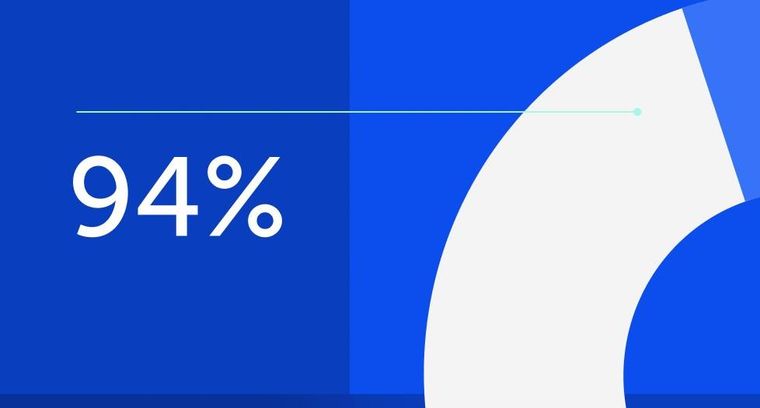
94% of researchers rate our articles as excellent or good
Learn more about the work of our research integrity team to safeguard the quality of each article we publish.
Find out more
ORIGINAL RESEARCH article
Front. Mater., 13 June 2023
Sec. Polymeric and Composite Materials
Volume 10 - 2023 | https://doi.org/10.3389/fmats.2023.1156702
Synthesis and reporting of new nanoparticles with diverse properties is important in chemistry. A one-step, rapid and controllable synthesis of the new Fe3O4 surrounded in Ti-MOF nanostructures was carried out with microwave technology. After identifying and confirming the structure, Fe3O4 surrounded in Ti-MOF nanostructures was used as a suitable catalyst with high thermal resistance and recyclable in a three-component reaction of phenylhydrazine, malononitrile and aldehyde to synthesis novel pyrazole derivatives. Continuing investigations on Fe3O4 surrounded in Ti-MOF nanostructures, its antimicrobial properties were tested on Gram-positive bacterial species, Gram-negative bacterial species and fungi bacterial. Identification of Fe3O4 surrounded in Ti-MOF nanostructures with morphology and size distribution technique (SEM), surface area technique (BET), Infrared spectroscopy (FT-IR), Energy-Dispersive X-ray spectroscopy (EDX/EDX mapping), and Vibrating Sample Magnetometer (VSM) were performed. Synthesized pyrazole derivatives with Fe3O4 surrounded in Ti-MOF nanostructures than previously reported methods have less synthesis time and high efficiency. In antimicrobial properties high effects were observed based on MIC, MBC, and MFC values.
Recently, there have been many reports on the capabilities and applications of different nanostructures. Catalyst ability (Farsi, Mohammadi, and Saghanezhad, 2021), photocatalyst ability (Karimipour et al, 2021; Eshghi et al, 2023), and biological activity such as antimicrobial and antioxidant activity (Aghaee et al, 2022; Akbari et al, 2022) are an example of them. Metal–organic frameworks (MOFs) are nanocompounds that have attracted the attention of scientists recently due to their unique capabilities (Karimi et al, 2021). One of the most important reported uses of these compounds is their energy applications (Sharafi-Badr, Hayati, and Mahmoudi, 2022). Due to the widespread use of nanostructures such as MOFs, efficient synthesis methods of these samples are an essential issue. Investigations show that choosing the method of synthesizing MOF nanostructures, developing new nanostructures, and improving their surface properties is an essential and profound challenge (Zhou et al, 2022), and the properties of the final product are affected by the physical properties and the nature of the structure (Asghar, Iqbal, and Noor, 2020). One of the most effective methods for synthesizing these nanostructures is the microwave technique. The use of microwave paths in synthesizing MOF nanostructures has improved the physicochemical properties of these nanostructures. Crystallinity, thermal stability, and particle size distribution are among these properties (Abd El Salam and Sharara, 2019). The synthesis of nanostructures in a high specific level of the product and short time are other features of this efficient method (Abdi et al, 2022). A magnetic core–shell structure of MOF magnets can be synthesized. Easy recycling in use as a catalyst for the synthesis of heterocyclic compounds is a distinctive property of core–shell magnetic nanostructures.
Pyrazole derivatives are heterocyclic compounds that have shown broad pharmacological and pharmacological properties. This kind of heterocyclic compound has been used alone or together with various other structures such as antifungal agents (Bendaha et al, 2011), antiviral agents (El-Sabbagh et al, 2009), antidepressant agents (Abdel-Aziz, Abuo-Rahma, and Hassan, 2009), antimicrobial agents (Gouda et al, 2010), and sectional agonists for nicotinic acid receptors (Van Herk et al, 2003), p38 kinase inhibitors (Graneto et al, 2007), and CDK inhibitors (Kryštof et al, 2006).
Synthetic derivatives of pyrazole are often widely used in developing anticancer agents (Kumar, Saini, et al, 2013a). Furthermore, natural products containing pyrazole have shown medicinal properties. As shown in Figure 1, some pyrazole derivatives such as 4-methylpyrazole- 3(5)-carboxylic acid, pyrazole-3(5)-carboxylic acid, pyrazophorin, and pyrazophorin B that are isolated from natural products with medicinal properties have been shown (Kumar, Kaur, et al, 2013b).
The experiment indicates that, in the pyrazole ring, the presence of cyano, aryl, or amino groups as specific substituents has an effective role in observing biological and bioactive effects (Mitchell et al, 2015). Therefore, it can be said that, from the point of view of medicinal chemistry, pyrazole heterocycles have a high potential for diversity-based organic synthesis (DOS) (Koehler, Shamji, and Schreiber, 2003).
To introduce this amount of diversity to the structure of chemical compounds, multicomponent reactions are the best possible tools (Sunderhaus and Martin, 2009; Syamala, 2009; Biggs-Houck, Younai, and Shaw, 2010).
Considering the importance of synthesizing heterocyclic compounds and reporting new methods, in this study, Fe3O4 surrounded by Ti-MOF nanostructures with the microwave method was synthesized. Fe3O4 surrounded by Ti-MOF nanostructures was used as a recyclable and efficient magnetic nanocatalyst in the synthesis of pyrazole-4-carbonitrile derivatives.
In the continuation of investigations on the properties of Fe3O4 surrounded by Ti-MOF nanostructures, its antibacterial and antifungal activities were investigated, and significant results were observed.
Solvents and chemical reagent from Merck were purchased. Fe3O4 nanostructures were purchased from Sigma-Aldrich.
The crystalline structures of the samples were investigated by X-ray powder diffraction (XRD, Rigaku SmartLab 9 kW) with a Cu-Kα radiation source (λ = 0.15406 nm). The morphology and particle size distribution of the nanostructures were analyzed by scanning electron microscopy (SEM, Hitachi Regulus 8,100) at 3.0 kV. The physical properties of the catalysts were investigated by nitrogen adsorption–desorption characterization (Micromeritics AXAP 2460) performed at −196°C. The specific surface area was calculated using the BET equation, and the pore structure parameters were calculated from the BJH method.
The types of functional groups of the samples were tested by Fourier transform infrared spectra (FT-IR, Nicolet iS50) with a wave number range of 400–4,000 cm−1.
A solution of 1 mmol Fe3O4 nanoparticle, 2 mmol Ti (NO3)4, and 2 mmol dipicolinic acid in 25 mL double-distilled water for 10 min at 75°C was stirred. Then, the mixture was put into the microwave reactor under irradiation (power of 500 W) at 25°C for 15 min. The products were cooled to room temperature. Finally, they were isolated and washed three times with deionized water/EtOH and dried at 25°C.
For synthesizing pyrazole-4-carbonitrile derivatives, in 2 mL EtOH:H2O (1:1), 1 mmol aromatic aldehydes, 1 mmol malononitrile, 1 mmol (2,4-dinitrophenyl)hydrazine, and 1 mg Fe3O4 surrounded by Ti-MOF nanostructures were added and stirred at 25°C. Reaction monitoring was performed by thin layer chromatography. After completion of the reaction, Fe3O4 surrounded by Ti-MOF nanostructures was separated by a magnet, and products were recrystallized in EtOH:H2O.
IR (KBr): 3,379, 3,319, 3,126, 3,042, 2,264, 1,621 cm−1; 1HNMR (DMSO-d6, 400 MHz): δ= 7.52 (1H, d, J = 8.7 Hz), 7.61 (1H, d, J = 9.3 Hz), 7.79 (2H, d, J = 8.84 Hz), 7.86 (1H, d, J = 8.8 Hz), 7.90 (2H, d, J = 8.4 Hz), 8.61 (1H, s), and 8.76 (1H, s) ppm; 13CNMR (DMSO-d6, 100 MHz): δ= 81.9, 114.9, 121.6, 122.7, 124.1, 128.5, 129.1, 129.4, 131.8, 133.7, 139.6, 148.2, 151.4, and 160.3 ppm; elemental analysis: C16H9FN6O4 calculated: C, 52.18; H, 2.46; N, 22.82; O, 17.38. Found: C, 52.25; H, 2.41; N, 22.79, O, 17.41.
IR (KBr): 3,386, 3,314, 3,135, 3,024, 2,247, and 1,626 cm−1; 1HNMR (DMSO-d6, 400 MHz): δ= 7.49 (1H, d, J = 8.8 Hz), 7.63 (1H, d, J = 9.2 Hz), 7.75 (2H, d, J = 8.8 Hz), 7.85 (1H, d, J = 8.7 Hz), 7.95 (2H, d, J = 8.5 Hz), 8.64 (1H, s), and 8.71 (1H, s) ppm; 13CNMR (DMSO-d6, 100 MHz): δ= 82.6, 115.3, 121.4, 122.3, 123.9, 128.9, 129.6, 129.9, 131.5, 134.1, 139.1, 148.6, 151.7, and 161.4 ppm; elemental analysis: C16H9BrN6O4 calculated: C, 44.78; H, 2.11; N, 19.58; O, 14.91. Found: C, 44.81; H, 2.09; N, 19.57, O, 14.93.
IR (KBr): 3,371, 3,331, 3,116, 3,047, 2,264, and 1,625 cm−1; 1HNMR (DMSO-d6, 400 MHz): δ= 7.54 (1H, d, J = 8.6 Hz), 7.65 (1H, d, J = 9.1 Hz), 7.71 (2H, d, J = 8.7 Hz), 7.80 (1H, d, J = 8.4 Hz), 7.91 (2H, d, J = 8.4 Hz), 8.58 (1H, s), and 8.76 (1H, s) ppm; 13CNMR (DMSO-d6, 100 MHz): δ= 83.61, 115.7, 121.8, 122.6, 124.3, 128.5, 129.1, 129.5, 131.2, 135.6, 140.1, 148.7, 153.6, and 162.1 ppm; elemental analysis: C16H9N7O6 calculated: C, 48.62; H, 2.30; N, 24.80; O, 24.28. Found: C, 48.59; H, 2.33; N, 24.84, O, 24.31.
Biological activity of Fe3O4 surrounded by Ti-MOF nanostructures and antibacterial and antifungal activities based on minimum inhibitory concentration (MIC), minimum bactericidal concentration (MBC), and minimum fungicidal concentration (MFC) values against Gram-negative bacterial strains and Gram-positive bacteria strains according to the CLSI guidelines M07-A9, M26-A, and M27-A2 were evaluated (Abdieva et al, 2022; Afrough et al, 2021; Zeraati et al, 2022; Hosseinzadegan et al, 2020a). In antimicrobial investigations, all tests were repeated three times, and the average results were reported.
In this study, Fe3O4 surrounded by Ti-MOF nanostructures was synthesized according to Figure 2.
To identify and confirm the structure of Fe3O4 surrounded by Ti-MOF nanostructures, related characterization techniques such as SEM, BET, EDX/EDX mapping, FT-IR, and VSM were used.
The morphology and particle size distribution of Fe3O4 surrounded by Ti-MOF nanostructures by SEM image (Figure 3) were investigated. According to this image, the morphology of Fe3O4 surrounded by Ti-MOF nanostructures was a uniform crystalline system with needle-shaped distribution. The homogeneous morphology with narrow particle size distribution of Fe3O4 surrounded by Ti-MOF nanostructures can be attributed to the efficient microwave-assisted synthesis under optimal conditions (microwave power: 500 W, temperature: 25°C, and time duration: 15 min). Compared to the previous sample (Moghaddam-manesh et al, 2022), the morphology of samples was homogeneous which can be related to the type of the synthesis method and also mild conditions of the microwave-assisted process. Particle size distribution of Fe3O4 surrounded by Ti-MOF nanostructures shows that the particles are in the nanorange, and the average particle size was 97 nm.
FIGURE 3. SEM image (A) and distribution histogram (B) of Fe3O4 surrounded by Ti-MOF nanostructures.
N2 adsorption/desorption isotherms of synthesized Fe3O4 surrounded by Ti-MOF nanostructures are given in Figure 4.
BET results showed that Fe3O4 surrounded by Ti-MOF nanostructures has a specific surface area of approximately 37.500 m2/g. This amount-specific surface confirmed that Fe3O4 surrounded by Ti-MOF nanostructures has a desirable surface for catalytic reactions and biological agents. It means compared to the classical route (Zeraati et al, 2021), the microwave-assisted method has been influential on the synthesis of Fe3O4 surrounded by Ti-MOF nanostructures with potential specific surface area.
The FTIR spectrum of Fe3O4 surrounded by Ti-MOF nanostructures is given in Figure 5. In the FTIR spectrum of Fe3O4 surrounded by Ti-MOF nanostructures, the peak near 3,421 cm−1 was due to the hydration of water. The absorption near 3,000 cm−1 was due to C–H groups. The peak related to carbonyl groups and C=N was near 1,600 cm−1 and 1,550 cm−1, respectively. (Bakhshi et al, 2022). The absorption due to C=C groups was shown near 1,400 cm−1. The absorption values related to Ti–O and Fe–O were given near 670 cm−1 (Al-Amin et al, 2016) and 530 cm−1 (Hosseinzadegan, Hazeri, and Maghsoodlou, 2020b), respectively.
Based on the FTIR spectrum, the peaks and absorption of a group present in the structure of Fe3O4 surrounded by Ti-MOF nanostructures were observed.
The EDX and EDS mapping proved the presence of elements and compounds used in synthesizing Fe3O4 surrounded by Ti-MOF nanostructures. The elements of Fe, C, O, and N in EDX and EDS mapping were observed. These elements were in the structure of reactants. EDX and EDS mapping of Fe3O4 surrounded by Ti-MOF nanostructures are given in Figure 6. According to these analyses, the related elements were distributed as homogeneous which confirmed the successful dispersion of Fe3O4 elements surrounded by Ti-MOF nanostructures.
FIGURE 6. EDX (A) and EDS mapping [(B1) combination; (B2) O; (B3) N; (B4) C] of Fe3O4 surrounded by Ti-MOF nanostructures.
The magnetic saturation of Fe3O4 surrounded by Ti-MOF nanostructures is given in the curve of Figure 7. The magnetic saturation value for Fe3O4 surrounded by Ti-MOF nanostructures was 0.022 emu/g. Magnetic saturation for Fe3O4 MNPs was 0.055 emu/g (Hosseinzadegan et al, 2020a). Reduction of magnetic saturation in Fe3O4 surrounded by Ti-MOF nanostructures proves the presence of Ti-MOF particles around Fe3O4.
The magnetism of Fe3O4 surrounded by Ti-MOF nanostructures makes them easy to separate after performing the desired reactions by magnets, which was another feature of synthesized Fe3O4 surrounded by Ti-MOF nanostructures.
Figure 8 shows the XRD patterns of Fe3O4 surrounded by Ti-MOF nanostructures. According to this image, the patterns related to the formation of Fe3O4 surrounded by Ti-MOF nanostructures were successfully conformed. Furthermore, according to the Debye–Scherer equation, the mean crystalline size is approximately 91 nm. The width of peaks confirmed the narrow crystalline size distribution of final products. The crystalline behavior of Fe3O4 surrounded by Ti-MOF nanostructures was in agreement with the previous literature (Li et al, 2019).
Based on the spectral data and analyzed, Figure 9 is proposed for Fe3O4 surrounded by Ti-MOF nanostructures.
Fe3O4 surrounded by Ti-MOF nanostructures was used as magnetic catalysts to synthesize pyrazole-4-carbonitrile derivatives based on Scheme 1.
SCHEME 1. Fe3O4 surrounded by Ti-MOF nanostructures as magnetic nanocatalysts in the synthesis of pyrazole-4-carbonitrile derivatives.
The first step in the synthesis of derivatives was optimization of the reaction conditions. According to Table 1, for 5-amino-1-(2,4-dinitrophenyl)-3-phenyl-1H-pyrazole-4-carbonitrile (D1), milligrams of catalyst, solvent and temperature were optimized.
In 3 mg of catalyst, 1 to 1 H2O:EtOH as a solvent, ambient temperature, and high efficiency were observed, and other derivatives listed in Table 2 were synthesized using optimal conditions.
Derivatives D2, D4, and D6 were new compounds synthesized in this study. In Scheme 2, the proposed mechanism for the synthesis of derivatives is presented.
SCHEME 2. Proposed mechanisms for the synthesis of pyrazole-4-carbonitrile derivatives by Fe3O4 surrounded by Ti-MOFs.
One of the factors that made Fe3O4 surrounded by Ti-MOF nanostructures important in synthesizing pyrazole-4-carbonitrile derivatives was their recyclability. After synthesizing the products, the catalyst with H2O and EtOH was washed and dried at ambient temperature under vacuum. After drying, it was used again in the synthesis of derivatives. Investigations proved that magnetic nanoparticles could be used up to five times without significantly reducing efficiency in the synthesis of D1 (Figure 10).
Since (2,4-dinitrophenyl)hydrazine has low reactivity, so far, two methods have been reported for the synthesis of pyrazole-4-carbonitrile derivatives using 2 (2,4-dinitrophenyl)hydrazine as a reagent, and the results are given in Table 3.
Based on the results of Table 3, the catalysts used in this study, in addition to convenient synthesis conditions of derivatives and synthesis of novel derivatives, took less time and had higher efficiency.
Although the magnetic MOF structures similar to the synthesized compound in this study have been reported so far, the difference between this study and the previous report is the use of different metals. In this study, titanium was used, but in the reported study, molybdenum was used. In addition, in the previous report, the synthesized Fe3O4/Mo-MOF compound was used as a catalyst in the synthesis of pyrano [2,3-d]pyrimidine derivatives, but Fe3O4 surrounded by Ti-MOF nanostructures synthesized in this study was used for the synthesis of pyrazole derivatives (Abdtawfeeq et al, 2022).
Antibacterial and antifungal activities of Fe3O4 surrounded by Ti-MOF nanostructures as biological activity were studied. MIC, MFC, and MBC values on Gram-negative bacterial species including Shigella dysenteriae and Klebsiella pneumoniae; Gram-positive bacteria species including Bacillus cereus and Staphylococcus epidermidis; and fungi strains including Fusarium oxysporum and Candida albicans for Fe3O4 surrounded by Ti-MOF nanostructures were studied (Table 4).
TABLE 4. Antibacterial and antifungal activities of Fe3O4 surrounded by Ti-MOF and comparison with commercial drugs.
Based on previous studies, compounds containing titanium have significant antimicrobial and antibacterial properties (He et al, 2017; Azizi-Lalabadi et al, 2019). In this study, it was found that Fe3O4 surrounded by Ti-MOF nanostructures affects all studied bacterial strains, Gram-positive bacterial strains, and fungal strains that its high effectiveness can be attributed to the presence of titanium in its structure. As mentioned in Sections 1–2, the high specific surface area of Fe3O4 surrounded by Ti-MOF nanostructures was also influential in its antibacterial and antifungal activity. A comparison of the antibacterial and antifungal properties of Fe3O4 surrounded by Ti-MOF nanostructures with cefazolin, gentamicin, tolnaftate, and terbinafine showed that synthesized Fe3O4 surrounded by Ti-MOF nanostructures has a higher effect on Shigella dysenteriae, Bacillus cereus (bacterial strains), Fusarium oxysporum, and Candida albicans (fungal strain) than cefazolin (antibacterial) and terbinafine (antifungal), which were known as a commercial antibacterial and antifungal drugs (Potbhare et al, 2019; Chouke, Dadure, et al, 2022a; Chouke, Shrirame, et al, 2022b).
In short, novel Fe3O4 surrounded by Ti-MOF nanostructures using the microwave method was synthesized, and their structure was identified and confirmed by SEM, BET, FT-IR, EDX/EDX mapping, and VSM. Analysis of SEM and BET showed that the microwave method has the uniformity of morphology and specific surface area of Fe3O4 surrounded by Ti-MOF nanostructures, which can increase its catalytic and biological properties and continue to be discussed. Fe3O4 surrounded by Ti-MOF nanostructures was used as a recyclable and efficient catalyst in synthesizing pyrazole-4-carbonitrile derivatives and proved that derivatives were synthesized under better conditions than the previously presented methods. Derivatives were synthesized in 15–30 min with the efficiency of 89%–94%. Three new pyrazole derivatives were synthesized and identified by spectral data. Another advantage of using Fe3O4 surrounded by Ti-MOF nanostructures as a catalyst was its easy separation after completion of the reaction by a magnet. In the continuation of our research on Fe3O4 surrounded by Ti-MOF nanostructures, its antibacterial and antifungal activities were evaluated, and significant results were observed. The minimum inhibitory concentration for the derivatives on the studied bacterial and fungal species was observed between 16 and 128 μg/mL. In some of the studied strains, higher effectiveness than known commercial drugs was also observed, which can be attributed to the presence of titanium and high specific surface area of it.
The original contributions presented in the study are included in the article/Supplementary Material; further inquiries can be directed to the corresponding author.
Conceptualization, BQ and ZA; methodology, SF; form analysis, HC; investigation, ZA; resources, UA; data curation, ZA; writing—original draft preparation, SH; writing—review and editing, ZA, MS and MYA; supervision, IW; project administration, RS; funding acquisition, BQ. All authors contributed to the article and approved the submitted version.
The authors express their gratitude to the Deanship of Scientific Research at King Khalid University for funding this work through the Large Research Group Project under grant number RGP.02/214/43.
The authors declare that the research was conducted in the absence of any commercial or financial relationships that could be construed as a potential conflict of interest.
All claims expressed in this article are solely those of the authors and do not necessarily represent those of their affiliated organizations, or those of the publisher, the editors, and the reviewers. Any product that may be evaluated in this article, or claim that may be made by its manufacturer, is not guaranteed or endorsed by the publisher.
The Supplementary Material for this article can be found online at: https://www.frontiersin.org/articles/10.3389/fmats.2023.1156702/full#supplementary-material
Abd El Salam, H., and Sharara, T.(2019). A novel microwave synthesis of manganese based MOF for adsorptive of Cd (II), Pb (II) and Hg (II) ions from aqua medium. Egypt. J. Chem. 62 (5), 0–851. doi:10.21608/ejchem.2019.6524.1550
Abdel-Aziz, M., Abuo-Rahma, G. E.-D. A., and Hassan, A. A. (2009). Synthesis of novel pyrazole derivatives and evaluation of their antidepressant and anticonvulsant activities. Eur. J. Med. Chem. 44 (9), 3480–3487. doi:10.1016/j.ejmech.2009.01.032
Abdi, J., Abdollah Jamal Sisi, , Hadipoor, M., and Khataee, A. (2022). State of the art on the ultrasonic-assisted removal of environmental pollutants using metal-organic frameworks. J. Hazard. Mater. 424, 127558. doi:10.1016/j.jhazmat.2021.127558
Abdieva, G. A., Patra, I., Al-Qargholi, B., Shahryari, T., Narendra Pal Singh Chauhan, , and Moghaddam-Manesh, M. (2022). An efficient ultrasound-assisted synthesis of Cu/Zn hybrid MOF nanostructures with high microbial strain performance. Front. Bioeng. Biotechnol. 10, 861580. doi:10.3389/fbioe.2022.861580
Abdtawfeeq, T. H., Farhan, Z. A., Al-Majdi, K, Mohammed Abed Jawad, , Zabibah, R. S., Riadi, Y., et al. (2022). Ultrasound-assisted and one-pot synthesis of new Fe3O4/Mo-MOF magnetic nano polymer as a strong antimicrobial agent and efficient nanocatalyst in the multicomponent synthesis of novel Pyrano [2, 3-d] pyrimidines derivatives. J. Inorg. Organomet. Polym. Mater. 33, 472–483. doi:10.1007/s10904-022-02514-7
Afrough, T., Bakavoli, M., Eshghi, H., Hamid, B., and Moghaddam-Manesh, M. (2021). Synthesis, characterization and in vitro antibacterial evaluation of novel 4-(1-(Pyrimidin-4-yl) ethyl)-12 H-pyrimido [4′, 5′: 5, 6] [1, 4] thiazino [2, 3-b] quinoxaline derivatives. Polycycl. Aromat. Compd. 41 (4), 735–745. doi:10.1080/10406638.2019.1614640
Aghaee, M., Mohammadi, K., Hayati, P., Sharafi-Badr, P., Yazdian, F., Gutierrez Alonso, A., et al. (2022). A novel 3D Ag (I) metal-organic coordination polymer (Ag-MOCP): Crystallography, Hirshfeld surface analysis, antibacterial effect and molecular docking studies. J. Solid State Chem. 310, 123013. doi:10.1016/j.jssc.2022.123013
Akbari, Z., Montazerozohori, M., Naghiha, R., Hayati, P., Micale, N., Cristani, M., et al. (2022). Some new antimicrobial/antioxidant nanostructure zinc complexes: Synthesis, crystal structure, Hirshfeld surface analyses and thermal behavior. Results Chem. 4, 100636. doi:10.1016/j.rechem.2022.100636
Al-Amin, , Mohammad, , Dey, S. C., Taslim Ur Rashid, , Md Ashaduzzaman, , and Sayed Md Shamsuddin, (2016). Solar assisted photocatalytic degradation of reactive azo dyes in presence of anatase titanium dioxide. Int. J. Latest Res. Eng. Technol. 2 (3), 14–21.
Aryan, R., Hamid, B., Nojavan, M., and Rezaei, M. (2017). Novel biocompatible glucose-based deep eutectic solvent as recyclable medium and promoter for expedient multicomponent green synthesis of diverse three and four substituted pyrazole-4-carbonitrile derivatives. Res. Chem. Intermed. 43 (8), 4731–4744. doi:10.1007/s11164-017-2908-5
Asghar, A., Iqbal, N., and Noor, T. (2020). Ultrasonication treatment enhances MOF surface area and gas uptake capacity. Polyhedron 181, 114463. doi:10.1016/j.poly.2020.114463
Azizi-Lalabadi, M., Ali, E., Divband, B., and Alizadeh-Sani, M. (2019). Antimicrobial activity of Titanium dioxide and Zinc oxide nanoparticles supported in 4A zeolite and evaluation the morphological characteristic. Sci. Rep. 9 (1), 17439–17510. doi:10.1038/s41598-019-54025-0
Bakhshi, A., Saravani, H., Rezvani, A., Sargazi, G., and Shahbakhsh, M. (2022). A new method of Bi-MOF nanostructures production using UAIM procedure for efficient electrocatalytic oxidation of aminophenol: A controllable systematic study. J. Appl. Electrochem. 52, 709–728. doi:10.1007/s10800-021-01664-9
Bendaha, H., Yu, L., Touzani, R., Souane, R., Giaever, G., Corey, N., et al. (2011). New azole antifungal agents with novel modes of action: Synthesis and biological studies of new tridentate ligands based on pyrazole and triazole. Eur. J. Med. Chem. 46 (9), 4117–4124. doi:10.1016/j.ejmech.2011.06.012
Biggs-Houck, , James, E., Younai, A., and Shaw, J. T. (2010). Recent advances in multicomponent reactions for diversity-oriented synthesis. Curr. Opin. Chem. Biol. 14 (3), 371–382. doi:10.1016/j.cbpa.2010.03.003
Chouke, P. B., Dadure, K. M., Potbhare, A. K., Bhusari, G. S., Mondal, A., Chaudhary, K., et al. (2022a). Biosynthesized δ-Bi2O3 nanoparticles from Crinum viviparum flower extract for photocatalytic dye degradation and molecular docking. ACS omega 7 (24), 20983–20993. doi:10.1021/acsomega.2c01745
Chouke, P. B., Shrirame, T., Potbhare, A. K., Mondal, A., Chaudhary, A. R., Mondal, S., et al. (2022b). Bioinspired metal/metal oxide nanoparticles: A road map to potential applications. Mater. Today Adv. 16, 100314. doi:10.1016/j.mtadv.2022.100314
Sabbagh, E., Osama, I., Baraka, M. M., Ibrahim, S. M., Pannecouque, C., Andrei, G., et al. (2009). Synthesis and antiviral activity of new pyrazole and thiazole derivatives. Eur. J. Med. Chem. 44 (9), 3746–3753. doi:10.1016/j.ejmech.2009.03.038
Eshghi, F., Mehrabadi, Z., Farsadrooh, M., Hayati, P., Javadian, H., Karimi, M., et al. (2023). Photocatalytic degradation of remdesivir nucleotide pro-drug using [Cu (1-methylimidazole) 4 (SCN) 2] nanocomplex synthesized by sonochemical process: Theoretical, hirshfeld surface analysis, degradation kinetic, and thermodynamic studies. Environ. Res. 222, 115321. doi:10.1016/j.envres.2023.115321
Farsi, R., Mohammadi, M. K., and Seyyed Jafar Saghanezhad, (2021). Sulfonamide-functionalized covalent organic framework (COF-SO 3 H): An efficient heterogeneous acidic catalyst for the one-pot preparation of polyhydroquinoline and 1, 4-dihydropyridine derivatives. Res. Chem. Intermed. 47, 1161–1179. doi:10.1007/s11164-020-04322-5
Gouda, M. A., Ma, B., Shoeib, A. I., and Khalil, A. M. (2010). Synthesis and antimicrobial of new anthraquinone derivatives incorporating pyrazole moiety. Eur. J. Med. Chem. 45 (5), 1843–1848. doi:10.1016/j.ejmech.2010.01.021
Graneto, , Matthew, J., Kurumbail, R. G., Vazquez, M. L., Sheng Shieh, H., JenniferPawlitz, L., et al. (2007). Synthesis, crystal structure, and activity of pyrazole-based inhibitors of p38 kinase. J. Med. Chem. 50 (23), 5712–5719. doi:10.1021/jm0611915
He, X., Zhang, X., Wang, X., and Qin, L. (2017). Review of antibacterial activity of titanium-based implants’ surfaces fabricated by micro-arc oxidation. Coatings 7 (3), 45. doi:10.3390/coatings7030045
Hosseinzadegan, S., Hazeri, N., Malek Taher Maghsoodlou, , Moghaddam-Manesh, M., and Shirzaei, M. (2020b). Synthesis and evaluation of biological activity of novel chromeno [4, 3-b] quinolin-6-one derivatives by SO 3 H-tryptamine supported on Fe 3 O 4@ SiO 2@ CPS as recyclable and bioactive magnetic nanocatalyst. J. Iran. Chem. Soc. 17 (12), 3271–3284. doi:10.1007/s13738-020-01990-3
Hosseinzadegan, S., Hazeri, N., and Malek Taher Maghsoodlou, (2020a). Synthesis of novel thiazolo [3, 2-a] chromeno [4, 3-d] pyrimidine-6 (7H)-ones by bioactive Fe3O4@ gly@ thiophen@ Cu (NO3) 2 as reusable magnetic nanocatalyst. Appl. Organomet. Chem. 34 (9), e5797. doi:10.1002/aoc.5797
Karimi, M., Mehrabadi, Z., Farsadrooh, M., Bafkary, R., Derikvandi, H., Hayati, P., et al. (2021). “Metal–organic framework,” in Interface science and Technology (Amsterdam, Netherlands: Elsevier), 279–387.
Karimipour, Z., Reza Jalilzadeh Yengejeh, , Haghighatzadeh, A., Mohammadi, M. K., and Rouzbehani, M. M. (2021). UV-induced photodegradation of 2, 4, 6-trichlorophenol using Ag–Fe 2 O 3–CeO 2 photocatalysts. J. Inorg. Organomet. Polym. Mater. 31, 1143–1152. doi:10.1007/s10904-020-01859-1
Koehler, A. N., Shamji, A. F., and Schreiber, S. L. (2003). Discovery of an inhibitor of a transcription factor using small molecule microarrays and diversity-oriented synthesis. J. Am. Chem. Soc. 125 (28), 8420–8421. doi:10.1021/ja0352698
Kryštof, V., Cankař, P., Fryšová, I., Jan, S., George, K., Džubák, P., et al. (2006). 4-Arylazo-3, 5-diamino-1 H-pyrazole CDK inhibitors: SAR study, crystal structure in complex with CDK2, selectivity, and cellular effects. J. Med. Chem. 49 (22), 6500–6509. doi:10.1021/jm0605740
Kumar, H., Saini, D., Jain, S., and Jain, N. (2013a). Pyrazole scaffold: A remarkable tool in the development of anticancer agents. Eur. J. Med. Chem. 70, 248–258. doi:10.1016/j.ejmech.2013.10.004
Kumar, V., Kaur, K., Gupta, G. K., and Sharma, A. K. (2013b). Pyrazole containing natural products: Synthetic preview and biological significance. Eur. J. Med. Chem. 69, 735–753. doi:10.1016/j.ejmech.2013.08.053
Li, C.-H., Huang, C.-L., Chuah, X.-F., Duraisamy Senthil Raja, , Hsieh, C.-T., and Lu, S.-Y. (2019). Ti-MOF derived TixFe1− xOy shells boost Fe2O3 nanorod cores for enhanced photoelectrochemical water oxidation. Chem. Eng. J. 361, 660–670. doi:10.1016/j.cej.2018.12.097
M'Hamed, , Mohamed, O., and OmarAlduaij, K. (2016). Green and effective one-pot synthesis of 5-Oxo-pyrazolidine and 5-Amino-2, 3-dihydro-1H-Pyrazole derivatives through Ball Milling under catalyst-free and solvent-free conditions. Asian J. Chem. 28 (3), 543–547. doi:10.14233/ajchem.2016.19397
Mitchell, L. H., Drew, A. E., ScottRibich, A., Rioux, N., Swinger, K. K., Jacques, S. L., et al. (2015). Aryl pyrazoles as potent inhibitors of arginine methyltransferases: Identification of the first PRMT6 tool compound. ACS Med. Chem. Lett. 6 (6), 655–659. doi:10.1021/acsmedchemlett.5b00071
Moghaddam-manesh, M., Sargazi, G., Roohani, M., Nooshin Gholipour Zanjani, , Khaleghi, M., and Hosseinzadegan, S. (2022). Synthesis of PVA/Fe3O4@ SiO2@ CPS@ SID@ Ni as novel magnetic fibrous composite polymer nanostructures and evaluation of anti-cancer and antimicrobial activity. Polym. Bull., 1–12. doi:10.1007/s00289-022-04584-6
Potbhare, A. K., Ratiram Gomaji Chaudhary, , Chouke, P. B., Yerpude, S., Mondal, A., Sonkusare, V. N., et al. (2019). Phytosynthesis of nearly monodisperse CuO nanospheres using Phyllanthus reticulatus/Conyza bonariensis and its antioxidant/antibacterial assays. Mater. Sci. Eng. C 99, 783–793. doi:10.1016/j.msec.2019.02.010
Sharafi-Badr, P., Hayati, P., and Mahmoudi, G. (2022). “Biological MOFs (bio-MOFs) for energy applications,” in Metal-organic framework-based nanomaterials for energy conversion and storage (Amsterdam, Netherlands: Elsevier), 81–103.
Sunderhaus, J. D., and Martin, S. F. (2009). Applications of multicomponent reactions to the synthesis of diverse heterocyclic scaffolds. Chemistry–A Eur. J. 15 (6), 1300–1308. doi:10.1002/chem.200802140
Syamala, M. (2009). Recent progress in three-component reactions. An update. Org. Prep. Proced. Int. 41 (1), 1–68. doi:10.1080/00304940802711218
Van Herk, T, J. B., Amch Van den Nieuwendijk, , Van Der Klein, P. A. M., Ijzerman, A. P., Stannek, C., Burmeister, A., et al. (2003). Pyrazole derivatives as partial agonists for the nicotinic acid receptor. J. Med. Chem. 46 (18), 3945–3951. doi:10.1021/jm030888c
Zeraati, M., Ali, M., Vafaei, S., Narendra Pal Singh Chauhan, , and Sargazi, G. (2021). Taguchi-assisted optimization technique and density functional theory for green synthesis of a novel Cu-MOF derived from caffeic acid and its anticancerious activities. Front. Chem., 972. doi:10.3389/fchem.2021.722990
Zeraati, M., Moghaddam-Manesh, M., Khodamoradi, S., Hosseinzadegan, S., Golpayegani, A., Narendra Pal Singh Chauhan, , et al. (2022). Ultrasonic assisted reverse micelle synthesis of a novel Zn-metal organic framework as an efficient candidate for antimicrobial activities. J. Mol. Struct. 1247, 131315. doi:10.1016/j.molstruc.2021.131315
Keywords: Fe3O4/Ti-MOF nanostructure, magnetic nanocatalyst, pyrazole, Gram-positive bacterial species, Gram-negative bacterial species
Citation: Qubais Saeed B, Waleed I, Chlib Alkaaby HH, Farhan Jawad S, Altimari US, Ahmed AL-Sarraj ZS, Shbeeb RT, Hadrawi SK, Suliman M and Alshahrani MY (2023) Synthesis of novel Fe3O4 nanostructures surrounded by Ti-MOF nanostructures as bioactive and efficient catalysts in three-component synthesis of new pyrazole derivatives. Front. Mater. 10:1156702. doi: 10.3389/fmats.2023.1156702
Received: 01 February 2023; Accepted: 26 May 2023;
Published: 13 June 2023.
Edited by:
Ahmed A. Abdala, Texas A&M University at Qatar, QatarReviewed by:
Payam Hayati, Iran University of Science and Technology, IranCopyright © 2023 Qubais Saeed, Waleed, Chlib Alkaaby, Farhan Jawad, Altimari, Ahmed AL-Sarraj, Shbeeb, Hadrawi, Suliman and Alshahrani. This is an open-access article distributed under the terms of the Creative Commons Attribution License (CC BY). The use, distribution or reproduction in other forums is permitted, provided the original author(s) and the copyright owner(s) are credited and that the original publication in this journal is cited, in accordance with accepted academic practice. No use, distribution or reproduction is permitted which does not comply with these terms.
*Correspondence: Ziyad Shihab Ahmed AL-Sarraj, Wml5YWRzaGloYWIuYWhtZWRhbHNhcnJhakBnbWFpbC5jb20=, emlhZC5zaGloYWJAYXUuZWR1Lmlx
Disclaimer: All claims expressed in this article are solely those of the authors and do not necessarily represent those of their affiliated organizations, or those of the publisher, the editors and the reviewers. Any product that may be evaluated in this article or claim that may be made by its manufacturer is not guaranteed or endorsed by the publisher.
Research integrity at Frontiers
Learn more about the work of our research integrity team to safeguard the quality of each article we publish.