- 1Shenzhen Key Laboratory of Marine Bioresource and Eco-environmental Science, College of Life Sciences and Oceanography, Shenzhen University, Shenzhen, China
- 2College of Physics and Optoelectronic Engineering, Shenzhen University, Shenzhen, China
- 3School of Traffic and Environment, Shenzhen Institute of Information Technology, Shenzhen, China
- 4BNU-HKUST Laboratory of Green Innovation, Advanced Institute of Natural Sciences, Beijing Normal University at Zhuhai, Zhuhai, China
- 5College of Civil and Transportation Engineering, Shenzhen University, Shenzhen, China
Our study offers a quantitative framework for microbial-induced calcium carbonate precipitation (MICP) to uplift the properties of recycled aggregate concrete (RAC). In this regard, a marine alkalophilic bacterium Bacillus sp. B6 was employed, and its growth and mineralization efficiency under seawater conditions was investigated. Optimization of MICP was achieved with different nutrient sources and bacterial introduction methods (dip and spray). The efficiency of treated recycled aggregates (RA) was determined by using scanning electron microscopy (SEM) and energy dispersive X-ray (EDX) in combination with basic technologies of density improvement and water absorption. The results show that the optimal nutrient sources of carbon and nitrogen are glucose (7 g L−1) and tryptone (5 gL−1), respectively. Spray treated recycled aggregate (RA) is reported to be efficient with a reduction in water absorption (∼19%), and improvement in bulk (∼2.4%) and apparent density (∼1.7%). The effectiveness of the MICP treatment process is confirmed with SEM and EDX observations, indicating the filling of microcracks with calcium carbonates. As such, the mechanical properties of RAC with spray treated RA significantly increase by up to ∼24% in compressive strength as compared to the control treatment. Current findings will help revitalize the construction industry by utilizing the MICP-treated RA and minimizing the load on natural aggregates.
1 Introduction
The construction industry makes a significant contribution to waste material production i.e. 1.3 billion tonnes per year (Ghaffar et al., 2020) and CO2 emissions of up to 60% (Müller et al., 2013). It is estimated that around 70% of the human population will be in urban areas by 2050 and construction waste will increase to 2.2 billion tonnes by 2025 (Environment, 2018). Concrete is the major pillar of the modern construction industry, and demand for concrete is ever-growing, reaching up to 30 billion tons per year globally (Monteiro et al., 2017). Additionally, developing countries are financing huge in building concrete infrastructures and per capita production of concrete is higher than any other construction material. On the other hand, developed countries face the challenge of refurnishing or upgrading aged infrastructures (Monteiro et al., 2017). In this regard, investigation on minimizing the carbon footprints, reuse of construction waste, developing sustainable construction materials (Melton, 2022), and evaluating the consequences of construction waste on the environment (Zhao et al., 2020) is the prime focus in the construction industry.
Globally, natural aggregates (NA) consumption was 48.3 billion tons in 2015, and the demand is increasing by 1% per year (The Freedonia Group, 2019). Furthermore, it also has been predicted that the NA demand in the coming two or three decades will be doubled due to the current increasing rate of concrete production. An effective way to combat the depletion of NA would be choosing alternative construction material with construction and demolition (C&D) waste without sacrificing the performance of concrete (Zhao et al., 2020). Mistri et al. (2020) reported that around 40% of total municipal solid waste comes from C&D, which has been a serious problem for the environment and living biota. Generally, C&D waste materials disposed of in landfills cover huge land areas (Xiao, 2018) and contaminate soil and water resources. Apparently, converting C&D wastes into sustainable construction material will reduce the growing stress on NA resources, (Mukharjee and Barai, 2014), landfill area, soil and water contamination (Wang B. et al., 2021; Mistri et al., 2021).
The quality of recycled aggregate (RA) is based on the sources of involved materials (Kou and Poon, 2015) and their characteristics (Mistri et al., 2019). In addition, attached mortar (AM) on the surface of RA is weaker and more porous as compared to the old parent rock (Behera et al., 2014). Moreover, RA has notably high water absorption (around 3%–12%) as opposed to NA (<1%), which ultimately affects the hardened and fresh properties of recycled aggregate concrete (RAC) (Khan et al., 2018). Micro-pores and micro-cracks on RA result in weak interfacial properties (Kisku et al., 2017). It is found that RAC with 100% RA has 30% less compressive strength as compared to natural aggregate concrete (Pradhan et al., 2017). Most RAC originating from C&D waste material generally have lower grades as they already serve their services. Thus, pre-processing and proper characterization are imperative to achieve better quality RAC.
Recent research found that RAC can be strengthened cost-effectively and environmentally friendly manner without the removal of AM (Wang et al., 2017; Zeng et al., 2019; Ouyang et al., 2022). Strengthening AM fills the micro-cracks and pores of RAC, which leads to improvements in mechanical properties and less water absorption (Kaur et al., 2020). The application of microbial-induced calcium carbonate precipitation (MICP) by the bio-mineralization process has emerged as a highly effective and innovative method in improving the properties related to concrete infrastructure (Wang et al., 2017; Cao et al., 2018; 2020; Joshi et al., 2019; Zhan et al., 2019; Feng et al., 2020). This methodology is also called bio-deposition, as bacteria are used on the surface or inside the concrete structure to precipitate the CaCO3 through the bio-mineralization process. The basic principle of the bio-mineralization process is that the bacterial vegetative cells produce CO32− via a series of metabolic processes, and at the end CO32− reacts with Ca2+ to produce CaCO3 precipitation as shown in Eq. 1. Furthermore, it is important to assure the viability of bacterial spores (Huang et al., 2022).
Recently, some researchers have tried to apply MICP to improve RA properties. Several scientists (Lu et al., 2022; Ouyang et al., 2022) investigated RA modification by applying MICP, and their results confirmed the effectiveness and feasibility of microbial modification. Wang et al. (2017) applied MICP and found that water absorption of RAC for 6–8 mm aggregate range was improved by 14.5%, while a 13.9% increase was observed for the 12–16 mm aggregate range. This water absorption improvement could be due to calcite precipitation induced by bacteria inside the RAC pores. However, overall MICP efficiency on RAC is dependent on many factors, including types of cementitious media, bacterial culture concentration, method of application (i.e. spraying or immersion), environmental conditions, aggregates size, etc.
The long-term durability concerns have limited the use of RA in concrete structures. However, RAC is an emerging topic in the construction industry due to the depletion of NA resources and the rapid increase in construction waste (Beattie et al., 2022; Rosa et al., 2022). So far, there is no detailed research and mechanistic understanding of improving RA strength by MICP and its application in RAC under seawater conditions. Previously, the majority of studies aiming at improving RA properties using MICP techniques were supplemented with additional calcium sources which compromise the economic viability of RAC (Wu et al., 2018; Zeng et al., 2019; Zhan et al., 2019; Feng et al., 2020). Moreover, those studies only investigated the effect of MICP on RA via dip or immersion method that was particularly made for laboratory-based bio-deposition.
To the best of our knowledge, no study has been conducted so far on the commercial generation of RAC with MICP that can be applied for to the construction of infrastructure in seawater. Therefore, this study was designed for the effective application of MICP in the modification of RA and production of sustainable RAC for the marine environment. In this regard, a marine alkalophilic bacterium Bacillus sp. B6 which was isolated previously and stored in our lab was employed, and its growth and mineralization efficiency under seawater conditions was investigated and optimized. Modification of RA was carried out by applying MICP under the proposed growth conditions and various exposure methods (dip and spray) were applied for introducing MICP on RA, and the efficiency of RA was visualized by using scanning electron microscopy (SEM) and energy dispersive X-ray (EDX) in combination with basic technologies of density improvement and water absorption. Finally, the modified RA was used to develop the concrete specimen and its compressive strength was tested. The compressive strength and micro properties of the RAC were observed to predict the MICP mechanism. Our results form the basis for optimizing the culturing condition and identifying the feasibility of using RA with spray treatment to enhance the MICP. The current study provides novel and valuable information for our mechanistic understanding and improved properties of RA and RAC. The developed method is expected to boost RA utilization in the concrete industry, leading toward the sustainable construction era.
2 Experimental program
2.1 Recycled aggregates
The RAs used in this work were recycled aggregates obtained from a demolished building waste landfill site in Shenzhen, China and shown in Figure 1. RAs were divided into two particle sizes, i.e. 5–10 mm and 10–20 mm. A constant weight of RA was achieved by drying at 80°C and repeatedly cleaning with tap water to remove the impurities.
2.2 Bacterial strain and optimum cultivation
Bacillus sp. B6, which was screened from the coastal sediments of mangrove forests in Futian, Shenzhen, and recognized as alkalophilic Bacillus, was used in this study. The optimum condition for culture growth was obtained via various carbon and nitrogen sources, later best source of carbon and nitrogen was further applied to detect their suitable concentration for bacterial growth. The bio-deposition treatments on RA were selected on the basis of best-grown culture. Figure 2 shows the overall schematic illustration of the experimental procedure adopted for this study.
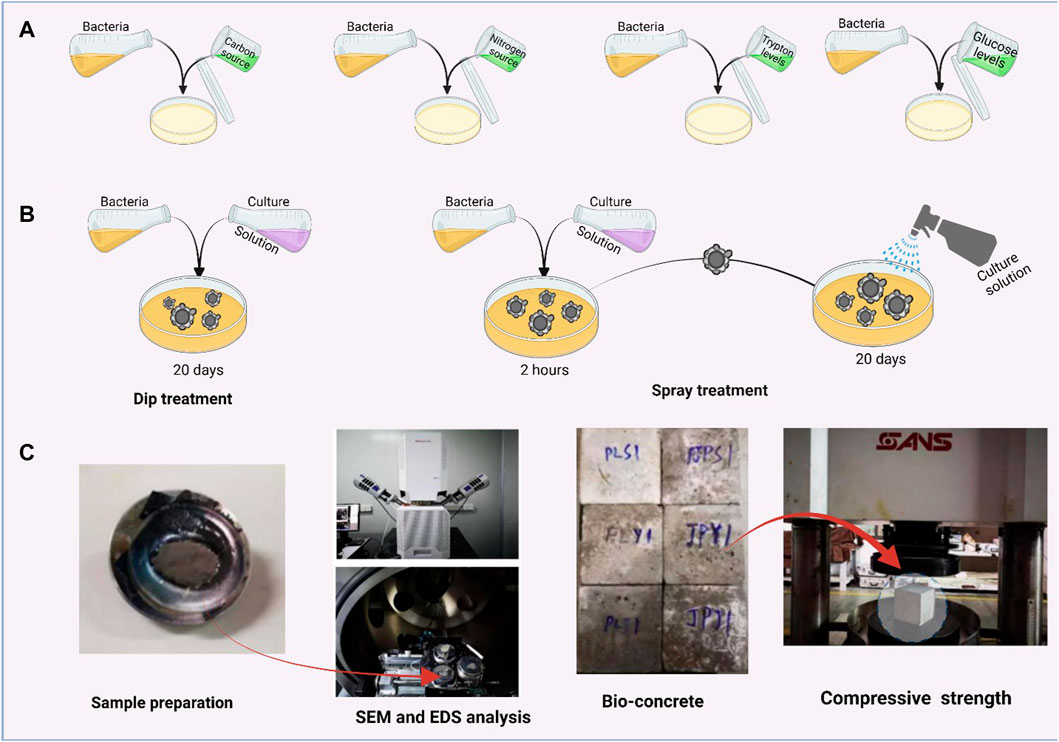
FIGURE 2. Schematic illustration of experimental procedures (A) Optimization of bacterial growth under different sources of carbon and nitrogen (B) Bio deposition of recycled aggregates via dip and spray treatments (C) SEM-EDX analysis of treated aggregates, formation of the concrete specimen and testing of flexural strength.
2.3 Seawater mineralization solution
The composition of the seawater mineralization solution is shown in Table 1. The pH of the solution was adjusted to 10.5 by NaOH to provide an alkaline environment for microbial mineralization and deposition.
Artificial sea water solution A (ASW-A) and artificial sea water solution B (ASW-B) solution refer to basic seawater mineralization medium and their composition is provided below in Table 2 and Table 3.
2.4 Bacterial deposition treatment on RA
RAs were treated by using two methods, i.e. dip and spray treatments. In dip treatment, RAs were soaked in seawater mineralization solution containing B6 at a concentration of 1 × 108 cells mL−1. The blank treatment group contains only deionized water without the addition of bacteria, the nutrient group contains nutrient solution without bacteria, and the mixed group contains both bacteria and nutrient solution. The soaking time for dip treatment was 20 days, and the ambient temperature was 27°C. For spraying treatment, RAs were firstly soaked in seawater mineralization solution containing B6 for 120 min to allow B6 cells to stick to the surface of RA. After that, RAs were taken out and sprayed with the same mineralization culture solution every 12 h. The spray time was 20 days and the ambient temperature was controlled at 27 C. Further information regarding experimental treatments for both dip and spray methods is provided in Table 4.
2.5 Effect of bacterial deposition
The treatment efficiency was quantified regarding water absorption, bulk density, and apparent density. High-resolution SEM-EDX analysis of RA under different treatment methods was performed to show the precipitation for filling of pores and cracks in RA and change in their elemental composition.
2.5.1 Water absorption
Both treatments and all three groups were subjected to water absorption test. Water absorption refers to the percentage of weight of water in the saturated state of RA to the weight of RA in the state of net dry weight. The experiment was carried out in accordance with the relevant regulations in “Recycled Coarse Aggregate for Concrete” (GB/T 25,177-2010). A portion of cleaned and dried RA (about 500 g) was placed in a white square box and soaked in water. The water surface should be about 5 mm higher than the surface of the RA sample. After soaking for 24 h, take it out of the box, and dry the surface of the aggregate by air blower to achieve saturated surface dry state. The mass was immediately weighed, recorded as G1. Then the sample was placed in a blast drying oven with a temperature of 105 C to dry to constant weight, which is the aggregate in the state of net dry weight. The sample was weighed after cooling to room temperature, recorded as G2. All the measurement was repeated three times. Water absorption rate is determined based on following formula as shown in Eq. 2.
2.5.2 Bulk density
Bulk density refers to the mass of recycled aggregate per unit volume in the bulk state. The experiment was carried out according to the relevant regulation in “Recycled Coarse Aggregates for Concrete” (GB/T 25,177-2010). Cleaned and dried RA was poured evenly into a 500 mL volumetric cylinder, assuring aggregates were fallen naturally without touching the volumetric cylinder. After the cylinder was full, it was weighed and its mass and recorded as mt. Then the mass of the volume cylinder was weighed out and recorded as mv. The bulk density is measured based on the following formula as shown in Eq. 3, where V is the volume of the cylinder.
2.5.3 Apparent density
Apparent density refers to the mass per unit volume of recycled aggregate after the voids in the container and aggregate are removed. The cleaned and dried recycled aggregate was soaked into a 500 mL volumetric cylinder and shaken to remove air bubbles. After the bubbles were drained, water was poured into the volumetric cylinder and the apparent density was measured based on the relevant regulations described in “Recycled Coarse Aggregate for Concrete” (GB/T 25,177-2010).
2.5.4 Characterization of the biogenic precipitation
The morphology of the precipitates on the surface of the aggregates was investigated by use of a scanning electron microscope (SEM, JEOL JSM-7600F). Coating of dry samples with carbon layer was done before the examination. Furthermore, EDX measurement was also performed to double check the chemical composition of particles that were present on aggregate surface.
2.6 Production of RAC
Ordinary Portland cement (P.O. 42.5) produced by Fenyi Conch Cement Co., Ltd and China ISO standard sand provided by Xiamen Aisio Co., Ltd. were used to produce the concrete specimen. The MICP-treated RAs were deployed into RAC test blocks, and the size of the test block was kept 10 cm × 10 cm × 10 cm. A total of 9 different types of RAC were prepared, each of which was set up in three parallel groups. The changes in mechanical properties were observed by comparing the compressive strength of the test block types treated in different ways. The material used in the formulation of RAC is provided in Table 5.
2.6.1 Compressive strength of RAC
Compressive strength refers to the maximum pressure that the recycled concrete can withstand per unit area. The experiment was carried out with reference to the “Inspection Method of Cement Mortar Strength” (GB/T 17671-1999), with the following steps. Remove the test block from the curing room and clean the surface. Place the test block on the table of the pressure testing machine, and align the center intersection of the concrete with the center of the ball axis of the lower table. Adjust the upper plate to about 2 cm away from the test block. Adjust the loading speed of the press on the system to 0.5 MPa–0.8 MPa. Start the press, when the test block begins to approach failure and begins to deform rapidly, the system will stop pressurizing on its own, and record the failure load FC, accurate to 1 kN, based on following formula as shown in Eq. 4.
RC is the compressive strength (MPa), FC is the maximum load (N) at failure and A is the area of the pressed part in mm2.
2.7 Statistical analysis
All the experiments were performed in triplicates. The data were analyzed by analysis of variance (ANOVA) and the means were compared by Tukey’s test (p < 0.05) using the GraphPad Prism software (version 5.0). Error bars on each graph show the standard deviation.
3 Results and discussion
3.1 Effects of nutrient sources on optimal bacterial growth
Microbes play a major role in mineralization and precipitation through different metabolic activities involving various pathways in the presence of specific organic and/or inorganic nutrients in the surrounding environment (Garg et al., 2022). Almost all the microbes in the natural environment are able to induce the mineralization process, however, their efficiency of mineralization differs. In our previous work, we screened a marine bacterium Bacillus sp. B6 from the coastal sediments of mangrove forests and evaluated its calcium precipitation activity (CPA). Our result disclosed that B6 possesses high CPA, higher than most of the reported mineralization strains, indicating the potential for self-healing of concrete damage and modification of RA (Zhang et al., 2019).
On the other hand, even if one kind of bacterial strain has strong CPA, the quantity of bacterial cells is also a crucial influential factor for the effective modification of RA. In this regard, we identified the best source and optimal concentration of carbon (Figure 3A, B) and nitrogen (Figure 3C, D) nutrients for the growth of B6 bacterium in the seawater environment. Six sugars (glucose, maltose, fructose, mannose, sucrose soluble starch) and two organic acid salts (sodium formate and sodium L-lactate) were used as carbon sources to explore the effects of different carbon sources on the growth of B6 as shown in Figure 3A. It was found that the plateau OD600 values of mannose and sodium formate were the lowest. Additionally, L-sodium lactate had little effect on the growth of B6. The OD600 values of sucrose, soluble starch, maltose, fructose, and glucose in the plateau phase were relatively higher, indicating that the growth of vegetative cells was promoted to a certain extent. Among them, the OD600 value of glucose was the highest, reaching up to 0.33, and the lag period of glucose was also the shortest among the carbon sources. Therefore, glucose was identified as the dominant carbon source in B6 mineralized growth cultures. Furthermore, we tested the role of glucose on bacterial culture at different concentrations as shown in Figure 3B. We found that when glucose concentration is higher than 5 gL-1, the growth of vegetative cells is relatively vigorous. With the further increase of concentration, the OD600 value of the plateau phase is in the range of 0.52–0.53, and the difference is not large. In order to save costs, the glucose concentration at 7 gL-1 is found to be the dominant carbon source concentration.
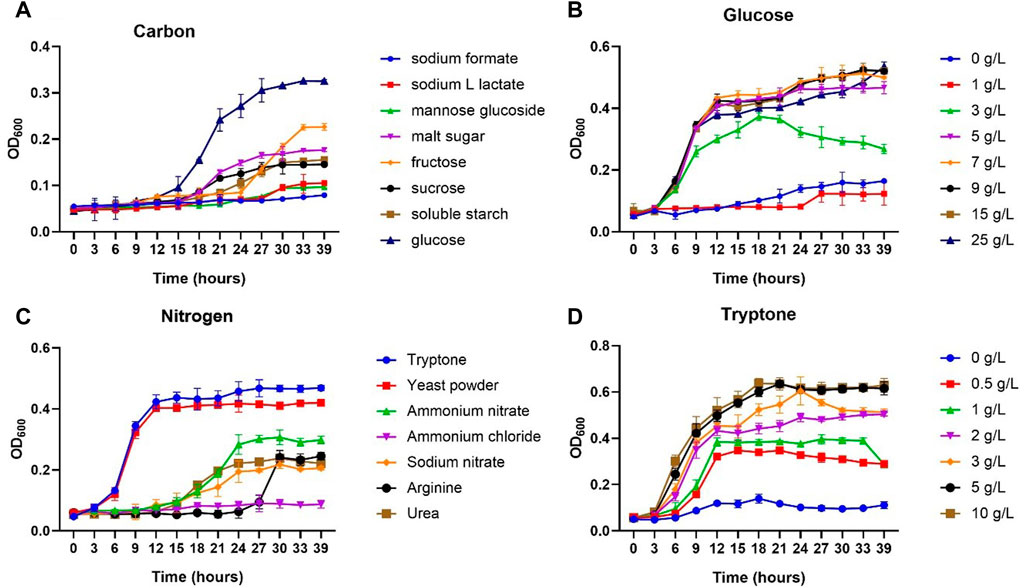
FIGURE 3. Detection of nutrient sources (A) different sources of carbon (B) different levels of glucose (C) different sources of nitrogen and (D) different levels of tryptone, at different time interval.
Similarly, we tested the effects of three organic nitrogen sources, yeast powder, tryptone, and urea, and four inorganic nitrogen sources including sodium nitrate, ammonium nitrate, ammonium chloride, and arginine, on the growth cycle of B6. Among them, tryptone was the best nitrogen source, and the OD600 value in the plateau phase was 0.47. As such, tryptone was chosen as the nitrogen source for the mineralization and growth of the B6 bacteria. Later, we applied different tryptone concentrations to isolate the optimum level of tryptone for B6 bacterial growth. A concentration level of 5 gL-1 was chosen as an effective tryptone concentration of nitrogen source.
Carbon and nitrogen sources are critical factors controlling the growth and CaCO3 precipitation (Zhang et al., 2019). CO32− in CaCO3 originates from enzymes and the synthesis of these enzymes depends on carbon and nitrogen metabolism (Bergh et al., 2020). Furthermore, carbon and nitrogen metabolism are interconnected, requiring shared functioning between these elements. The increase in the growth of bacteria increases the probability of mineralization to a certain extent, and maintaining a high cell number contributes to a high rate of mineralization deposition (Zhang et al., 2017). The rapid bacterial growth and reproduction of bacterial cells mean that bacteria synthesize a large number of intermediate metabolites with carbon/nitrogen sources by cellular respiration, so cellular respiration is strong, which helps to generate energy (Zhang et al., 2016a). Cell metabolism entails energy, and metabolism enhancement helps bacteria produce calcium carbonate as a metabolite and consequently improves the overall efficiency of mineralization (Zhang et al., 2016b; Seifan et al., 2018).
3.2 Evaluation of treatment method for bio deposition
3.2.1 Visualization of microbial deposition and characterization
The microstructure of RA treated with/without bacteria under different methods is shown in Figure 4. As shown in Figures 4A,B, the surface of RA treated in the mixed group was covered with a lot of particles from the dip and spray treatments, and the density of precipitates in the spraying group is higher than that in the dip group, almost covering the whole scanning area. However, the cracks and pores of RA in the nutrient group were only partially filled with a little substance no matter what treatment method was applied. Compared with the mixed group, almost no precipitation was observed on the surface of RA in the blank group. These results may suggest the contribution of bacteria in substance precipitation. The formation of precipitates in the nutrient group may also be due to certain unexpected bacteria as all the mineralization process in our experiment was not sterile cultivation. Moreover, the EDX analysis in Figure 4 disclosed that the main elements of the precipitates in the mixed group are C, O, and Ca, and the mass ratio of each element is close to 3:12:10.
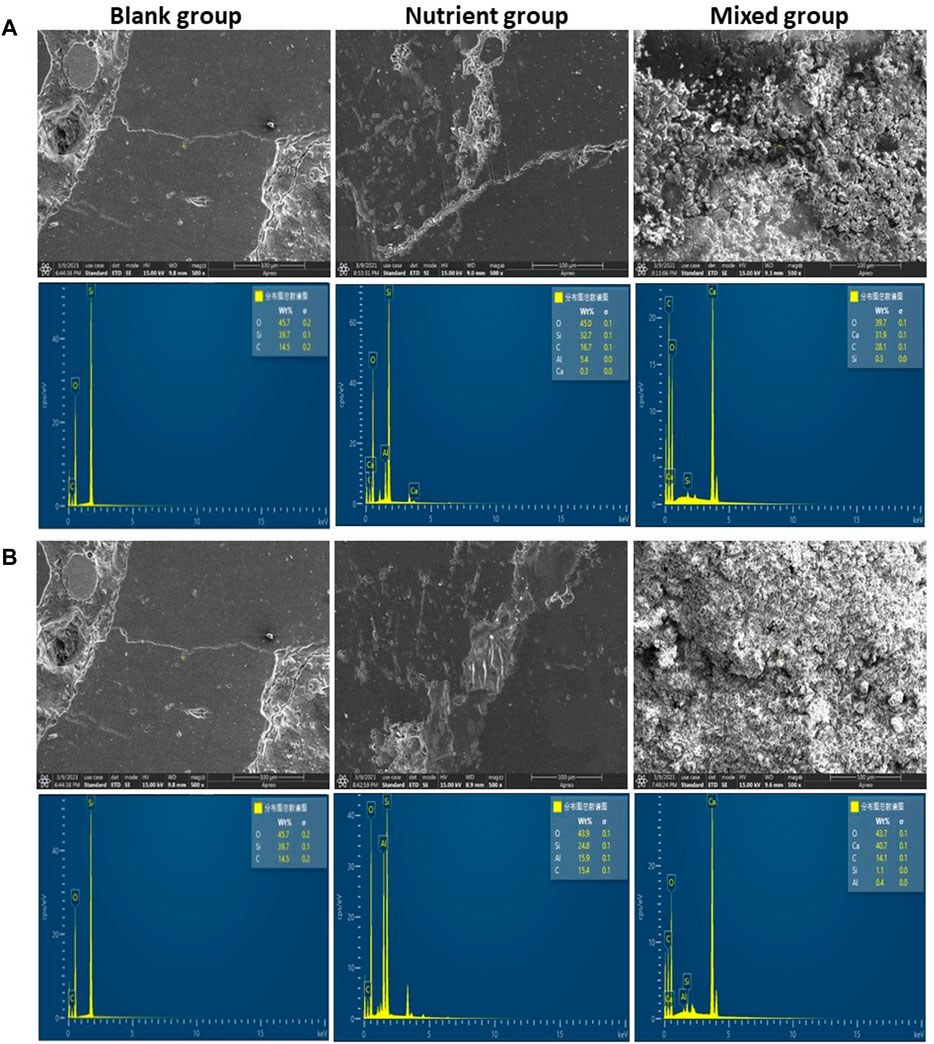
FIGURE 4. SEM-EDX images of RA exposed to blank (deionized water only), nutrient (nutrient solution only) and mixed group (nutrient solution + B6): (A) presents the dip treatment and (B) presents the spray treatments.
Combined with the higher resolution SEM microscopic morphology result in Figure 5, it can be inferred that the precipitate is calcium carbonate in calcite type. In addition, it can be noted that the contribution of B6 in the production of calcium carbonate can be further confirmed by the trace of bacterial cells, which is clearly visible and marked with a red circle in Figure 5.
3.2.2 Microbial community with nutrients modestly improves the duration indexes
The dense calcium carbonate layer on the surface of RA has a positive impact on improving the physical properties of RA and RAC. Herein, we evaluated the response of different duration indexes such as water absorption, bulk density, apparent density exposed to dip and spray treatment. Figure 6 shows the water absorption, bulk density and apparent density of RA before and after microbial modification in different treatment groups. For RA with diameter size 5–10 mm in the mixed group, the decline in water absorption was recoded ∼11% for dip treatment and ∼19% for spray treatment. No significant reduction in water absorption was observed with the increase in size of RA (diameter size 10–20 mm) with dip treatment. Moreover, it can be seen that in the absence of B6, no visible change of water absorption of RA was achieved under any treatment. Therefore, the reduction in water absorption of treated RA can be due to the presence of the MICP that plugged the microcracks (Zeng et al., 2019). Previously, Wu et al. (2018) adopted the bacterial respiration technique to produce calcium carbonate precipitation and reported a decline in water absorption of RA by 10, 13, and 23% for particle sizes of 20, 10, and 5 mm, respectively. This can be associated with the increase in micro-hardness of the interfacial transition zone of RA after microbial treatment. It was possible to obtain a reduction in water absorption of aggregate (Qiu et al., 2014), and the effect would be more visible in the case of RA with small size originating from inferior quality concrete.
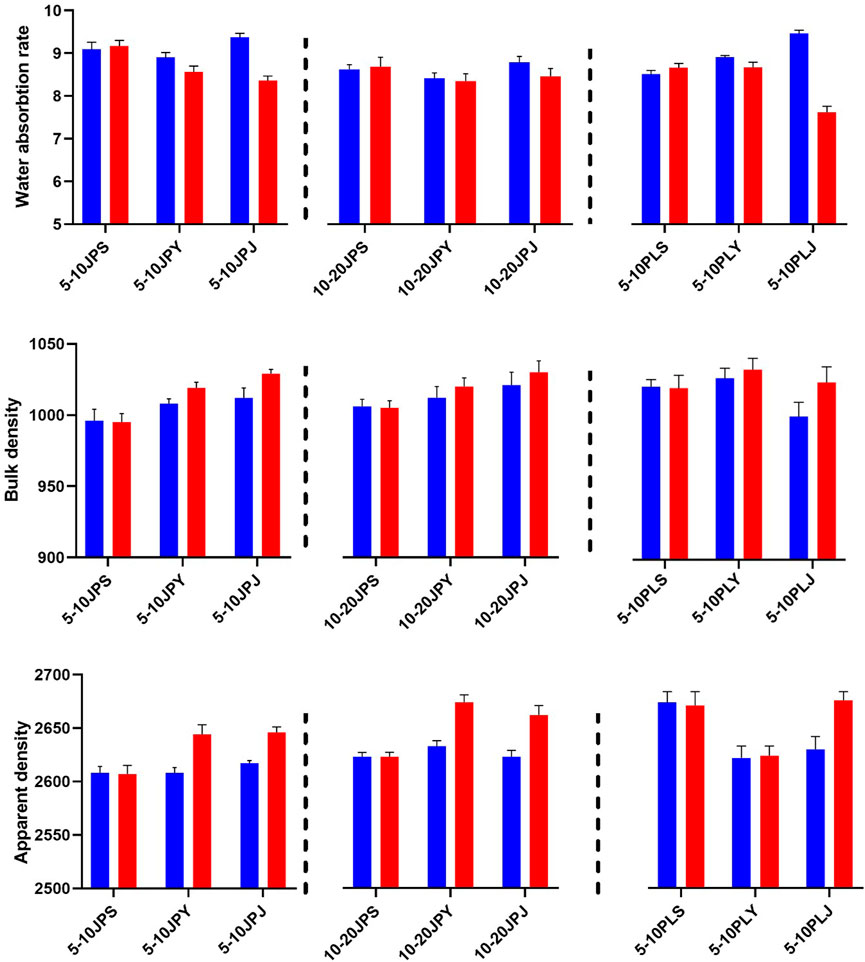
FIGURE 6. Effect of dip and spray treatment on water absorption, bulk density and apparent density. For all the samples, “JP” presents dip treatment, “PL” presents spray treatment. The last letter S, Y, and J presents blank (distilled water only), nutrient (nutrient solution only) and mixed (nutrient + B6) group, respectively. 5–10 and 10–20 are the size of recycled aggregates in millimeter (mm). The color of bar presents before and after treatment for blue and red, respectively.
In addition, the effect of MICP treatment on the bulk and apparent density of RA in different groups is similar to that on water absorption as shown in Figure 6. Compared with the blank group, the bulk density of the mixed group for dip treatment was ∼1.7% and 2.4% increase for spray treatment in case of RA diameter size range 5–10 mm. Similarly, the increase in apparent density was recorded after treatment for RA (diameter size 5–10 mm) ∼1.5% in the case of the mixed group for dip treatment and ∼1.7% for spray treatment. Singh et al. (2018) investigated the modification effect of ureolytic/non-ureolytic bacteria on the interfacial transition zone of RA and reported the increase in bulk density of microbial-treated aggregates, which is in line with our experiment. The dense calcium carbonate layer formed on RA as shown in SEM results effectively reduce the water absorption and improve the bulk and apparent density of aggregate. Thus, the proposed modification techniques seem promising for the performance enhancement in terms of duration indexes of RA.
3.3 Proposed MICP treatment process for RAC production
We constructed the RAC based on the above-mentioned comparison of the effects on water absorption decrease and improvement in bulk and apparent densities of treated RA. The size of the RAC blocks was 10 cm × 10 cm × 10 cm, and a total of 9 blocks were prepared according to our desired research plan in Section 2.5. Each of the three groups was set in parallel, and the mechanical properties were observed by comparing the compressive strength of the test blocks.
The development of the compressive strength of concrete at age of 28 days made of RA exposed to dip or spray treatment was determined as shown in Figure 7. It was found that for RAC made of B6 dip-treated RA (5–10 mm), the compressive strength increased ∼16.7% as compared with the blank group. But for the same treatment, when the size of RA was 10–20 mm, the increase in the compressive strength of RAC was only ∼8.9% as compared to the blank group. This can be associated with the result of the higher water absorption in larger sizes of RA as previously stated in Section 3.1 and 3.2. Earlier Yu et al. (2019) also reported a decrease in compressive strength with the increase in RA size. In addition, the small particle size of RA may provide a larger specific surface area, which is more suitable for the adhesion of microorganisms followed by an increase in the compressive strength of RAC.
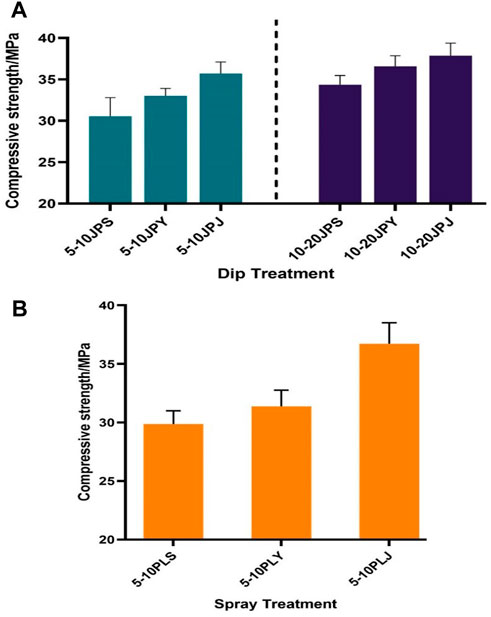
FIGURE 7. Effect of (A) dip and (B) spray treatment on compressive strength of recycled aggregates concrete (RAC). For all the samples, “JP” presents dip treatment, “PL” presents spray treatment. The last letter S, Y, and J presents blank (distilled water only), nutrient (nutrient solution only) and mixed (nutrient + B6) group, respectively. 5–10 and 10–20 are the size of RAC in millimeter (mm).
It is worth mentioning that by use of B6 spray-treated RA of the same size (5–10 mm), the compressive strength of RAC increased ∼24.1% compared with the blank group, higher than the 16.7% achieved by dip-treatment. The higher efficiency of spray treatment can actually be explained by the above SEM result that more substances were precipitated on RA under spray treatment. The reason why spray treatment is superior to dip treatment is probably that, compared with immersing aggregates totally under the water, spraying allows bacterial cells growing on the surface of the aggregates to acquire more oxygen from the air, thus achieving higher respiration efficiency. This is good because a better result can be obtained by using a lesser amount of microbes and nutrient culture via spray treatment. Treatment of RA can be economized by spraying bacterial culture onto the surface of RA at regular intervals and thus achieving long-lasting concrete structures on an industrial scale. Zhan et al. (2019) reported a ∼32% increase in the compressive strength of mortar containing recycled fine aggregates and treated with MICP under different sources of calcium. All the previous reported studies adopted different experimental conditions and the overall efficiency of the MICP on RAC depends on different factors, so the results are not comparable. As our results and experimental programs are novel, for reference we provide comprehensive information on previously published literature on RA treated with MICP in Table 6.
The approach adopted in this study holds more practical and economic benefits, especially for developing infrastructure in a seawater environment with spray-treated MICP RAC. It is evident that the MICP modification via spray treatment has a beneficial effect on the physical properties of RA and RAC. The development of RAC through the suggested treatment method will help to reduce the application of natural aggregates, decrease the overall cost and protect the environment from the hazardous effects of the construction industry. Furthermore, this approach can be adopted to develop infrastructure in a sea environment, stream restoration and reduced the carbon footprints of the construction industry as shown in Figure 8.
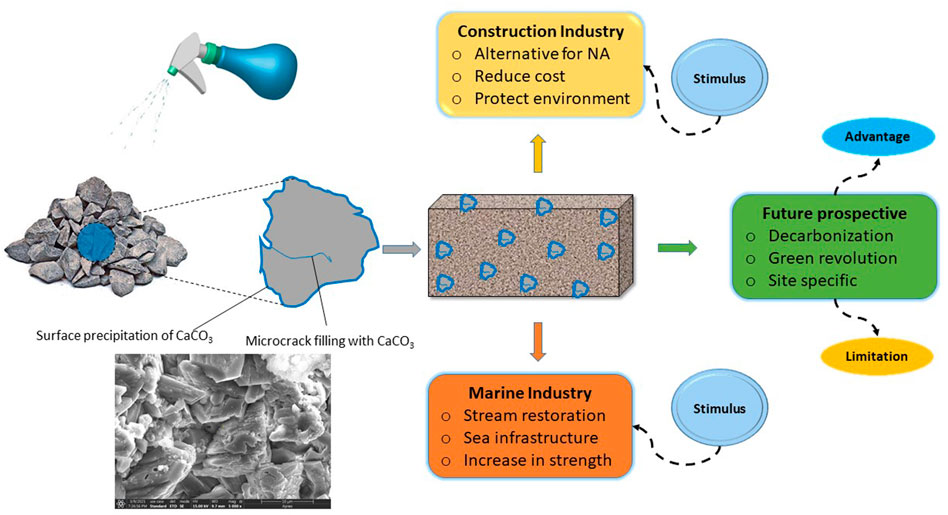
FIGURE 8. Schematic illustration of evaluation of RA treated with MICP, emerging role in construction industry/marine industry with future goals and prospects.
The proposed treatment process in this study can be used as a reference in engineering applications. However, due to the limited influencing factors taken into consideration in this study, whether the parameters reported here are optimal for other experimental conditions might require further verification. To meet the requirements of application in engineering practice, it is essential to continue optimizing the proposed MICP treatment process under different environment scenarios. For example, the current study proves that spraying culture medium on the surface of RA instead of soaking the RA in culture medium is more efficient and economical treatment method. The indentification of bacteria with higher natural MICP ability, cheaper nutrients, industrial wastewater containing calcium sources and testing of proposed approaches in changing environment is worth more in-depth exploration for future studies.
4 Conclusion
With the development of the sustainable cement industry, the self-healing properties of concrete have received widespread attention. As such, current findings will help to revitalize the construction industry by utilizing the self-healing RA to produce RAC and minimize the load on natural aggregates. Herein, the optimization of the MICP treatment process with different nutrient sources for seawater environment was achieved, improving the properties of RA. In particular, dip and spray methods were employed to test the modification effects on RA and RAC. Based on the experimental results, the following conclusions can be summarized.
• The carbon and nitrogen sources in seawater environments were optimized to cultivate bacteria with excellent growth ability that can effectively facilitate the MICP process on RA in seaside environments.
• The optimal nutrient sources of carbon and nitrogen were detected as glucose (7 gL-1) and tryptone (5 gL-1) respectively.
• The physical properties of RA, treated with spray treatment are significantly improved as compared to the untreated and dip treatment, especially for aggregates with a smaller particle size (5–10 mm). Visual observation with SEM elucidates micro mechanism and the role of the MICP in treated RA. Furthermore, the in-depth understanding of MICP process is carried out via the elemental composition of precipitate with EDX.
• The results show that bacterial-treated RA supplemented with nutrients via spray treatment significantly affects RAC’s mechanical properties by improving compressive strength.
• Although this research provides a way forward for developing sustainable RAC with microbial-treated RA. Still, efforts should be made to identify the novel bacterial strains with higher MICP and ensure the working of the proposed approach in different environmental conditions. More studies should be planned to achieve healing effectiveness on larger RA.
Data availability statement
The original contributions presented in the study are included in the article/supplementary material, further inquiries can be directed to the corresponding authors.
Author contributions
MAA and BL: Conceptualization, Data curation, Writing–original draft. Formal analysis. QL: Methodology, Software. MA: Formal analysis. JZ: Investigation, Visualization. YZ: Supervision, Review and Editing. XD: Funding acquisition, Supervision, Writing–review and editing. All authors read and approved the manuscript.
Funding
This research is funded by China’s national key research and development project (Grants No. 2018YFE0125000, national natural science foundation (No. 52178229 and No. 51508338), and the Shenzhen R&D project of China (No. 20200812162503001).
Conflict of interest
The authors declare that the research was conducted in the absence of any commercial or financial relationships that could be construed as a potential conflict of interest.
Publisher’s note
All claims expressed in this article are solely those of the authors and do not necessarily represent those of their affiliated organizations, or those of the publisher, the editors and the reviewers. Any product that may be evaluated in this article, or claim that may be made by its manufacturer, is not guaranteed or endorsed by the publisher.
References
Beattie, R. E., Su, B., Thill, R., and Hristova, K. R. (2022). Recycled concrete aggregates are an economic form of urban riparian erosion management with limited impacts on freshwater chemistry and microbial diversity. J. Hazard. Mater. 434, 128934. doi:10.1016/j.jhazmat.2022.128934
Behera, M., Bhattacharyya, S. K., Minocha, A. K., Deoliya, R., and Maiti, S. (2014). Recycled aggregate from C&D waste and its use in concrete – a breakthrough towards sustainability in construction sector: A review. Constr. Build. Mater. 68, 501–516. doi:10.1016/j.conbuildmat.2014.07.003
Bergh, J. M., Miljević, B., Šovljanski, O., Vučetić, S., Markov, S., Ranogajec, J., et al. (2020). Preliminary approach to bio-based surface healing of structural repair cement mortars. Constr. Build. Mater. 248, 118557. doi:10.1016/j.conbuildmat.2020.118557
Cao, M., Khan, M., and Ahmed, S. (2020). Effectiveness of calcium carbonate whisker in cementitious composites. Period. Polytech. Civ. Eng. doi:10.3311/PPci.14288
Cao, M., Li, L., and Khan, M. (2018). Effect of hybrid fibers, calcium carbonate whisker and coarse sand on mechanical properties of cement-based composites. Mater. Construcción 68, e156. doi:10.3989/mc.2018.01717
Environment, U. N. (2018). Global status report 2018. UNEP - U. N. Environ. Programme. Available at: http://www.unep.org/resources/report/global-status-report-2018 (Accessed March 23, 2022).
Feng, Z., Zhao, Y., Zeng, W., Lu, Z., and Shah, S. P. (2020). Using microbial carbonate precipitation to improve the properties of recycled fine aggregate and mortar. Constr. Build. Mater. 230, 116949. doi:10.1016/j.conbuildmat.2019.116949
Garg, R., Garg, R., and Eddy, N. O. (2022). Microbial induced calcite precipitation for self-healing of concrete: A review. J. Sustain. Cement-Based Mater. 0, 1–14. doi:10.1080/21650373.2022.2054477
GB/T 17671-1999 (n.d.) Chinesestandard (2022). Available at: https://www.chinesestandard.net/PDF.aspx/GBT17671-1999 (Accessed June 9, 2022).
Ghaffar, S. H., Burman, M., and Braimah, N. (2020). Pathways to circular construction: An integrated management of construction and demolition waste for resource recovery. J. Clean. Prod. 244, 118710. doi:10.1016/j.jclepro.2019.118710
Huang, R., Lu, Y., Ahmad, M., Zhang, J., and Deng, X. (2022). The viability of spores is the key factor for microbial induced calcium carbonate precipitation. Appl. Microbiol. Biotechnol. 107, 543–552. doi:10.1007/s00253-022-12319-w
Joshi, S., Goyal, S., Mukherjee, A., and Reddy, M. S. (2019). Protection of concrete structures under sulfate environments by using calcifying bacteria. Constr. Build. Mater. 209, 156–166. doi:10.1016/j.conbuildmat.2019.03.079
Kaur, N. P., Majhi, S., Dhami, N. K., and Mukherjee, A. (2020). Healing fine cracks in concrete with bacterial cement for an advanced non-destructive monitoring. Constr. Build. Mater. 242, 118151. doi:10.1016/j.conbuildmat.2020.118151
Khan, M., Cao, M., and Ali, M. (2018). Effect of basalt fibers on mechanical properties of calcium carbonate whisker-steel fiber reinforced concrete. Constr. Build. Mater. 192, 742–753. doi:10.1016/j.conbuildmat.2018.10.159
Kisku, N., Joshi, H., Ansari, M., Panda, S. K., Nayak, S., and Dutta, S. C. (2017). A critical review and assessment for usage of recycled aggregate as sustainable construction material. Constr. Build. Mater. 131, 721–740. doi:10.1016/j.conbuildmat.2016.11.029
Kou, S., and Poon, C. (2015). Effect of the quality of parent concrete on the properties of high performance recycled aggregate concrete. Constr. Build. Mater. 77, 501–508. doi:10.1016/j.conbuildmat.2014.12.035
Lu, C.-H., Bu, S., Shahin, M. A., Zheng, Y., and Cheng, L. (2022). Mitigation of alkali-silica reaction by microbially induced CaCO3 protective layer on aggregates. Constr. Build. Mater. 328, 127065. doi:10.1016/j.conbuildmat.2022.127065
Mistri, A., Bhattacharyya, S. K., Dhami, N., Mukherjee, A., and Barai, S. V. (2020). A review on different treatment methods for enhancing the properties of recycled aggregates for sustainable construction materials. Constr. Build. Mater. 233, 117894. doi:10.1016/j.conbuildmat.2019.117894
Mistri, A., Bhattacharyya, S. K., Dhami, N., Mukherjee, A., and Barai, S. V. (2019). Petrographic investigation on recycled coarse aggregate and identification the reason behind the inferior performance. Constr. Build. Mater. 221, 399–408. doi:10.1016/j.conbuildmat.2019.06.085
Mistri, A., Dhami, N., Bhattacharyya, S. K., Barai, S. V., Mukherjee, A., and Biswas, W. K. (2021). Environmental implications of the use of bio-cement treated recycled aggregate in concrete. Resour. Conservation Recycl. 167, 105436. doi:10.1016/j.resconrec.2021.105436
Monteiro, P. J. M., Miller, S. A., and Horvath, A. (2017). Towards sustainable concrete. Nat. Mater 16, 698–699. doi:10.1038/nmat4930
Müller, D. B., Liu, G., Løvik, A. N., Modaresi, R., Pauliuk, S., Steinhoff, F. S., et al. (2013). Carbon emissions of infrastructure development. Environ. Sci. Technol. 47, 11739–11746. doi:10.1021/es402618m
Mukharjee, B. B., and Barai, S. V. (2014). Influence of incorporation of nano-silica and recycled aggregates on compressive strength and microstructure of concrete. Constr. Build. Mater. 71, 570–578. doi:10.1016/j.conbuildmat.2014.08.040
Ouyang, J., Liu, K., Sun, D., Xu, W., Wang, A., and Ma, R. (2022). A focus on Ca2+ supply in microbial induced carbonate precipitation and its effect on recycled aggregate. J. Build. Eng. 51, 104334. doi:10.1016/j.jobe.2022.104334
Pradhan, S., Kumar, S., and Barai, S. V. (2017). Recycled aggregate concrete: Particle Packing Method (PPM) of mix design approach. Constr. Build. Mater. 152, 269–284. doi:10.1016/j.conbuildmat.2017.06.171
Qiu, J., Tng, D. Q. S., and Yang, E.-H. (2014). Surface treatment of recycled concrete aggregates through microbial carbonate precipitation. Constr. Build. Mater. 57, 144–150. doi:10.1016/j.conbuildmat.2014.01.085
Rosa, L., Becattini, V., Gabrielli, P., Andreotti, A., and Mazzotti, M. (2022). Carbon dioxide mineralization in recycled concrete aggregates can contribute immediately to carbon-neutrality. Resour. Conservation Recycl. 184, 106436. doi:10.1016/j.resconrec.2022.106436
Seifan, M., Sarmah, A., Samani, A., Ebrahiminezhad, A., Younes, G., and Berenjian, A. (2018). Mechanical properties of bio self-healing concrete containing immobilized bacteria with iron oxide nanoparticles. Appl. Microbiol. Biotechnol. 102, 4489–4498. doi:10.1007/s00253-018-8913-9
Singh, L. P., Bisht, V., Aswathy, M. S., Chaurasia, L., and Gupta, S. (2018). Studies on performance enhancement of recycled aggregate by incorporating bio and nano materials. Constr. Build. Mater. 181, 217–226. doi:10.1016/j.conbuildmat.2018.05.248
The Freedonia Group (2019). Global construction aggregates. Free. Group. Available at:https://www.freedoniagroup.com/industry-study/global-construction-aggregates-3742.htm (Accessed March 23, 2022).
Wang, B., Yan, L., Fu, Q., and Kasal, B. (2021a). A comprehensive review on recycled aggregate and recycled aggregate concrete. Resour. Conservation Recycl. 171, 105565. doi:10.1016/j.resconrec.2021.105565
Wang, J., Vandevyvere, B., Vanhessche, S., Schoon, J., Boon, N., and De Belie, N. (2017). Microbial carbonate precipitation for the improvement of quality of recycled aggregates. J. Clean. Prod. 156, 355–366. doi:10.1016/j.jclepro.2017.04.051
Wang, R., Jin, P., Ding, Z., and Zhang, W. (2021b). Surface modification of recycled coarse aggregate based on Microbial Induced Carbonate Precipitation. J. Clean. Prod. 328, 129537. doi:10.1016/j.jclepro.2021.129537
Wu, C.-R., Zhu, Y.-G., Zhang, X.-T., and Kou, S.-C. (2018). Improving the properties of recycled concrete aggregate with bio-deposition approach. Cem. Concr. Compos. 94, 248–254. doi:10.1016/j.cemconcomp.2018.09.012
Xiao, J. (2018). “Recycled aggregate concrete,” in Recycled aggregate concrete structures springer tracts in civil engineering. Editor J. Xiao (Berlin, Heidelberg: Springer), 65–98. doi:10.1007/978-3-662-53987-3_4
Yu, F., Sun, D., Wang, J., and Hu, M. (2019). Influence of aggregate size on compressive strength of pervious concrete. Constr. Build. Mater. 209, 463–475. doi:10.1016/j.conbuildmat.2019.03.140
Zeng, W., Zhao, Y., Poon, C. S., Feng, Z., Lu, Z., and Shah, S. P. (2019). Using microbial carbonate precipitation to improve the properties of recycled aggregate. Constr. Build. Mater. 228, 116743. doi:10.1016/j.conbuildmat.2019.116743
Zhan, M., Pan, G., Wang, Y., Fu, M., and Lu, X. (2019). Recycled aggregate mortar enhanced by microbial calcite precipitation. Mag. Concr. Res. 72, 622–633. doi:10.1680/jmacr.18.00417
Zhang, J., Mai, B., Cai, T., Luo, J., Wu, W., Liu, B., et al. (2017). Optimization of a binary concrete crack self-healing system containing bacteria and oxygen. Materials 10, 116. doi:10.3390/ma10020116
Zhang, J., Wang, C., Wang, Q., Feng, J., Pan, W., Zheng, X., et al. (2016a). A binary concrete crack self-healing system containing oxygen-releasing tablet and bacteria and its Ca2+-precipitation performance. Appl. Microbiol. Biotechnol. 100, 10295–10306. doi:10.1007/s00253-016-7741-z
Zhang, J., Wu, R., Li, Y., Zhong, J., Deng, X., Liu, B., et al. (2016b). Screening of bacteria for self-healing of concrete cracks and optimization of the microbial calcium precipitation process. Appl. Microbiol. Biotechnol. 100, 6661–6670. doi:10.1007/s00253-016-7382-2
Zhang, J., Xie, L., Huang, X., Liang, Z., Liu, B., Han, N., et al. (2019). Enhanced calcite precipitation for crack healing by bacteria isolated under low-nitrogen conditions. Appl. Microbiol. Biotechnol. 103, 7971–7982. doi:10.1007/s00253-019-10066-z
Zhao, Y., Peng, L., Feng, Z., and Lu, Z. (2021). Optimization of microbial induced carbonate precipitation treatment process to improve recycled fine aggregate. Clean. Mater. 1, 100003. doi:10.1016/j.clema.2021.100003
Keywords: self-healing, recycled aggregates, microbial induced calcium precipitation, marine bacteria, spray treatment, mechanical properties
Citation: Ahmad MA, Liu B, Li Q, Adeel M, Zhang J, Zhou Y and Deng X (2023) Bio-deposition approaches for sustainable execution of recycled aggregates in concretes. Front. Mater. 10:1131673. doi: 10.3389/fmats.2023.1131673
Received: 25 December 2022; Accepted: 16 January 2023;
Published: 20 February 2023.
Edited by:
Xi Jiang, The University of Tennessee, Knoxville, United StatesReviewed by:
Erol Yilmaz, Recep Tayyip Erdoğan University, TürkiyeAyaz Ahmad, University of Galway, Ireland
Aatika Sikandar, Guangxi University, China
Copyright © 2023 Ahmad, Liu, Li, Adeel, Zhang, Zhou and Deng. This is an open-access article distributed under the terms of the Creative Commons Attribution License (CC BY). The use, distribution or reproduction in other forums is permitted, provided the original author(s) and the copyright owner(s) are credited and that the original publication in this journal is cited, in accordance with accepted academic practice. No use, distribution or reproduction is permitted which does not comply with these terms.
*Correspondence: Xu Deng, dengxu@szu.edu.cn; Yingwu Zhou, ywzhou@szu.edu.cn
†These authors contributed equally to this work