- 1School of Environmental and Chemical Engineering, Jiangsu Ocean University, Lianyungang, China
- 2Lianyungang Fire Rescue Detachment, Lianyungang, China
- 3Jiangsu Institute of Marine Resources Development, Lianyungang, China
Numerical model was built with fire dynamic simulator and theocratical simulation was carried out to investigate the suppression effect of water mist on ignition and combustion process of typical solid material polymethyl methacrylate under external radiant heat flux. Characteristic parameters such as ignition time, surface temperature, heat release rate and temperature distribution of flame central plane during ignition and combustion process under different thermal radiant fluxes were obtained and compared with experimental results. The suppression effect of spray droplets on ignition and combustion process was analyzed and discussed. The results show the theoretical calculations of combustion characteristic parameters are in good agreement with experimental measurements. Water mist droplets can effectively delay the ignition time. Quantitative data proves that the water mist flow rate at 0.9 L/(min·m2) can delay the ignition time of samples by about 1,100 s while the radiant heat flux is 50 kW/m2. The simulation results can provide theoretical support and data reference for typical solid material fire prevention and fire extinguishment in practice.
1 Introduction
Polymer-based organic synthetic materials have been widely used in various fields of production and daily life in modern society (An et al., 2022; Wang et al., 2023). For example, polymethyl methacrylate (PMMA), as typical thermoplastic material, has been widely used in machining, architectural decoration, and other fields because of its excellent properties. The polymer material is flammable, making it easy to burn and cause fire accidents (Nelson, 1990). A large number of experiments and numerical studies have been carried out on its combustion characteristics, especially systematic research on the combustion behavior under different radiant heat fluxes (Kashiwagi et al., 1979; Tsai et al., 2001; Urbas et al., 2004; Luche et al., 2011; Acem et al., 2017). Typical combustion parameters such as surface temperature (ST), smoke density and heat radiation during the pyrolysis and combustion process of burning samples were measured by cone calorimeter. Results showed that the surface temperature was about 673 K when the combustion occurred, and the external radiation heat flux and flame radiation heat were helpful to promote continuous combustion. Researchers have also carried out detailed experiments, theoretical analysis and discussion on PMMA combustion characteristic parameters such as heat release rate (HRR), mass loss rate (MLR) and ignition time (IT) (Yang et al., 2006; Wang et al., 2017; Chen et al., 2019). Others conducted relevant experimental and numerical studies on water mist fire suppression to explore the influence mechanism of water mist on extinguishing PMMA flame (Cong et al., 2005) and the interaction between water mist droplets and various types of flame spread and smoke movement (You et al., 2006).
Theoretical and experimental studies both showed that water mist, as one of the effective means for fire prevention, can efficiently inhibit the combustion behavior of solid combustibles under external radiant heat flux. In experimental studies, however, it is always difficult to strictly control the key parameters of water mist such as particle size and water flow rate as a single variable, and this further brings challenges especially when it is coupled with a cone calorimeter and other test platforms.
To obtain further insight into the suppression effect of water mist droplet on typical combustion parameters such as surface temperature and heat release rate of PMMA sheets applied to external radiant heat flux, a theoretical model based on previous studies was established in the present study and the calculation results were compared with experimental measurements to verify the reliability of the model. The ignition time, surface temperature, heat release rate and other parameters of the test samples were analyzed in detail. On this basis, water mist droplets were introduced and the influence on the ignition and combustion process was investigated. In particular, the suppression effect of different water mist flow rates on ignition time, surface temperature and heat release rate under external radiation was discussed. The results can provide theoretical basis and technical support for water-based fire suppression methods suitable for polymer fire scenario.
2 Numerical modeling
2.1 Basic configuration
The simulation software fire dynamic simulator (FDS), which was widely used in fire science research and fire protection technology, was selected as the numerical simulation tool. Validation studies showed the numerical tool was suitable for the simulation of combustion behavior of samples under external thermal radiation in a cone calorimeter. As shown in Figure 1A, the numerical model was built in an open space of 0.3 m × 0.3 m×0.5 m, and the flammable sample part was set on a 10 cm × 10 cm combustion platform according to the standard specifications of a cone calorimeter. The burning sample was located on the top of the platform. External thermal radiation was defined using the input parameter “EXTERNAL_FLUX” under the built-in “SURF” command of the numerical tool. Function EXTERNAL_FLUX was simply a virtual source that heats the solid. It can be thought as a perfect radiant panel or conical heating unit like cone calorimeter in real world. The unit of this parameter was kW/m2. Typical settings in present paper were 30, 35, 50 and 65 kW/m2. The water mist nozzle was arranged 140 mm above the center of the sample, and the mist cone angle was 54°. Under the conditions of defined height and water mist cone angle in Figure 1B, the cross-sectional area of the conical mist field formed on the upper surface of the combustion sample was 15,708 mm2 to ensure the sprayed droplets can completely cover the upper surface of the sample. In the numerical model, water droplets were introduced into the calculation zone via a sprinkler. Parameters such as particle velocity, flow rate and spray angle were defined. The initial speed of the droplets was set to 0.5 m/s. When water droplet strikes the sample surface, it stuck and was reassigned a new speed and direction. Since the surface was horizontal, the direction was randomly chosen. Once contacting the sample surface, the water droplets reduced the fuel pyrolysis rate by cooling the sample surface and also changing the chemical reactions that liberate fuel gases from the solid. A thermocouple was set in the center of the upper surface of PMMA sample to measure the surface temperature during the ignition and combustion process. Both the ambient temperature and the initial temperature of the spray droplets were set to 20°C.
2.2 Properties of burning sample
In the present study, the combustion samples are ordinary PMMA plates, which are colorless and transparent solid at room temperature. Four typical simulation conditions were set according to relevant literature (Table 1). Simulation conditions No. 1 and No. 3 were set strictly according to the experimental operating conditions, and water mist was introduced as a control parameter in conditions No. 2 and No. 4. See Table 1 for more specific parameter settings of the PMMA samples used in the numerical simulation.
2.3 Grid sensitivity analysis
To determine the reasonable grid size of the numerical model, the flame characteristic diameter size D* was investigated:
Where Q is the combustion heat release rate, kW; ρ∞ is the air density, and its value is 1.205 kg/m3; cp is the heat capacity of air, whose value is 1.005 kJ/(kg K); T∞ is ambient temperature, the value is 20°C (293.15 K); g is the acceleration of gravity, and the value is 9.8 m/s2.
The grid size is the most important numerical parameter in the model, as it dictates the spatial and temporal accuracy of the discretized partial differential equations. Gird sensitivity analysis considers the extent to which uncertainty in model inputs influences model output. According to the grid size requirement stated in FDS technical reference, it is suggested that the selection of numerical grid size δx should match with flame characteristic size D*. And more precisely, a certain multiple relationship between them should be ensured to obtain reliable calculation results. According to the experimental data (Wang et al., 2017), the corresponding flame characteristic size D* under different radiant heat flux conditions and the corresponding δx values under the multiple relationships of 5, 16, and 20 are calculated using Eq. 1, the results are shown in Table 2.
It can be seen that when the radiant heat flux is between 15 and 65 kW/m2, the corresponding heat release rate is about 3–8 kW, and the flame characteristic size D* lies between 0.0939 and 0.1390 m. Thus, the suggested grid size is 0.47–2.78 cm if calculated by 5–20 times. Within this range, the calculation results are proved to be reliable. The final grid size decision is the comprehensive consideration of both model accuracy and computer capacity. To determine the suitable grid size quantitatively, the radiant heat flux of 50 kW/m2 was taken as an example, four uniform grid sizes of 0.5 cm, 1.0 cm, 1.5 cm, and 2.0 cm were selected to carry out grid independence analysis.
The simulation result of surface temperature depicted in Figure 2 is observed to gradually increase under external radiant heat flux and tends to stabilize at constant temperature eventually. From the view of the calculation, results of the four grid sizes are basically identical. A detailed comparison indicates that the subtle difference is the minor variation of finally stable temperature. With the increase of mesh fineness, the surface temperature of samples eventually tends to coincide. Quantitative analysis shows that the temperature difference between the calculated results of 0.5 cm and 1.0 cm meshes at the stable stage is no more than ±1°C. This verifies the reliability of the numerical model and suggests that continuous improvement of the mesh fineness will no longer improve the accuracy of calculation results distinctly. Therefore, considering the result accuracy, time cost, and efficiency of numerical calculation, 1.0 cm grid is selected as the computational mesh size in the follow-up simulation study.
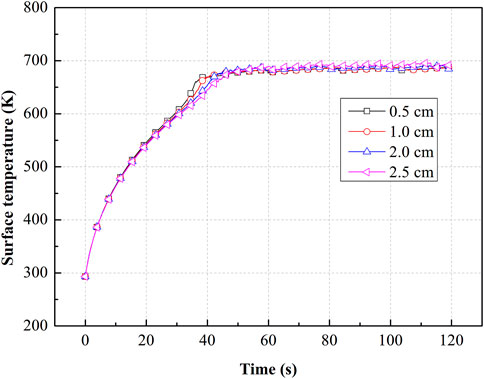
FIGURE 2. Surface temperature of samples with different grid sizes under radiant heat flux 50 kW/m2.
3 Results and discussion
3.1 Surface temperature of PMMA samples under thermal radiation
Due to its good heat conduction performance, temperature of combustible solid will rise quickly once exposed to external heat radiation. It is of great significance to explore the temperature change characteristic and identify its effects on ignition and combustion process, which plays an important role in fire prevention in real life.
Figure 3 presents the numerical simulation results and experimental data (Tsai et al., 2001) of surface temperature under 30 kW/m2 and 50 kW/m2 radiation fluxes. It is shown that the surface temperature rise of combustible samples under external radiant heat can be roughly divided into two phases: continuous rise and stabilization. At the initial stage of the temperature rise process, the calculation results of the theoretical model are in good agreement with the experimental measurements. As the heating time increases, both theoretical calculations and experimental measurements of surface temperature tend to stabilize and the final temperature of both methods are in clear agreement. It is worth noting that the temperature rise curve reveals the time reaching to the steady state of numerical simulation is obviously earlier than experimental tests. The reason for this is presumed to be the heat loss between the combustible solid surface and the surrounding environment in realistic experimental scenarios. Heat dissipated into the ambient environment leads to a slight decrease of the temperature rise rate compared with ideal conditions. Based on the above calculation results, the steady-state surface temperature maintains at 670–690 K and is consistent with the previous findings of PMMA spontaneous ignition critical temperature such as 695 ± 30 K (Gong et al., 2020), 650–680 K (Safronava et al., 2015) and 670–690 K (Lyon et al., 2007). These results verify the reliability of the numerical model and further confirm the accuracy of the simulation in predicting surface temperature of ignition and combustion process.
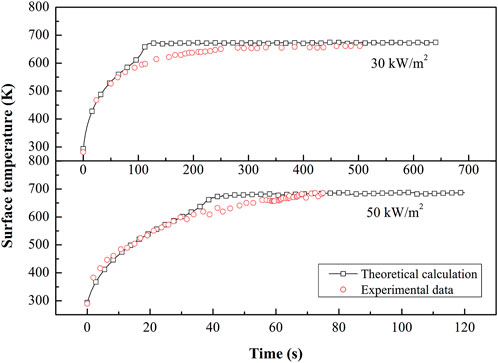
FIGURE 3. Theoretical calculation and experimental measurements of surface temperature under different radiant heat fluxes.
3.2 Effect of external radiant heat flux on heat release rate
Heat release rate of combustible materials, which has a significant impact on fire development and flame propagation rate, is an important parameter describing the combustion intensity. Combustible material exhibits different heat release rate properties in different burning scenarios or at different stages of the same fire scenario. For fire risk evaluation and fire prevention, it is necessary to understand the impact of radiant heat fluxes on heat release rate during ignition and fully developed combustion processes.
Figure 4 shows the heat release rate results from theoretical model and experiments (Wang et al., 2017) under three different radiant heat flux intensity (35 kW/m2, 50 kW/m2, and 65 kW/m2). From exposure to thermal radiation to the end of the experiment, the combustion process of all samples can basically be divided into four stages. At the first stage, the surface temperature of the sample gradually increases and no continuous flame is generated. With the exposure time increases, the sample surface reaches its ignition point and the sample starts to burn. The second stage is distinguished by a rapid increase in heat release rate, which is shown as a steep growth curve in the figure. Samples at the third stage are completely ignited. The heat release rates maintain at a high level and then further increase gradually to the maximum. With the continuous consumption of the sample, the heat release rates at the last stage begin to decline until the flame completely extinguished and eventually tend to zero. Both numerical and experimental results show significant effect of external radiant heat flux on the ignition time, the peak heat release rate, and the combustion duration. The higher the thermal radiation, the higher temperature rise rate and the shorter ignition time will be. In the ignition and full combustion phases, the radiant heat flux shows a significant influence on the heat release rate. The increase rate of HRR and peak HRR at fully developed combustion stage increase with raising external radiation intensity. This trend is consistent with both numerical and experimental results. The accurate prediction of HRR by simulation method further proves chosen model can provide reliable combustion characteristic data in ignition and combustion process.
3.3 Emperature field distribution on flame central plane
Accurate measurement of the whole temperature field distribution (TFD) of flame section by experimental methods is always difficult and the cost is generally enormous. However, it is convenient to obtain the temperature field through numerical simulation. To further investigate the evolution of flame shape and sectional temperature during the whole combustion process, Figure 5 shows the sequence diagram of temperature field distribution on the flame central plane. Overall, the evolution process of the flame shape, which intuitively shows four stages of the combustion process, is consistent with the variation of HRR under radiant heat flux 50 kW/m2 shown in Figure 4. According to the experimental results (Wang et al., 2017), the ignition time and time to peak HRR under 50 kW/m2 radiant heat flux are 16 s and 795 s respectively. The simulation results in Figure 4 show that the HRR starts to increase rapidly at about 15 s and achieves the peak at about 750 s. The temperature field profiles shown in Figure 5 also confirm the trend of fire development and flame evolution. These fundamental data of typical combustion process will be useful for comparing results of subsequent fire suppression with water mist.
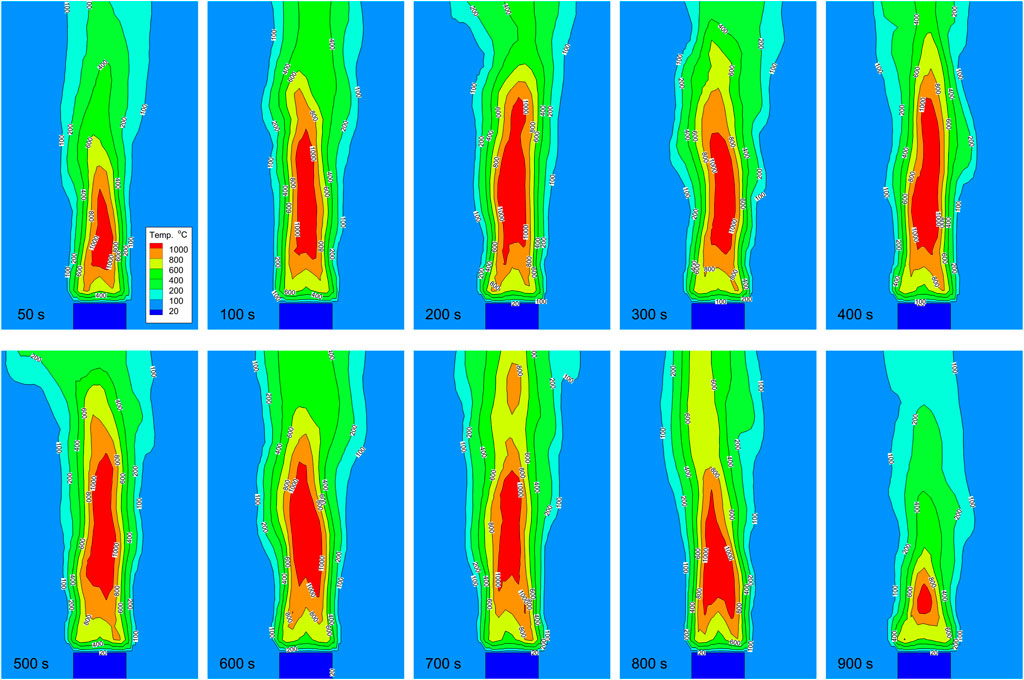
FIGURE 5. Temperature contour of flame central section of test No. 3 under radiant heat flux 50 kW/m2.
3.4 Suppression effect of water mist on ignition and combustion characteristics
The combustion behavior of flammable PMMA samples under different external radiative heat flux has been discussed above. In the actual fire protection scenario, it has realistic meaning and significance in inhibiting material ignition, reducing fire spread speed, and controlling fire-affected area by using the cooling effect of water droplets to prolong ignition time or reduce intensity of combustion. Based on the operating conditions No. 1 and No. 3, this section compares and analyzes the effect of water mist on surface temperature, heat release rate, and other combustion characteristic parameters. The intensity of external thermal radiation was set to 50 kW/m2 to simulate the heating state of solid combustibles in a fully developed fire scenario. Four spray flow rates at 0.50, 0.75, 0.90, and 1.00 L/(minm2) were selected in the model. Diameter of the droplet was 200 μm and the initial velocity was 0.5 m/s. The simulation duration was 1800–2,400 s. Development of ST, HRR, and TFD on flame central plane were recorded in Figure 6–8.
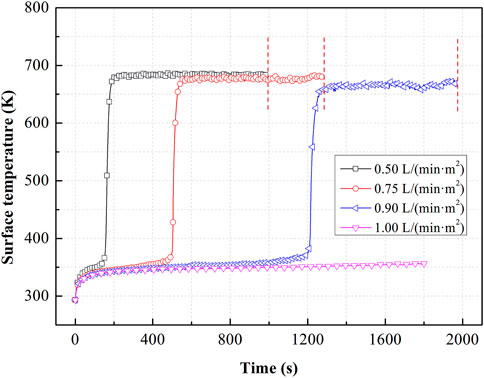
FIGURE 6. Surface temperature of test No. 2 at different water mist flow rates under radiant heat flux 50 kW/m2.
In Figure 6, the surface temperature rise curve has changed greatly compared with Figures 2, 3. The calculation results show that as the water mist flow rate increases, the initial surface temperature increase rate of samples exposed to radiant heat flux decreases significantly. And the corresponding ignition time is also evidently delayed. From Figure 6, the time periods before complete combustion phase of the first three samples applied with different mist flow rates are delayed by about 200 s, 560 s, and 1,330 s, respectively. It is also worth noting that the samples may still be ignited by external radiation as the exposure time increases. At the fully developed combustion stage, the surface temperature of burning samples maintains around 660–680 K, which decreases slightly with increasing of water mist flow rate. When the water mist flow rate reaches 1.0 L/(min·m2), the surface temperature will increase by only 60°C in 30 min without being ignited.
Figure 7 shows the effect of water mist at different flow rates on heat release rate during the ignition and combustion process under radiant heat flux 50 kW/m2. With the application of water mist droplets, especially as the increase of water flow rate, the ignition time of samples is distinctly delayed. The peak HRR is also lower compared with the case without water mist in Figure 4. No significant increase of HRR is observed when the water mist flow rate is 1.0 L/(minm2), indicating that the samples do not burn at all during the whole simulation. For selected water flow rates between 0.5 and 1.0 L/(minm2), when the flow rate is low, the droplets can decelerate the surface temperature rise by means of heat absorption and evaporation, thus further delay the ignition time. On the other hand, as the exposure time under the radiative heat flux increases, the strong thermal radiation intensity is still able to evaporate the droplets and finally ignite the samples. It is reasonable to deduce that when the spray flow rate increases to a certain level, even if the samples are exposed to external radiation for a sufficiently long time, they will still not be ignited. Based on the above results and analysis, the ignition time of samples exposed to external radiation 50 kW/m2 can be delayed by about 1,100 s while the water mist flow rate is 0.9 L/(min·m2). If the flow rate is continuously increased to 1.0 L/(min·m2), there is no combustion and flame propagation observed in 2,400 s. In former experiments (Cong et al., 2005), testing results showed the fire suppression time of water mist at flow rate 2.17 L/(min·m2) is 3.3 s and flame extinction time is 72.3 s. The tendency is consistent with the simulation results. Fire protection and suppression effect of water mist on solid combustible materials under external radiant heat flux shows enormous potential and significance for preventing solid fire accidents and extinguishing rapid fire spread at the initial stage.
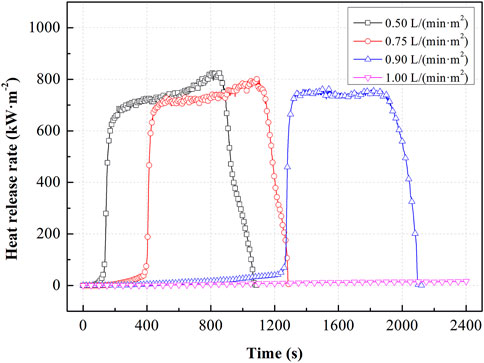
FIGURE 7. Heat release rate of test No.4 at different water mist flow rates under radiant heat flux 50 kW/m2.
Figure 8 depicts the temperature field distribution on the flame central plane. The radiation intensity is 50 kW/m2 and water mist flow rate is 0.75 L/(min·m2). Compared with the combustion process when no water spray was applied (Figure 5), the temperature field differences are mainly reflected in two aspects. Firstly, the continuous flame zone above the sample surface does not appear until 550 s continuous exposure to external radiation, confirming the significant delay of ignition time. Secondly, the high-temperature flame region shrinks and diverges clearly during the combustion process and the temperature of the gas-phase flame region visibly decreases, which indicate that the flame has been suppressed and cooled by water droplets effectively. The evolutionary trend is more noticeable with the continuous increase of water mist flow rate. The variation of the temperature field distribution on the flame central plane explains the reduction of ST and HRR, which further demonstrates the beneficial effect of water mist droplets in suppressing the solid combustibles fire in the presence of radiant heat.
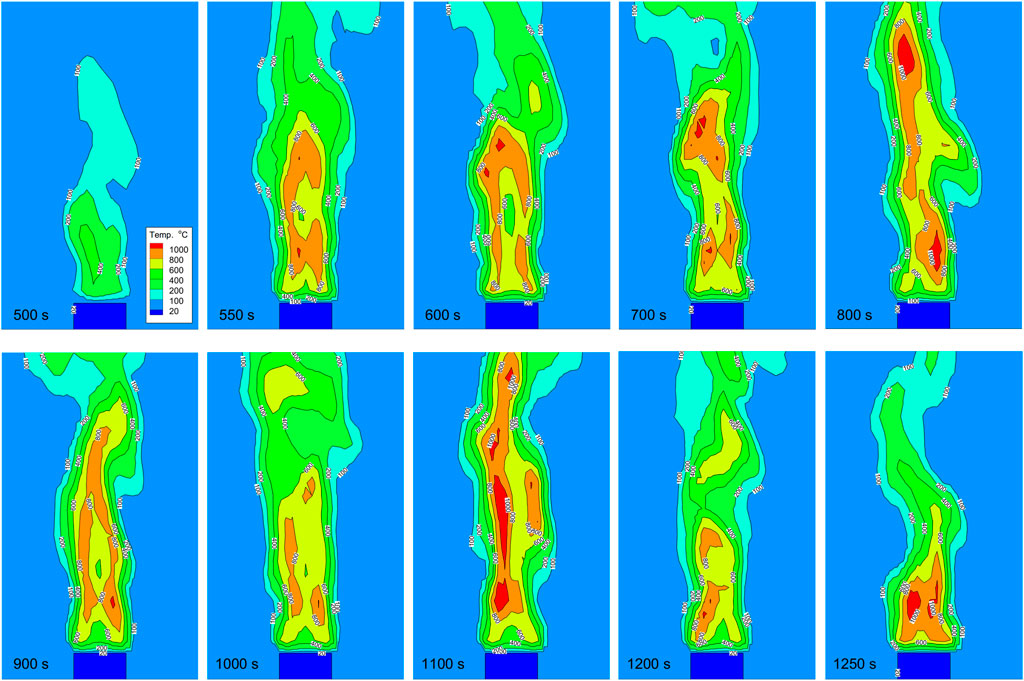
FIGURE 8. Temperature contour of flame central section of test No. 4 at water mist flow rate 0.75 L/(min·m2) under heat flux 50 kW/m2.
4 Conclusion
The suppression effect of water mist on the combustion of PMMA samples under external radiation intensity is studied by numerical simulation. The ignition and combustion process of PMMA sheet under external radiant heat flux was calculated theoretically by using the numerical simulation tool FDS. Characteristic parameters such as surface temperature and heat release rate during the ignition and combustion process were obtained and compared with the previous experiments. The results show that the theoretical calculation of surface temperature, heat release rate, and other combustion characteristic parameters are in good agreement with the experimental measurements. Fire suppression effects of water droplets on the ignition and combustion process of samples under heat radiation are analyzed and discussed. The surface temperature, combustion heat release rate, and flame morphology of the burning samples dramatically change with the application of water mist, indicating that water droplets can effectively delay the ignition time and inhibit the combustion process. Theoretical calculation shows water mist flow rate at 0.9 L/(min·m2) is perfectly capable of prolonging the ignition time of samples under external heat radiation 50 kW/m2 for about 1,100 s. This remarkable effectiveness shows positive significance for guiding fire rescue and fire prevention in practice. In the next step, more different particle sizes will be considered and the impact on heat release rate, mass loss rate, surface temperature and CO productivity will be studied and discussed to get a better understanding of the combustion and fire suppression process.
Data availability statement
The original contributions presented in the study are included in the article/supplementary material, further inquiries can be directed to the corresponding author.
Author contributions
LZ: conceptualization, methodology, writing-review and editing. DC: validation, writing and editing. YC: resources and data curation. HL: writing-review and editing, validation. TY: formal analysis and visualization. CL: writing-review and editing. HZ: resources, supervision and investigation.
Acknowledgments
The authors gratefully acknowledge the financial supports from the Natural Science Foundation of Jiangsu Province (BK20201030), the Jiangsu Province Industry-University-Research Cooperation Project (BY2021369), the Lianyungang Postdoctoral Research Funding Program (LYG2022004) and the Natural Science Research Project of Jiangsu Universities (17KJB620001).
Conflict of interest
The authors declare that the research was conducted in the absence of any commercial or financial relationships that could be construed as a potential conflict of interest.
Publisher’s note
All claims expressed in this article are solely those of the authors and do not necessarily represent those of their affiliated organizations, or those of the publisher, the editors and the reviewers. Any product that may be evaluated in this article, or claim that may be made by its manufacturer, is not guaranteed or endorsed by the publisher.
References
Acem, Z., Brissinger, D., Collin, A., Parent, G., Boulet, P., Quach, T. H. Y., et al. (2017). Surface temperature of carbon composite samples during thermal degradation. Int. J. Therm. Sci. 112, 427–438. doi:10.1016/j.ijthermalsci.2016.11.007
An, W., Shi, L., Cai, M., Li, S., Peng, L., and Wang, Z. (2022). Influence of window intervals on upward flame spread over discrete polymethyl methacrylate plates in vertical channel of building facades. J. Build. Eng. 46, 103775. doi:10.1016/j.jobe.2021.103775
Chen, X., Tang, Y., Wang, Q., and Wang, E. (2019). Analysis on the combustion performance of PMMA board. Fire Sci. Technol. 38, 1499–1502.
Cong, B., Qin, J., Liao, G., Yao, B., and Zhang, H. (2005). Experimental study of solid PMMA combustion characteristic under water mist. Prog. Nat. Sci. 15, 94–99.
Gong, J., Zhang, M., Zhai, C., Yang, L., Zhou, Y., and Wang, Z. (2020). Experimental, analytical and numerical investigation on auto-ignition of thermally intermediate PMMA imposed to linear time-increasing heat flux. Appl. Therm. Eng. 172, 115137. doi:10.1016/j.applthermaleng.2020.115137
Kashiwagi, T. (1979). Effects of attenuation of radiation on surface temperature for radiative ignition. Combust. Sci. Technol. 20, 225–234. doi:10.1080/00102207908946911
Luche, J., Rogaume, T., Richard, F., and Guillaume, E. (2011). Characterization of thermal properties and analysis of combustion behavior of PMMA in a cone calorimeter. Fire Saf. J. 46, 451–461. doi:10.1016/j.firesaf.2011.07.005
Lyon, R. E., and Quintiere, J. G. (2007). Criteria for piloted ignition of combustible solids. Combust. Flame 151, 551–559. doi:10.1016/j.combustflame.2007.07.020
Nelson, G. L. (1990). Fire and polymers: Hazards identification and prevention. Oxford, England: Oxford University Press.
Safronava, N., Lyon, R. E., Crowley, S., and Stoliarov, S. I. (2015). Effect of moisture on ignition time of polymers. Fire Technol. 51, 1093–1112. doi:10.1007/s10694-014-0434-1
Tsai, T. H., Li, M. J., Shih, I. Y., Jih, R., and Wong, S. C. (2001). Experimental and numerical study of autoignition and pilot ignition of PMMA plates in a cone calorimeter. Combust. Flame 124, 466–480. doi:10.1016/S0010-2180(00)00219-4
Urbas, J., Parker, W. J., and Luebbers, G. E. (2004). Surface temperature measurements on burning materials using an infrared pyrometer: Accounting for emissivity and reflection of external radiation. Fire Mater. 28, 33–53. doi:10.1002/fam.844
Wang, K., Liu, Y., Wang, X., Zhou, Y., Meng, X., and Wang, Q. (2017). Experimental study of the influence of heat flux on the combustion characteristics of polymethyl methacrylate. Fire Sci. Technol. 36, 16–19.
Wang, T., Tang, Y., Wang, Z., An, W., and Chen, X. (2023). Flame spread over cables in a utility tunnel: Effect of longitudinal wind and inclination angle. Tunn. Undergr. Space Technol. 131, 104848. doi:10.1016/j.tust.2022.104848
Yang, X., Peng, X., Lu, G., and Liu, S. (2006). The study of relationship between radiant heat flux and combustion parameter based on the CONE data. Fire Sci. Technol. 25, 469–472.
You, Y., Li, Y., Huo, R., Li, S., and Zhou, Y. (2006). Numerical simulation study on cabin fire under sprinkler control. Fire Sci. Technol. 25, 613–617.
Keywords: water mist, radiant heat flux, PMMA, heat release rate, ignition time
Citation: Zhao L, Cao D, Chen Y, Liu H, You T, Li C and Zhang H (2023) Numerical simulation study on suppression effect of water mist on PMMA combustion under external radiant heat flux. Front. Mater. 10:1110610. doi: 10.3389/fmats.2023.1110610
Received: 29 November 2022; Accepted: 09 January 2023;
Published: 17 January 2023.
Edited by:
Ying Zhang, Wuhan University of Technology, ChinaReviewed by:
Weiguang An, China University of Mining and Technology, ChinaChangfa Tao, Hefei University of Technology, China
Copyright © 2023 Zhao, Cao, Chen, Liu, You, Li and Zhang. This is an open-access article distributed under the terms of the Creative Commons Attribution License (CC BY). The use, distribution or reproduction in other forums is permitted, provided the original author(s) and the copyright owner(s) are credited and that the original publication in this journal is cited, in accordance with accepted academic practice. No use, distribution or reproduction is permitted which does not comply with these terms.
*Correspondence: Hongming Zhang, emhhbmdobUBqb3UuZWR1LmNu