per NHA schedule rates (Rajanpur) (Shirazi, 2011).
Furthermore, Table 8 presents the cost according to two different design approaches, Design-I and Design-II, which utilize Terrazyme to improve the subgrade. According to Design-I, the cost effectiveness is calculated as US$ 51,131, representing a 29% cost saving compared to the standard design cost of US$ 72,738. Design-II involves the use of Terrazyme in combination with borrowed soil to improve the subgrade. The cost according to Design-II is US$ 61,526, with a cost effectiveness of 15.45%. The cost effectiveness percentages indicate the cost savings achieved by using Terrazyme compared to the standard design or other alternatives. This demonstrates the economic advantage of incorporating Terrazyme in the subgrade improvement process.
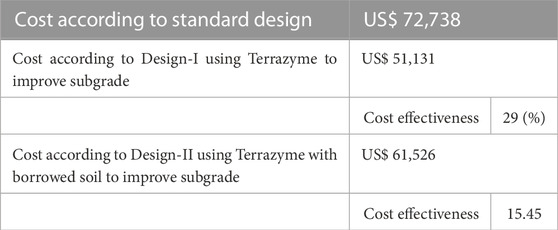
TABLE 8. USAID road rehabilitation program Honduras (Murphy and Kramer, 2003).
By conducting this cost-effective analysis, project planners and engineers can make informed decisions regarding the use of Terrazyme as a soil stabilizer, considering its cost implications and potential savings. It is important to note that the cost analysis presented in Tables 7, 8 is specific to the project and the conditions considered in the study. Project-specific factors, such as soil conditions, project size, and local cost variations, should be taken into account when assessing the cost effectiveness of soil stabilizers.
5 Conclusion and recommendations
5.1 Conclusion
The significant findings from the present study are as follows:
I. Laboratory testing results indicate that Mirpur soil possesses low strength characteristics under moist conditions, making it unsuitable as a subgrade material without appropriate treatment. The soil is reactive with Terrazyme, as evidenced by its CEC value and the presence of clay minerals detected through XRD analysis.
II. Atterberg’s limits testing revealed significant changes in the treated soil, particularly in the LL, which decreased from its untreated value. The PI of the treated soil also decreased, indicating a reduction in its plasticity due to cementation caused by Terrazyme.
III. The MDD of the treated soil increased, along with a slight increase in the OMC. Terrazyme’s ability to promote tighter bonding between soil particles resulted in denser compaction and increased moistening and bonding capacity.
IV. The UCS of the treated soil exhibited a substantial increase under both unsoaked and soaked conditions. The improved chemical bonding between soil particles, facilitated by Terrazyme, led to a more stable structure, enhancing the resistance to water penetration and erosion.
V. Significant improvements were observed in the CBR of the treated soil, indicating its enhanced ability to resist excess moisture. The reduction in swell potential demonstrated that Terrazyme reduced the absorption of water by the fine particles of soil, aligning with the requirements set by the International Building Code IBC 2006.
In conclusion, the findings conclusively demonstrate the effectiveness of Terrazyme in improving the engineering properties of Mirpur soil. The optimal dosage of Terrazyme for achieving these improvements should be further investigated, considering all relevant engineering properties. These results have important implications for the practical application and further research in this field.
5.2 Recommendations
Recommendations for future research studies include:
I. Investigate the effect of Terrazyme on the engineering characteristics of high plastic and collapsible soils. Assess the shear strength parameters and modulus of resilience of enzymatically stabilized soil to understand its performance in challenging soil conditions.
II. Explore the feasibility of using molasses, an extract of sugarcane juice, as a potential soil stabilizer. Given the significant waste of sugar molasses extracts in Pakistan, analyzing its effectiveness in soil stabilization could provide an environmentally friendly and cost-effective solution.
III. Implement the technique of applying biological products for soil stabilization in field trials. This practical application will help assess the efficacy of this method in addressing technical and financial challenges encountered in real-world scenarios.
IV. Foster efforts to develop a locally produced biological soil stabilizer in Pakistan. Since these products are derived from fermentation processes using vegetable and sugarcane extracts, which are abundant in the country, establishing a domestic production line would enhance accessibility and affordability.
V. Conduct a comprehensive study comparing the effects of conventional and non-conventional soil stabilizers on the engineering properties of soil. Explore the potential benefits of other biological soil stabilizers such as urease, permazyme, and Fuji-baton, alongside the specific enzyme (Terrazyme) investigated in this research.
VI. Undertake field investigations to validate the results obtained from laboratory testing. Field studies will provide valuable insights into the performance and practicality of biological soil stabilization techniques in real-world scenarios.
VII. Conduct biological soil stabilization at ambient temperatures, preferably below 50°C. Many biological soil stabilizers are temperature-sensitive and function optimally under mild temperature conditions. Assessing their performance under realistic temperature ranges will enhance the applicability of these stabilizers.
Data availability statement
The original contributions presented in the study are included in the article/supplementary material, further inquiries can be directed to the corresponding authors.
Author contributions
Conceptualization, MN and SU; data curation, MN, SU, SC, MA, LJ, and SI; formal analysis, MN, SC, MA, MK, LJ, MM, and SI; funding acquisition, MA, LJ, MM, and SI; investigation, MN and SU; methodology, MN, SU, SC, and MA; resources, SC, MA, MK, LJ, MM, and SI; software, MN, SC, and MK; supervision, MN; validation, SU, MA, LJ, and SI; visualization, MA, LJ, JA, and SI; writing—original draft, MN and SU; writing—review and editing, JA, MK, and MN. All authors contributed to the article and approved the submitted version.
Acknowledgments
The author extend their appreciation to the deanship of scientific research at King Khalid University for funding this work through small groups RGP.1/391/43.
Conflict of interest
The authors declare that the research was conducted in the absence of any commercial or financial relationships that could be construed as a potential conflict of interest.
Publisher’s note
All claims expressed in this article are solely those of the authors and do not necessarily represent those of their affiliated organizations, or those of the publisher, the editors and the reviewers. Any product that may be evaluated in this article, or claim that may be made by its manufacturer, is not guaranteed or endorsed by the publisher.
References
Ahmad, J., Khan, M. A., and Ahmad, S. (2023). State of the art on factors affecting the performance of MICP treated fine aggregates. Mater. Today Proc. doi:10.1016/j.matpr.2023.04.087
Baldrian, P., Merhautová, V., Petránková, M., Cajthaml, T., and Šnajdr, J. (2010). Distribution of microbial biomass and activity of extracellular enzymes in a hardwood forest soil reflect soil moisture content. Appl. Soil Ecol. 46 (2), 177–182. doi:10.1016/j.apsoil.2010.08.013
Bara, S. M., and Tiwary, A. K. (2023). Effect of waste foundry sand and Terrazyme on geotechnical characteristics of clay soil. Mater. Today Proc. doi:10.1016/j.matpr.2023.03.380
Bergmann, R. (2000). Soil stabilizers on universally accessible trails. San Dimas, CA: USDA Forest Service, San Dimas Technology and Development Center.
Canakci, H., Aziz, A., and Celik, F. (2015). Soil stabilization of clay with lignin, rice husk powder and ash. Geomechanics Eng. 8 (1), 67–79. doi:10.12989/gae.2015.8.1.067
D1557-12 (2021). A standard test methods for laboratory compaction characteristics of soil using modified effort (56,000 ft-lbf/ft3 (2,700 kN-m/m3)). D1557-12.
Eujine, G. N., Somervell, L. T., Chandrakaran, S., and Sankar, N. (2014). Enzyme stabilization of high liquid limit clay. Electron. J. Geotechnical Eng. 19, 6989–6995.
Ganapathy, G. P., Gobinath, R., Akinwumi, I. I., Kovendiran, S., Thangaraj, M., Lokesh, N., et al. (2017). Bio-enzymatic stabilization of a soil having poor engineering properties. Int. J. Civ. Eng. 15 (3), 401–409. doi:10.1007/s40999-016-0056-8
Gu, D. M., Huang, D., Liu, H. L., Zhang, W. G., and Gao, X. C. (2019). A DEM-based approach for modeling the evolution process of seepage-induced erosion in clayey sand. Acta Geotech. 14 (6), 1629–1641. doi:10.1007/s11440-019-00848-0
He, S. (2019). Chemical stabilization of expansive soils using liquid ionic soil stabilizers (LISS). Arlington, TX: The University of Texas at Arlington.
Hinojosa, M. B., Carreira, J. A., García-Ruíz, R., and Dick, R. P. (2004). Soil moisture pre-treatment effects on enzyme activities as indicators of heavy metal-contaminated and reclaimed soils. Soil Biol. Biochem. 36 (10), 1559–1568. doi:10.1016/j.soilbio.2004.07.003
Hoang, T., Alleman, J., Cetin, B., Ikuma, K., and Choi, S. G. (2019). Sand and silty-sand soil stabilization using bacterial enzyme-induced calcite precipitation (BEICP). Can. Geotechnical J. 56 (6), 808–822. doi:10.1139/cgj-2018-0191
Khan, T. A., and Taha, M. R. (2015). Effect of three bioenzymes on compaction, consistency limits, and strength characteristics of a sedimentary residual soil. Adv. Mater. Sci. Eng. 2015, 1–9. doi:10.1155/2015/798965
Koohpeyma, H. R., Vakili, A. H., Moayedi, H., Panjsetooni, A., and Nazir, R. (2013). Investigating the effect of lignosulfonate on erosion rate of the embankments constructed with clayey sand. ScientificWorldJournal 2013, 1–6. doi:10.1155/2013/587462
Kushwaha, S. S., Kishan, D., and Dindorkar, N. (2018). Stabilization of expansive soil using eko soil enzyme for highway embankment. Mater. today Proc. 5 (9), 19667–19679. doi:10.1016/j.matpr.2018.06.329
Li, J., Bi, W., Yao, Y., and Liu, Z. (2023). State-of-the-Art review of utilization of microbial-induced calcite precipitation for improving moisture-dependent properties of unsaturated soils. Appl. Sciences-Basel 13 (4), 2502. doi:10.3390/app13042502
Li, Y. J., Li, L., and Dan, H. C. (2011). Study on application of TerraZyme in road base course of road. Appl. Mech. Mater. 97, 1098–1108. doi:10.4028/www.scientific.net/amm.97-98.1098
Malko, J. A., Brazetti, R., Casagrande, M. D., and Silva, B. H. A. (2016). Applicability of soil-enzyme for paving. Jpn. Geotech. Soc. Spec. Publ. 2 (61), 2082–2085. doi:10.3208/jgssp.oth-10
Mekonnen, E., Kebede, A., Tafesse, T., and Tafesse, M. (2017). Application of microbial bioenzymes in soil stabilization. Int. J. Microbiol. 2020, 1725482. doi:10.1155/2020/1725482
Mitikie, B. B., and Lee, T. S. (2019). Experimental investigation of enzyme stabilization and its effect on clay brick. J. Constr. Eng. Manag. 145 (4). doi:10.1061/(asce)co.1943-7862.0001609
Monkul, M. M., and Ozden, G. (2007). Compressional behavior of clayey sand and transition fines content. Eng. Geol. 89 (3-4), 195–205. doi:10.1016/j.enggeo.2006.10.001
Murphy, S., and Kramer, T. (2003). “Rehabilitation of secondary pavements and the cost-benefits of treating the sub-base and base with organic soil stabilizers,” in Maintenance and rehabilitation of pavements and technological control (Guimaraes, Portugal: University of Minho) 203, 883–892.
Naik, S., Scholin, J., and Goss, B. (2016). Stabilization of phytase enzyme on montmorillonite clay. J. Porous Mater. 23 (2), 401–406. doi:10.1007/s10934-015-0093-8
Osinubi, K. J., Bafyau, V., and Eberemu, A. O. (2009). “Bagasse ash stabilization of lateritic soil,” in Appropriate technologies for environmental protection in the developing world (Dordrecht: Springer), 271. doi:10.1007/978-1-4020-9139-1_26
Parsons, R. L., and Milburn, J. P. (2003). Engineering behavior of stabilized soils. Geomaterials 2003 (1837), 20–29. doi:10.3141/1837-03
Patel, U., Singh, S., and Chaudhari, S. (2018). Effect of bio enzyme-Terrazyme on compaction, consistency limits and strength characteristics of expansive soil. Int. Res. J. Eng. Technol. 5 (3), 1602–1605.
Peng, H. T., Su, H. T., Zhang, X. P., and Wang, J. (2011). An experimental comparison of compressive strengths of soils stabilized with enzyme and ground quicklime. Adv. Mater. Res. 280, 9–12. doi:10.4028/www.scientific.net/amr.280.9
Pooni, J., Robert, D., Gunasekara, C., Giustozzi, F., and Setunge, S. (2021). Mechanism of enzyme stabilization for expansive soils using mechanical and microstructural investigation. Int. J. Geomechanics 21 (10), 2164. doi:10.1061/(asce)gm.1943-5622.0002164
Prost, R., Koutit, T., Benchara, A., and Huard, E. (1998). Sate and location of water adsorbed on clay minerals: consequences of the hydration and swelling-shrinkage phenomena. Clays Clay Minerals 46 (2), 117–131. doi:10.1346/ccmn.1998.0460201
Rajoria, V., and Kaur, S. (2014). A review on stabilization of soil using bio-enzyme. Int. J. Res. Eng. Technol. 3 (1), 75–78. doi:10.15623/ijret.2014.0301011
Ramesh, H. N., and Sagar, S. R. (2015). Effect of drying on the strength properties of Terrazyme treated expansive and non-expansive soils,” in 50th Indian geotechnical conference (Pune, India: Spinger).
Rao, K. M., and Subbarao, G. V. R. (2012). Optimum fly ash for mechanical stabilization of expansive soils using 2(2) factorial experimental design. Nat. Hazards 60 (2), 703–713. doi:10.1007/s11069-011-0040-1
Renjith, R., Robert, D. J., Gunasekara, C., Setunge, S., and O’Donnell, B. (2020). Optimization of enzyme-based soil stabilization. J. Mater. Civ. Eng. 32 (5), 3124. doi:10.1061/(asce)mt.1943-5533.0003124
Saini, V., and Vaishnava, P. (2015). Soil stabilization by using Terrazyme. Int. J. Adv. Eng. Technol. 8 (4), 566.
Shankar, A., Rai, H. K., and Mithanthaya, R. (2009). Bio-enzyme stabilized lateritic soil as a highway material. J. Indian Roads Congr. 70 (2), 141–151.
Singh, A., and Garg, P. (2015). “Evaluation of renolith as a subgrade stabilizer,” in 50th Indian geotechnical conference (Pune, Maharashtra, India: Spinger).
Steinweg, J. M., Dukes, J. S., and Wallenstein, M. D. (2012). Modeling the effects of temperature and moisture on soil enzyme activity: linking laboratory assays to continuous field data. Soil Biol. Biochem. 55, 85–92. doi:10.1016/j.soilbio.2012.06.015
Taha, M. R., Khan, T. A., Jawad, I. T., Firoozi, A. A., and Firoozi, A. A. (2013). Recent experimental studies in soil stabilization with bio-enzymes–a. Electron. J. Geotechnical Eng. 18, 3881–3894.
Thomas, A. G., and Rangaswamy, B. K. (2021). Strength behavior of enzymatic cement treated clay. Int. J. Geotechnical Eng. 15 (3), 259–272. doi:10.1080/19386362.2019.1622854
Thomas, A., Tripathi, R. K., and Yadu, L. K. (2018). A laboratory investigation of soil stabilization using enzyme and alkali-activated ground granulated blast-furnace slag. Arabian J. Sci. Eng. 43 (10), 5193–5202. doi:10.1007/s13369-017-3033-x
Thomas, G., and Rangaswamy, K. (2020). Dynamic soil properties of nanoparticles and bioenzyme treated soft clay. Soil Dyn. Earthq. Eng. 137, 106324. doi:10.1016/j.soildyn.2020.106324
Tomar, U., and Baishya, R. (2020). Seasonality and moisture regime control soil respiration, enzyme activities, and soil microbial biomass carbon in a semi-arid forest of Delhi, India. Ecol. Process. 9 (1), 50. doi:10.1186/s13717-020-00252-7
Yan, Z., Gowthaman, S., Nakashima, K., and Kawasaki, S. (2022). Polymer-assisted enzyme induced carbonate precipitation for non-ammonia emission soil stabilization. Sci. Rep. 12 (1), 8821. doi:10.1038/s41598-022-12773-6
Yilmaz, I. (2004). Relationships between liquid limit, cation exchange capacity, and swelling potentials of clayey soils. Eurasian Soil Sci. 37 (5), 506–512.
Keywords: bio-enzymes, terrazyme, soil stabilization, CBR, UCS, XRD, SEM
Citation: Nadeem M, Ullah S, Chen S, Alkahtani MQ, Khan MA, Salih R, Jian L, Mursaleen M, Islam S and Ahmad J (2023) Evaluation of engineering properties of clayey sand bio-mediated with terrazyme enzyme. Front. Mater. 10:1195310. doi: 10.3389/fmats.2023.1195310
Received: 28 March 2023; Accepted: 10 July 2023;
Published: 10 August 2023.
Edited by:
Hui Rong, Tianjin Chengjian University, ChinaReviewed by:
Jue Li, Chongqing Jiaotong University, ChinaRui Zhang, Changsha University of Science and Technology, China
Copyright © 2023 Nadeem, Ullah, Chen, Alkahtani, Khan, Salih, Jian, Mursaleen, Islam and Ahmad. This is an open-access article distributed under the terms of the Creative Commons Attribution License (CC BY). The use, distribution or reproduction in other forums is permitted, provided the original author(s) and the copyright owner(s) are credited and that the original publication in this journal is cited, in accordance with accepted academic practice. No use, distribution or reproduction is permitted which does not comply with these terms.
*Correspondence: Sami Ullah, sami.ullah@kfueit.edu.pk; Mohammad Mursaleen, mursaleenm@gmail.com; Rania Salih, rania.salih2@rsu.edu.sd; Mohammad Arsalan Khan, arsalan.khan@ifg.uni-kiel.de