- 1Department of Ultrasonography, The First People’s Hospital of Yuhang District, Hangzhou, China
- 2Department of Oral Implantation, Affiliated Haikou Hospital, Xiangya Medical School, Central South University (Hainan Provincial Stomatology Centre), Haikou, Hainan, China
- 3Department of Stomatology, Center for Plastic and Reconstructive Surgery, Zhejiang Provincial People’s Hospital (Affiliated People’s Hospital, Hangzhou Medical College), Hangzhou, China
Exosomes are secreted by various cells including stem cells, dendritic cells, and tumor cells, also known as the cell-derived extracellular vesicles. Exosomes, can carry informative cargos from host cells, thus have been employed as potential nanomaterials for their multifarious biological functions in biomedical fields, such as drug and genes delivery, tumor targeting, and disease treatment. Recently, the biological applications of exosomes in bone tissue engineering have gained increasing attention. Some important progress has been made while the tissue regeneration and functional recovery of boneremain as the key challenges to be addressed. In this article, we first made a summary of exosomes and their applications in the regeneration of bone and cartilage tissue. Then, modification approaches used for exosomes to equip them with excellent capacities are summarized. Finally, current concerns and future outlooks of exosomes in bone/cartilage tissue engineering and regeneration are discussed.
1 Introduction
Bone-related diseases have a high incidence for many pathogenesis, including infection, inflammation, traumatism, malignant tumor, ageing, and congenital malformation. (Liu et al., 2018; Hayashi et al., 2019; Wan et al., 2020; Ling et al., 2021; Yang et al., 2022) The regeneration and reconstruction of bone tissues embraces an extensive set of biological and clinical significance. For clinical strategies, the main concept is the morphological and functional reestablishment of bone tissue. Current therapeutic treatments for bone tissues include bone transplantation (e.g., autogenous bone or allogeneic bone), (Behrend et al., 2016), bone substitution materials (e.g., titanium plate), (Diwu et al., 2020), stem cell transplantation (e.g., bone marrow stem cells, bMSCs), (Benavides et al., 2021), biological agents (e.g., growth factors), (Kitaura et al., 2022), gene therapy, (Gao et al., 2022), distraction osteogenesis, (Qi et al., 2009), and barrier membrane to guide bone regeneration. (Han et al., 2018) The purposes of applying bone filling materials in guided bone regeneration (GBR) procedures are: 1) supporting the barrier film to avoid collapse; (Cox et al., 2022) 2) scaffolding for new bone to grow in from the receptive area; (Roseti et al., 2017) 3) stimulating the growth of new bone from the receptive area; (Robling and Bonewald, 2020) 4) resisting to surface soft tissue pressure; (Hao et al., 2022) 5) protecting the new bone mass and avoiding its absorption. (Cosman, 2009) Therefore, four basic characteristics of bone defect repair materials must be considered: 1) biocompatibility, 2) mechanical tolerance, 3) biodegradability, and 4) induce reproduction. (Wang et al., 2021a) Over the past decades, the research and development of biological materials have been intensive, with the purpose to create or complete the designed materials with the abovementioned properties. (Wang et al., 2021a) However, since the process of bone regeneration is complicated, the biological materials using for healing bone injury/diseases are still under active investigation , and the challenges to be addressed are the poor biocompatibility, undesirable morphological regeneration, and limited functional restoration. (Tandon et al., 2018; Yang et al., 2022) Therefore, the biological materials and nanomedicines with the excellent properties of bone regeneration are highly desired.
Nowadays, some biological products have been incorporated into the clinical usages, such as human bone morphogenetic proteins (hBMPs; e.g., hBMP-2 and hBMP-7), (Salazar et al., 2016), β-tricalcium phosphate (β-TCP), (Bohner et al., 2020), and hydroxyapatite. (Palmer et al., 2008) These bone formation materials could promote the bone tissue regeneration to some extent, but there is still work to do. For examples, the hBMP-2 and hBMP-7 have been approved for use in Europe and the United States, but the high financial costs, disappointed clinical efficacy, and the adverse side effects limited the wide applications of them. (Garrison et al., 2007; Huang et al., 2014; Gillman and Jayasuriya, 2021) Moreover, the gene therapy provides a promising and alternative approach for bone tissue regeneration. For the essences of gene therapy such as the delivery of complementary DNA (cDNA) and messenger RNA (mRNA), the cost, disadvantages of current available genetic delivery methods, unpredictable osteogenic effects, safety concerns, and delivery barriers (e.g., blood bone barrier) of the genetic strategies also limited the clinical applications. (Chaudhuri et al., 1993) Therefore, the regenerative medicines, which could autonomously regulate the bone formation, have drawn more attentions than other therapies. Recently, the exosomes have been widely investigated for bone tissues regeneration, which could act as the carriers of various genes or drugs and alsoregulate the bone formation process themselves. (Guo et al., 2021a; Bei et al., 2021; Chang et al., 2022; Yao et al., 2022)
Exosomes are identified as the nanovesicles which could be constitutively released by plasma membrane fusion, with the responsibility of mediating local and systemic cell-to-cell interaction by transferring the mRNAs, miRNAs, or proteins. (Kalluri and LeBleu, 2020; Kimiz-Gebologlu and Oncel, 2022) Exosomes, with the nanostructures ranging from 50 nm to 200 nm, are an emerging promising therapeutic nanomaterials in biomedical fields, such as targeted drug delivery, clinical diagnosis, and immune regulation. (Kimiz-Gebologlu and Oncel, 2022; Paskeh et al., 2022; Yu et al., 2022) The potential of exosomes in tissue engineering has been valued as a promising strategy. (Alvarez-Erviti et al., 2011; Tevlin et al., 2022) For bone tissue engineering, the applications of exosomes are attracting significant attention. (Li et al., 2018) Various studies have demonstrated that stem cell derived exosomal-based nanomaterials could effectively restore the critical-sized bone defects and promote bone regeneration. (Li et al., 2018; Tevlin et al., 2022) Exosomal-based therapeutic strategies for various bone defects are promising. Some studies have proven that exosomes played significant parts in various physiological or pathological progresses of bone tissues regeneration, such as immunomodulation, angiogenesis, and wound healing. (Dai et al., 2020a; Li et al., 2021a; An et al., 2021; Yu et al., 2021a) Moreover, the exosomes markedly reduce the immunogenicity. (Xu et al., 2020; Liang et al., 2021) Recent studies of exosomal-based technology, led by various structural modifications and with the applications of lipid vectors for agent delivery, have triggered interests in the employment of exosomes in bone regeneration medicine as a safe, available and effective means of bone tissue restoration. (Batrakova and Kim, 2015; Barile and Vassalli, 2017) Here, we would introduce the sources of various exosomes, and also summarize the various structural modifications of exosomes. Particularly, the unique properties and biological functions of exosomal-based nanomedicines in bone tissue engineering would be highlighted, and the current challenges and prospects would also be discussed.
2 Sources and characterizations of exosomes
2.1 Construction of exosomes
Some previous studies have found that exosomes existed in abundance in blood, milk, feces, and saliva, (Ayyar and Moss, 2021; Heo and Kang, 2022), which could be secreted by various sources, such as mesenchymal stem cells (MSCs), macrophages, monocytes, and endothelial progenitor cells (Figures 1A, 2A). (Kamerkar et al., 2017; Zhou et al., 2019; Zhao et al., 2020; Sun et al., 2021) Therefore, researchers could collect various exosomes for the clinical or laboratory samples via ultracentrifugation (Figure 2B). These exosomes could polarize immune cells, osteoblasts, osteoclasts, chondrocytes, and endothelial cells (ECs) to favorable phenotypes for osteochondral regeneration. (Zhang et al., 2018) For the inclusion compounds containing nucleic acids, proteins, and metabolites (Figure 1B), exosomes could kindle some physiological changes, such as mediating over inflammatory response, affecting gene expression, and inducting signaling pathways by various load or surface modifications. (Valadi et al., 2007; Yu et al., 2021a; Lai et al., 2021; Zhong et al., 2021)
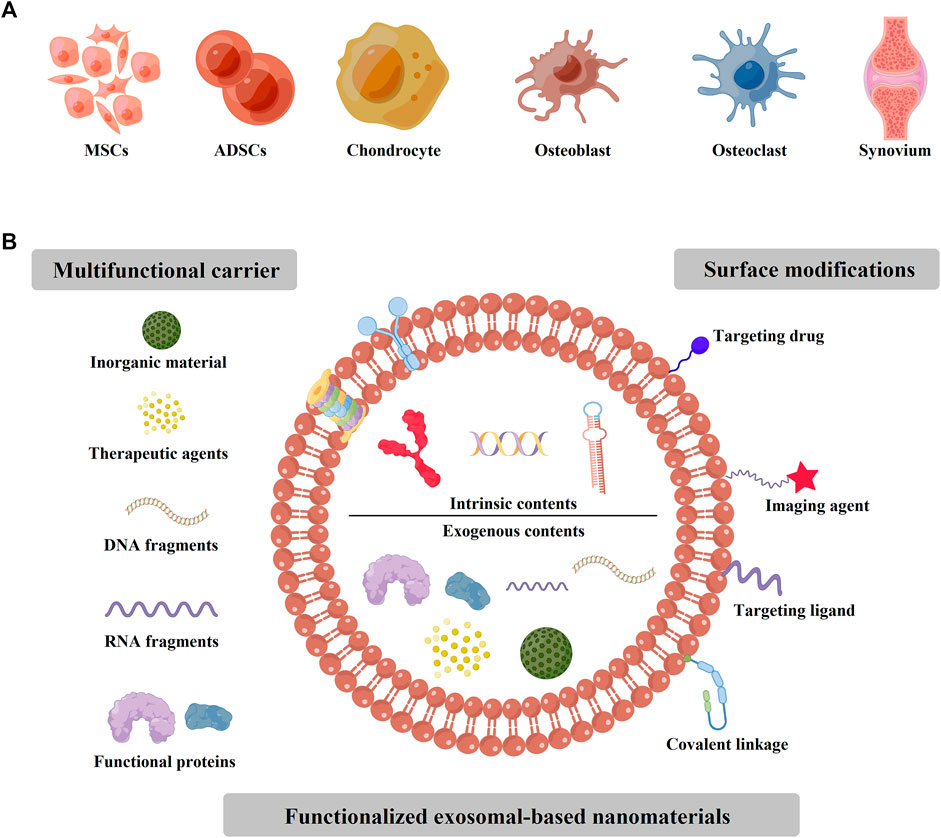
FIGURE 1. The sources of exosomes for the applications of bone/cartilage tissue engineering. (A) The cells or tissues sources of exosomes applied for bone/cartilage tissue engineering, which include MSCs, ADSCs, chondrocyte, osteoblast, osteoclast, and synovium. (B) The intrinsic and exogenous contents of various functionalized exosomal-based nanomaterials. For some materials, the exosomes were applied as the multifunctional carriers, such as inorganic material, drugs, DNA or RNA fragments, and some functional proteins. Moreover, some surface modifications could be loaded onto the exosome membrane, such as imaging or targeting ligand.
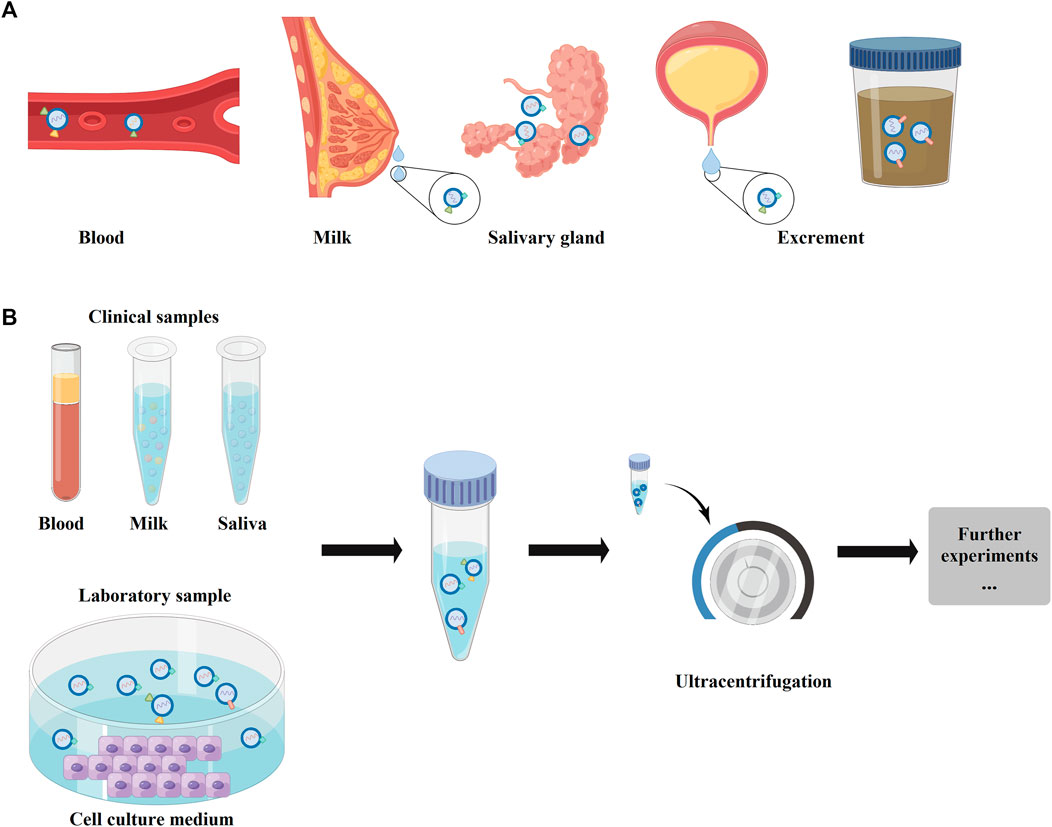
FIGURE 2. The physiological distributions and the main extraction method of exosomes. (A) The physiological distributions of exosomes, which could exist in blood, milk, salivary gland, and excrements (urine or feces). (B) The main extraction method of exosomes. For clinical samples, the exosomes could be collected from blood, milk, or saliva. In laboratory, the exosomes mainly were extracted from cell culture mendium.
The original cell types are diverse, so the representation of tissue-specific molecules of exosomes are polyphyletic and complicated. (Valadi et al., 2007) Therefore, some studies have proven that the biomarkers of exosomes could serve as the disease-specific markers, which would be applied for detection and diagnosis of some diseases, such as tumors, inflammatory, and autoimmune diseases. (Hoshino et al., 2015; Huang et al., 2020; Li et al., 2021b; Yu et al., 2021b; Kawada-Horitani et al., 2022) The host cell and the physiological microenvironment types both would regulate the content of their secreted exosomes. (Kalluri, 2016; Thery et al., 2018) For example, the cell types determine the exosomes as anti-inflammatory (e.g., MSCs and dendritic cells) or pro-inflammatory (e.g., tumor cells, macrophages, and intestinal epithelial cells), which would also control their effectiveness in regulating biological behaviors of various cellular phenotypes. (Arabpour et al., 2021; Hassanzadeh et al., 2021) Therefore, the interactions between cells and microenvironment would alter the final contents of the secreted exosomes, which is critical process in achieving homeostasis and regulating immune responses. (Mashouri et al., 2019; Wortzel et al., 2019) In normal physiological state, the quantity of secreted exosomes was limited. (Mashouri et al., 2019) Therefore, some researchers made some stimulus onto cells, then the stimuli-introduced exosomes would be secreted with a greater number and contain alteration of nucleic acids, proteins, and metabolites (Figure 3A). (Dai et al., 2020a) Moreover, some studies have reported that the stimuli-introduced exosomes possessed the increased therapeutic potential and the enhanced targeting to specific microenvironments. (Kalluri, 2016; Wang et al., 2019) The external stimulus applied in current studies includes biochemical factors (e.g., lipopolysaccharides, LPS; BMP-2; Kartogenin; IFNγ and TNFα) (Zhou et al., 2019; Jiao et al., 2020; Zhang et al., 2020; Wang et al., 2021b) and mechanical factors (e.g., centrifugal force and three dimensional (3D) culturing environment; shown as Figure 3A). Taken together, these promising strategies give us with abundant technologies to engineer progenitor cells for the high-efficiency extraction of exosomes.
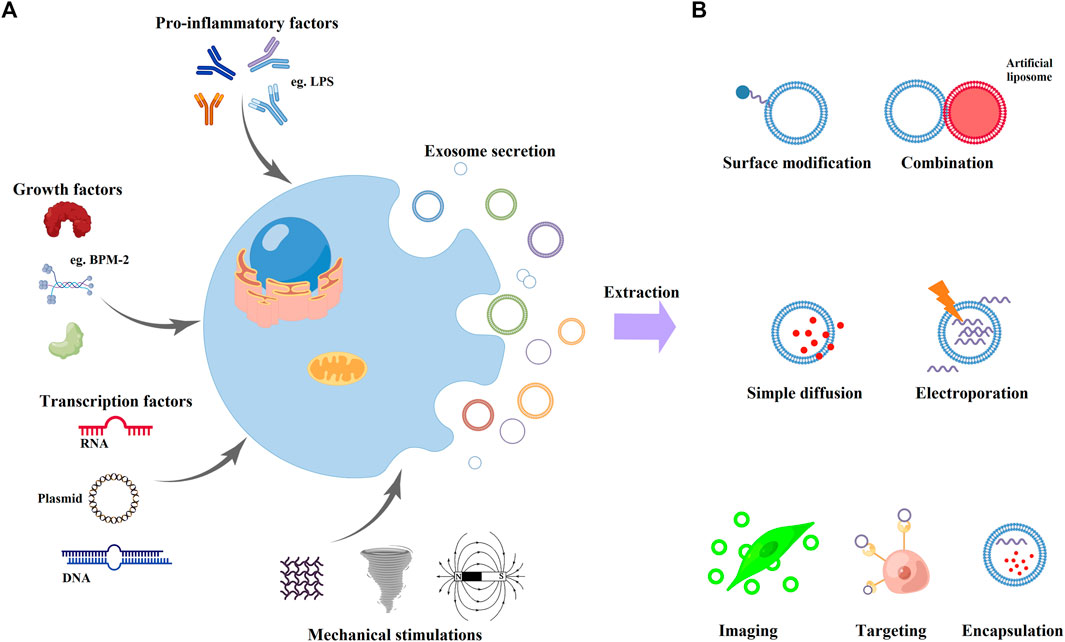
FIGURE 3. Engineering methods to improve exosome properties. (A) Engineering of progenitor cells with various physiological (pro-inflammatory factors, growth factors, and transcription factors) or mechanical stimulations (vibration, rotation, and magnetic field). (B) Direct functionalization approaches of exosomes. Researchers could modify the exosome membrane with direct surface modifications and fusion with artificial liposomes. Moreover, exosomes could load other materials by simple diffusion or electroporation. Finally, the functionalized exosomal-based nanomaterials could achieve specific purposes, such as imaging, targeting, and encapsulation.
2.2 Functionalization of exosomes
While some exosomes could be functionalized at the progenitor cells, some artificial modifications or various cargos were also applied to exosomes for specialized purposes. (Liang et al., 2021) The application potentials of native exosomes mainly include gene therapy, in-vivo imaging, and drug delivery. (Luan et al., 2017; Salunkhe et al., 2020) Therefore, for endowing theranostic properties, enhancing targeting, and improving drug encapsulation into exosomes, some molecules would be functionalized with exosomes artificially to gain the gene knockdown or imaging capabilities (Figures 1B, 3B). (Ocansey et al., 2020)
The membrane of exosomes could flexibly deform with proteins embedded in the phospholipid bilayer. (Luan et al., 2017) The membrane proteins have abundance of amine groups and alkyl groups, which provide the sites for various surface modifications of biological macromolecules. (Urano et al., 2016; Irfan et al., 2020) For example, to improve the affinity to recipient cells, aptamers, the oligonucleotides with high specificity and great targeting to MSCs, were used to functionalize with exosomes by covalent bonds. (Su et al., 2019; Salunkhe et al., 2020) And then, the Apt-exosomes could be largely internalized MSCs and accumulation in bone tissue, showing great potential for osteoporosis. (Su et al., 2019) Moreover, the amphiphilic property of phospholipid bilayer allows for partition fixation of cholesterol fibrils, and the cholesterol fibrils could be modified with oligonucleotides. (Dai et al., 2020b) The DNA or RNA aptamers grafted exosomes showed optimized pharmacokinetics, circulatory stability, and improved biological functions in bone diseases. (Luo et al., 2019) In addition to the surface modification strategies, the exosomes also possess the high-capacity loading ability of various materials, such as phospholipidic shell, microRNA-155, and curcumin (Figures 1B, 3B). (Liao et al., 2018; Tian et al., 2018; Pang et al., 2020; Varga et al., 2020) The encapsulation methods of exosomes mainly include freeze-thaw method, passive diffusion, and electroporation. (Liu et al., 2021; Xi et al., 2021; Shi et al., 2022) Taken together, exosomes are highly flexible nanocarriers with both exterior and interior editability structures for efficient delivery of small molecule drugs, siRNAs, miRNAs, and surface ligands to achieve targeted delivery and imaging with exceptional loading capacity.
3 Cell internalization mechanisms of exosomes
The cell internalization of various exosomes is the precondition for exosomes to exert their functions. (Mulcahy et al., 2014) Various exosome uptake mechanisms have been reported, such as protein interactions, clathrin-mediated endocytosis, phagocytosis, macropino-cytosis and plasma or endosomal membrane fusion. The protein interactions have proven that specific protein-protein interactions could mediate exosomes attachment and internalization into target cells, such as tetraspanins, (Hemler, 2001), integrins, (Wortzel et al., 2019), immunoglobulins, (Inoue and Tsukahara, 2021), proteoglycans, (Baietti et al., 2012), and lectins (Song et al., 2021). For endocytosis of exosomes, clathrin-mediated endocytosis, (Tian et al., 2014), caveolin-dependent endocytosis, (Tu et al., 2021), micropinocytosis, (Tu et al., 2021), and phagocytosis were widely investigated. (Patil et al., 2021) The essence exosome membrane is phospholipid bilayer, and the membrane fusion of exosomes and cells would happen, and some studies have suggested that the fusion could be enhanced under acidic microenvironment. (Colombo et al., 2014; Robbins and Morelli, 2014; Patil et al., 2021) Moreover, cell-specific exosome uptake was also detected, which mainly due to artificial modifications, such as DNA aptamer, (Ma et al., 2019; Ma et al., 2022), RGD peptide, (Tian et al., 2021), and small molecule drugs. (Wu et al., 2021) Understanding cell internalization mechanisms of exosomes is a vital object of the exosomal-based materials. However, limited number of studies could not reveal the total mechanisms of cell internalization processes for various exosomes, which requires further exploration.
4 Application of exosomes for bone and cartilage tissue engineering
Recently, some studies have demonstrated that exosomes could intervene bone reconstruction process in different bone diseases. The protein and microRNAs contained in exosomes could be used to treat osteoporosis, fractures, and other bone diseases. (Li et al., 2016; Ni et al., 2020; Nakao et al., 2021) The underlying mechanism of bone reconstruction underwent a paradigm shift from cell differentiation and replacement to secretory and paracrine signaling. In this part, we would summarize that application of exosomes for treating bone diseases.
4.1 Bone regeneration
Various bone diseases could lead to bone loss, such as osteoarthritis, osteoporosis, bone fracture, parodontopathy, and osteonecrosis (Figure 4D; Table 1). (Cosman et al., 2016) Osteal and chondral conditions might due to various sources other than disrupted homeostasis of the bone or cartilage tissues. (Jiang and Tuan, 2015) Therefore, bone tissue regeneration is a vital process for various bone diseases. (Dimitriou et al., 2011) The exosomes applied in bone regeneration were mainly derived from bMSCs, and some other stem cells were also included, such as adipose-derived stem cells (ADSCs) and embryonic stem cells (ESCs). (Li et al., 2018; Zhang et al., 2019a; Nakao et al., 2021) In exosomes, some growth factors or RNA have been founded, such as the receptor activator of NF-κ B ligand(RANKL) and microRNA-214. (Nakao et al., 2021; Wang et al., 2022a) For instance, the RANKL is one of the major regulators of osteoclastogenesis, which can be contained in exosomes and secreted by osteoblasts. (Nakao et al., 2021) The exosomes with RANKL could bind to RANK on osteoblasts, and then kindle the progress of osteoclastogenesis. (Nakao et al., 2021) On the contrary, the osteoblasts could secrete RANK-loaded exosomes, and the exosomes subsequently intercept the RANK signal pathway with inverse feedback by binding to RANKL on osteoclasts. Moreover, osteoclasts also could secrete exosomes enriched with miRNA-214, which could be internalized by osteoclasts through the recognition of ephrinA2/EphA2 and then inhibit the functions of osteoclasts. (Wang et al., 2022a)
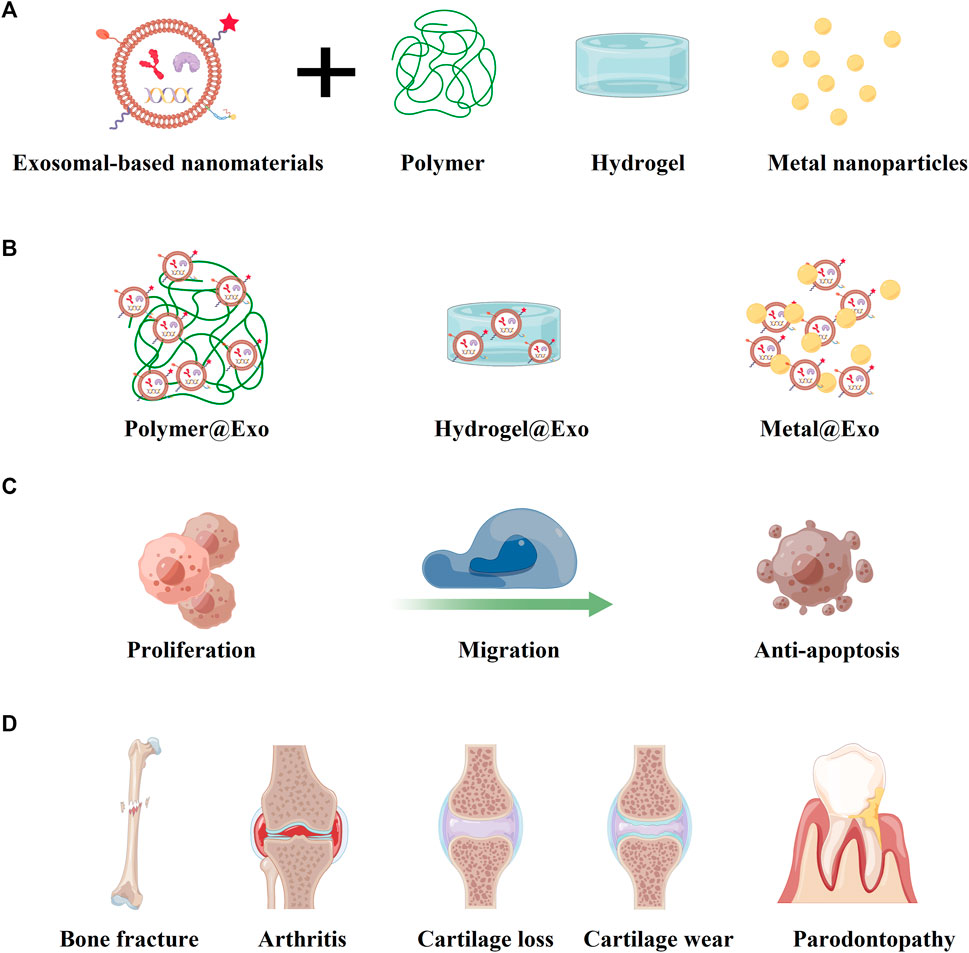
FIGURE 4. Multiple biological applications of exosomal-based nanomaterials by combining with other materials. (A) The exosomal-based nanomaterials could combine with polymer, hydrogel, and metal nanoparticles. (B) The formations of exosomal-based complexes of polymer@Exo, hydrogel@Exo, and metal@Exo. (C) Various exosomal-based complexes possess the improved capacities of proliferation, migration, and anti-apoptosis. (D) The bone and cartilage conditions in the limelight of exosomal-based research.
Exosomes with osteogenic functions have presented great potential in therapies of bone defect. For example, osteogenic exosomes could promote the healing of bone fractures in CD9−/− mouse model. (Furuta et al., 2016) The underlying mechanism of the healing progress includes the recruitment of progenitor cells or stem cells, and more important process is that the miRNAs coated in exosomes could facilitate angiogenesis and osteogenesis. (Furuta et al., 2016) Osteogenic exosomes can accelerate progenitor cells or stem cells to osteogenic differentiation and matrix mineralization, and the regenerated new bone showed a more robust calcium deposition and calcium phosphate nucleation. (Lei et al., 2022) Moreover, the exosomes show excellent delivery properties. (Vader et al., 2016) For example, exosomes could integrate with titanium nanotubes to form a new type nanomaterial, which could effectively promote bone regeneration. Wei et al. (2019) used BPM-2 to pretreat macrophages, and then the macrophage-derived exosomes were collected and applied to cate titanium nanotube implants (Exo@Ti). (Wei et al., 2019) The Exo@Ti could significantly increase the expression of alkaline phosphatase and BPM-2, which were the osteoblastic differentiation markers in the early stage of osteogenesis. (Wei et al., 2019) Wang et al. found that the macrophage-derived exosomes carrying titania nanotube arrays (Exo@TNAs) could simultaneously promote the osteogenesis of MSCs and angiogenesis of endothelial cells (Figure 4B). (Wang et al., 2022b) Furthermore, some researchers have found that exosomes could regulate macrophages and then promote bone regeneration. (Guo et al., 2021b) Li et. at had reported that ASC-derived exosomes (ASC-Exos) loaded with gelatine nanoparticles (GNPs) by inverse charge attraction, which could regulate M1/M2 macrophage polarization. (Li et al., 2022) The ASC-Exos containing abundant miRNA-451a, miRNA-21, and miRNA-148a could inhibited the expression of M1 macrophage markers and upregulated the expression of M2 macrophage markers, which could regulate the immune metabolism of bone tissue and further enhance bone healing. (Li et al., 2022)
For further enhancing the bone regeneration, some polymer materials were applied with exosomes (Figures 4A, B). Li et al. combined the polydopamine-coating poly(lactic-co-glycolic acid) (PLGA/pDA) scaffolds with exosomes derived from human adipose-derived stem cells (hASCs). (Li et al., 2018) The hASC-derived exosomes could be immobilized and released by the PLGA/pDA scaffold under different environmental conditions. (Li et al., 2018) The slow and consistent release of hASC-derived exosomes could enhance bone regeneration significantly, at least partially through its osteogenic induction effects and capacities of promoting MSCs migration and homing in the newly formed bone tissue. (Li et al., 2018) Mi et. at constructed a natural polymer hyaluronic-acid-based hydrogel (HA hydrogel), engineered endothelial cell-derived exosomes (EC-ExomiR-26a-5p), and APY29, an IRE-1α inhibitor, which could specifically deliver EC-ExomiR-26a-5p to osteoblast/osteoclast and promote bone fracture repair (Figure 4). (Mi et al., 2022) Moreover, Li et. at had reported the ASC-Exos loaded with GNPs, and the GNP-Exos exhibited good biocompatibility and strong mechanical adaptability. (Li et al., 2022) The combination of exosomes and biological materials shows great potential in enhancing the mechanical propertoes and achieving controlled release of exosomes, which might provide a promising therapeutic direction for expending the biological applications various bone diseases (Table 1). (Shin et al., 2016)
4.2 Cartilage regeneration
In some bone diseases, defects of bone tissue might also be accompanied by the loss of cartilage. (Wu et al., 2022) Therefore, the cartilage regeneration is also significant for the bone tissue engineering. Cartilage, a connective tissue without vascular tissue, has abundant collagen fibers, proteoglycans, hyaluronic acid, and chondrocytes components. (Krishnan and Grodzinsky, 2018) The regeneration of injured cartilage is difficult for the avascular structure, which would result in limited supply of oxygen, nutrients, and infiltration and the delivery of available signal molecules to precursor cells. (Bhattacharjee et al., 2015)
Among various diseases, osteoarthritis is the most representative disease, which could cause physiological changes in the composition and structure of cartilage tissue. (Scanzello and Goldring, 2012) Osteoarthritis can occur in various joints, such as knee-joint and temporomandibular joint (TMJ), and some researchers have conducted many studies to explore the therapeutic potential of exosomes for cartilage regeneration (Table 2). (Scanzello and Goldring, 2012; Zhang et al., 2019b; Jansen and Mastbergen, 2022) For example, Tao et al. demonstrated that exosomes derived from miRNA-140-5p-overexpressing synovial mesenchymal stem cells (SMSC-140-Exos) could enhance cartilage tissue regeneration by activating Yes-associated protein (YAP) with the Wnt signaling pathway. (Tao et al., 2017) Zhang et al. reported that the MSC-derived exosomes could promote cartilage repair by increasing proliferation, suppressing apoptosis, and modulating immune reactivity (Figure 4C). (Zhang et al., 2018) They also found that the MSC-derived exosomes possessed the ability of attenuating inflammation and restoring matrix homeostasis, which could relieve TMJ osteoarthritis. (Zhang et al., 2019b)
Beyond the alone application of exosomes in cartilage tissue regeneration, the exosomes are also combined with other materials to enhance their biological functions. Zhang et al. synthesized an injectable mussel-inspired highly adhesive hydrogel enriched with exosomes, in which the hydrogel with high bonding strength to the wet surface was prepared using a crosslinked network of alginate-dopamine, chondroitin sulfate, and regenerated silk fibroin (AD/CS/RSF). The AD/CS/RSF/EXO hydrogel could promote the cell migration, proliferation, and differentiation of bMSCs, which could help the repair of cartilage defects. (Zhang et al., 2021) Hu et al. reported a Gelatin methacrylate (Gelma)/nanoclay hydrogel (Gel-nano) loaded with human umbilical cord MSCs derived exosomes (hUC-MSCs-Exos), and the hUC-MSCs-Exos contained abundant miRNA-23a-3p. The miRNA-23a-3p could promote cartilage regeneration via activating the classical PTEN/AKT signal pathway. Gel-nanoclay@Exos hydrogel showed great potential for the regeneration of cartilage defects. (Hu et al., 2020) Furthermore, exosomes also can be employed as the carriers to deliver genes, proteins, or drugs to cells. (Duan et al., 2021) WNT3a can run to the benefit of cartilage regeneration by activating the WNT-β-catenin pathway. (Bertrand et al., 2020) Bethan et al. demonstrated that the WNT3a loaded exosomes activated canonical WNT signaling and improved the repair of osteochondral defects. (Thomas et al., 2021) In addition to the exosomes derived from various MSCs, some exosomes secreted by platelets (pExos) also presented promising therapeutic effects on subtalar osteoarthritis. Zhang et al. incorporated the pExos into thermosensitive hydrogel for prolonging the retention time of pExos in the joint, thereby the pExos could cloud continuously be released from the thermosensitive hydrogel and promote the cell proliferation and migration of bMSCs and chondrocytes. Moreover, the long-time controlled-release of pExos also could facilitate the chondrogenic differentiation of bMSCs and suppress inflammation-induced chondrocyte degeneration. (Zhang et al., 2022)
5 Advantages and challenges of exosomes in bone/cartilage tissue engineering
In the field of bone and cartilage regenerative medicine, the “cell-free regeneration strategy” in which exosomes are used as carriers of a variety of bioactive molecules has many advantages, (Chew et al., 2019), including that: 1) almost no cytotoxicity and low immunogenicity, and the possibility of immune rejection after allogeneic administration is low, and then the exosomes have a wide range of application; (Mai et al., 2021) 2) the exosomes can avoid the potential risks of embolism and infection spread caused by direct cell transplantation, which indicates the exosomes possessing excellent biological safety; (Mao et al., 2021) 3) the exosomes can be prepared into convenient storage and clinical application of biological agents, stored at −20 °C for 6 months without loss of biological activity. (Sohrabi et al., 2022) However, the mechanisms of communication between cells by exosomes carrying various bioactive molecules are still unclear, which cannot be accurately regulated and has certain risks. (Wang et al., 2019; Wortzel et al., 2019; Dai et al., 2020a; Kalluri and LeBleu, 2020)
It has been found that mesenchymal stem cell-derived exosomes can promote the occurrence and development of tumors by delivering related miRNAs to neighboring cells or activating signaling pathways. Cancer cells can use exosomes to release signals to normal cells in the local microenvironment to promote their carcinogenesis. (Dai et al., 2020a; Jiang et al., 2021) Exosomes released by distant metastatic cancer cells have a stronger ability to induce cell migration. (Sung et al., 2021) In addition, the biological distribution of exosomes in vivo is one of the important determinants of their toxicity. (Zhou et al., 2021) When exosomes are used for regenerative therapy, the delivery path, dose, biological distribution and metabolic dynamics of exosomes in vivo should be clearly defined in order to guide exosomes to reach the tissue defect area to play their functions and ensure the safety of their clinical application. (Zhou et al., 2021; Kimiz-Gebologlu and Oncel, 2022) Combining exosomes with various forms of tissue engineering scaffolds can not only effectively load exosomes, but also effectively retain exosomes in the tissue defect site for a long time. The combined exosomal-based materials could meet the need for efficient retention and continuous release of exosomes, improve the stability of proteins and nucleic acids and other contents, and provide an ideal microenvironment for tissue regeneration to effectively play its repair role. To further improve the therapeutic effects, exosomal-based materials would provide a new strategy for the repair of tissue injury in the field of tissue engineering.
6 Conclusion and future perspectives
Recently, various cell-derived exosomes have presented great capacities in bone and cartilage tissue engineering for the therapeutic strategies of osteoarticular diseases. (Lara-Barba et al., 2021) Exosomes derived from different cells might contain disparate biological information for the diversities of proteins, microRNA, and biomarkers. (Yu et al., 2021b) Compared with the stem-cell or liposome based therapies, the exosomal-based nanomaterials show safer and more efficient therapeutic efficacy. (Mori et al., 2019; Zhu et al., 2019; Yu et al., 2021b; Liu et al., 2022a) The nanostructures, low immunogenicity, capacities of infiltrate-multiple biological barriers (such as blood brain barrier and blood bone barrier), and properties to carry various therapeutic drugs make it considered as the great potential direction for bone tissue engineering. (Xu et al., 2016; Mori et al., 2019) Moreover, various modification loaded to the natural exosomes might promote the capacity of exosome to permeate multiple biological barriers and enhance the targeting of certain tissues, which would be the frontier hotspots of exosomal-based nanomedicines for bone tissue engineering. (Xu et al., 2016; Song et al., 2019)
In this review, we have summarized the biological applications of exosomes and exosome-derived materials on bone and cartilage tissue engineering, where more studies are needed to make the biological applications of exosomes moving forward. Further investigations of exosomes would be focusing on the improvements of production and purification techniques of exosomes, establishments of the standardized guidelines, protocols of drug delivery methods, and enhancements of specific tissue targeting capabilities. (Barile and Vassalli, 2017; Wei et al., 2021; Liu et al., 2022b; Lai et al., 2022) Moreover, the origin sources of exosomes also highly draw great concerns. The abundant sources of exosomes, various combination with artificial liposomes, and multiple modification strategies make the exosomal-based nanomaterials with great biological application prospects. (Lai et al., 2022) Furthermore, we expect that more exosomal-based nanomedicines can be developed and used for clinical diseases.
Author contributions
All authors listed have made a substantial, direct, and intellectual contribution to the work and approved it for publication.
Funding
This manuscript was funded by Medical Health Science and Technology Project of Zhejiang Province (Grant Nos.2021KY068 and 2023KY028), and supported by the Hainan Provincial Natural Science Foundation High-level Talents Project of China (Grant No. 821RC725). All figures in this review werecreated by Figdraw.
Conflict of interest
The authors declare that the research was conducted in the absence of any commercial or financial relationships that could be construed as a potential conflict of interest.
Publisher’s note
All claims expressed in this article are solely those of the authors and do not necessarily represent those of their affiliated organizations, or those of the publisher, the editors and the reviewers. Any product that may be evaluated in this article, or claim that may be made by its manufacturer, is not guaranteed or endorsed by the publisher.
References
Alvarez-Erviti, L., Seow, Y., Yin, H., Betts, C., Lakhal, S., and Wood, M. J. (2011). Delivery of siRNA to the mouse brain by systemic injection of targeted exosomes. Nat. Biotechnol. 29, 341–345. doi:10.1038/nbt.1807
An, Y., Lin, S., Tan, X., Zhu, S., Nie, F., Zhen, Y., et al. (2021). Exosomes from adipose-derived stem cells and application to skin wound healing. Cell Prolif. 54, e12993. doi:10.1111/cpr.12993
Arabpour, M., Saghazadeh, A., and Rezaei, N. (2021). Anti-inflammatory and M2 macrophage polarization-promoting effect of mesenchymal stem cell-derived exosomes. Int. Immunopharmacol. 97, 107823. doi:10.1016/j.intimp.2021.107823
Ayyar, K. K., and Moss, A. C. (2021). Exosomes in intestinal inflammation. Front. Pharmacol. 12, 658505. doi:10.3389/fphar.2021.658505
Baietti, M. F., Zhang, Z., Mortier, E., Melchior, A., Degeest, G., Geeraerts, A., et al. (2012). Syndecan-syntenin-ALIX regulates the biogenesis of exosomes. Nat. Cell Biol. 14, 677–685. doi:10.1038/ncb2502
Barile, L., and Vassalli, G. (2017). Exosomes: Therapy delivery tools and biomarkers of diseases. Pharmacol. Ther. 174, 63–78. doi:10.1016/j.pharmthera.2017.02.020
Batrakova, E. V., and Kim, M. S. (2015). Using exosomes, naturally-equipped nanocarriers, for drug delivery. J. Control Release 219, 396–405. doi:10.1016/j.jconrel.2015.07.030
Behrend, C., Carmouche, J., Millhouse, P. W., Ritter, L., Moskal, J., Rubery, P., et al. (2016). Allogeneic and autogenous bone grafts are affected by historical donor environmental exposure. Clin. Orthop. Relat. Res. 474, 1405–1409. doi:10.1007/s11999-015-4572-7
Bei, H. P., Hung, P. M., Yeung, H. L., Wang, S., and Zhao, X. (2021). Bone-a-Petite: Engineering exosomes towards bone, osteochondral, and cartilage repair. Small 17, 2101741. doi:10.1002/smll.202101741
Benavides, F. P., Pinto, G. B. A., Heckler, M. C. T., Hurtado, D. M. R., Teixeira, L. R., Monobe, M. M. S., et al. (2021). Intrathecal transplantation of autologous and allogeneic bone marrow-derived mesenchymal stem cells in dogs. Cell Transpl. 30, 096368972110344. doi:10.1177/09636897211034464
Bertrand, J., Kraft, T., Gronau, T., Sherwood, J., Rutsch, F., Liote, F., et al. (2020). BCP crystals promote chondrocyte hypertrophic differentiation in OA cartilage by sequestering Wnt3a. Ann. Rheum. Dis. 79, 975–984. doi:10.1136/annrheumdis-2019-216648
Bhattacharjee, M., Coburn, J., Centola, M., Murab, S., Barbero, A., Kaplan, D. L., et al. (2015). Tissue engineering strategies to study cartilage development, degeneration and regeneration. Adv. Drug Deliv. Rev. 84, 107–122. doi:10.1016/j.addr.2014.08.010
Bohner, M., Santoni, B. L. G., and Dobelin, N. (2020). β-tricalcium phosphate for bone substitution: Synthesis and properties. Acta Biomater. 113, 23–41. doi:10.1016/j.actbio.2020.06.022
Chang, W., Li, M., Song, L., Miao, S., Yu, W., and Wang, J. (2022). Noncoding RNAs from tissue-derived small extracellular vesicles: Roles in diabetes and diabetic complications. Mol. Metab. 58, 101453. doi:10.1016/j.molmet.2022.101453
Chaudhuri, A., Polyakova, J., Zbrzezna, V., Williams, K., Gulati, S., and Pogo, A. O. (1993). Cloning of glycoprotein D cDNA, which encodes the major subunit of the Duffy blood group system and the receptor for the Plasmodium vivax malaria parasite. Proc. Natl. Acad. Sci. U. S. A. 90, 10793–10797. doi:10.1073/pnas.90.22.10793
Chew, J. R. J., Chuah, S. J., Teo, K. Y. W., Zhang, S., Lai, R. C., Fu, J. H., et al. (2019). Mesenchymal stem cell exosomes enhance periodontal ligament cell functions and promote periodontal regeneration. Acta Biomater. 89, 252–264. doi:10.1016/j.actbio.2019.03.021
Colombo, M., Raposo, G., and Théry, C. (2014). Biogenesis, secretion, and intercellular interactions of exosomes and other extracellular vesicles. Annu. Rev. Cell Dev. Biol. 30, 255–289. doi:10.1146/annurev-cellbio-101512-122326
Cosman, F., Crittenden, D. B., Adachi, J. D., Binkley, N., Czerwinski, E., Ferrari, S., et al. (2016). Romosozumab treatment in postmenopausal women with osteoporosis. N. Engl. J. Med. 375, 1532–1543. doi:10.1056/nejmoa1607948
Cosman, F. (2009). Treatment of osteoporosis and prevention of new fractures: Role of intravenously administered bisphosphonates. Endocr. Pract. 15, 483–493. doi:10.4158/ep08306.orr1
Cox, Z. C., Green, C. C., Otero, J. E., Mason, J. B., and Martin, J. R. (2022). Varus collapse in total knee arthroplasty: Does fixation or bone fail first? J. Arthroplasty 37, 162–167. doi:10.1016/j.arth.2021.09.012
Dai, J., Su, Y., Zhong, S., Cong, L., Liu, B., Yang, J., et al. (2020). Exosomes: Key players in cancer and potential therapeutic strategy. Signal Transduct. Target Ther. 5, 145. doi:10.1038/s41392-020-00261-0
Dai, Y., Zhang, M., Shi, X., Wang, K., Gao, G., Shen, L., et al. (2020). Kinetic study of Aβ(1-42) amyloidosis in the presence of ganglioside-containing vesicles. Colloids Surf. B Biointerfaces 185, 110615. doi:10.1016/j.colsurfb.2019.110615
Dimitriou, R., Jones, E., McGonagle, D., and Giannoudis, P. V. (2011). Bone regeneration: Current concepts and future directions. BMC Med. 9, 66. doi:10.1186/1741-7015-9-66
Diwu, W., Dong, X., Nasif, O., Alharbi, S. A., Zhao, J., and Li, W. (2020). In-vivo investigations of hydroxyapatite/Co-polymeric composites coated titanium plate for bone regeneration. Front. Cell Dev. Biol. 8, 631107. doi:10.3389/fcell.2020.631107
Duan, L., Xu, X., Xu, L., Chen, H., Li, X., Alahdal, M., et al. (2021). Exosome-mediated drug delivery for cell-free therapy of osteoarthritis. Curr. Med. Chem. 28, 6458–6483. doi:10.2174/1875533xmtexenjqg4
Furuta, T., Miyaki, S., Ishitobi, H., Ogura, T., Kato, Y., Kamei, N., et al. (2016). Mesenchymal stem cell-derived exosomes promote fracture healing in a mouse model. Stem Cells Transl. Med. 5, 1620–1630. doi:10.5966/sctm.2015-0285
Gao, Y., Xin, H., Cai, B., Wang, L., Lv, Q., Hou, Y., et al. (2022). RNA interference-based osteoanabolic therapy for osteoporosis by a bone-formation surface targeting delivery system. Biochem. Biophys. Res. Commun. 601, 86–92. doi:10.1016/j.bbrc.2022.02.080
Garrison, K. R., Donell, S., Ryder, J., Shemilt, I., Mugford, M., Harvey, I., et al. (2007). Clinical effectiveness and cost-effectiveness of bone morphogenetic proteins in the non-healing of fractures and spinal fusion: A systematic review. Health Technol. Assess. 11, 1–150, iii-iv. doi:10.3310/hta11300
Gillman, C. E., and Jayasuriya, A. C. (2021). FDA-approved bone grafts and bone graft substitute devices in bone regeneration. Mater Sci. Eng. C Mater Biol. Appl. 130, 112466. doi:10.1016/j.msec.2021.112466
Guo, H., Li, B., Wu, M., Zhao, W., He, X., Sui, B., et al. (2021). Odontogenesis-related developmental microenvironment facilitates deciduous dental pulp stem cell aggregates to revitalize an avulsed tooth. Biomaterials 279, 121223. doi:10.1016/j.biomaterials.2021.121223
Guo, S., Gu, J., Ma, J., Xu, R., Wu, Q., Meng, L., et al. (2021). GATA4-driven miR-206-3p signatures control orofacial bone development by regulating osteogenic and osteoclastic activity. Theranostics 11, 8379–8395. doi:10.7150/thno.58052
Han, J., Ma, B., Liu, H., Wang, T., Wang, F., Xie, C., et al. (2018). Hydroxyapatite nanowires modified polylactic acid membrane plays barrier/osteoinduction dual roles and promotes bone regeneration in a rat mandible defect model. J. Biomed. Mater Res. A 106, 3099–3110. doi:10.1002/jbm.a.36502
Hao, M., Wang, Y., Li, L., Liu, Y., Bai, Y., Zhou, W., et al. (2022). Tough engineering hydrogels based on swelling-freeze-thaw method for artificial cartilage. ACS Appl. Mater Interfaces 14, 25093–25103. doi:10.1021/acsami.2c02990
Hassanzadeh, A., Rahman, H. S., Markov, A., Endjun, J. J., Zekiy, A. O., Chartrand, M. S., et al. (2021). Mesenchymal stem/stromal cell-derived exosomes in regenerative medicine and cancer; overview of development, challenges, and opportunities. Stem Cell Res. Ther. 12, 297. doi:10.1186/s13287-021-02378-7
Hayashi, M., Nakashima, T., Yoshimura, N., Okamoto, K., Tanaka, S., and Takayanagi, H. (2019). Autoregulation of osteocyte Sema3A orchestrates estrogen action and counteracts bone aging. Cell Metab. 29, 627–637.e5. doi:10.1016/j.cmet.2018.12.021
Hemler, M. E. (2001). Specific tetraspanin functions. J. Cell Biol. 155, 1103–1108. doi:10.1083/jcb.200108061
Heo, J., and Kang, H. (2022). Exosome-based treatment for atherosclerosis. Int. J. Mol. Sci. 23, 1002. doi:10.3390/ijms23021002
Hoshino, A., Costa-Silva, B., Shen, T. L., Rodrigues, G., Hashimoto, A., Tesic Mark, M., et al. (2015). Tumour exosome integrins determine organotropic metastasis. Nature 527, 329–335. doi:10.1038/nature15756
Hu, H., Dong, L., Bu, Z., Shen, Y., Luo, J., Zhang, H., et al. (2020). miR-23a-3p-abundant small extracellular vesicles released from Gelma/nanoclay hydrogel for cartilage regeneration. J. Extracell. Vesicles 9, 1778883. doi:10.1080/20013078.2020.1778883,
Huang, R. L., Yuan, Y., Tu, J., Zou, G. M., and Li, Q. (2014). Exaggerated inflammatory environment decreases BMP-2/ACS-induced ectopic bone mass in a rat model: Implications for clinical use of BMP-2. Osteoarthr. Cartil. 22, 1186–1196. doi:10.1016/j.joca.2014.06.017
Huang, Y., Li, R., Ye, S., Lin, S., Yin, G., and Xie, Q. (2020). Recent advances in the use of exosomes in sjogren's syndrome. Front. Immunol. 11, 1509. doi:10.3389/fimmu.2020.01509
Inoue, R., and Tsukahara, T. (2021). Composition and physiological functions of the porcine colostrum. Animal Sci. J. = Nihon chikusan Gakkaiho 92, e13618. doi:10.1111/asj.13618
Irfan, R., Mousavi, S., Alazmi, M., and Saleem, R. S. Z. (2020). A comprehensive review of aminochalcones. Molecules 25, 5381. doi:10.3390/molecules25225381
Jansen, M. P., and Mastbergen, S. C. (2022). Joint distraction for osteoarthritis: Clinical evidence and molecular mechanisms. Nat. Rev. Rheumatol. 18, 35–46. doi:10.1038/s41584-021-00695-y
Jiang, C., Zhang, N., Hu, X., and Wang, H. (2021). Tumor-associated exosomes promote lung cancer metastasis through multiple mechanisms. Mol. Cancer 20, 117. doi:10.1186/s12943-021-01411-w
Jiang, Y., and Tuan, R. S. (2015). Origin and function of cartilage stem/progenitor cells in osteoarthritis. Nat. Rev. Rheumatol. 11, 206–212. doi:10.1038/nrrheum.2014.200
Jiao, Y., Li, W., Wang, W., Tong, X., Xia, R., Fan, J., et al. (2020). Platelet-derived exosomes promote neutrophil extracellular trap formation during septic shock. Crit. Care 24, 380. doi:10.1186/s13054-020-03082-3
Kalluri, R., and LeBleu, V. S. (2020). The biology, function, and biomedical applications of exosomes. Science 367, eaau6977. doi:10.1126/science.aau6977
Kalluri, R. (2016). The biology and function of exosomes in cancer. J. Clin. Invest. 126, 1208–1215. doi:10.1172/jci81135
Kamerkar, S., LeBleu, V. S., Sugimoto, H., Yang, S., Ruivo, C. F., Melo, S. A., et al. (2017). Exosomes facilitate therapeutic targeting of oncogenic KRAS in pancreatic cancer. Nature 546, 498–503. doi:10.1038/nature22341
Kawada-Horitani, E., Kita, S., Okita, T., Nakamura, Y., Nishida, H., Honma, Y., et al. (2022). Human adipose-derived mesenchymal stem cells prevent type 1 diabetes induced by immune checkpoint blockade. Diabetologia 65, 1185–1197. doi:10.1007/s00125-022-05708-3
Kimiz-Gebologlu, I., and Oncel, S. S. (2022). Exosomes: Large-scale production, isolation, drug loading efficiency, and biodistribution and uptake. J. Control Release 347, 533–543. doi:10.1016/j.jconrel.2022.05.027
Kitaura, H., Marahleh, A., Ohori, F., Noguchi, T., Nara, Y., Pramusita, A., et al. (2022). Role of the interaction of tumor necrosis factor-alpha and tumor necrosis factor receptors 1 and 2 in bone-related cells. Int. J. Mol. Sci. 23, 1481. doi:10.3390/ijms23031481
Lai, H. C., James, A., Luff, J., De Souza, P., Quek, H., Ho, U., et al. (2021). Regulation of RNA degradation pathways during the lipopolysaccharide response in Macrophages. J. Leukoc. Biol. 109, 593–603. doi:10.1002/jlb.2ab0420-151rr
Lai, J. J., Chau, Z. L., Chen, S. Y., Hill, J. J., Korpany, K. V., Liang, N. W., et al. (2022). Exosome processing and characterization approaches for research and technology development. Adv. Sci. (Weinh) 9, 2103222. doi:10.1002/advs.202103222
Lara-Barba, E., Araya, M. J., Hill, C. N., Bustamante-Barrientos, F. A., Ortloff, A., Garcia, C., et al. (2021). Role of microRNA shuttled in small extracellular vesicles derived from mesenchymal stem/stromal cells for osteoarticular disease treatment. Front. Immunol. 12, 768771. doi:10.3389/fimmu.2021.768771
Lei, F., Li, M., Lin, T., Zhou, H., Wang, F., and Su, X. (2022). Treatment of inflammatory bone loss in periodontitis by stem cell-derived exosomes. Acta Biomater. 141, 333–343. doi:10.1016/j.actbio.2021.12.035
Li, C., Ni, Y. Q., Xu, H., Xiang, Q. Y., Zhao, Y., Zhan, J. K., et al. (2021). Roles and mechanisms of exosomal non-coding RNAs in human health and diseases. Signal Transduct. Target Ther. 6, 383. doi:10.1038/s41392-021-00779-x
Li, D., Liu, J., Guo, B., Liang, C., Dang, L., Lu, C., et al. (2016). Osteoclast-derived exosomal miR-214-3p inhibits osteoblastic bone formation. Nat. Commun. 7, 10872. doi:10.1038/ncomms10872
Li, Q., Yu, H., Sun, M., Yang, P., Hu, X., Ao, Y., et al. (2021). The tissue origin effect of extracellular vesicles on cartilage and bone regeneration. Acta Biomater. 125, 253–266. doi:10.1016/j.actbio.2021.02.039
Li, R., Li, D., Wang, H., Chen, K., Wang, S., Xu, J., et al. (2022). Exosomes from adipose-derived stem cells regulate M1/M2 macrophage phenotypic polarization to promote bone healing via miR-451a/MIF. Stem Cell Res. Ther. 13, 149. doi:10.1186/s13287-022-02823-1
Li, W., Liu, Y., Zhang, P., Tang, Y., Zhou, M., Jiang, W., et al. (2018). Tissue-engineered bone immobilized with human adipose stem cells-derived exosomes promotes bone regeneration. ACS Appl. Mater Interfaces 10, 5240–5254. doi:10.1021/acsami.7b17620
Liang, Y., Duan, L., Lu, J., and Xia, J. (2021). Engineering exosomes for targeted drug delivery. Theranostics 11, 3183–3195. doi:10.7150/thno.52570
Liao, T. L., Hsieh, S. L., Chen, Y. M., Chen, H. H., Liu, H. J., Lee, H. C., et al. (2018). Rituximab may cause increased hepatitis C virus viremia in rheumatoid arthritis patients through declining exosomal MicroRNA-155. Arthritis Rheumatol. 70, 1209–1219. doi:10.1002/art.40495
Ling, Z., Yang, C., Tan, J., Dou, C., and Chen, Y. (2021). Beyond immunosuppressive effects: Dual roles of myeloid-derived suppressor cells in bone-related diseases. Cell Mol. Life Sci. 78, 7161–7183.
Liu, A., Wang, Q., Zhao, Z., Wu, R., Wang, M., Li, J., et al. (2021). Nitric oxide nanomotor driving exosomes-loaded microneedles for achilles tendinopathy healing. ACS Nano 15, 13339–13350. doi:10.1021/acsnano.1c03177
Liu, H., Zhang, H., Han, Y., Hu, Y., Geng, Z., and Su, J. (2022). Bacterial extracellular vesicles-based therapeutic strategies for bone and soft tissue tumors therapy. Theranostics 12, 6576–6594. doi:10.7150/thno.78034
Liu, H., Zhang, Q., Wang, S., Weng, W., Jing, Y., and Su, J. (2022). Bacterial extracellular vesicles as bioactive nanocarriers for drug delivery: Advances and perspectives. Bioact. Mater. 14, 169–181. doi:10.1016/j.bioactmat.2021.12.006
Liu, M., Sun, Y., and Zhang, Q. (2018). Emerging role of extracellular vesicles in bone remodeling. J. Dent. Res. 97, 859–868. doi:10.1177/0022034518764411
Luan, X., Sansanaphongpricha, K., Myers, I., Chen, H., Yuan, H., and Sun, D. (2017). Engineering exosomes as refined biological nanoplatforms for drug delivery. Acta Pharmacol. Sin. 38, 754–763. doi:10.1038/aps.2017.12
Luo, Z. W., Li, F. X., Liu, Y. W., Rao, S. S., Yin, H., Huang, J., et al. (2019). Aptamer-functionalized exosomes from bone marrow stromal cells target bone to promote bone regeneration. Nanoscale 11, 20884–20892. doi:10.1039/c9nr02791b
Ma, W., Yang, Y., Zhu, J., Jia, W., Zhang, T., Liu, Z., et al. (2022). Biomimetic nanoerythrosome-coated aptamer-DNA tetrahedron/maytansine conjugates: pH-responsive and targeted cytotoxicity for HER2-positive breast cancer. Adv. Mater 34, 2109609. doi:10.1002/adma.202109609
Ma, W., Zhan, Y., Zhang, Y., Shao, X., Xie, X., Mao, C., et al. (2019). An intelligent DNA nanorobot with in vitro enhanced protein lysosomal degradation of HER2. Nano Lett. 19, 4505–4517. doi:10.1021/acs.nanolett.9b01320
Mai, Z., Chen, H., Ye, Y., Hu, Z., Sun, W., Cui, L., et al. (2021). Translational and clinical applications of dental stem cell-derived exosomes. Front. Genet. 12, 750990. doi:10.3389/fgene.2021.750990
Mao, W., Wang, K., Wu, Z., Xu, B., and Chen, M. (2021). Current status of research on exosomes in general, and for the diagnosis and treatment of kidney cancer in particular. J. Exp. Clin. Cancer Res. 40, 305. doi:10.1186/s13046-021-02114-2
Mashouri, L., Yousefi, H., Aref, A. R., Ahadi, A. M., Molaei, F., and Alahari, S. K. (2019). Exosomes: Composition, biogenesis, and mechanisms in cancer metastasis and drug resistance. Mol. Cancer 18, 75. doi:10.1186/s12943-019-0991-5
Mi, B., Chen, L., Xiong, Y., Yang, Y., Panayi, A. C., Xue, H., et al. (2022). Osteoblast/osteoclast and immune cocktail therapy of an exosome/drug delivery multifunctional hydrogel accelerates fracture repair. ACS Nano 16, 771–782. doi:10.1021/acsnano.1c08284
Mori, M. A., Ludwig, R. G., Garcia-Martin, R., Brandao, B. B., and Kahn, C. R. (2019). Extracellular miRNAs: From biomarkers to mediators of physiology and disease. Cell Metab. 30, 656–673. doi:10.1016/j.cmet.2019.07.011
Mulcahy, L. A., Pink, R. C., and Carter, D. R. (2014). Routes and mechanisms of extracellular vesicle uptake. J. Extracell. Vesicles 3, 24641. doi:10.3402/jev.v3.24641
Nakao, Y., Fukuda, T., Zhang, Q., Sanui, T., Shinjo, T., Kou, X., et al. (2021). Exosomes from TNF-alpha-treated human gingiva-derived MSCs enhance M2 macrophage polarization and inhibit periodontal bone loss. Acta Biomater. 122, 306–324. doi:10.1016/j.actbio.2020.12.046
Ni, Z., Zhou, S., Li, S., Kuang, L., Chen, H., Luo, X., et al. (2020). Exosomes: Roles and therapeutic potential in osteoarthritis. Bone Res. 8, 25. doi:10.1038/s41413-020-0100-9
Ocansey, D. K. W., Zhang, L., Wang, Y., Yan, Y., Qian, H., Zhang, X., et al. (2020). Exosome-mediated effects and applications in inflammatory bowel disease. Biol. Rev. Camb Philos. Soc. 95, 1287–1307. doi:10.1111/brv.12608
Palmer, L. C., Newcomb, C. J., Kaltz, S. R., Spoerke, E. D., and Stupp, S. I. (2008). Biomimetic systems for hydroxyapatite mineralization inspired by bone and enamel. Chem. Rev. 108, 4754–4783. doi:10.1021/cr8004422
Pang, Y., Shi, J., Yang, X., Wang, C., Sun, Z., and Xiao, R. (2020). Personalized detection of circling exosomal PD-L1 based on Fe(3)O(4)@TiO(2) isolation and SERS immunoassay. Biosens. Bioelectron. 148, 111800. doi:10.1016/j.bios.2019.111800
Paskeh, M. D. A., Entezari, M., Mirzaei, S., Zabolian, A., Saleki, H., Naghdi, M. J., et al. (2022). Emerging role of exosomes in cancer progression and tumor microenvironment remodeling. J. Hematol. Oncol. 15, 83. doi:10.1186/s13045-022-01305-4
Patil, M., Saheera, S., Dubey, P. K., Kahn-Krell, A., Kumar Govindappa, P., Singh, S., et al. (2021). Novel mechanisms of exosome-mediated phagocytosis of dead cells in injured heart. Circulation Res. 129, 1006–1020. doi:10.1161/circresaha.120.317900
Qi, M. C., Zou, S. J., Han, L. C., Zhou, H. X., and Hu, J. (2009). Expression of bone-related genes in bone marrow MSCs after cyclic mechanical strain: Implications for distraction osteogenesis. Int. J. Oral Sci. 1, 143–150. doi:10.4248/ijos.09021
Robbins, P. D., and Morelli, A. E. (2014). Regulation of immune responses by extracellular vesicles. Nat. Rev. Immunol. 14, 195–208. doi:10.1038/nri3622
Robling, A. G., and Bonewald, L. F. (2020). The osteocyte: New insights. Annu. Rev. Physiol. 82, 485–506. doi:10.1146/annurev-physiol-021119-034332
Roseti, L., Parisi, V., Petretta, M., Cavallo, C., Desando, G., Bartolotti, I., et al. (2017). Scaffolds for bone tissue engineering: State of the art and new perspectives. Mater Sci. Eng. C Mater Biol. Appl. 78, 1246–1262. doi:10.1016/j.msec.2017.05.017
Salazar, V. S., Gamer, L. W., and Rosen, V. (2016). BMP signalling in skeletal development, disease and repair. Nat. Rev. Endocrinol. 12, 203–221. doi:10.1038/nrendo.2016.12
Salunkhe, S., DheerajBasak, M., Chitkara, D., and Mittal, A. (2020). Surface functionalization of exosomes for target-specific delivery and in vivo imaging & tracking: Strategies and significance. J. Control Release 326, 599–614. doi:10.1016/j.jconrel.2020.07.042
Scanzello, C. R., and Goldring, S. R. (2012). The role of synovitis in osteoarthritis pathogenesis. Bone 51, 249–257. doi:10.1016/j.bone.2012.02.012
Shi, Y., Guo, S., Liang, Y., Liu, L., Wang, A., Sun, K., et al. (2022). Construction and evaluation of liraglutide delivery system based on milk exosomes: A new idea for oral peptide delivery. Curr. Pharm. Biotechnol. 23, 1072–1079. doi:10.2174/1389201022666210820114236
Shin, J., Cho, J. H., Jin, Y., Yang, K., Lee, J. S., Park, H. J., et al. (2016). Mussel adhesion-inspired reverse transfection platform enhances osteogenic differentiation and bone formation of human adipose-derived stem cells. Small 12, 6266–6278. doi:10.1002/smll.201601868
Sohrabi, B., Dayeri, B., Zahedi, E., Khoshbakht, S., Nezamabadi Pour, N., Ranjbar, H., et al. (2022). Mesenchymal stem cell (MSC)-derived exosomes as novel vehicles for delivery of miRNAs in cancer therapy. Cancer Gene Ther. 29, 1105–1116. doi:10.1038/s41417-022-00427-8
Song, H., Li, X., Zhao, Z., Qian, J., Wang, Y., Cui, J., et al. (2019). Reversal of osteoporotic activity by endothelial cell-secreted bone targeting and biocompatible exosomes. Nano Lett. 19, 3040–3048. doi:10.1021/acs.nanolett.9b00287
Song, Y., Wang, M., Tong, H., Tan, Y., Hu, X., Wang, K., et al. (2021). Plasma exosomes from endometrial cancer patients contain LGALS3BP to promote endometrial cancer progression. Oncogene 40, 633–646. doi:10.1038/s41388-020-01555-x
Su, T., Xiao, Y., Xiao, Y., Guo, Q., Li, C., Huang, Y., et al. (2019). Bone marrow mesenchymal stem cells-derived exosomal MiR-29b-3p regulates aging-associated insulin resistance. ACS Nano 13, 2450–2462. doi:10.1021/acsnano.8b09375
Sun, Y., Liu, G., Zhang, K., Cao, Q., Liu, T., and Li, J. (2021). Mesenchymal stem cells-derived exosomes for drug delivery. Stem Cell Res. Ther. 12, 561. doi:10.1186/s13287-021-02629-7
Sung, B. H., Parent, C. A., and Weaver, A. M. (2021). Extracellular vesicles: Critical players during cell migration. Dev. Cell 56, 1861–1874. doi:10.1016/j.devcel.2021.03.020
Tandon, B., Blaker, J. J., and Cartmell, S. H. (2018). Piezoelectric materials as stimulatory biomedical materials and scaffolds for bone repair. Acta Biomater. 73, 1–20. doi:10.1016/j.actbio.2018.04.026
Tao, S. C., Yuan, T., Zhang, Y. L., Yin, W. J., Guo, S. C., and Zhang, C. Q. (2017). Exosomes derived from miR-140-5p-overexpressing human synovial mesenchymal stem cells enhance cartilage tissue regeneration and prevent osteoarthritis of the knee in a rat model. Theranostics 7, 180–195. doi:10.7150/thno.17133
Tevlin, R., desJardins-Park, H., Huber, J., DiIorio, S. E., Longaker, M. T., and Wan, D. C. (2022). Musculoskeletal tissue engineering: Adipose derived stromal cell implementation for the treatment of osteoarthritis. Biomaterials 286, 121544. doi:10.1016/j.biomaterials.2022.121544
Thery, C., Witwer, K. W., Aikawa, E., Alcaraz, M. J., Anderson, J. D., Andriantsitohaina, R., et al. (2018). Minimal information for studies of extracellular vesicles 2018 (MISEV2018): A position statement of the international society for extracellular vesicles and update of the MISEV2014 guidelines. J. Extracell. Vesicles 7, 1535750. doi:10.1080/20013078.2018.1535750
Thomas, B. L., Eldridge, S. E., Nosrati, B., Alvarez, M., Thorup, A. S., Nalesso, G., et al. (2021). WNT3A-loaded exosomes enable cartilage repair. J. Extracell. Vesicles 10, e12088. doi:10.1002/jev2.12088
Tian, T., Cao, L., He, C., Ye, Q., Liang, R., You, W., et al. (2021). Targeted delivery of neural progenitor cell-derived extracellular vesicles for anti-inflammation after cerebral ischemia. Theranostics 11, 6507–6521. doi:10.7150/thno.56367
Tian, T., Zhang, H. X., He, C. P., Fan, S., Zhu, Y. L., Qi, C., et al. (2018). Surface functionalized exosomes as targeted drug delivery vehicles for cerebral ischemia therapy. Biomaterials 150, 137–149. doi:10.1016/j.biomaterials.2017.10.012
Tian, T., Zhu, Y. L., Zhou, Y. Y., Liang, G. F., Wang, Y. Y., Hu, F. H., et al. (2014). Exosome uptake through clathrin-mediated endocytosis and macropinocytosis and mediating miR-21 delivery. J. Biol. Chem. 289, 22258–22267. doi:10.1074/jbc.m114.588046
Tu, C., Du, Z., Zhang, H., Feng, Y., Qi, Y., Zheng, Y., et al. (2021). Endocytic pathway inhibition attenuates extracellular vesicle-induced reduction of chemosensitivity to bortezomib in multiple myeloma cells. Theranostics 11, 2364–2380. doi:10.7150/thno.47996
Urano, E., Ablan, S. D., Mandt, R., Pauly, G. T., Sigano, D. M., Schneider, J. P., et al. (2016). Alkyl amine bevirimat derivatives are potent and broadly active HIV-1 maturation inhibitors. Antimicrob. Agents Chemother. 60, 190–197. doi:10.1128/aac.02121-15
Vader, P., Mol, E. A., Pasterkamp, G., and Schiffelers, R. M. (2016). Extracellular vesicles for drug delivery. Adv. Drug Deliv. Rev. 106, 148–156. doi:10.1016/j.addr.2016.02.006
Valadi, H., Ekstrom, K., Bossios, A., Sjostrand, M., Lee, J. J., and Lotvall, J. O. (2007). Exosome-mediated transfer of mRNAs and microRNAs is a novel mechanism of genetic exchange between cells. Nat. Cell Biol. 9, 654–659. doi:10.1038/ncb1596
Varga, Z., Feher, B., Kitka, D., Wacha, A., Bota, A., Berenyi, S., et al. (2020). Size measurement of extracellular vesicles and synthetic liposomes: The impact of the hydration shell and the protein corona. Colloids Surf. B Biointerfaces 192, 111053. doi:10.1016/j.colsurfb.2020.111053
Wan, Z., Zhang, P., Lv, L., and Zhou, Y. (2020). NIR light-assisted phototherapies for bone-related diseases and bone tissue regeneration: A systematic review. Theranostics 10, 11837–11861.
Wang, Y., Gao, C., Gao, T., Zhao, L., Zhu, S., and Guo, L. (2021). Plasma exosomes from depression ameliorate inflammation-induced depressive-like behaviors via sigma-1 receptor delivery. Brain Behav. Immun. 94, 225–234. doi:10.1016/j.bbi.2021.02.004
Wang, Y., Liu, J., Ma, J., Sun, T., Zhou, Q., Wang, W., et al. (2019). Exosomal circRNAs: Biogenesis, effect and application in human diseases. Mol. Cancer 18, 116. doi:10.1186/s12943-019-1041-z
Wang, Y., Zhang, L., Wang, K., Zhou, H., Li, G., Xu, L., et al. (2022). Circulating exosomes from mice with LPS-induced bone loss inhibit osteoblast differentiation. Calcif. Tissue Int. 111, 185–195. doi:10.1007/s00223-022-00977-x
Wang, Z., Kapadia, W., Li, C., Lin, F., Pereira, R. F., Granja, P. L., et al. (2021). Tissue-specific engineering: 3D bioprinting in regenerative medicine. J. Control Release 329, 237–256. doi:10.1016/j.jconrel.2020.11.044
Wang, Z., Zhao, F., Zhao, Y., Bai, L., and Hang, R. (2022). Simultaneously enhanced osteogenesis and angiogenesis via macrophage-derived exosomes upon stimulation with titania nanotubes. Biomater. Adv. 134, 112708. doi:10.1016/j.msec.2022.112708
Wei, F., Li, M., Crawford, R., Zhou, Y., and Xiao, Y. (2019). Exosome-integrated titanium oxide nanotubes for targeted bone regeneration. Acta Biomater. 86, 480–492. doi:10.1016/j.actbio.2019.01.006
Wei, H., Chen, Q., Lin, L., Sha, C., Li, T., Liu, Y., et al. (2021). Regulation of exosome production and cargo sorting. Int. J. Biol. Sci. 17, 163–177. doi:10.7150/ijbs.53671
Wortzel, I., Dror, S., Kenific, C. M., and Lyden, D. (2019). Exosome-mediated metastasis: Communication from a distance. Dev. Cell 49, 347–360. doi:10.1016/j.devcel.2019.04.011
Wu, H., Fu, M., Liu, J., Chong, W., Fang, Z., Du, F., et al. (2021). The role and application of small extracellular vesicles in gastric cancer. Mol. Cancer 20, 71. doi:10.1186/s12943-021-01365-z
Wu, Y., Li, J., Zeng, Y., Pu, W., Mu, X., Sun, K., et al. (2022). Exosomes rewire the cartilage microenvironment in osteoarthritis: From intercellular communication to therapeutic strategies. Int. J. Oral Sci. 14, 40. doi:10.1038/s41368-022-00187-z
Xi, X. M., Xia, S. J., and Lu, R. (2021). Drug loading techniques for exosome-based drug delivery systems. Pharmazie 76, 61–67. doi:10.1691/ph.2021.0128
Xu, R., Greening, D. W., Zhu, H. J., Takahashi, N., and Simpson, R. J. (2016). Extracellular vesicle isolation and characterization: Toward clinical application. J. Clin. Invest. 126, 1152–1162. doi:10.1172/jci81129
Xu, Z., Zeng, S., Gong, Z., and Yan, Y. (2020). Exosome-based immunotherapy: A promising approach for cancer treatment. Mol. Cancer 19, 160. doi:10.1186/s12943-020-01278-3
Yang, C., Dong, Z., Ling, Z., and Chen, Y. (2022). The crucial mechanism and therapeutic implication of RNA methylation in bone pathophysiology. Ageing Res. Rev. 79, 101641. doi:10.1016/j.arr.2022.101641
Yao, Y., Jiang, Y., Song, J., Wang, R., Li, Z., Yang, L., et al. (2022). Exosomes as potential functional nanomaterials for tissue engineering. Adv. Healthc. Mater, e2201989. doi:10.1002/adhm.202201989
Krishnan, Y., and Grodzinsky, A. J. (2018). Cartilage diseases. Matrix Biol. 71-72, 51–69. doi:10.1016/j.matbio.2018.05.005
Yu, D., Li, Y., Wang, M., Gu, J., Xu, W., Cai, H., et al. (2022). Exosomes as a new frontier of cancer liquid biopsy. Mol. Cancer 21, 56. doi:10.1186/s12943-022-01509-9
Yu, L., Sui, B., Fan, W., Lei, L., Zhou, L., Yang, L., et al. (2021). Exosomes derived from osteogenic tumor activate osteoclast differentiation and concurrently inhibit osteogenesis by transferring COL1A1-targeting miRNA-92a-1-5p. J. Extracell. Vesicles 10, e12056. doi:10.1002/jev2.12056
Yu, W., Hurley, J., Roberts, D., Chakrabortty, S. K., Enderle, D., Noerholm, M., et al. (2021). Exosome-based liquid biopsies in cancer: Opportunities and challenges. Ann. Oncol. 32, 466–477. doi:10.1016/j.annonc.2021.01.074
Zhang, F. X., Liu, P., Ding, W., Meng, Q. B., Su, D. H., Zhang, Q. C., et al. (2021). Injectable Mussel-Inspired highly adhesive hydrogel with exosomes for endogenous cell recruitment and cartilage defect regeneration. Biomaterials 278, 121169. doi:10.1016/j.biomaterials.2021.121169
Zhang, P. F., Gao, C., Huang, X. Y., Lu, J. C., Guo, X. J., Shi, G. M., et al. (2020). Cancer cell-derived exosomal circUHRF1 induces natural killer cell exhaustion and may cause resistance to anti-PD1 therapy in hepatocellular carcinoma. Mol. Cancer 19, 110. doi:10.1186/s12943-020-01222-5
Zhang, S., Chuah, S. J., Lai, R. C., Hui, J. H. P., Lim, S. K., and Toh, W. S. (2018). MSC exosomes mediate cartilage repair by enhancing proliferation, attenuating apoptosis and modulating immune reactivity. Biomaterials 156, 16–27. doi:10.1016/j.biomaterials.2017.11.028
Zhang, S., Teo, K. Y. W., Chuah, S. J., Lai, R. C., Lim, S. K., and Toh, W. S. (2019). MSC exosomes alleviate temporomandibular joint osteoarthritis by attenuating inflammation and restoring matrix homeostasis. Biomaterials 200, 35–47. doi:10.1016/j.biomaterials.2019.02.006
Zhang, Y., Hao, Z., Wang, P., Xia, Y., Wu, J., Xia, D., et al. (2019). Exosomes from human umbilical cord mesenchymal stem cells enhance fracture healing through HIF-1α-mediated promotion of angiogenesis in a rat model of stabilized fracture. Cell Prolif. 52, e12570. doi:10.1111/cpr.12570
Zhang, Y., Wang, X., Chen, J., Qian, D., Gao, P., Qin, T., et al. (2022). Exosomes derived from platelet-rich plasma administration in site mediate cartilage protection in subtalar osteoarthritis. J. Nanobiotechnology 20, 56. doi:10.1186/s12951-022-01245-8
Zhao, S., Mi, Y., Guan, B., Zheng, B., Wei, P., Gu, Y., et al. (2020). Tumor-derived exosomal miR-934 induces macrophage M2 polarization to promote liver metastasis of colorectal cancer. J. Hematol. Oncol. 13, 156. doi:10.1186/s13045-020-00991-2
Zhong, L., Liao, D., Li, J., Liu, W., Wang, J., Zeng, C., et al. (2021). Rab22a-NeoF1 fusion protein promotes osteosarcoma lung metastasis through its secretion into exosomes. Signal Transduct. Target Ther. 6, 59. doi:10.1038/s41392-020-00414-1
Zhou, Y., Li, P., Goodwin, A. J., Cook, J. A., Halushka, P. V., Chang, E., et al. (2019). Exosomes from endothelial progenitor cells improve outcomes of the lipopolysaccharide-induced acute lung injury. Crit. Care 23, 44. doi:10.1186/s13054-019-2339-3
Zhou, Y., Zhang, Y., Gong, H., Luo, S., and Cui, Y. (2021). The role of exosomes and their applications in cancer. Int. J. Mol. Sci. 22, 12204. doi:10.3390/ijms222212204
Keywords: exosome, bone tissue engineering, bone defect, regenerative materials, exosomal-based materials
Citation: Chen R, Cheng Y, Zhang W, Zhang H and Yuan X (2023) The biological applications of exosomal-based materials in bone/cartilage tissue engineering. Front. Mater. 10:1152378. doi: 10.3389/fmats.2023.1152378
Received: 27 January 2023; Accepted: 20 February 2023;
Published: 28 February 2023.
Edited by:
Min-Ho Kim, Kent State University, United StatesReviewed by:
Han Liu, Shanghai University, ChinaXiaoru Shao, Affiliated Hospital of Jining Medical University, China
Copyright © 2023 Chen, Cheng, Zhang, Zhang and Yuan. This is an open-access article distributed under the terms of the Creative Commons Attribution License (CC BY). The use, distribution or reproduction in other forums is permitted, provided the original author(s) and the copyright owner(s) are credited and that the original publication in this journal is cited, in accordance with accepted academic practice. No use, distribution or reproduction is permitted which does not comply with these terms.
*Correspondence: Hongbiao Zhang, Mjg5NTg3MDk4QHFxLmNvbQ==; Xiaoli Yuan, eXVhbnhsMTA4MEAxNjMuY29t
†These authors have contributed equally to this work