- 1Key Laboratory for Magnetism and Magnetic Materials of the Ministry of Education, School of Physical Science and Technology, Lanzhou University, Lanzhou, China
- 2Hubei Engineering and Technology Research Center for Functional Fiber Fabrication and Testing, Wuhan Textile University, Wuhan, China
Introduction: Although electric field mediated the magnetic anisotropy in ferromagnetic/ferroelectric structure have an intense report, the angle between the magnetic uniaxial anisotropy and strain anisotropy influencing the rearrangement of the magnetic moment has not well investigated.
Methods: Keithley 2410 direct current power supply was used to provide voltage through the Cu wires. Static magnetic properties of CoZr layer were measured through VSM (MicroSense EV9). Dynamic magnetic properties were obtained by FMR (JEOL JES-FA 300 spectrometer, power of 1-mW, X-band at 8.969 GHz).
Results and Discussion: Electric field-mediated, room-temperature magnetic anisotropy of CoZr/Pb(Mg1/3Nb2/3)O3–PbTiO3 (PMN–PT) structures, in which easy axis of CoZr layer along either direction of [01-1] or [100] of PMN–PT, was investigated. Measured with vibrating sample magnetometer, for [01-1] easy-axis direction sample, when an electric field was applied, these directions of easy axis and hard axis remains unchanged. However, for [100] easy-axis direction sample, these directions of easy axis and hard axis were changed obviously with applying electric field, attributable to the competition between magnetic uniaxial anisotropy of CoZr layer and piezostrain anisotropy of PMN-PT substrate. Nevertheless, change of the resonance magnetic field with electric field–measured by ferromagnetic resonance–exhibited non-volatile behavior, which possibly indicates magnon-driven magnetoelectric coupling existing in CoZr/PMN–PT structures.
1 Introduction
Advancements in ferromagnetic materials, either single-phase (Zhao et al., 2006; Chu et al., 2008; Lebeugle et al., 2008; Catalan and Scott, 2009) or heterostructures (Murugavel et al., 2004; Zurbuchen et al., 2005; He et al., 2007), have promising practical applications in magnetoelectric devices. Multiferroic heterostructures which exhibit strong coupled magnetization and electric polarization across the interfaces, especially ferromagnetic/ferroelectric (FM/FE) structures (Liu et al., 2013; Cui et al., 2014; Li et al., 2014; Yang S. W. et al., 2014), have stimulated much research. Magnetic properties (mediated by electric field) and ferroelectricity (mediated by magnetic field) can be realized by magnetoelectric (ME) coupling. Recently, with the development of ME random–access memory (which can substantially improve storage density), research has focused on how to exploit electric field-mediated the magnetic properties (Liu et al., 2013; Yang S. W. et al., 2014). The common ferroelectric crystal material PMN-PT (Wu et al., 2011; Yang L. F. et al., 2014) exhibits a d33 parameter that is much higher than the d31 parameter, consequently, this material is often used in FM/FE structures. When applying electric field on the PMN–PT layer, strong strain or charge effect at the interface of FM and PMN-PT layers can mediate magnetic properties of the adjacent FM layer. Because of strain that is caused by the piezo-electric and electrostrictive effects (attributable to applied electric field) in the FE material (Thiele et al., 2007; Pertsev, 2008; Wang et al., 2014; Zhou et al., 2021), large magnetic response was produced in FM layer, which can lead to a strong ME coupling. Thus, one always observes butterfly-type behavior. The charge accumulation/dissipation is relevant to the spin carriers, which is induced in the interfacial FM/FE layer (Weisheit et al., 2007; Molegraaf et al., 2009). These above two main mechanisms form an important basis for ME coupling in FM/FE structure to mediate magnetic anisotropy. Hu and Nan (2009) reported the influence of the residual strains or modes of applied electric fields on the magnetic easy axis for various FM films (such as Fe, Ni, Fe3O4 and CoFe2O4) grown on FE substrates (such as PZN-PT, BTO, and PZT). Wang et al. (2014) used an electric field to mediate the magnetic anisotropy of Co/PMN–PT heterostructure in which easy axis of Co. thin film was along [01-1] direction of PMN–PT substrate. The angle between the magnetic uniaxial anisotropy and strain anisotropies can influence the rearrangement of the magnetic moment. Therefore, in this report, at room-temperature, non-volatile electric field-mediated magnetic anisotropy of CoZr/PMN–PT heterostructures, in which the easy axis of CoZr layer was along either [01-1] or [100] direction of PMN–PT, was investigated. For the [01-1] easy-axis sample, when an electric field was applied, these directions of easy axis and hard axis remains unchanged, which was measured by vibrating sample magnetometer (VSM). However, for [100] easy-axis direction sample, these directions of easy axis and hard axis were changed obviously with applying electric field, attributable to the competition between magnetic uniaxial anisotropy of CoZr layer and piezostrain anisotropy of PMN-PT substrate. In addition, change of resonance magnetic field (Hr) under various electric fields measured by ferromagnetic resonance (FMR) exhibited non-volatile behavior, which possibly indicates magnon-driven magnetoelectric coupling in CoZr/PMN–PT heterostructures.
2 Materials and methods
The 40 nm-thick CoZr thin films were deposited on (011)-oriented PMN-PT substrate by magnetron sputtering. The oblique sputtering angle is 20°. Six Zr chips were regularly placed onto a Co. target. The easy axes of CoZr thin film were along the [01-1] or [100] direction (sample 1 and sample 2, respectively) of PMN–PT substrate during sputtering, respectively. 20 nm-thick Pt layer was sputtered on the top surface, and 100 nm-thick Pt layer was prepared on the bottom side, which for use as top electrode and bottom electrode, respectively. Two Cu wires were connected to them, respectively. Keithley 2410 direct current power supply was used to provide voltage through the Cu wires. Static magnetic properties of CoZr layer were measured through VSM (MicroSense EV9). Dynamic magnetic properties were obtained by FMR (JEOL JES-FA 300 spectrometer, power of 1-mW, X-band at 8.969 GHz).
3 Results and discussion
3.1 Electric field control of static magnetic property
For the sample 1, the inset of Figure 1A shows typical in-plane magnetic hysteresis loops of CoZr layer along easy axis and hard axis at unpoled (P0) state, measured with the VSM. There was obvious in-plane uniaxial anisotropy, attributable to 20° oblique sputtering angle. The easy axis of CoZr layer is along [01-1] direction of PMN-PT substrate at P0 state (Figure 2A), regarded as 0°. The hard axis is along y direction, which can be defined as 90°. Then, the sample1 was pre-polarized by an applied electric field of ±10 kV/cm for two cycles. For brevity, only data at the hard axis was plotted. The electric field applied from top side to bottom side for sample 1 was defined as positive (+), whereas negative (−) (Figure 2A). The maximum value of electric field applied on the sample 1 was 10 kV/cm; Figure 1A shows representative magnetic hysteresis loops, measured at 90° at the Pr+ state and ±10 kV/cm. Pr+ state referred to the remnant polarization state after applied electric field applied decreasing from 10 kV/cm to 0 kV/cm. When the electric field was changed from 10 kV/cm to −10 kV/cm, saturation magnetic field decreased, which indicates a decrease of the magnetic anisotropy. That is, magnetic anisotropy of sample 1 can be mediated by the electric field. In addition, rotating of the angle-remanent curves was measured at Pr+ state and −10 kV/cm. Remanent magnetization (Mr) state was achieved by reducing applied magnetic field 1000 Oe to 0 Oe. Mr was measured in the plane of every 5° by attaching sample 1 to rotatable holder of VSM. Figure 1B showed angle-dependence of Mr at Pr+ state and −10 kV/cm. Here, these curves indicated a two-fold symmetric magnetic uniaxial anisotropy. Maximum and minimum values of Mr at 0° and 90°, respectively was obtained. When applying different electric fields, value of Mr changed, while the direction of hard axis remained unchanged. However, for sample 2 (Figure 1D), the direction of hard axis generated an obvious 30° change. Noticeably, the direction of the 0° still represent the direction of easy axis for sample 2, which is along the [100] of PMN-PT substrate (Figure 2B). Concomitantly, the relative remanent magnetization Mr/Ms at the hard axis exhibited an increase of 0.3. Figure 1C shows representative magnetic hysteresis loops measured at 90° under various electric fields, which indicates similar behavior with the rotating-angle result (Figure 1D).
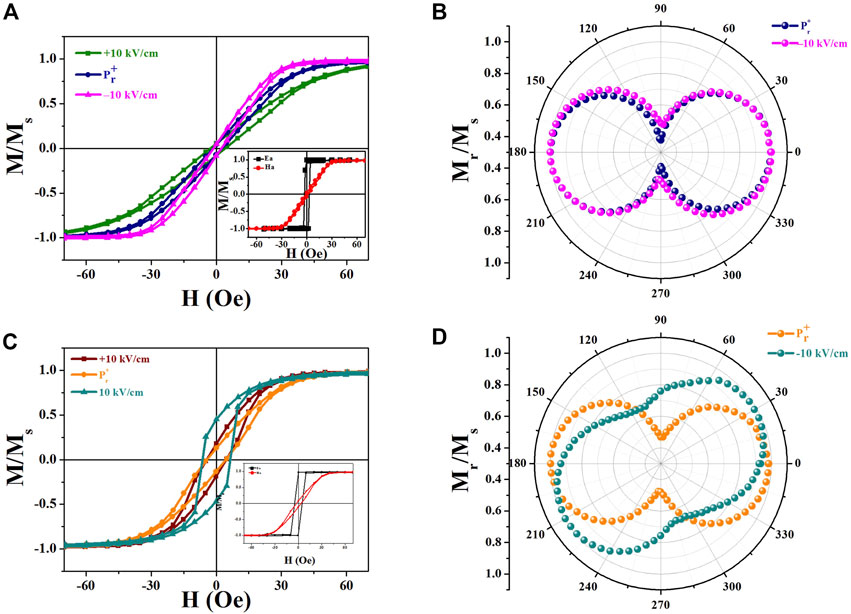
FIGURE 1. Magnetic hysteresis loops at the hard axis under various electric fields for the CoZr layer along (A) (01–1) and (C) (100) of the PMN–PT substrate; rotating angle-remanent curves under various electric fields for the CoZr layer along (B) (01–1) and (D) (100) of the PMN–PT substrate. Inset: magnetic hysteresis loops at the easy and hard axes at the P0 state.
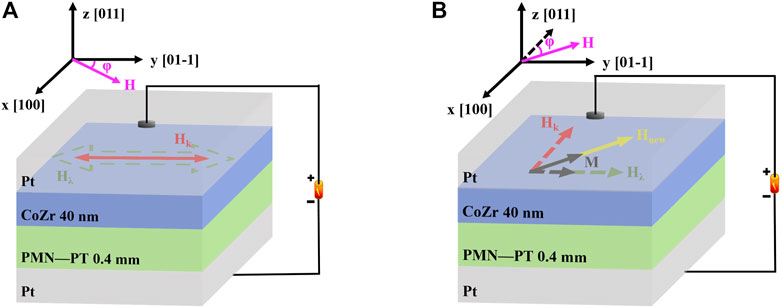
FIGURE 2. Schematic of magnetic uniaxial and piezostrain anisotropy for the CoZr layer along (A) (01–1) and (B) (100)of the PMN–PT substrate. φ is the angle of the applied magnetic field with respect to the direction of easy axis at P0 state.
3.2 Schematic of heterostructures
The aforementioned data can be explained as follows. For sample 1, the easy axis of CoZr was parallel to (01–1) direction of PMN–PT substrate, which indicates that direction of magnetic uniaxial anisotropy Hk was with that of easy axis of the piezostrain Hλ (originating from PMN–PT substrate) (Figure 2A). Thus, the direction of easy/hard axis can negligibly shift under various electric fields. Nevertheless, for sample 2, easy axis of CoZr was parallel to the [100] direction of PMN–PT substrate, which indicates that there was an angle between direction of magnetic uniaxial anisotropy and that of easy axis of piezostrain from the PMN–PT substrate (Figure 2B). When applying the various electric fields, there was a competition between Hk and Hλ, which can lead to divergence of the magnetic moment (M) from original easy axis and rearrangement along new direction of magnetic anisotropy field. In addition, Hλ increased with increasing the applied electric field. Upon obtaining a sufficiently large Hλ, the magnetic moment arranged along the direction of Hλ. This is the reason that the direction of the easy axis generated an obvious 30° change in sample 2.
3.3 Electric field control of dynamic magnetic property
Next, the dynamic magnetic properties of two samples mediated by electric field can be investigated at room temperature, which was measured by FMR. Figure 3A shows the configuration for FMR spectroscopy. The sample dimensions were 1 mm × 1 mm. For the sample 1, at P0 state, FMR integral spectra were obtained with angle φ (between [01-1] direction of PMN–PT substrate and that of applied magnetic field) rotating from 0° to 90° (Figure 3B). Considering the precession of magnetization was stimulated by microwave excitation with special phase coupling, the spectra contained real part and imaginary part, which was a mixture of them. Therefore, the FMR function as follows (Zhou et al., 2017):
where
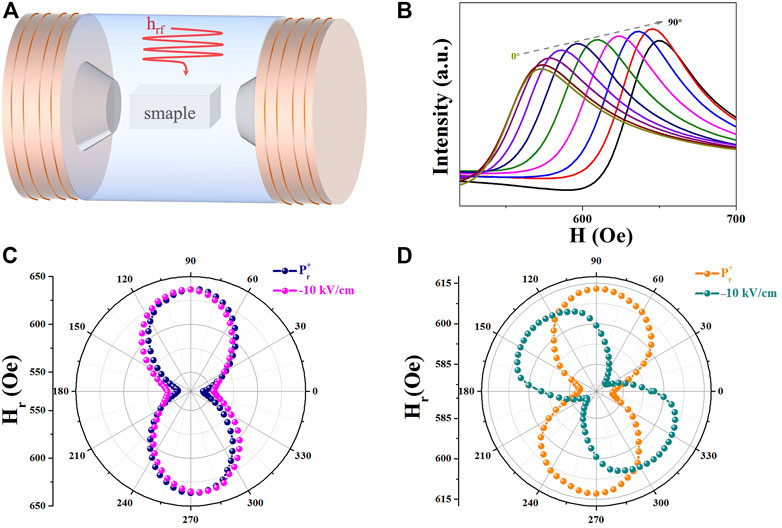
FIGURE 3. (A) Configuration for FMR spectroscopy; (B) in-plane rotating-angle FMR integral spectra for the CoZr layer along the (01–1) PMN–PT substrate at the P0 state; Hr−φ curve under various electric fields for the CoZr layer along (C) (01–1) and (D) (100) of the PMN–PT substrate.
FMR integral curves under different angles φ can be fit on the basis of Eq. 1. These related parameters such as Hr can be obtained. Further, Hr−φ curve at Pr+ state and −10 kV/cm was shown in Figure 3C, which demonstrates an obvious in-plane uniaxial anisotropy of CoZr layer originating from oblique magnetron. The position of minimal value of Hr was at 0°, indicating easy axis along 0°. The maximal value of Hr was at 90°, which represented hard axis along 90°. The directions of easy axis and hard axis remained unchanged for sample 1 even applying various electric field, which is consistent with the result measured by VSM as shown in (Figure 1B). Nevertheless, for sample 2, the direction of the easy axis generated an obvious 45° change, compared with the data at Pr+ state and −10 kV/cm as shown in (Figure 3D). The results measured by FMR has a difference with the results obtained with the VSM, attributable to the two points: 1) the direction of in-plane magnetic uniaxial anisotropy is inconsistent with sample design’s direction as shown in Figure 2 due to the CoZr thin film without a single crystal structure. Additionally, due to the ferroelectric domain distributing in PMN-PT substrate with applying electric field, the direction of piezostrain anisotropy stemming from PMN-PT substrate is not necessarily consistent with that in a real state. 2) The another ME coupling mechanism excepting for piezostrain effect can be considered in CoZr/PMN-PT structure.
3.4 Mechanism of electric field control of magnetic property
Additionally, these measured FMR integral curves under various electric fields can also be fit on the basis of Eq. 1. For sample 1, Hr−E curve at 90° can be obtained as shown in (Figure 4A), which reveals non-volatile electric field tunning of magnetic behavior. Moreover, the results for sample 2 (Figure 4B) were similar to those in Figure 4A. Generally, two main ME coupling mechanisms, piezostrain effect and charge effect, exist in FM/FE structure. The S−E curve for the two samples as shown in Figure 4C indicates piezostrain effect stemming from PMN-PT substrate with applying electric field can transfer to CoZr layer in a manner that resulting in a butterfly-like magnetic response. In comparison with FMR results in Figures 4A,B, loop-like Hr−E curves indicated non-volatile behavior, different from that of butterfly-like S−E curve. The above result indicated that another ME coupling mechanism must be into consideration. However, the magnitude of charge screening length for conventional charge effect was on the order of angstrom for typical FM film according to previous reports on MgO-based magnetic tunnel junctions and FeNi/PMN-PT multiferroic heterostructures (Shiota et al., 2012; Wang et al., 2012). Therefore, the transposition of the ME coupling mechanism should be considered with care. But in fact, 40 nm-thick CoZr layer was prepared in our CoZr/PMN–PT structure. This thickness was much larger than charge screening length, and thus conventional charge effect can be ignored. In the present study (Jia et al., 2014), we have showed that the spin-polarized screening charge is surface confined, in the spin channel a local non-uniform spiral spin density builds up at the interface and goes over into the initial FM magnetization within the spin-diffusion length of the order of tens of nanometers. The interfacial spiral spin density can be viewed as a magnonic accumulation stabilized by the interfacial, spin-dependent charge rearrangement at the contact region between the FM and the FE (with the electric polarization P). Meanwhile, we have reported this direct ME coupling can pass into the initial FM magnetization and steer the magnetic dynamics in single crystal Fe thin film with thicknesses of 4, 15, and 30 nm (Zhou et al., 2018a) and single crystal Co. thin film of 14 nm (Zhou et al., 2018b). That is, the diffusion length of magnon-driven interfacial ME coupling (belong to charge effect) can reach magnitude of nanometers (Zhou et al., 2019). Furthermore, strong correlation between magnetization M and polarization P can be received in the magnon-driven interfacial ME coupling (Figure 4D), which lead to non-volatile magnetic response such as loop-like Hr−E curve as shown in Figures 4A, B. Therefore, piezostrain effect and magnon-driven ME coupling belonging to charge effect coexist in our CoZr/PMN–PT structure.
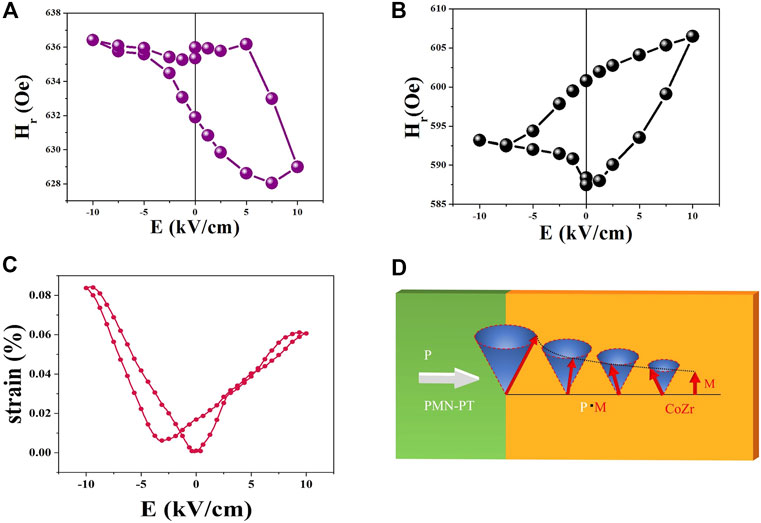
FIGURE 4. Hr−E curve at the hard axis under various electric fields for the CoZr layer along (A) (01–1) and (B) (100) of the PMN–PT substrate; (C) S–E curve; (D) schematic of P–M interaction
4 Conclusions
We reported electric-field mediated magnetic anisotropy in CoZr/PMN–PT heterostructures, in which the easy axis of CoZr layer was along (100) and (01-1) directions of PMN–PT substrate, respectively. For [01-1] easy-axis sample, electric-field-induced the direction of the easy axis to remain in almost the same position. However, for the (001) easy-axis sample, the applied electric field changed the direction of the easy axis and hard axis, attributable to competition between the magnetic uniaxial anisotropy and piezostrain anisotropy. Additionally, change of resonance magnetic field with electric field measured by ferromagnetic resonance exhibited non-volatile behavior, which possibly indicates magnon-driven magnetoelectric coupling in CoZr/PMN–PT heterostructures.
Data availability statement
The original contributions presented in the study are included in the article/Supplementary Material, further inquiries can be directed to the corresponding authors.
Author contributions
CZ and CJ conceptualized this work. ZW, FL fabricated the samples, performed the VSM and FMR measurements and collected experimental data. ZW and DZ wrote the paper, and all authors conducted data analysis.
Funding
The National Natural Science Foundation of China (No. 51901163) supported by CZ, and the Natural Science Foundation of Gansu Province (Grant No. 21JR7RA472) supported by CJ. This work was funded from the National Natural Science Foundation of China (No. 51901163) and the Natural Science Foundation of Gansu Province (Grant No. 21JR7RA472).
Conflict of interest
The authors declare that the research was conducted in the absence of any commercial or financial relationships that could be construed as a potential conflict of interest.
Publisher’s note
All claims expressed in this article are solely those of the authors and do not necessarily represent those of their affiliated organizations, or those of the publisher, the editors and the reviewers. Any product that may be evaluated in this article, or claim that may be made by its manufacturer, is not guaranteed or endorsed by the publisher.
References
Catalan, G., and Scott, J. F. (2009). Physics and applications of bismuth ferrite. Adv. Mater. 21, 2463–2485. doi:10.1002/adma.200802849
Chu, Y. H., Martin, L. W., Holcomb, M. B., Gajek, M., Han, S. –J., He, Q., et al. (2008). Electric-field control of local ferromagnetism using a magnetoelectric multiferroic. Nat. Mater. 7, 478–482. doi:10.1038/nmat2184
Cui, B., Song, C., Sun, Y., Wang, Y. Y., Zhao, Y. L., Li, F., et al. (2014). Exchange bias field induced symmetry-breaking of magnetization rotation in two-dimension. Appl. Phys. Lett. 105, 152402. doi:10.1063/1.4898350
He, H. C., Wang, J., Zhou, J. P., and Nan, C. M. (2007). Ferroelectric and ferromagnetic behavior of Pb(Zr0.52Ti0.48)O3-Co0.9Zn0.1Fe2O4 multilayered thin films prepared via solution processing. Adv. Funct. Mater. 17, 1333–1338. doi:10.1002/adfm.200600476
Hu, J. M., and Nan, C. W. (2009). Electric-field-induced magnetic easy-axis reorientation in ferromagnetic/ferroelectric layered heterostructures. Phys. Rev. B 80, 224416. doi:10.1103/physrevb.80.224416
Jia, C. L., Wei, T. L., Jiang, C. J., Xue, D. S., Sukhov, A., and Berakdar, J. (2014). Mechanism of interfacial magnetoelectric coupling in composite multiferroics. Phys. Rev. B 90, 054423. doi:10.1103/physrevb.90.054423
Lebeugle, D., Colson, D., Forget, A., Viret, M., Bataille, A. M., and Gukasov, A. (2008). Electric-field-induced spin flop inBiFeO3Single crystals at room temperature. Phys. Rev. Lett. 100, 227602. doi:10.1103/physrevlett.100.227602
Li, P. S., Chen, A. T., Li, D. L., Zhao, Y. G., Zhang, S., Yang, L. F., et al. (2014). Electric field manipulation of magnetization rotation and tunneling magnetoresistance of magnetic tunnel junctions at room temperature. Adv. Mater. 26, 4320–4325. doi:10.1002/adma.201400617
Liu, M., Howe, B. M., Grazulis, L., Mahalingam, K., Nan, T. X., Sun, N. X., et al. (2013). Voltage-impulse-induced non-volatile ferroelastic switching of ferromagnetic resonance for reconfigurable magnetoelectric microwave devices. Adv. Mater. 25, 4886–4892. doi:10.1002/adma.201301989
Molegraaf, H. J. A., Hoffman, J., Vaz, C. A. F., Gariglio, S., van der Marel, D., Ahn, C. H., et al. (2009). Magnetoelectric effects in complex oxides with competing ground states. J. M. Triscone. Adv. Mater. 21, 3470–3474. doi:10.1002/adma.200900278
Murugavel, P., Padhan, P., and Prellier, W. (2004). Enhanced magnetoresistance in ferromagnetic Pr0.85Ca0.15MnO3∕ferroelectric Ba0.6Sr0.4TiO3 superlattice films. Appl. Phys. Lett. 85, 4992–4994. doi:10.1063/1.1825075
Pertsev, N. A. (2008). Giant magnetoelectric effect via strain-induced spin reorientation transitions in ferromagnetic films. Phys. Rev. B 78, 212102. doi:10.1103/physrevb.78.212102
Shiota, Y., Nozaki, T., Bonell, F., Murakami, S., Shinjo, T., and Suzuki, Y. (2012). Induction of coherent magnetization switching in a few atomic layers of FeCo using voltage pulses. Nat. Mater 11, 39–43. doi:10.1038/nmat3172
Thiele, C., Dörr, K., Bilani, O., Rödel, J., and Schultz, L. (2007). Influence of strain on the magnetization and magnetoelectric effect in La0.7A0.3MnO3/PMN−PT(001) (A=Sr,Ca). Phys. Rev. B 75, 054408. doi:10.1103/PhysRevB.75.054408
Wang, F. L., Zhou, C., Zhang, C., Dong, C. H., Yang, C. C., Jiang, C. J., et al. (2014). Piezoelectric control of magnetic dynamics in Co/Pb(Mg1/3Nb2/3)O3-PbTiO3 heterostructure. Appl. Phys. Lett. 105, 062407. doi:10.1063/1.4893142
Wang, W. G., Li, M. G., Hageman, S., and Chien, C. L. (2012). Electric-field-assisted switching in magnetic tunnel junctions. Nat. Mater 11, 64–68. doi:10.1038/nmat3171
Weisheit, M., Fahler, S., Marty, A., Souche, Y., Poinsignon, C., and Givord, D. (2007). Electric field-induced modification of magnetism in thin-film ferromagnets. Science 315, 349–351. doi:10.1126/science.1136629
Wu, T., Bur, A., Zhao, P., Mohanchandra, K. P., Wong, K., Wang, K. L., et al. (2011). Giant electric-field-induced reversible and permanent magnetization reorientation on magnetoelectric Ni/(011) [Pb(Mg1/3Nb2/3)O3](1−x)–[PbTiO3]x heterostructure. Appl. Phys. Lett. 98, 012504. doi:10.1063/1.3534788
Yang, L. F., Zhao, Y. G., Gao, Y., Yang, Y. J., Huang, H. L., Miao, P. X., et al. (2014). Bipolar loop-like non-volatile strain in the (001)-oriented Pb(Mg1/3Nb2/3)O3-PbTiO3 single crystals. Sci. Rep. 4, 4591. doi:10.1038/srep04591
Yang, S. W., Peng, R. C., Jiang, T., Liu, Y. K., Feng, L., Wang, J. J., et al. (2014). Non-volatile 180° magnetization reversal by an electric field in multiferroic heterostructures. Adv. Mater. 26, 7091–7095. doi:10.1002/adma.201402774
Zhao, T., Scholl, A., Zavaliche, F., Lee, K., Barry, M., Doran, A., et al. (2006). Electrical control of antiferromagnetic domains in multiferroic BiFeO3 films at room temperature. Nat. Mater. 5, 823–829. doi:10.1038/nmat1731
Zhou, C., Dunzhu, G. S., Yao, J. L., and Jiang, C. J. (2017). Piezostrain control of magnetic anisotropy in Co2FeAl/Pb(Mg1/3Nb2/3)O3-30%PbTiO3 heterostructure. J. Alloy. Compd. 710, 680–684. doi:10.1016/j.jallcom.2017.03.310
Zhou, C., Shen, L. K., Liu, M., Gao, C. X., Jia, C. L., Jiang, C. J., et al. (2018a). Long-range nonvolatile electric field effect in epitaxial Fe/Pb(Mg1/3Nb2/3)0.7Ti0.3O3 heterostructures. Adv. Funct. Mater. 28, 1707027. doi:10.1002/adfm.201707027
Zhou, C., Shen, L., Liu, M., Gao, C. X., Jia, C. L., and Jiang, C. J. (2018b). Strong nonvolatile magnon-driven magnetoelectric coupling in single-crystal Co/[PbMg1/3Nb2/3O3]0.71[PbTiO3]0.29 heterostructures. Phys. Rev. Appl. 9, 014006. doi:10.1103/PhysRevApplied.9.014006
Zhou, C., Zhang, M. F., Feng, C. F., Xu, M. Y., Wang, S. X., and Jiang, C. J. (2019). Magnon-driven interfacial magnetoelectric coupling in Co/PMN-PT multiferroic heterostructures. Phys. Chem. Chem. Phys. 21, 21438–21444. doi:10.1039/c9cp04169a
Zhou, C., Zhu, D. Y., Liu, F. F., Feng, C. F., Zhang, M. F., Ding, L., et al. (2021). Electric-field-induced in-plane effective 90° magnetization rotation in Co2FeAl/PMN-PT structure. Chin. Phys. B 30, 057504. doi:10.1088/1674-1056/abd7de
Keywords: non-volatile, CoZr/PMN-PT heterostructure, pie-zostrain effect, magnon-driven magnetoelectric coupling, magnetic anisotropy
Citation: Wang Z, Zhu D, Liu F, Zhou C and Jiang C (2023) Non-volatile electric field-mediated magnetic anisotropy in CoZr/ PMN-PT structure. Front. Mater. 10:1083794. doi: 10.3389/fmats.2023.1083794
Received: 29 October 2022; Accepted: 12 January 2023;
Published: 25 January 2023.
Edited by:
Lvkang Shen, Xi’an Jiaotong University, ChinaReviewed by:
Shuai Zhang, Huazhong University of Science and Technology, ChinaJinwei Rao, ShanghaiTech University, China
Lulu Pan, Institute of Physics (CAS), China
Copyright © 2023 Wang, Zhu, Liu, Zhou and Jiang. This is an open-access article distributed under the terms of the Creative Commons Attribution License (CC BY). The use, distribution or reproduction in other forums is permitted, provided the original author(s) and the copyright owner(s) are credited and that the original publication in this journal is cited, in accordance with accepted academic practice. No use, distribution or reproduction is permitted which does not comply with these terms.
*Correspondence: Cai Zhou, c3pob3VAd3R1LmVkdS5jbg==; Changjun Jiang, amlhbmdjaGpAbHp1LmVkdS5jbg==