- 1Construction Company, State Grid Jiangsu Electric Power Co., Ltd, Nanjing, China
- 2Huazhong University of Science and Technology, Wuhan, China
The high volume and unfavorable properties of high-water-content slurries make their treatment difficult and costly. Improving the slurries through prefabricated drains combined with surcharge or vacuum preloading represents an environmental and economic means of reusing these slurries as subsoil. As conventional methods are inefficient in dealing with low-permeability slurries, flocculation is often applied. In this study, the influence of the flocculant dosage on the drainage behavior is observed, and the secondary consolidation of the slurries is examined through laboratory tests. Flocculation is found to accelerate the consolidation process, although the flocculant dosage has an optimal value when combined with the surcharge preloading method. The coefficient of secondary consolidation is more significant in slurries improved by the flocculation-enhanced surcharge (vacuum) preloading method than in the case without flocculation. The use of flocculation must be carefully controlled.
1 Introduction
The maintenance of navigation channels and the expansion of storage capacity produce massive amounts of slurry that are dredged from rivers, lakes, seas, and reservoirs (Kang et al., 2016). The dredged slurries have several unfavorable properties, including a high water content, low bearing capacity, and low permeability, which make their management difficult (Liu et al., 2022). Traditional methods, such as stacking in the storage yard and transporting to the outside environment, do not enable any reuse of the slurries and may cause environmental problems.
The use of dredged slurries as filling and reclamation materials is a popular post-treatment application (Kang et al., 2016). Obviously, the slurries cannot be reused directly because of their unfavorable properties, so prefabricated drains combined with surcharge and vacuum preloading methods are often applied to improve the strength of the slurries (Walker and Indraratna, 2006; Indraratna et al., 2011). The mechanism of the surcharge (vacuum) preloading method increases the hydraulic gradient, which accelerates the water flow (Pu et al., 2020). However, due to the low permeability and high fine-particle content, the drainage path can easily become blocked, which reduces the efficiency of the preloading method. Consolidation takes time and the does not produce any real improvement in quality, especially when the soil layer is thick.
To improve the efficiency of the surcharge (vacuum) preloading method, flocculation can be introduced to the slurry treatment process (Wang et al., 2017; Wu et al., 2022). Flocculants change the physicochemical actions between the soil particles and gather the fine particles to form flocs, which change the inner structure of the soil layer (Kang et al., 2019). After changing the clay fabric and particle levels, the consolidation properties, including compressibility and permeability, are modified accordingly (Xu et al., 2021). The influence of the dosage and type of flocculant on the sedimentation behavior of slurries has been studied for several years. For example, Zhao et al. (2016) conducted experiments using lime as a flocculant in the vacuum preloading method. They found that flocculation reduces the loss of vacuum pressure and increases the water drainage efficiency. Wang et al. (2019) applied anionic polyacrylamide (APAM) and FeCl3 to treat dredged slurries, and reported that the composite flocculants not only increased the size of soil particles, but also stabilized the heavy metals. Lei et al. (2019) found that APAM-enhanced vacuum preloading accelerates the dissipation of pore water pressure, while Liu et al. (2020) conducted settling-column tests to optimize the dosage of flocculants. Although there has been some work on the effects of flocculation, the settlement process of APAM-enhanced preloading methods has seldom been investigated.
Previous work has usually focused on the principal consolidation. However, the secondary consolidation characteristics are also essential, having a significant effect on the safety of the ground. Studies on the secondary consolidation of slurries have been carried out by Yin et al. (2003), who proposed a rheological model based on numerous consolidation and triaxial tests, and Lei et al. (2013, 2016), who compared the secondary consolidation of two slurries and found that the secondary consolidation coefficient increased with the initial loading and decreased with subsequent loading. Slurries treated by the vacuum preloading method have a smaller secondary consolidation coefficient; additionally, the secondary consolidation coefficient increases with the water content. The abovementioned studies are based on normal soft soil. The influence of flocculation on secondary consolidation requires further study.
This paper describes the results of a series of model tests using the organic flocculant APAM to enhance the surcharge (vacuum) preloading method. Different dosages of APAM are applied to slurry samples, and the influence of the dosage on the effect of the enhancement method is examined. The time-dependent height of the slurries is recorded and analyzed to observe the promotion of water drainage. After improvement, the secondary consolidation behavior is evaluated through oedometer tests.
2 Sample preparation and laboratory tests
2.1 Test materials
The soils used in the tests were collected from a dredged slurry dumping site in Wenzhou, China. The basic properties of the soil are summarized in Table 1. The distribution of soil particle sizes is shown in Figure 1.
Previous work has shown that the organic flocculant AN926SH produces a good flocculation effect (Wu et al., 2017). Thus, this is chosen as the flocculant in the tests.
2.2 Test apparatus
The apparatus for the surcharge preloading tests is shown in Figure 2. The device measures 25 cm in length, 25 cm in width, and 60 cm in height. A scale sticker with an accuracy of 1 mm is placed on the surface of the box to allow the settlement of the soil to be measured. The bracket shown in Figure 2 consists of three metal sheets. Holes are punched in the bottom sheet and wrapped by five-sheet geotextiles to form the uniform permeable upper surface on the soil. The upper two metal sheets hold weights to form the surcharge preloading. The chamber separates into two parts (upper and bottom) so that the consolidated soil can be rapidly removed. The apparatus used for the vacuum preloading method is shown in Figure 3. Each test chamber measures 70 cm in length, 10 cm in width, and 40 cm in height. The tubes are connected to the vacuum pump and prefabricated vertical drains are laid in the bottom of the box, offering a path along which the vacuum pressure is expected to spread and allowing pore water to flow out.
2.3 Experimental procedure
Two different tests were conducted to test the influence of flocculation on promoting the water drainage of the soil and on the secondary consolidation behavior.
2.3.1 Surcharge (vacuum) preloading improvement
Surcharge preloading and vacuum preloading tests were conducted according to the following steps. First, anAN926SH solution with a mass ratio of 1:1000 was prepared by routine methods. Vaseline was applied to the inner wall of the test chamber to reduce the friction between the loading plate and the wall. The water contents of the specimens were arranged according to Table 2. After slurry samples with the target water contents had been prepared, they were mixed with the flocculant solution until large flocs were formed and supernatant appeared. The slurries and flocculants were then poured into the surcharge test chamber. The loading bracket and 11 pieces of 5.5 kg weight were then placed on the mixture to form the surcharge preloading. During slurry consolidation under preloading, the height of the soil layer was recorded. When the height had become stable, the drained pore water was drawn out, and the wet density and water content of the settled soil were measured.
The chamber shown in Figure 3 was used in the vacuum preloading tests. Prefabricated horizontal drains were laid on the bottom of the chamber and connected to the vacuum pump. After pouring the slurry/flocculant mixture into the chamber, membranes were placed on top of the chamber to seal it. The vacuum pressure offered by the pump was fixed to −50 kPa throughout the improvement process. According to pretesting, the water content of the slurries would have reduced to approximately 60% once the height of the soil reached 7 cm, at which time the vacuum pump would be closed. Details of the vacuum preloading test are presented in Table 3.
2.3.2 Consolidation test
The samples obtained in another series of surcharge preloading and vacuum preloading tests in Section 2.3.1 were then subjected to consolidation tests. Figure 4 shows the apparatus and samples for the test. The chamber for preparing samples treated by surcharge preloading is similar to that shown in Figure 5A, but it consists of two parts. The upper part of the chamber can be taken off after finishing the surcharge preloading. Then the samples were collected from the two red line height in the sublayer once the improvement stopped by cut rings to make the samples for the consolidation tests. In this series of surcharge preloading tests, 18 pieces of 5.5 kg weight were applied on the sand layer by the metal bracket. A triple low-pressure oedometer, as shown in Figure 4, was used to measure the coefficient of secondary consolidation. Loading was applied according to the standard method at 12.5 kPa, 25 kPa, 50 kPa, 100 kPa, 200 kPa, and 300 kPa in turn.
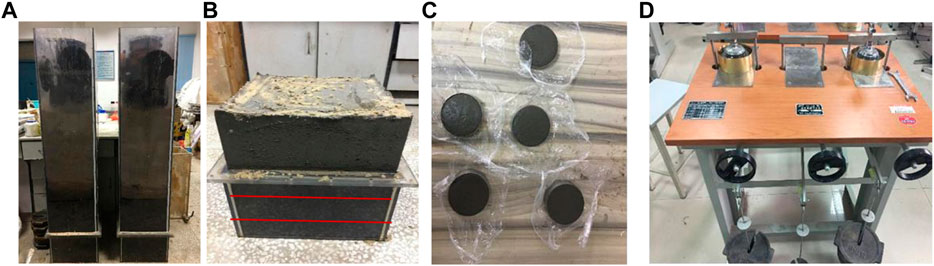
FIGURE 4. Samples and apparatus for consolidation tests: (A) chamber; (B) sampling position; (C) samples for consolidation; (D) triple oedometer.
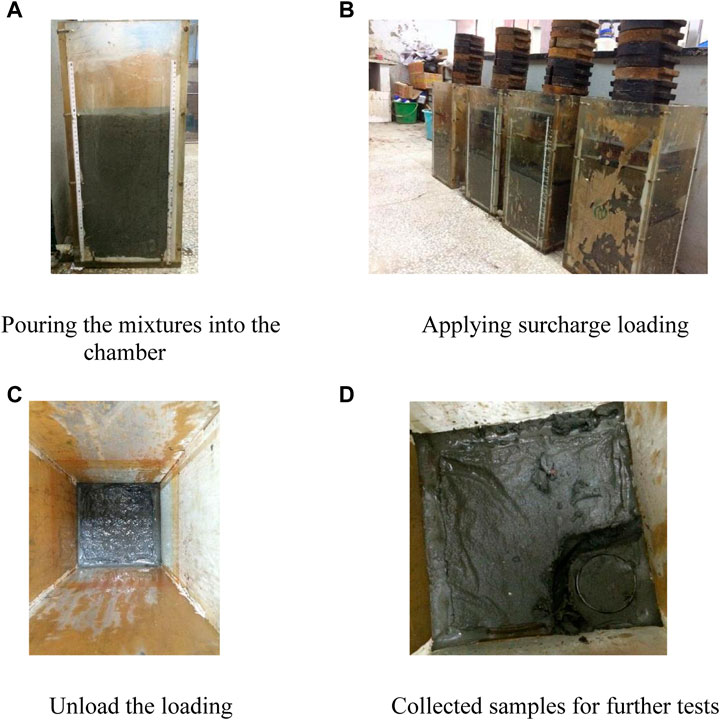
FIGURE 5. Experimental procedure for surcharge preloading tests: (A) pouring the mixtures into the chamber; (B) applying surcharge loading; (C) unload the loading; (D) collected samples for further tests.
3 Test results and analysis
3.1 Effect of flocculation on pore water drainage
Figure 6 shows the settlement of slurries with the parameters listed in Table 2. A1 represents the case in which no flocculant was added to the slurry. It is evident that, with the same water content, the addition of APAM leads to a greater degree of settlement. Moreover, the water drainage for cases with flocculation finishes within 40 h, while the consolidation is still ongoing in case A1. The speed of settlement in cases A2, A3, and A4 is much faster than that in case A1 in the early stage, indicating that flocculation significantly improves the consolidation coefficient and the permeability coefficient of the soil. Considering the flocculation mechanism, the formation of large flocs increases the void ratio and improves the drainage path of pore water, which further increases the permeability of the slurries.
Comparison of the ultimate height indicates that flocculation also influences the final improvement effect. The eventual settlement in case A4 is smaller than in cases A2 and A3, indicating that the improvement effect of flocculation is not always positively correlated with the dosage. In a specific range, the effect increases with increasing dosage because flocculation increases the permeability of the soil. However, when too much flocculant is used, the drainage effect decreases because the flocculant is combined with too much water, which makes drainage more difficult. An overdose of flocculant wraps the soil particles, preventing the inner water from flowing out.
Figure 7 shows the height variation in case B, where the equivalent water content is lower than in case A. Comparing the results in Figures 6, 7, the speed of settlement, as well as the ultimate settlement, exhibits little difference between cases A and B when no flocculant is used. For the cases with flocculant, an equivalent water content of 300% produces a larger degree of settlement and higher consolidation speed because of the greater void ratio. Moreover, the effect of flocculation is more evident with a higher water content when surcharge preloading is applied. Figure 8 illustrates the settlement of slurries under vacuum preloading combined with flocculation. As stated in Table 3, cases A and C have no flocculant added. The settlement in cases with flocculation is greater than in cases without flocculation. Different from the performance in the surcharge preloading method, the height initially decreases more slowly in slurries with flocculant than in those without flocculant, but the opposite is eventually the case. As stated when describing the test apparatus, the bottom of the surcharge preloading test is impermeable, so the water drains out through the upper surface. Considering the self-weighted settlement, soil in the lower stratum is denser and has lower permeability. In contrast, the upper part of the slurry samples has large voids and high permeability. Flocculation increases the void ratio of the upper slurry, so the initial settlement is higher. In the case of vacuum preloading, the permeable boundary is at the bottom. Under the flocculation effect, the lower stratum is thicker and denser than in the case without flocculation because the self-weight consolidation proceeds faster in the case with flocculation. Hence, the permeability is lower than in the case without flocculation. This causes a smaller initial height reduction in the case with flocculation. With time, clogging gradually occurs in the cases without flocculation, which makes the permeability lower than in cases with flocculation. Thus, the settlement develops slower thereafter.
Comparing the results with different equivalent water contents, Figure 8 indicates that the finish times for cases C and D are almost the same. In contrast, cases A and B have significantly different finish times, indicating that the flocculation effect influences samples with relatively low water contents more than those with extremely high water contents when vacuum preloading is applied. This investigation seems contrary to the results obtained with samples improved by surcharge preloading, but this is not necessarily the case. Because a water content of 300% is considered high, the smaller difference for a water content of 400% is more likely to be the result of an insufficient flocculant dosage. Cases without flocculation show different consolidation speeds because the clogging around the drain is more severe at higher water contents.
The results presented in Table 4 indicate that the slurry water content and wet density improve after surcharge preloading. Flocculation enhanced preloading method reduces the water content of the soil more than in cases without flocculation. Compared with the results of 250% water content, the case with higher initial water content improved more. Cases with flocculation have a higher wet density, which corresponds to a lower water content.
3.2 Effect of flocculation on the secondary consolidation behavior of slurries
As the treated slurries are expected to be used in infrastructure construction, knowledge of the long-term performance is essential. Thus, the secondary consolidation behavior is now studied in detail. Secondary consolidation refers to the deformation of the soil layer and the variation of the void changes over the long term, which significantly influence the reuse of dredged fills. According to previous work, the secondary consolidation behavior is related to the loading in the principal consolidation stage. Hence, different loading values were applied to samples improved by the vacuum preloading method and the surcharge preloading method. The parameters of the samples improved by surcharge loading are listed in Table 5. In group E, additional water was added with the same volume as the flocculation solution in group F.
The evolution of the void ratio for representative cases following surcharge preloading and vacuum preloading is shown in Figures 9, 10. According to Yin et al. (2003), the e-logt curve can be divided into primary consolidation stage and secondary consolidation stage. The secondary consolidation part of each curve is almost linear, and the gradient reflects the coefficient of secondary consolidation. In both cases with surcharge preloading, the curves seem more stable when larger preloading is applied. In contrast, the gradient for the cases under vacuum preloading initially increases with the loading and decreases afterward, which is similar to the results obtained by Lei et al. (2016). Comparing the results in cases E and F, it is evident that the gradients in case F are larger. To clarify this, the coefficients of secondary consolidation are calculated.
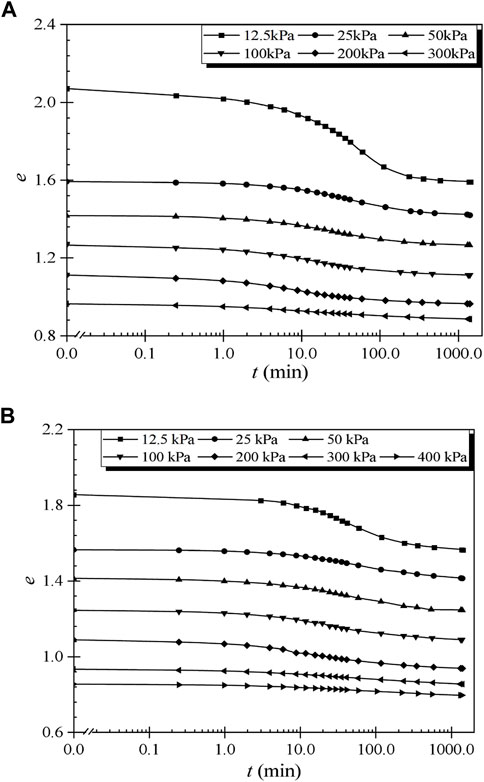
FIGURE 9. Variation of void ratio with time for samples after surcharge preloading: (A) case E; (B) case F.
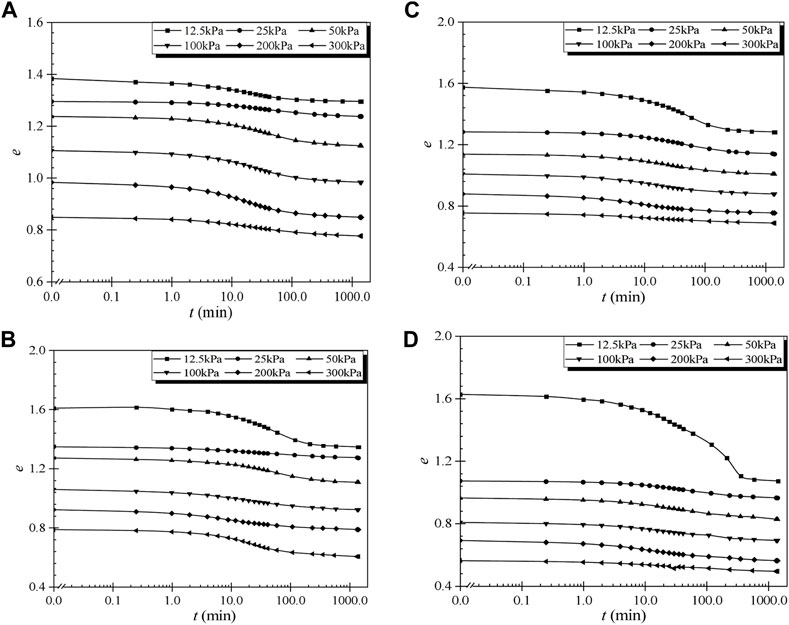
FIGURE 10. Variation of void ratio with time for samples after vacuum preloading improvement: (A) case C1; (B) case C2; (C) case D1; (D) case D2.
Using the conventional method of Yin et al. (2003), the coefficients of secondary consolidation were obtained (see Figure 11). The coefficient of secondary consolidation is larger when flocculation plays a role, and is typically 1.five to two times that of cases without flocculation. Figure 12 shows the coefficient of secondary consolidation for samples improved by the vacuum preloading method. Similar to the results shown in Figure 11, the cases with flocculation have larger coefficients in general. Moreover, the coefficient varies significantly with the loading when flocculation is applied. Comparing the results shown in Figure 12, a higher initial water content produces a larger coefficient of secondary consolidation after vacuum preloading combined with flocculation, indicating that flocculation has a greater effect on increasing the secondary consolidation coefficient when the initial water content is higher.
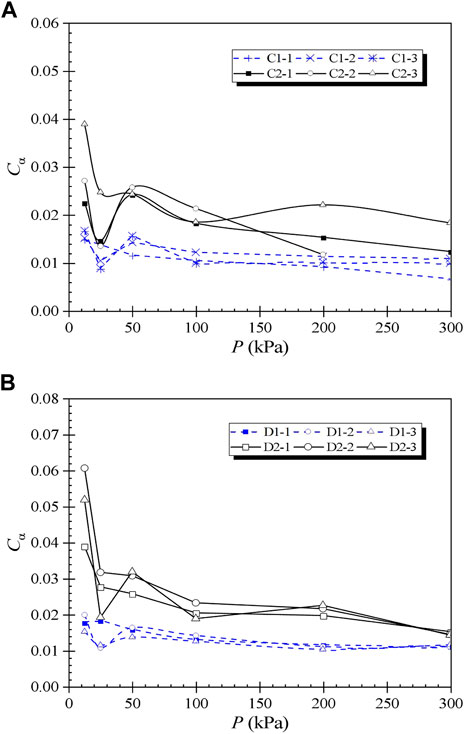
FIGURE 12. Coefficient of secondary consolidation of samples improved by vacuum preloading method: (A) Case C; (B) Case D.
Considering the flocculation mechanism, there are two reasons for the increase in the secondary consolidation coefficient. First, the flocculant APAM consists of long polymer chains with molecular weights of 4–20 million, which form a reticular structure combined with the soil particles. Because the reticular structure is nonuniform, the settlement cannot stabilize over the test duration. Second, the formation of flocs changes the pore structure in the soil. The bone formed by the flocs have high elasticity, so the degree of consolidation in the secondary consolidation stage is large.
4 Discussion
The analysis in the previous section indicates that the usage of APAM has significant advantages in improving the consolidation speed and ultimate consolidation effects, especially when the soil has a high initial water content. Flocculation increases the permeability of slurries with several fine particles in the case of both surcharge preloading and vacuum preloading. The use of flocculants prevents clogging in the slurries using the vacuum preloading method, which significantly increases the efficiency of this improvement method. However, as shown by the secondary consolidation behavior, the coefficient of secondary consolidation is enhanced following flocculation, which may cause greater post-construction settlement. Therefore, the amount of flocculant should be chosen considering the multi-effects.
For example, as stated in the results of surcharge preloading tests, settlements increase before flocculant amount reaching the optimized value but decrease after then with the increase of flocculant’s amount. Therefore, considering the influence of flocculation on both settlement and secondary consolidation, the amount of the flocculant is better to be lower than the optimized value. As shown in Figure 6, the variation of the flocculant amount changes the settlement little for the two test amounts. In that case, the effect of secondary consolidation is the determining factor.
In practice, pre-tests are suggested to be conducted before applying the proper amount of flocculants in engineering practice because the optimized value of flocculant amount is affected by the properties of the slurries. Based on the test results of this work, both primary consolidation performance and secondary consolidation performance should be considered. In a word, when using APAM in soil improvement, the dosage must be determined carefully.
5 Conclusion
This manuscript has described the performance of slurries improved by two flocculant-enhanced methods, especially the influence of flocculation on water drainage and secondary consolidation. This study has mainly been conducted through laboratory experiments. By analyzing the test results, the following conclusions can be drawn.
• The application of flocculant improves the permeability coefficient. Furthermore, it improves the speed of settlement and the ultimate value of settlement.
• There is an optimized flocculant dosage from the perspective of water drainage. When the dosage of APAM is lower than the optimal value, an increase in the dosage will improve the efficiency of the surcharge preloading method. However, the efficiency decreases when the dosage exceeds the optimal value.
• In both the surcharge preloading and vacuum preloading processes, the influence of a sufficient dosage of flocculant is more significant when the initial water content is higher.
• The consolidation tests show that the application of APAM increases the secondary consolidation coefficient, which would cause larger post-construction settlement. Therefore, the dosage of APAM needs to be determined carefully prior to its application.
Data availability statement
The original contributions presented in the study are included in the article/supplementary material further inquiries can be directed to the corresponding author.
Author contributions
Data collection, JC; Formal analysis, ZF; Funding acquisition, CH and KS; Investigation, JC; Methodology, JC; Project administration, CH and RZ; Resources, CH and RZ; Writing—original draft, JC, CH and QP.
Funding
This work is funded by the Science and Technology Project of State Grid Jiangsu Electric Power Co., Ltd. (Grant No. J2020070).
Conflict of interest
Author CH, ZF, KS, and QP were employed by the company State Grid Jiangsu Electric Power Co., Ltd.
The remaining authors declare that the research was conducted in the absence of any commercial or financial relationships that could be construed as a potential conflict of interest.
Publisher’s note
All claims expressed in this article are solely those of the authors and do not necessarily represent those of their affiliated organizations, or those of the publisher, the editors and the reviewers. Any product that may be evaluated in this article, or claim that may be made by its manufacturer, is not guaranteed or endorsed by the publisher.
References
Indraratna, B., Rujikiatkamjorn, C., Ameratunga, J., and Boyle, P. (2011). Performance and prediction of vacuum combined surcharge consolidation at Port of Brisbane. J. Geotech. Geoenviron. Eng. 137 (11), 1009–1018. doi:10.1061/(asce)gt.1943-5606.0000519
Kang, G., Tsuchida, T., and Athapaththu, A. M. R. G. (2016). Engineering behavior of cement-treated marine dredged clay during early and later stages of curing. Eng. Geol. 209, 163–174. doi:10.1016/j.enggeo.2016.05.008
Kang, X., Bate, B., Chen, R. P., Yang, W., and Wang, F. (2019). Physicochemical and mechanical properties of polymer-amended kaolinite and fly ash–kaolinite mixtures. J. Mat. Civ. Eng. 31 (6), 04019064. doi:10.1061/(asce)mt.1943-5533.0002705
Lei, H., Wang, X., Chen, L., Huang, M., and Han, J. (2016). Compression characteristics of ultra-soft clays subjected to simulated staged preloading. KSCE J. Civ. Eng. 20 (2), 718–728. doi:10.1007/s12205-015-0343-y
Lei, H., Xu, Y., Li, X., Jiang, M., and Liu, L. (2019). Effect of polyacrylamide on improvement of dredger fill with vacuum preloading method. J. Mat. Civ. Eng. 31 (9), 04019193. doi:10.1061/(asce)mt.1943-5533.0002860
Lei, H. Y., Chen, L., Ding, X. D., Zheng, G., and Kang, J. W. (2013). Properties of secondary consolidation of two types of dredger fill sites. Chin. J. Geotech. Eng. 33 (S1), 90–96.
Liu, F., Wu, W., Fu, H., Wang, J., Hai, J., Cai, Y., et al. (2020). Application of flocculation combined with vacuum preloading to reduce river-dredged sludge. Mar. Georesour. Geotechnol. 38 (2), 164–173. doi:10.1080/1064119x.2018.1564092
Liu, S., Sun, H., Geng, X., Cai, Y., Shi, L., Deng, Y., et al. (2022). Consolidation considering increasing soil column radius for dredged slurries improved by vacuum preloading method. Geotext. Geomembr. 50 (3), 535–544. doi:10.1016/j.geotexmem.2022.01.002
Pu, H., Yang, P., Lu, M., Zhou, Y., and Chen, J. N. (2020). Piecewise-linear large-strain model for radial consolidation with non-Darcian flow and general constitutive relationships. Comput. Geotech. 118, 103327. doi:10.1016/j.compgeo.2019.103327
Walker, R., and Indraratna, B. (2006). Vertical drain consolidation with parabolic distribution of permeability in smear zone. J. Geotech. Geoenviron. Eng. 132 (7), 937–941. doi:10.1061/(asce)1090-0241(2006)132:7(937)
Wang, J., Huang, G., Fu, H., Cai, Y., Hu, X., Lou, X., et al. (2019). Vacuum preloading combined with multiple-flocculant treatment for dredged fill improvement. Eng. Geol. 259, 105194. doi:10.1016/j.enggeo.2019.105194
Wang, J., Ni, J., Cai, Y., Fu, H., and Wang, P. (2017). Combination of vacuum preloading and lime treatment for improvement of dredged fill. Eng. Geol. 227, 149–158. doi:10.1016/j.enggeo.2017.02.013
Wu, Y., Tran, Q. C., Zhang, X., Lu, Y., Xu, J., and Zhang, H. (2022). Experimental investigation on the treatment of different typical marine dredged sludges by flocculant and vacuum preloading. Arab. J. Geosci. 15 (7), 642–715. doi:10.1007/s12517-022-09839-0
Wu, Y., Zhou, Z., Wang, D., Lu, Y., Wang, J., Lin, F., et al. (2017). Experimental study of Ningbo waste slurry treatment by vacuum preloading with flocculants. J. Dalian Univ. Technol. 57 (02), 157–163.
Xu, G. Z., Qiu, C. C., Song, M. M., Cao, Y. P., and Yin, J. (2021). Flocculant effects on fluidity and strength behavior of cemented dredged clay with high water content. Mar. Georesour. Geotechnol. 39 (8), 951–961. doi:10.1080/1064119x.2020.1786198
Yin, Z. Z., Zhang, H. B., Zhu, J. G., and Li, G. W. (2003). Secondary consolidation of soft soils. Chin. J. Geotech. Eng.-Chin. Ed.- 25 (5), 521–526.
Keywords: flocculation, slurries, secondary consolidation, preloading method, drainage
Citation: Han C, Fan Z, Zhang R, Cheng J, Sun K and Peng Q (2023) Consolidation characteristics of high-water-content slurries improved by flocculation-enhanced surcharge (vacuum) preloading method. Front. Mater. 10:1166551. doi: 10.3389/fmats.2023.1166551
Received: 15 February 2023; Accepted: 27 March 2023;
Published: 13 April 2023.
Edited by:
Bing Bai, Beijing Jiaotong University, ChinaCopyright © 2023 Han, Fan, Zhang, Cheng, Sun and Peng. This is an open-access article distributed under the terms of the Creative Commons Attribution License (CC BY). The use, distribution or reproduction in other forums is permitted, provided the original author(s) and the copyright owner(s) are credited and that the original publication in this journal is cited, in accordance with accepted academic practice. No use, distribution or reproduction is permitted which does not comply with these terms.
*Correspondence: Rongjun Zhang, ce_zhangrj@hust.edu.cn