- 1Yellow River Laboratory, Zhengzhou University, Zhengzhou, China
- 2State Key Laboratory of Hydroscience and Engineering, Tsinghua University, Beijing, China
- 3School of Civil Engineering and Architecture, Zhengzhou University of Aeronautics, Zhengzhou, China
The service performance of concrete structures in a water environment differs from that in a normal environment. An accurate evaluation of the mechanical properties and service status of wet concrete is related to the reliable design and safe operation of concrete structures, e.g., hydraulics, marine engineering, bridges, and tunnels. To promote the application of new and high-performance concrete to complex water environments and grasp the future development trend, the research progress on the service performance of concrete in water environments was reviewed worldwide. Starting from the internal water content of concrete, the existing research is combed, the influence of water content, water pressure, and loading rate on the static and dynamic characteristics of concrete in a water environment is summarized, and the influence mechanism is analyzed. The literature review demonstrates that the static compressive strength of wet concrete is lower than that of dry concrete; however, the elastic modulus improves. With an increase in the strain rate, the compressive strength of wet and dry concretes improves, and the rate sensitivity of the wet concrete is greater than that of the normal concrete. Pore structure characteristics mainly affect the static strength of the wet concrete. The improvement in the dynamic strength of the wet concrete is caused by the combined effect of the concrete rate sensitivity, “Stefan” effect, and water pressure.
1 Introduction
Concrete, one of the most widely used building materials, is a typical porous medium and is regarded as a multiphase composite material (Ulrik and MONTEIRO, 1993; Du and LI, 2012). Its internal pore structure exhibits the characteristics of large morphological differences, disorderly distribution, and a large aperture size covering range, which significantly affects its strength, deformation, and durability (Jin et al., 2005).
The process of concrete structure from pouring to service often occurs in different water environments, e.g., moisture in concrete mixing, wet air from water vapor recirculation (Li et al., 2022), and macro water environments in rivers and lakes. When the concrete has long been in a water environment, water penetrates the concrete through the pore structure, which causes the concrete to be under different humidity states. Studies have demonstrated that (Alexandridis and Gardner, 1981; De Schutter and Taerwe, 1996; Wu et al., 2012; Josué César Bastos et al., 2022) the humidity state affects the mechanical properties of concrete. Simultaneously, the mechanical performance of concrete under static and dynamic loads is significantly different (Bischoff and Perry, 1991; Fu et al., 1991; Javier and MALVAR, 1998), and the water environment makes this difference more complex (Li and WANG, 2006). An extensive study has been conducted on these problems.
Starting from the influence of water environment on the mechanical properties of concrete, this study investigated the mechanical properties change of concrete after water treatment, the law of internal water content change, and the mechanism of pore water on the mechanical properties of concrete and other related studies, and analyzed the potential relationship between water environment and concrete internal water content, water content and concrete mechanical properties. Thus, this paper is narrated from the following aspects: 1) the change rule of the internal water content of concrete in a water environment and its influence on mechanical properties; 2) the effect of pore water on the static and dynamic characteristics of concrete; 3) the influence mechanism of the concrete pore structure, internal water quantity characteristics, and pore water on concrete performance. Furthermore, this study proposes the prospect of a possible development direction in this field.
2 Internal water content and its effects on mechanical properties of concrete
2.1 Water content of concrete in water environment
The internal water content of concrete reflects its humidity state; therefore, the internal water content of concrete and its variation law have been considered as bases for studying the mechanical effect of pore water. Several studies (Li, 2009; Li, 2014; Wang, 2017; Zhang, 2017; Zhang et al., 2019) investigated the change rule of the concrete water content with soaking time in an unconfined water environment. A summary of this is shown in Figure 1. At the beginning of immersion (within 5 h), the water content increased linearly and rapidly and then increased nonlinearly to near saturation. Finally, after a long period of slight growth, it remained constant, which can be regarded as “reaching saturation.” Wang et al. (Wang et al., 2017) studied the variation in the water content of underwater concrete under pressure. The water pressure at all the levels (1, 2, 5, and 10 MPa) still conforms to the aforementioned law, and the greater the water pressure, the greater the saturated water content and the shorter the saturation time are. However, in the saturated state, when the water pressure increased from 2 MPa to 5 MPa, the water content increased by 0.77%. When the water pressure increased from 5 MPa to 10 MPa, the water content increased by nearly 0.1%. Finally, when the water pressure was unloaded, the water content decreased gradually (Wang et al., 2017). This indicates that even in the saturated concrete, the pores contain gas, which hinders the infiltration of the pressurized water. When the water pressure was unloaded, the gas pressure discharged the water and returned it to its natural saturated state.
2.2 Influence of water content on the elastic modulus of concrete
Water can be regarded as incompressible relative to air. The pore water in concrete exhibits a greater elastic modulus than air; therefore, the elastic modulus increases with an increase in the water content under the same porosity condition (Nevillea, 1973; Rossi et al., 1992; Mehta and Monteiro, 2014; Zheng and Li, 2011). Liu et al. (Zhu et al., 2012; Liu et al., 2014; Liu et al., 2020) conducted experiments on the influence of the water content on the elastic modulus of concrete. The experimental results (normalization, as shown in Figure 2) support the following conclusion: the elastic modulus approximately and linearly increases with an increase in the water content.
Considering concrete as a multiphase composite material, several researchers (Kuster and Toksöz, 1974; Qiu and Weng, 1990; Yaman, 2002; Yaman et al., 2002; Wang and LI, 2005; Zheng and Li, 2011) have applied the equivalent inclusion theory of mesomechanics (equivalent representative body, as shown in Figure 3), considering the different factors discussed in the calculation formula of the concrete elastic modulus.
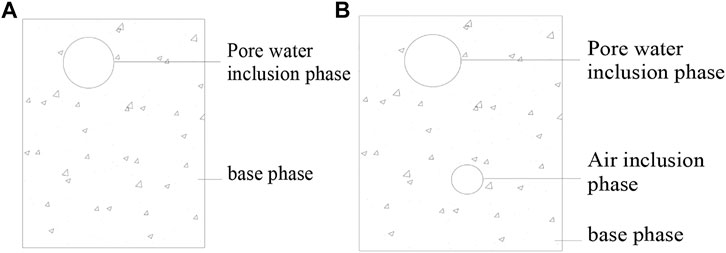
FIGURE 3. Equivalent representative body of concrete (A) single-phase inclusion; (B) dual-phase inclusion.
According to elasticity mechanics, the elastic modulus of concrete is defined as
where K and G are the volume modulus and shear modulus of the concrete matrix, respectively, Gm is the bulk modulus and shear modulus of the matrix without pores, c is the volume fraction of inclusions, and benchmarks i and m represent the inclusion and matrix phases, respectively.
Yama et al. (Yaman, 2002; Yaman et al., 2002) classified pores into active and inactive based on the connection of concrete pores with the outside world and assumed that pore water cannot penetrate inactive pores and environmental humidity affects the elastic modulus of concrete by affecting active pores. By comparing existing calculation models, it was observed that the “Kuster–Toksoz” model (Kuster and Toksöz, 1974) could effectively predict the elastic modulus of wet concrete. However, the model did not consider the influence of pore water on the shear modulus of concrete and the further hydration of cement, resulting in a large deviation between the calculated results and experimental data. Zheng et al. (Zheng and Li, 2011) and Wang et al. (Wang and LI, 2005) considered the deformation of pores, microcracks, pore water viscosity, and further hydration of cement. The modified model exhibits good applicability to the experimental data (Yaman et al., 2002). However, the aforementioned studies considered concrete as the inclusion of the matrix phase and saturated pore water phase, which is not suitable for wet concrete.
Based on the Mori–Tanaka theory (Qiu and Weng, 1990), Wang et al. (Wang and Li, 2007) considered the effects of water viscosity, late hydration of cement, and pore geometry on the elastic modulus of wet concrete. They used the modified differential decomposition method of mesomechanics to decompose unsaturated concrete into saturated concrete equivalent medium and dry pores and assumed that the elastic properties of the unsaturated concrete depended on the water content, pore geometry, and elasticity of the matrix concrete. Bai et al. (Bai et al., 2010) regarded concrete as the inclusion of the matrix, air, and pore water phases, and these two modified models can effectively predict the elastic modulus of wet concrete. A comparison of the theoretical models above, as shown in Table 1, indicates that a model with more inclusions has a greater scope of application.
2.3 Influence of the water content on the compressive strength of concrete
Researchers (Cadoni et al., 2001; Yan et al., 2005; Wang and LI, 2006a; Wang and LI, 2007a) conducted the strength test of concrete with different water contents, and the results indicated that wet concrete exhibited higher compressive strength than dry concrete. Wang et al. (Wang and LI, 2006a; Wang and LI, 2007a; Wang and LI, 2007b) compared the strength of saturated and dry concrete, and the results indicated that the compressive strength of the saturated concrete decreased by 4.5%, and the splitting tensile strength decreased by 11.41% compared with that of the dry concrete. Li et al. (Li, 2004) studied the influence of different water–cement ratios and soaking times on the strength of dry concrete and obtained similar results. With an increase in soaking time, the compressive strength of the concrete decreased, and the influence of humidity on the concrete with a high water–cement ratio was evident. A few researchers (Reinhardt et al., 1990; Ross and DAVID, 1996; DavidHARRIS CEM, 2000) conducted tests on the saturation of concrete specimens, and the results indicated that the strength of concrete decreased with an increase in saturation.
Li et al. and Zhang et al. (Li, 2009; Liu et al., 2011; Li, 2014; Zhang et al., 2019) studied the change rule of the water content of dry concrete after immersion and its influence on the compressive strength. The normalized results are illustrated in Figure 4. Although the data were discrete, the compressive strength decreased with an increase in saturation. These studies regarded water content as the main influencing factor, ignoring the influence of internal porosity on strength. Existing studies (Lian and Zhuge, 2010; Lian et al., 2011; Yu et al., 2015; Zhou et al., 2015) demonstrate that porosity adversely affects the compressive strength and elastic modulus of concrete. Therefore, under the same mix proportion and material conditions, the higher the saturation of concrete, the stronger the water absorption capacity of the concrete is, and the greater the internal porosity, the lower the strength is.
Moreover, the water content affects the fracture performance of concrete. Deng et al. (Deng et al., 2014) studied variations in the fracture properties of concrete immersed in water. It was observed that the fracture toughness decreased with an increase in the immersion time, and the decrease trend was first increased and then decreased. Wittmann et al. and Zhang et al. (Wittmann et al., 2007; Zhang et al., 2010) observed that completely dry concrete exhibited higher fracture energy, and the fracture energy of the concrete in the dry state was 1.18 and 1.57 times the saturation of 75% and 100%, respectively. Similar results were obtained by Ross et al. (Rossi and Boulay, 1990; Rossi, 1994; Zhang et al., 2016).
3 Effect of pore water on the mechanical properties of concrete
When the concrete structure is at a certain depth in the water environment, it is subjected to water pressure that cannot be ignored. Simultaneously, different pore water pressures were generated at different surface depths of the structure. The pore water pressure inside the concrete surface is the same as the environmental water pressure (Bažant and Najjar, 1972). Compared with the study of concrete performance in a non-pressurized water environment, the pressurized water environment is closer to engineering practice.
3.1 Effect of pore water on the static strength of concrete
Researchers have conducted studies on the static strength of concrete under different water pressures, and no conclusions have been reached. Haynes et al. (Haynes and Highberg, 1976) performed out a static uniaxial compression test of concrete at a water depth of 6,100 m, and its strength decreased by 10% compared with the reference group. Subsequently, Bjerkli et al. (Bjerkli et al., 1993) studied the mechanical properties of concrete under water pressures at 400 and 800 m and observed that under the same curing and testing conditions, water pressure did not indigenously affect the compressive strength of the concrete.
Li et al. and Du et al. (Du et al., 2009; Li and DU, 2011) placed concrete of different maximum aggregate sizes under pressurized water to study the effects of water pressure and action time on the compressive strength of the concrete. The results indicated that when the maximum aggregate size was the same, the compressive strength loss rate increased with an increase in the water pressure and action time. When other conditions are the same, the larger the maximum aggregate particle size, the greater the compressive strength loss rate is. Xue et al. (Xue et al., 2020) observed that under high pore water pressure, the uniaxial compressive strength of concrete decreased with an increase in the exposure time and water pressure. At this instant, the uniaxial load exhibits four stages: elastic, stable crack propagation, unstable crack propagation, and failure. Tian et al. (Tian et al., 2014; Wang, 2017) studied the mechanical properties of saturated concrete at water pressures of 0, 2, 5, and 10 MPa. The results indicated that the compressive strength increased with an increase in water pressure when the strain rate was 0.01/s, and the maximum increase was 83%. Zhang et al. and Zhou et al. (Zhang, 2017; Zhou et al., 2020) conducted similar experiments and obtained similar results.
Furthermore, researchers have studied the multiaxial loading under water pressure. Tian et al. and Peng et al. (Tian et al., 2014; Peng et al., 2015) conducted constant triaxial tests on concrete under different pore water pressures. It was observed that the strength of concrete under natural drying conditions was greater than that of saturated concrete, and the peak stress and strain of concrete increased with an increase in confining pressure (Imran and PANTAZOPOULOU, 1996). Li et al. (Li et al., 2007) and Chen et al. (Chen et al., 2010) studied the compressive strengths of dry and saturated concrete under water pressure and triaxial stress. The test results indicated that the strength of dry and saturated concrete increased with an increase in the confining pressure under sealing conditions, and the confining pressure effect of dry concrete was evident. When the specimen was directly exposed to water, the compressive strengths of the dry and saturated concretes significantly decreased. Under confining pressures of 2 and 4 MPa, the compressive strength of the dry concrete decreased by 56.8% and 62.1%, and that of saturated concrete decreased by 15.3% and 30.9%, respectively. Vu et al. (Vu et al., 2009) conducted triaxial compression tests under confining pressures of 20–650 MPa, and the results indicated that the shear strength of the dry concrete almost linearly increased with confining pressure increase. Under a confining pressure of 200 MPa and above, the ultimate compressive strength of concrete decreases with an increase in saturation.
3.2 Effect of pore water on the dynamic strength of concrete
Owing to the viscosity and incompressibility of pore water, the occurrence and expansion of internal cracks and the formation of macroscopic cracks in concrete under dynamic loads differ from those in the natural state (Fu et al., 2021). In addition, the rate sensitivity of concrete makes its dynamic strength in a water environment different from that in the natural state (Cao et al., 2010).
Researchers (Klepaczko and BRARA, 2001; Mori, 2001; Lin and YAN, 2006; Yan and LIN, 2006; Liu et al., 2017) have studied the compressive strength of concrete under different water pressures and loading rates. The normalized results are shown in Figure 5. The results indicated that the compressive strength of dry concrete and wet concrete increased with an increase in the strain rate. When the strain rate is 10–5/s, the dry concrete has a higher compressive strength; however, with an increase in the strain rate, the difference between the compressive strengths of wet concrete and dry concrete is narrowed and even slightly reversed. Wang et al. (Peng et al., 2015; Wang et al., 2017) demonstrated that the compressive strength of concrete increased with an increase in water pressure at the same strain rate. When the water pressure was 10 MPa, the compressive strength increased by 84.4% compared with that at 0 MPa. The water pressure increased the sensitivity of the concrete to the strain rate, and the increase was 144.9% when the water pressure was 5 MPa. In addition, it is observed that the water content and strain rate affect the failure mode of concrete; when the water content of the wet concrete is 1.27 times higher than that of the natural state, the cone failure of the concrete will occur under rapid loading, and with an increase in the water content and strain rate, the area of the crack surface and the amount of debris shedding of the specimen decrease as well; when the water content of concrete is lower than that of the natural state or under slow loading, oblique shear failure occurs (Wang et al., 2016a).
Lin et al. (Lin and YAN, 2006) studied the effect of water content on the strain-rate effect of concrete. The ultimate compressive strength of the saturated concrete at a quasi-static strain rate was lower than that of the natural concrete; however, the saturated concrete exhibited a higher strain rate sensitivity. Zhang et al. and Li et al. (Li, 2014; Zhang, 2017) performed dynamic compression tests in a water environment (Loading speed 0.05–3 MPa). It was observed that humidity increased the rate effect of the concrete compressive strength, and the compressive strength increased exponentially with the loading rate under the same humidity. In general, the rate effect of wet concrete is more evident than that of natural concrete, and an increase in water pressure aggravates the rate effect of the concrete.
Under biaxial compression, Wang et al. (Wang et al., 2016b) observed that the static compressive strength of dry concrete was greater than that of saturated concrete; however, this observation is in contrast to the results under high strain rates. The influence of the strain rate on the saturated concrete is evident, especially when subjected to lateral constraints. A few researchers have indicated that the mechanical properties of wet concrete decrease within a specific strain-rate range. Ding et al. (Ding and ZHOU, 2014) observed that under a dynamic load of 50/s, the dynamic compressive strength of concrete decreased with an increase in the water content, and the maximum decrease was 25.35%. Zhou et al. (Zhou et al., 2011) observed that when the strain rate was 10–2/s, the compressive strength of concrete decreased with an increase in the water content.
4 Influence mechanism of water environment on mechanical properties of concrete
When the concrete is in a water environment for a long time, water infiltrates into the concrete along the opening pores of the concrete, so that the concrete is under different humidity conditions, thereby exhibiting different water contents. As mentioned above, in the study of the effect of concrete water content or saturation on its compressive strength, the water content or saturation as the only index does not completely exclude the interference of the structural characteristics of concrete pores. According to the study results, the specimen with water content exhibited a smaller compressive strength; however, the higher the water content, the more space the specimen held water, i.e., there was a larger porosity, and the strength was bound to be smaller. Therefore, it is necessary to determine the relationship between the pore characteristics and strength. In addition, the interference of porosity should be excluded as far as possible in future studies.
As a dense material, the internal humidity response of concrete is not uniform when it is in a water environment. Therefore, a humidity gradient is present inside. Relevant studies have demonstrated that the humidity gradient influences the mechanical properties of wet concrete. When wet concrete is subjected to static load, the pore water, like a wedge, may accelerate crack propagation. When the wet concrete is subjected to rapid loading, pore water has a certain inhibitory effect on the occurrence and propagation of cracks, and the pore water pressure and “Stefan” effect affect the dynamic strength of concrete.
4.1 Influence mechanism of pore distribution on mechanical strength of concrete
Pore structures exist in aggregates, cementitious materials, and interface transition zones and can be divided into primary and secondary pores. The internal pore structure possesses the characteristics of large morphological differences, disorderly distribution, and wide pore size coverage, which significantly impact the strength, deformation, and durability of concrete (Luna-Galiano et al., 2016; Zhang et al., 2020; Liu et al., 2021; Chen et al., 2022; Ren et al., 2022). However, not all pore structures adversely affect concrete performance. For instance, the pore structure can serve as a water supply channel for the continuous hydration of cementitious materials and provide space for the growth of hydration products. Wu (Wu, 1979) classified pore structures into harmless pores (<20 nm), less harmful pores (20–50 nm), harmful pores (50–200 nm), and harmful pores of >200 nm based on the pore size. Based on this observation, several scholars have explored the relationship between the pore structure characteristics and strength considering various influencing factors.
Early studies established a relationship model between porosity and strength considering the relationship between porosity and strength (Table 2). In the formula, p is the porosity of concrete, σ0 is not always reliable for characterizing the strength of the porous material or calculating the strength of the specimen to the strength of porosity of 0, and the pore structure characteristic parameters that affect the strength include pore volume, pore effective diameter, pore surface area, and pore spacing coefficient (Li and ZHAO, 2021). The aforementioned model was not considered; therefore, it is not accurate in practical applications.
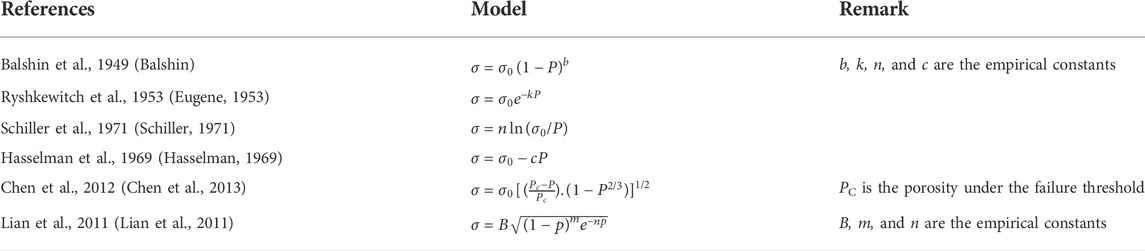
TABLE 2. Comparison of early models with regard to the relationship between compressive strength and porosity of concrete.
Moreover, scholars have tried to build comprehensive and applicable models, considering factors other than porosity (Table 3). Bu et al. (Bu and TIAN, 2016) extracted the pore structure characteristic parameters, e.g., porosity and pore size distribution, based on Mercury Intrusion Porosimetry (MIP) technology and established an exponential model of pore structure characteristics and strength. Zhao et al. (Zhao et al., 2014) established an extended the “Bhattacharjee” model (Rakesh and BHATTACHARJEE, 2003) considering porosity, average pore size, and hydration degree, which can clearly describe the relationship between the pore structure characteristics and strength of cement mortar. Based on this model, Zhang et al. (Zhang et al., 2021) analyzed the influence of pore size distribution on strength, obtained the sensitive pore size that affects the compressive strength of concrete, and corrected the existing model according to the sensitivity coefficient. The results indicated that this model possessed a greater correlation with the calculation results than the existing model. Li et al. (Li et al., 2018) proposed a simplified central-hole model (Figure 6) based on the three-phase and hollow sphere models. Explicit expressions for tensile strength, compressive strength, and porosity were derived considering the influence of the pore size. The results indicated that the compressive strength of concrete decreases with an increase in porosity, and the decrease in compressive strength is greater than that in tensile strength. The strength of concrete can be improved by reducing its pore size. In addition, a few scholars (Jin et al., 2017; Han et al., 2022) have used the fractal theory to characterize the pore size distribution and proposed new pore characteristic parameters. The established functional relationship model demonstrated a good prediction accuracy.
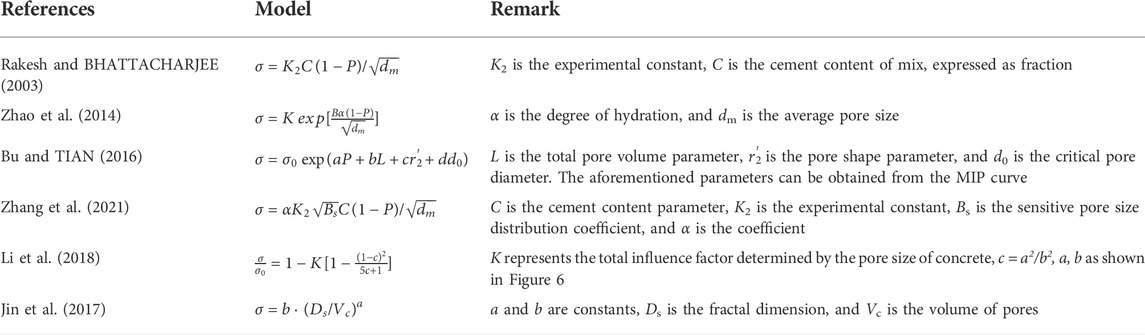
TABLE 3. Comparison of the relationship models with regard to compressive strength and the porosity of concrete.
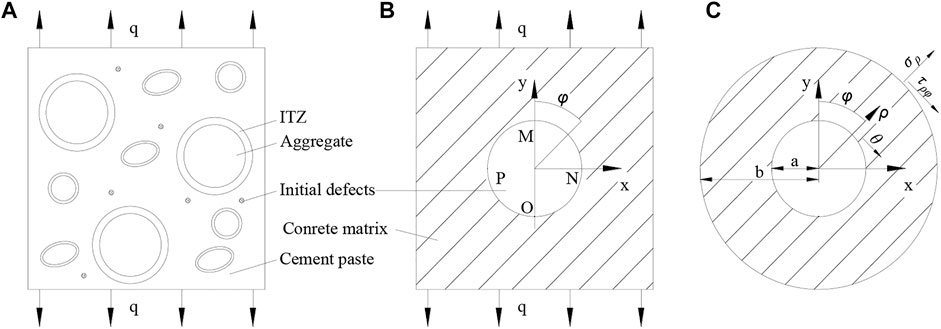
FIGURE 6. Equivalent process (A) stress state of a representative element; (B) a simplified central pore model; (C) equivalent polar coordinate model of (B) (Li et al., 2018)
4.2 Mechanism of pore water on concrete strength
4.2.1 Mechanism of humidity gradient on concrete strength
A few researchers believe that the internal humidity gradient of wet concrete will affect its strength. Xu et al. (Xu et al., 2017) studied the internal water content, distribution, and change law of concrete and observed that the internal moisture change of concrete was caused by the hydration reaction, evaporation, and free migration of water. The migration was divided into two stages. The first stage included upward migration caused by the density of water and solids, and the migration rate was the quadratic function of the water content. The second stage was caused by the humidity gradient and follows Fick’s law. Larrard et al. (Larrard et al., 1992; Gao et al., 2013; Xu et al., 2017) demonstrated that it was difficult for concrete to reach full saturation, and a humidity gradient existed inside, which will certainly impact the concrete performance. Popovic et al. (Popovics, 1986) stated that the internal moisture migration of concrete produces uneven deformation, which produces tensile and compressive stresses in the concrete and reduces its strength. Wei et al. (Wei, 2012; Gao and WEI, 2014) studied the formation law of humidity gradient inside the concrete pavements, analyzed the influence of humidity gradient on the shrinkage deformation and stress of concrete, and established the calculation method of warping deformation and stress caused by humidity. They conducted that the nonlinear humidity gradient caused the nonlinear shrinkage of concrete slab along the depth direction. This shrinkage deformation can be divided into two macro deformation modes of uniform shrinkage and warping, and the other part of the deformation is transformed into internal stress by internal constraints (as shown in Figure 7). Li et al. (Li and ZHENG, 2012) regarded that the humidity gradient makes the elastic modulus of each part of wet concrete different, resulting in different stresses, so the failure mode of wet concrete is different from that of dry concrete. The aforementioned studies have explained the reason for the decrease in the strength of wet concrete to a certain extent; however, relevant studies have confirmed that even if there is no humidity gradient, pore water affects the mechanical properties of concrete. Thus, this influence mechanism needs to be further studied.
4.2.2 Mechanism of pore water pressure on concrete strength
Wittmann (Wittmann, 1973) stated that the decrease in the static mechanical properties of wet concrete is caused by the separation of cementitious particles using pore water, which reduces the van der Waals force and surface energy (Wang et al., 2008), thus reducing the critical stress of the cracks. Kaplan et al. (Seyit, 1980; Tetsuri and CHIKAKO, 2014) stated that when concrete was subjected to load, the pores and channels perpendicular to the load direction were closed, and free water flowed inside the concrete. The viscosity of the water caused a pore water pressure gradient. Under a high loading rate, the pore water pressure increased, and the occurrence of cracks in the solid phase was delayed, thereby increasing the compressive strength of the concrete. Rossi et al. (Rossi, 1991a; Rossi, 1991b) used the “Stefan” effect to explain why the cracking process and mechanical properties of wet concrete at high strain rates are different from those of reference concrete. The “Stefan” effect refers to the existence of a thin layer of mucous membrane (water, oil, and other viscous liquids) between two flat parallel plates at a certain distance, and the reverse force will occur when the two plates are separated at a certain speed. The greater the speed, the greater the reverse force is (Zhou, 2007). Rossi regarded microporous and capillary walls as two parallel plates. When concrete is subjected to a dynamic load, the internal microstructure is deformed by extrusion, and the deformation time is short. Therefore, the large reverse force may be the reason for the increase in the rate sensitivity of the wet concrete.
Most of the aforementioned studies are qualitative descriptions and discussions based on test results and lack systematic theoretical studies. Based on the “Stefan” effect, Wang (Wang and LI, 2006b) systematically analyzed the pore water pressure mechanism affecting the static and dynamic compressive strengths of saturated concrete based on elasticity and fracture mechanics. The wing cracks are shown in Figure 8. They are used for calculation, and considering the interaction between the two cracks, the static compressive strength of saturated concrete is defined as
where KⅠ is the strength factor at the crack tip; β0 is the value when KⅠ considers the maximum value, which is a function of the friction coefficient between cracks; a1 is the relationship coefficient between pore water pressure and external pressure stress; l is the length of the airfoil crack; c is the half of the length of the oblique crack; w is the half of the crack spacing.
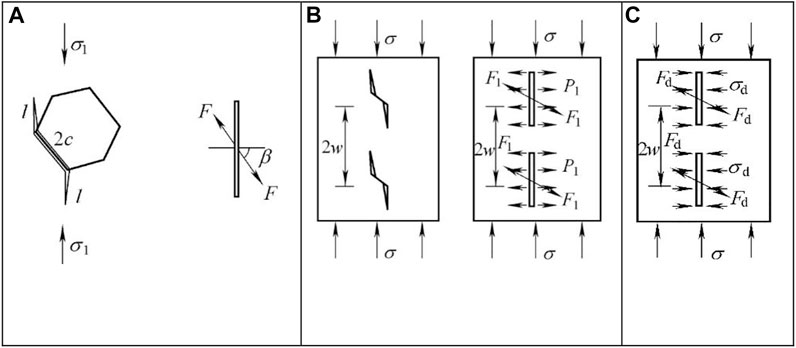
FIGURE 8. Wing cracks under the uniaxial load (A) wing crack; (B), (C) two interacting cracks and their equivalent forms (Gao and WEI, 2014)
Under rapid loading, the free water in the crack did not easily reach the crack tip (Figure 9), and σd is generated by the reaction force under the “Stefan” effect. Under dynamic loading, the stress intensity factor of the crack is defined as
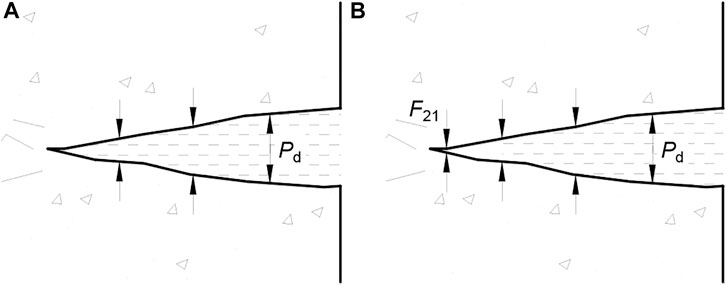
FIGURE 9. Water pressure distribution in cracks under different loading rates (A) water reaches the crack tip under slow loading; (B) water cannot reach the crack tip under fast loading.
Assuming that the actual fracture toughness of concrete matrix does not change with the loading rate, the dynamic compressive strength of concrete is defined as
where k(v) is the influence coefficient of the crack growth rate with regard to the stress intensity factor at the crack tip, D (β0) = cosβ0 [sinβ0cosβ0-μ (cos2β0-a2)-B1], a2 is the water pressure coefficient, and B1 is the viscosity coefficient; other symbols have the same definition.
Eqs. 4–8 are only applicable to dry or saturated concrete. Based on this, the factors influencing the pore water pressure of unsaturated concrete have been considered, as expressed in Eq. 9, and the aforementioned calculation formula has been modified in one of the studies (Wang and LI, 2006a) for application to general wet concrete.
where p is the pore water pressure; a1 and a2 are the coefficients of the relationship between the pore water pressure and the external load when the concrete volume is in the state of compression and expansion, respectively, and they are related to volume deformation εv, saturation S and crack propagation velocity V; in addition, a2 is related to the damage degree D of concrete; σc is the stress value when the volume compression of concrete is maximum.
The aforementioned study attempted to reveal the influence mechanism of pore water pressure on the static and dynamic compressive strength of wet concrete based on the theoretical level. When the loading rate was low, the free water in the pore could reach the micro-crack tip and be affected by the deformation of the concrete matrix. The pore water acted as a “wedge” to accelerate crack propagation and reduced the compressive strength of wet concrete. When the loading rate was high, the crack propagated rapidly, and it was difficult for pore water to reach the crack tip. Under the combined action of the pore water pressure and “Stefan” effect, the crack development was hindered, dynamic compressive strength of the wet concrete improved, and wet concrete exhibited more rate sensitivity than the dry concrete.
5 Conclusion
Several achievements have been obtained in research on the mechanical properties of wet concrete. In this study, the influence of water on the mechanical properties of concrete is reviewed. The following points have been concluded:
1) The static compressive strength of wet concrete was lower than that of dry concrete; however, the elastic modulus improved. This is because the water content of the concrete is positively correlated with porosity, and the strength decreases with an increase in porosity. However, the elastic modulus of water is greater than that of air; therefore, the elastic modulus increases with an increase in the water content.
2) With an increase in the strain rate, the compressive strengths of wet and dry concretes increased; however, the rate sensitivity of the wet concrete was more evident than that of the dry concrete. This is because the concrete exhibits a high rate sensitivity, coupled with the pore water pressure and “Stefan” effect, making the rate sensitivity prominent.
Previous studies on the relationship between the moisture state and mechanical properties of concrete have used drying and soaking methods to obtain specimens with different water contents, and the influence of porosity on concrete performance has not been excluded. In addition, most existing studies provide qualitative explanations based on experimental results, and a few provide quantitative explanations based on the theoretical level. Thus, future studies should focus on the following points:
1) While considering water content as a variable to study the concrete strength, the influence of irrelevant variables, e.g., porosity, can be excluded. Using the same specimen or a specimen with similar porosity, accurate pore characteristics and moisture migration information can be obtained based on X-ray Diffraction (XRD), Computed Tomography (CT), nondestructive testing, and other technologies.
2) Multifactor coupling tests, e.g., impact load, multi-axial stress state, and high-pressure water environment, can be conducted to meet the requirements of cement-based materials in complex water environments.
3) Theoretical studies on changes in the mechanical properties of wet concrete can be conducted based on various assumptions and models to further study and quantify the influence of water on the mechanical properties of fresh and hardened concrete.
Author contributions
JW: Conceptualization, Methodology, Investigation, Data curation, Writing—original draft. KS: Investigation, Formal analysis, Data curation, Writing—review and editing. YH: Resources, Writing—review and editing. QG: Resources, Writing—review and editing, Funding acquisition. QL: Resources, Validation, Writing—review and editing, Supervision, Funding acquisition.
Funding
This study is financially supported by the Open Research Fund Program of State key Laboratory of Hydroscience and Engineering (sklhse-2022-C-01), Natural Science Foundation of Henan (No.222300420081), National Natural Science Foundation of China (52179145, 51909244) and Program for Science and Technology Innovation Talents in Universities of Henan Province, China (No.21HASTIT013).
Acknowledgments
The authors gratefully acknowledge the support provided by above funding.
Conflict of interest
The authors declare that the research was conducted in the absence of any commercial or financial relationships that could be construed as a potential conflict of interest.
The handling editor JG declared a past co-authorship with the author JW
Publisher’s note
All claims expressed in this article are solely those of the authors and do not necessarily represent those of their affiliated organizations, or those of the publisher, the editors and the reviewers. Any product that may be evaluated in this article, or claim that may be made by its manufacturer, is not guaranteed or endorsed by the publisher.
References
Alexandridis, A., and Gardner, N. J. (1981). Mechanical behaviour of fresh concrete. Cem. Concr. Res. 11 (3), 323–339. doi:10.1016/0008-8846(81)90105-8
Bai, W. F., Chen, J. Y., and Sun, S. N. (2010). Effect of moisture in pore on initial elastic modulus of concrete[J]. J. Dalian Univ. Technol. 50 (05), 712–716.
Balshin, M. Relation of mechanical properties of powder metals and their porosity and the ultimate properties of porous metal-ceramic materials[C], 831–834.
Bažant, Z. P., and Najjar, L. J. (1972). Nonlinear water diffusion in nonsaturated concrete. Mat. Constr. 5 (1), 3–20. doi:10.1007/BF02479073
Bischoff, P., and Perry, S. H. (1991). Compressive behaviour of concrete at high strain rates. Mater. Struct. 24 (6), 425–450. doi:10.1007/BF02472016
Bjerkli, L., Jensen, J., and Rolf, (1993). Strain development and static compressive strength of concrete exposed to water pressure loading[J]. Int. Concr. Abstr. Portal 3 (90), 310–315.
Bu, J., and Tian, Z. (2016). Relationship between pore structure and compressive strength of concrete: Experiments and statistical modeling. Sadhana 3 (41), 337–344. doi:10.1007/s12046-016-0468-9
Cadoni, E., Labibes, K., Albertini, C., Berra, M., and Giangrasso, M. (2001). Strain-rate effect on the tensile behaviour of concrete at different relative humidity levels. Mat. Struct. 34 (34), 21–26. doi:10.1007/BF02482196
Cao, Y. Z., Zhang, S. T., and Meng, Q. X. (2010). Effect of loading rate on fracture energy of high-strength concrete[J]. J. Build. Mater. 13 (01), 90–93. doi:10.3969/j.issn.1007-9629.2010.01.019
Chen, J. H., Li, Y. L., Zhou, H., and Guo, H. (2022). Nuclear magnetic resonance study on concrete pore structure evolution under different curing environments. Adv. Charact. Funct. Compos. Mater. 5 (74), 1819–1827. doi:10.1007/s11837-022-05221-3
Chen, X. D., Wu, S. X., and Zhou, J. K. (2013). Influence of porosity on compressive and tensile strength of cement mortar. Constr. Build. Mater. 40, 869–874. doi:10.1016/j.conbuildmat.2012.11.072
Chen, Z. F. S., Hu, Y., and Li, Q. B. (2010). Behavior of concrete in water subjected to dynamic triaxial compression[J]. J. Eng. Mech. 136136 (3), 379–389. doi:10.1061/(ASCE)0733-939910.1061/(asce)0733-9399(2010)136:3(379)
DavidHARRIS Cem, W. (2000). Dynamic properties of mass concrete obtained from dam cores[J]. Mater. J. 3 (97), 290–296. 0889-325X(2000)l:3<290:DPOMCO>2.0.TX;2-#.
De Schutter, G., and Taerwe, L. (1996). Degree of hydration-based description of mechanical properties of early age concrete. Mat. Struct. 29 (190), 335–344. doi:10.1007/BF02486341
Deng, H. F., Yuan, X. F., Li, J. L., Luo, Q., and He, M. (2014). Fracture mechanics characteristics and deterioration mechanism of sandstone under reservoir immersion interaction[J]. Eart. Scie.—J. China Univ. Geosci. 39 (01), 108–114. doi:10.3799/dqkx.2014.011
Ding, N., and Zhou, J. K. (2014). Moisture effect on compressive behavior of concrete under dynamic loading. J. Cent. South Univ. 21 (12), 4714–4722. doi:10.1007/s11771-014-2481-7
Du, S. L., Li, Z. L., and Jin, X. Y. (2009). Preliminary study on the influence of pore water pressure on the compressive strength of concrete[J]. Yangtze River 40 (03), 54–55.
Du, S. L., and Li, Z. L. (2012). Review on damage of concrete by pore water pressure[J]. Pearl River 33 (03), 13–18. doi:10.3969/j.issn.1001-9235.2012.03.005
Eugene, R. (1953). Compression strength of porous sintered alumina and zirconia. J. Am. Ceram. Soc. 36 (2), 65–68. doi:10.1111/j.1151-2916.1953.tb12837.x
Fu, H. C., Erki, M. A., and Seckin, M. (1991). Review of effects of loading rate on concrete in compression. J. Struct. Eng. 117117 (12), 3660–3679. doi:10.1061/(ASCE)0733-944510.1061/(asce)0733-9445(1991)117:12(3645)
Fu, Q., Zhang, Z. R., Zhao, X., Hong, M., Guo, B., Yuan, Q., et al. (2021). Water saturation effect on the dynamic mechanical behaviour and scaling law effect on the dynamic strength of coral aggregate concrete. Cem. Concr. Compos. 120, 104034. doi:10.1016/j.cemconcomp.2021.104034
Gao, X., and Wei, Y. (2014). Modeling and analysis of moisture gradients in concrete pavements[J]. Eng. Mech. 31 (08), 183–188. doi:10.6052/j.issn.1000-4750.2013.03.0225
Gao, Y., Zhang, J., and Han, Y. (2013). Experimental study on the internal relative humidity in concrete under dry-wet cycles[J]. J. Build. Mater. 16 (03), 375–381. doi:10.3969/j.issn.1007-9629.2013.03.001
Han, X., Wang, B. M., and Feng, J. J. (2022). Relationship between fractal feature and compressive strength of concrete based on MIP. Constr. Build. Mater. 322, 126504. doi:10.1016/j.conbuildmat.2022.126504
Hasselman, D. P. H. (1969). Griffith flaws and the effect of porosity on tensile strength of brittle ceramics. J. Am. Ceram. Soc. 8 (52), 457. doi:10.1111/j.1151-2916.1969.tb11982.x
Haynes, H. H., and Highberg, R. S. (1976). Concrete properties at ocean depths. J. Wtrwy., Harb. Coast. Engrg. Div. 102 (4), 455–470. doi:10.1061/awhcar.0000347
Imran, I., and Pantazopoulou, S. J. (1996)., Experimental study of plain concrete under triaxial stress[J]ACI Mater. J. 6(966). Farmington Hills, MI: CO, 5892–6015. doi:10.1002/(SICI)1099-1794
Javier, L., and Malvar, C. (1998). Review of strain rate effects for concrete in tension. Mj 95 (6). doi:10.14359/418
Jin, N. G., Jin, X. Y., and Guo, J. F. (2005). Relationship modeling of pore structure and strength of concrete[J]. J. Zhejiang Univ. Sci. (11), 1680–1684.
Jin, S. S., Zhang, J. X., and Han, S. (2017). Fractal analysis of relation between strength and pore structure of hardened mortar. Constr. Build. Mater. 135, 1–7. doi:10.1016/j.conbuildmat.2016.12.152
Josué César Bastos, S. M. A., Marcusdersch, S. P. E. A. M., and Edwards, J. R. (2022). Degradation mechanisms of concrete due to water flow in cracks of prestressed railroad sleepers under cyclic loading. J. Mat. Civ. Eng. 4 (34), 4022025. doi:10.1061/(ASCE)MT.1943-5533.0004144
Klepaczko, J. R., and Brara, A. (2001). An experimental method for dynamic tensile testing of concrete by spalling. Int. J. Impact Eng. 25 (4), 387–409. doi:10.1016/S0734-743X(00)00050-6
Kuster, G., and Toksöz, M. (1974). Velocity and attenuation of seismic waves in two‐phase media: Part I. Theoretical formulations. Geophysics 39 (5), 587–606. doi:10.1190/1.1440450
Larrard, F., Bostvironnois, J., and Roper, H. (1992). Discussion: On the long-term strength losses of silica fume high-strength concretes[J]. Mag. Concr. Res. 44 (159), 143–145. doi:10.1680/macr.1991.43.155.109
Li, D. Q., Li, Z. L., Lv, C. C., Zhang, G., and Yin, Y. (2018). A predictive model of the effective tensile and compressive strengths of concrete considering porosity and pore size. Constr. Build. Mater. 170, 520–526. doi:10.1016/j.conbuildmat.2018.03.028
Li, G. (2004). The effect of moisture content on the tensile strength properties of concrete[D]. Gainesville: University of Florida.
Li, L. (2009). Study on effect of water content on concrete performance[D]. BeiJing: Beijing Jiaotong University.
Li, N., and Zhao, Y. R. (2021). Research progress of concrete internal damage based on X-ray CT technology[J]. Mater. Rep. 35 (21), 21169–21177.
Li, Q. B., Chen, Z. F. S., Sun, M. Y., and Lv, P. Y. (2007). Effect of water loading on strength of concrete[J]. J. Hydraulic Eng. (07), 786–791. doi:10.13243/j.cnki.slxb.2007.O7.004
Li, Q. B., and Wang, H. L. (2006). Influence of ambient water on properties of concrete [J]. Sci. Pap. Online (02), 83–94.
Li, S. L. (2014). YangLing agricultural high-tech industrial demonstration zone, shaanxi Province. Xianyang: Northwest A&F University. Preliminary studies on the effect of water content and pore water pressure on the concrete strength[D]
Li, T. J., Shi, K. F., Su, Y., Zhao, J., and Wamg, G. Q. (2022). Precipitation recycling characteristics of the upper and middle yellow river basin[J]. Yellow River 44 (02), 21–26. doi:10.3969/j.issn.1000-1379.2022.02.005
Li, X., and Zheng, D. (2012). Wet concrete strength model based on reliability analysis[J]. Eng. Mech. 29 (05), 161–165.
Li, Z. L., and Du, S. L. (2011). Experimental study on mechanical properties of concrete due to high seepage pore water pressure[J]. Eng. Mech. 28 (11), 72–77.
Lian, C., Zhuge, Y., and Beecham, S. (2011). The relationship between porosity and strength for porous concrete. Constr. Build. Mater. 25 (11), 4294–4298. doi:10.1016/j.conbuildmat.2011.05.005
Lian, C., and Zhuge, Y. (2010). Optimum mix design of enhanced permeable concrete - an experimental investigation. Constr. Build. Mater. 24 (12), 2664–2671. doi:10.1016/j.conbuildmat.2010.04.057
Lin, G., and Yan, D. M. (2006). Strain-rate sensitivity of concrete: Influence of moisture content. Key Eng. Mat. 326-328 (1-2), 1661–1664. doi:10.4028/www.scientific.net/KEM.326-328.1661
Liu, B. D., Li, P. F., Li, L., and Kang, Y. G. (2011). Experimental study on influence of water content on concrete strength[J]. J. Beijing Jiaot. Univ. 35 (01), 9–12.
Liu, B. D., Lv, W. J., Li, L., and Li, P. F. (2014). Effect of moisture content on static compressive elasticity modulus of concrete. Constr. Build. Mater. 69, 133–142. doi:10.1016/j.conbuildmat.2014.06.094
Liu, F., Zhang, G. X., Luo, T., Xin, J., and Jiang, W. (2021). Study on pore development and water migration regularity in the process of strength formation of hydraulic concrete. Meas. (. Mahwah. N. J). 183, 109811. doi:10.1016/j.measurement.2021.109811
Liu, H. J., Li, Z. L., Tan, C., Zhang, R., and Tong, T. T. (2020). Experimental study on influence of different moisture contents on fracture acoustic emission characteristics of concrete[J]. Adv. Eng. Sci. 52 (06), 153–161. doi:10.15961/j.jsuese.201900686
Liu, Q., Peng, G., Cao, P., and Xie, J. H. (2017). Influences of cyclic loading history to rate effect of water-saturated concrete under pressurized water environment[J]. J. Civ. Archit. Environ. Eng. 39 (06), 46–53. doi:10.11835/j.issn.1674-4764.2017.06.006
Luna-Galiano, Y., Fernández-Pereira, C., and Izquierdo, M. (2016). Contributions to the study of porosity in fly ash-based geopolymers. Relationship between degree of reaction, porosity and compressive strength. Mat. construcc. 66 (324), 098. doi:10.3989/mc.2016.10215
Mehta, P. K., and Monteiro, P. J. M. (2014). Concrete: microstructure, properties, and materials[M]. McGraw-Hill Education.
Mori, K. U. (2001). Influence of moisture content on compressive and tensile strength properties of concrete under high strain-rates[J]. J. Struct. Eng. 3 (47), 1673–1681.
Peng, G., Wang, Q. F., and Liang, C. H. (2015). Study on dynamic compressive properties of concrete under pore water pressure environment[J]. China Civ. Eng. J. 48 (01), 11–18. doi:10.15951/j.tmgcxb.2015.01.002
Popovics, S. (1986). Effect of curing method and final moisture condition on compressive strength of concrete[J]. J. Proc. 4 (83), 650–657.
Qiu, Y. P., and Weng, G. J. (1990). On the application of Mori-Tanaka's theory involving transversely isotropic spheroidal inclusions. Int. J. Eng. Sci. 28 (11), 1121–1137. doi:10.1016/0020-7225(90)90112-V
Rakesh, K., and Bhattacharjee, B. (2003). Porosity, pore size distribution and in situ strength of concrete. Cem. Concr. Res. 33 (1), 155–164. doi:10.1016/S0008-8846(02)00942-0
Reinhardt, W. R., Rossi, R., and van Mier, G. M. M. (1990). Joint investigation of concrete at high rates of loading. Mater. Struct. 23 (3), 213–216. doi:10.1007/BF02473020
Ren, J. G., Luo, X. X., Bai, R. Q., Pan, C., and Zhang, J. (2022). Pore characteristics of different phase in nano-modified concrete and their influences on the compressive strength. J. Build. Eng. 46, 103784. doi:10.1016/j.jobe.2021.103784
Ross, A., and David, M. J. J. (1996). Moisture and strain rate effects on concrete strength[J]. ACI Mater. J. 3 (93), 293–300. doi:10.1680/macr.1991.43.154.53
Rossi, P. (1991). A physical phenomenon which can explain the mechanical behaviour of concrete under high strain rates. Mater. Struct. 24 (6), 422–424. doi:10.1007/BF02472015
Rossi, P., and Boulay, C. (1990). Influence of free water in concrete on the cracking process. Mag. Concr. Res. 42 (152), 143–146. doi:10.1680/macr.1990.42.152.143
Rossi, P. (1991). Influence of cracking in the presence of free water on the mechanical behaviour of concrete. Mag. Concr. Res. 43 (154), 53–57. doi:10.1680/macr.1991.43.154.53
Rossi, P., van Mier, J. G. M., Boulay, C., and Le Maou, F. (1992). The dynamic behaviour of concrete: Influence of free water. Mater. Struct. 25 (9), 509–514. doi:10.1007/BF02472446
Rossi, R. (1994). Dynamic behaviour of concretes: From the material to the structure. Mater. Struct. 27 (6), 319–323. doi:10.1007/BF02473423
Schiller, K. K. (1971). Strength of porous materials. Cem. Concr. Res. 4 (1), 419–422. doi:10.1016/0008-8846(71)90035-4
Seyit, A. K. (1980). Factors affecting the relationship between rate of loading and measured compressive strength of concrete. Mag. Concr. Res. 32 (111), 79–88. doi:10.1680/macr.1980.32.111.79
Tetsuri, K., and Chikako, F. (2014). Mechanical properties of saturated concrete depending on the strain rate. Procedia Eng. 95, 442–453. doi:10.1016/j.proeng.2014.12.204
Tian, W., Peng, G., and Chen, X. Q. (2014). Dynamic characteristic study of concrete under pressure of water environment[J]. J. Civ. Eng. Manag. 31 (04), 50–54. doi:10.13579/j.cnki.2095-0985.2014.04.010
Ulrik, N. A., and Monteiro, P. J. M. (1993). Concrete: A three phase material. Cem. Concr. Res. 23 (1), 147–151. doi:10.1016/0008-8846(93)90145-Y
Vu, X. H., Malecot, Y., Daudeville, L., and Buzaud, E. (2009). Experimental analysis of concrete behavior under high confinement: Effect of the saturation ratio. Int. J. Solids Struct. 46 (5), 1105–1120. doi:10.1016/j.ijsolstr.2008.10.015
Wang, H., and Li, Q. (2007). Prediction of elastic modulus and Poisson's ratio for unsaturated concrete. Int. J. Solids Struct. 44 (5), 1370–1379. doi:10.1016/j.ijsolstr.2006.06.028
Wang, H., Wang, L. C., Song, Y. P., and Wang, J. (2016). Influence of free water on dynamic behavior of dam concrete under biaxial compression. Constr. Build. Mater. 112, 222–231. doi:10.1016/j.conbuildmat.2016.02.090
Wang, H. L., and Li, Q. B. (2006). Effect of pore water on the compressive strength of wet concrete[J]. Eng. Mech. (10), 141–144.
Wang, H. L., and Li, Q. B. (2007). Experiments of the compressive properties of dry and saturated concrete under different loading rates[J]. J. Hydroelectr. Eng. (01), 84–89.
Wang, H. L., and Li, Q. B. (2007). Meso-mechanism of effects of free water on mechanical properties of concrete under confined compression[J]. J. Tsinghua Univ. Sci. Technol. (09), 1443–1446. doi:10.13243/cnki.slxb.2007.05.005
Wang, H. L., and Li, Q. B. (2006). Micro-mechanism of static and dynamic strengths for saturated concrete[J]. J. Hydraulic Eng. (08), 958–962. doi:10.14062/j.issn.0454-5648.1979.03.010
Wang, H. L., and Li, Q. B. (2005). Saturated concrete elastic modulus prediction[J]. J. Tsinghua Univ. Sci. Technol. (06), 761–763. doi:10.16511l/j.crki.qhdxxb.2005.06.011
Wang, H. L., Li, Q. B., Sun, X. Y., and Jin, W. L. (2008). Mesomechanism of tensile strength reduction and tension constitutive model of saturated concrete[J]. J. Basic Sci. Eng. (01), 65–72. doi:10.16058/j.issn.10050930.2008.01.011
Wang, Q. F., Liu, Y. H., and Peng, G. (2016). Effect of water pressure on mechanical behavior of concrete under dynamic compression state. Constr. Build. Mater. 125, 501–509. doi:10.1016/j.conbuildmat.2016.08.058
Wang, Q. F., Liu, Y. H., and Peng, G. (2017). Water content in concrete under water pressure environment and the effect on its mechanical properties[J]. J. Hydraulic Eng. 48 (02), 193–202. doi:10.13243/j.cnki.slxb.20160539
Wang, Q. F. (2017). ShaanXi xi'an city. Xi'an: Xi'an University of Technology. Study on dynamic mechanical properties and mechanism of concrete in water environment[D]
Wei, Y. (2012). Mechanism of moisture warping and deformation calculations in concrete pavements[J]. Eng. Mech. 29 (11), 266–271. doi:10.6052/j.issn.1000-4750.2011.04.0229
Wittmann, F. H. (1973). Interaction of hardened cement paste and water. J. Am. Ceram. Soc. 56 (8), 409–415. doi:10.1111/j.1151-2916.1973.tb12711.x
Wittmann, F. H., Sun, Z., and Zhao, T. (2007). “Strength and fracture energy of concrete in seawater[C],” in Proceedings of the 6th International Conference on Fracture Mechanics of Concrete and Concrete Structures. Catania, 17–22.
Wu, S. X., Chen, X. D., and Zhou, J. K. (2012). Influence of strain rate and water content on mechanical behavior of dam concrete. Constr. Build. Mater. 36, 448–457. doi:10.1016/j.conbuildmat.2012.06.046
Wu, Z. W. (1979). An approach to the recent trends of concrete science and technology[J]. J. Chin. Ceram. Soc. (03), 262–270.
Xu, W. B., Li, Q. B., and Hu, Y. (2017). Water content variations in the process of concrete setting[J]. J. Hydroelectr. Eng. 36 (07), 92–103. doi:10.11660/slfdxb.20170710
Xue, W., Yao, Z., Jing, W., and Song, H. (2020). Mechanical damage and failure behavior of shaft-lining concrete after exposure to high pore-water pressure. J. Mat. Civ. Eng. 1 (32), 4019339. doi:10.1061/(ASCE)MT.1943-5533.0002922
Yaman, I. O. (2002). Active and non-active porosity in concrete - part 1: Experimental evidence. Mat. Struct. 35 (246), 102–109. doi:10.1617/13639
Yaman, I. O., Aktan, H. M., and Hearn, N. (2002). Active and non-active porosity in concrete Part II: Evaluation of existing models. Mat. Struct. 35 (246), 110–116. doi:10.1007/BF02482110
Yan, D. M., and Lin, G. (2006). Study on dynamic compressive properties of concrete with different loading paths[J]. J. Hydraulic Eng. (03), 360–364. doi:10.13243/j.cnki.slxb.2006.03.018
Yan, D. M., Lin, G., Wang, Z., and Zhang, Y. Q. (2005). Research on dynamic direct tensile properties of concrete under different environments[J]. J. Dalian Univ. Technol. (03), 416–421.
Yu, S., Li, K., and Feng, G. (2015). Experiment on water absorbing and surface pore property of concrete. Procedia Eng. 121 (C), 1443–1448. doi:10.1016/j.proeng.2015.09.057
Zhang, G. H., Li, X. H., and Wei, H. (2019). Experimental study on concrete humidity under water environment[J]. Adv. Sci. Technol. Water Resources 39 (06), 51–55. doi:10.3880/j.issn.1006-7647.2019.06.008
Zhang, G. H., Li, Z. L., Nie, K. Y., and Liu, M. H. (2016). Experimental study on fracture toughness of concrete with different moisture contents [J]. J. Hydroelectr. Eng. 35 (02), 109–116. doi:10.11660/slfdxb.20160213
Zhang, G. H. (2017). YangLing agricultural high-tech industrial demonstration zone, shaanxi Province. Xianyang: Northwest A&F University.Study on mechanical properties of concrete under different water pressure conditions[D]
Zhang, M., Yao, X. H., Guan, J. F., Li, L., Wang, J., and Qing, L. (2021). Study of concrete strength and pore structure model based on grey relation entropy. Materials 14 (2), 432. doi:10.3390/ma14020432
Zhang, P., Sun, Z. W., Zhao, Z. J., and Wittmann, F. H. (2010). Fracture energy and strain softening of concrete under seawater environment[J]. J. Civ. Architectural& Environ. Eng. 32 (01), 72–77.
Zhang, Z. Y., Zhou, J. T., Yang, J., Zou, Y., and Wang, Z. (2020). Understanding of the deterioration characteristic of concrete exposed to external sulfate attack: Insight into mesoscopic pore structures. Constr. Build. Mater. 260, 119932. doi:10.1016/j.conbuildmat.2020.119932
Zhao, H., Zhao, Q., Huang, D., and Zhang, S. (2014). Influence of pore structure on compressive strength of cement mortar. Sci. World J. 2014, 1–12. doi:10.1155/2014/247058
Zheng, D., and Li, X. X. (2011). Prediction of saturate concrete elastic modulus by considering pores and microcracks. Amr 168-170 (168), 594–598. doi:10.4028/www.scientific.net/AMR.168-170.594
Zheng, D., and Li, X. X. (2011). Review of researches on influences of pore water on static properties of concrete[J]. Adv. Sci. Technol. Water Resour. 31 (01), 90–94. doi:10.3880/j.issn.1006-7647.2011.01.021
Zhou, J. K., Chen, X. D., Wu, L. Q., and Kan, X. (2011). Influence of free water content on the compressive mechanical behaviour of cement mortar under high strain rate. Sadhana 36 (3), 357–369. doi:10.1007/s12046-011-0024-6
Zhou, J. K. (2007). Experimental study on dynamic bending - tensile mechanical properties and mechanism of high arch dam concrete[D]. Nanjing, JiangSu Province: Hohai University.
Zhou, J. K., Zhao, X. Y., Nie, Y., and Tian, Y. (2020). Influence of high water pressure on static and dynamic compressive strength of concrete. Adv. Civ. Eng. 2020, 1–9. doi:10.1155/2020/8814626
Zhou, S., Shen, A., Li, S., and Li, G. F. (2015). A relationship of mesoscopic pore structure and concrete bending strength. Mater. Res. Innovations 19 (10), S10–S100. doi:10.1179/1432891715Z.0000000002100
Keywords: wet concrete, pore water, static characteristics, dynamic characteristics, review
Citation: Wang J, Sun K, Hu Y, Guan Q and Li Q (2022) The mechanical properties of concrete in water environment: A review. Front. Mater. 9:996650. doi: 10.3389/fmats.2022.996650
Received: 18 July 2022; Accepted: 01 August 2022;
Published: 29 August 2022.
Edited by:
Junfeng Guan, North China University of Water Conservancy and Electric Power, ChinaReviewed by:
Syed Minhaj Saleem Kazmi, RMIT University, AustraliaRu Mu, Hebei University of Technology, China
Zhimin Wu, Dalian University of Technology, China
Copyright © 2022 Wang, Sun, Hu, Guan and Li. This is an open-access article distributed under the terms of the Creative Commons Attribution License (CC BY). The use, distribution or reproduction in other forums is permitted, provided the original author(s) and the copyright owner(s) are credited and that the original publication in this journal is cited, in accordance with accepted academic practice. No use, distribution or reproduction is permitted which does not comply with these terms.
*Correspondence: Qingbin Li, cWluZ2JpbmxpQG1haWwudHNpbmdodWEuZWR1LmNu