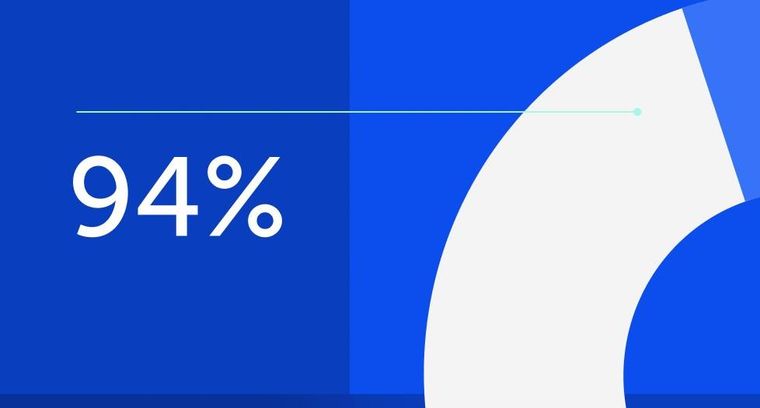
94% of researchers rate our articles as excellent or good
Learn more about the work of our research integrity team to safeguard the quality of each article we publish.
Find out more
MINI REVIEW article
Front. Mater., 07 September 2022
Sec. Biomaterials and Bio-Inspired Materials
Volume 9 - 2022 | https://doi.org/10.3389/fmats.2022.990931
This article is part of the Research TopicBiological Stimuli-Responsive Smart MaterialsView all 5 articles
Bone tumors are deadly and incurable diseases that invade large areas of bone, resulting in bone defects. Traditional therapies combining surgery, chemotherapy, and radiation have reached their limit of efficacy, motivating efforts to develop new therapeutic methods. Fortunately, the development of biomaterials provides innovative options for bone tumor treatment. Suitable biomaterials are capable of simultaneously providing tumor therapy and promoting bone regeneration. This review summarizes recent progress in the effort to achieve new strategies for bone tumor treatment using biomaterials, focusing on the innovative scaffold design. It also discusses the development of nanocarrier-based drug delivery systems and hyperthermia therapy for bone tumor treatment. In the future, biomaterial-based strategies are likely to become the most effective and reliable options for treating bone tumors, and they have the potential to greatly improve the prognosis and quality of life for patients.
Bone tumors can be broadly divided into primary bone tumors (sarcomas) and secondary bone tumors (metastases). Primary bone tumors are uncommon (comprising approximately only 0.2% of malignant tumors) in all age groups (Jiang et al., 2020). These tumors are heterogeneous, including osteosarcomas, chondrosarcomas, and Ewing sarcomas, which are mostly diagnosed in childhood and adolescence (Ambrosio et al., 2021). Approximately 30% of patients with primary bone tumors die within 5 years due to poor response to treatment. Metastatic bone tumors are frequent complications of many cancers at a later stage, with a high incidence in breast and prostate cancer in particular. Bone metastasis has a 5-year survival rate of only 10% as a result of poor prognosis (Gonzalez Diaz et al., 2019). Due to their high degree of malignancy and complexity, strong invasiveness, and considerable mortality, bone tumors bring great suffering to patients.
In the clinic, conventional therapies for bone tumors mainly include surgical interventions, chemotherapy, and radiotherapy. Unfortunately, surgical resection often fails to completely eradicate micrometastases, which is likely to result in postoperative recurrence and metastasis (Chen and Yao, 2022). In some cases, bone defects caused by surgery are the main cause of physical disability. Sometimes, attempts are made to eliminate tumors exclusively with chemotherapy and radiotherapy. However, drug resistance and strong side effects can appear during chemotherapy. Additionally, some bone tumors such as osteosarcoma are not sensitive to radiotherapy and are inclined to chemotherapy resistance (Miwa et al., 2021). Considering the challenges in bone tumor treatment and the clinical need for new approaches, in the last few years, researchers have focused on creating innovative biomaterials with the ability to elicit specific cell behaviors that are needed for both tumor therapy and bone tissue regeneration (Guerrieri et al., 2020).
In the first attempt of its kind, an American surgeon tried to implant calcium phosphates to repair damaged bone in the early 20th century (Dorozhkin, 2013). Implants designed to repair bone defects have since evolved, with some offering different levels of structural support and some stimulating bone regeneration using osteoinductive materials (Giannoudis et al., 2005). Various materials have been developed over the years, and bone-tissue engineering eventually arose as an independent scientific field in the 1990s (Koons et al., 2020). Bone-tissue engineering has witnessed the rise of some emerging fields, including biomaterial science, developmental and molecular science, and nanotechnology. In the past decade, research in these fields has inspired innovation in new biomaterials, scaffold design, fabrication techniques, and applications. Herein, we review the history of materials for bone repair and provide more details on osteosarcoma treatment, as this field has been dynamically developing due to urgent clinical needs. The timeline of major milestones in the progress of material development for bone repair and osteosarcoma treatment is illustrated in Figure 1.
FIGURE 1. Timeline of major milestones in the development of materials for bone repair and osteosarcoma treatment. The development of materials for bone repair began in the 1920s with the use of calcium phosphates and metal substitutes to replace lost bone at defect sites. Other forms of materials have been introduced throughout the subsequent years, such as bioglass and polymer materials, and bone-tissue engineering eventually arose as an independent field. The advent of 3D printing fabrication opened the path to designing bioactive scaffolds, which are the focus in the field of bone-tissue engineering. Furthermore, bone-tissue engineering has witnessed some emerging fields, including biomaterials science, nanotechnology, and their convergence with 3D printing, in the last few years. These fields have been dynamically evolving due to urgent needs from clinical applications.
The two main challenges in bone tumor therapy are eliminating tumor cells and facilitating bone regeneration (Ma et al., 2018; Liao et al., 2021). Some new therapies have shown strong potential, particularly the biomaterial-based strategy (including targeted drug delivery and hyperthermia therapy), which has demonstrated high anticancer effects (Tan et al., 2021). More importantly, biomaterial scaffolds can provide an ideal in vivo environment for cells to grow, proliferate, and differentiate, and they can also leverage the synergistic effect of bioactive molecules for bone tissue repair (Brunello et al., 2020; Tan et al., 2021). Thus, the biomaterial-based strategy is an innovative option that is capable of simultaneously achieving bone tumor therapy and promoting bone regeneration.
In this review, we summarize the recent progress in the development of biomaterials for bone tumor therapy and bone defect regeneration. We describe biomaterial scaffolds created for simultaneous bone tumor therapy and bone regeneration, focusing on innovative scaffold design. Finally, we also discuss nanocarrier-based drug delivery systems and hyperthermia therapy for bone tumor treatment.
When treating bone defects caused by tumor resection, the design of biomaterials must simultaneously meet the requirements of bone repair and tumor suppression. Therefore, biomaterial selection and structural design are critical (Du et al., 2019). The purpose of a biomaterial scaffold is not to simply replace the missing bone tissue but also to create a biomimetic microenvironment for the growth of cells and tissues by mimicking the natural extracellular matrix. The scaffold must inherit the biomaterial’s advantageous properties, such as excellent biocompatibility, adequate mechanical strength, biodegradation, and cell adhesion and transportation (Tevlin et al., 2014).
Biomaterials used in scaffolds can be divided into polymers, bioceramics, carbon containing materials, metals, and hydrogels (Marques et al., 2014). Table 1 shows bioactive scaffolds fabricated for bone tumor treatment and bone regeneration.
Polymeric materials have strong potential for application in bone tumor therapy due to their good biocompatibility and design flexibility. According to their origin, polymers can be simply classified into two groups, namely natural polymers and synthetic polymers (Filippi et al., 2020).
Natural polymers used for bone tissue regeneration mainly include collagen, gelatin, chitosan, and alginate (Marques et al., 2014; Zhao et al., 2020). They are similar to the components in the native extracellular matrix, ensuring superior biocompatibility and minute immunogenicity. One potential advantage of natural polymers is that they often contain functional molecules, which is advantageous for cellular adhesion (Bose et al., 2012). However, natural polymers also face many disadvantages. In some cases, pathogenic impurities may exist, which can trigger immunogenic reactions. Other disadvantages include low mechanical strength, poor elastic properties, and less control over degradability, which could limit their use in load-bearing applications (Peric Kacarevic et al., 2020).
Synthetic polymers such as polymethylmethacrylate (PMMA) and poly (lactic-coglycolic acid) (PLGA) have been investigated for bone tumor applications (Yu et al., 2019; Long et al., 2021). Unlike natural polymers, synthetic polymers can be fabricated to meet desired mechanical characteristics and geometric properties. However, poor biocompatibility and unsatisfactory hydrophilicity limit clinical applications of synthetic polymers. Furthermore, synthetic polymers produce undesirable degradation products and create a local acidic environment, which can have cytotoxic effects and cause inflammatory responses (Bharadwaz and Jayasuriya, 2020).
Calcium phosphate ceramics, including hydroxyapatite (HA), β-tricalcium phosphate, and bioactive glass (BG), are commonly used for orthopedic applications, as calcium phosphate is abundant in native human bone (Luo S. et al., 2019; Dang et al., 2018; Lu et al., 2018; H. Ma et al., 2020). Hydroxyapatite is present in natural bone as an inorganic component of bone matrix. It has excellent biocompatibility and osteoinduction properties, so it has been widely used in bone defect repair materials and has also been considered as a promising carrier for drug delivery to sites of bone disease (Marques et al., 2014; Tang et al., 2021). Hydroxyapatite could allow strong integration with host tissue, further promoting new bone formation in vivo and selectively and efficiently killing tumor cells in vitro to achieve the purpose of bone tumor therapy (Luo Y. et al., 2019). The design of bioceramics not only greatly expands bone tumor therapeutic strategies but also represents a new direction for bioceramics science. However, the drawback of brittleness could limit the utility of bioceramics in load-bearing support. Additionally, it is difficult to tune the resorption rate of bioceramics, resulting in a decline of mechanical properties (Turnbull et al., 2018).
Generally, an ideal metallic material possesses excellent biocompatibility and high mechanical performance, and it releases non-toxic ions. As typical representatives, magnesium (Mg), titanium, and their alloys are suitable for clinical applications (Huang et al., 2020). However, poor corrosion resistance could induce tissue reactions and raise the risk of loosening. The higher elastic modulus of metals relative to natural bone can also result in bone resorption and therapeutic failure. In addition, poor biodegradability may lead to further impairment of tissue ingrowth (Turnbull et al., 2018). However, surface modification has been applied to improve bioactivity in traditional biomaterials. It enhances metallic corrosion resistance and promotes osteoblast attachment through coating, showing superior osteogenesis and integration ability (Zhang et al., 2019).
It is well known that scaffolds have been significantly improved using nanobiological materials (Quadros et al., 2021). Nanomaterials, including polymeric nanoparticles, carbon-based nanomaterials, and metallic nanoparticles, have been designed to permit local drug delivery in bone tumor therapies. They also have excellent photothermal conversion ability and high adsorption ability, so they could serve as photothermal agents (Badila et al., 2021).
Carbon-based materials are potentially useful nanomaterials for bone tumor treatment. Most studied carbon materials for therapeutics are graphene, graphene oxide (GO), and reduced graphene oxide (rGO) (Li D. et al., 2018; Yin et al., 2021). Both GO and rGO are generated through graphene conversion (Geetha Bai et al., 2019). Graphene has exceptional mechanical properties, favorable osteoinductivity, and a large surface area. It can provide active sites that support cell differentiation, growth, and proliferation. However, the cytotoxicity of graphene and its derivatives has always been problematic (Fang et al., 2022).
With the development of biomaterial science, inorganic nanoparticles have also made their way into therapeutics through the use of nanotechnology (Fang et al., 2022). Metal nanoparticles such as Fe and Cu, which have been incorporated into scaffolds, have shown potential in promoting the proliferation and differentiation of mesenchymal stem cells (MSCs) in animal models (Dang et al., 2018; Lu et al., 2018; Ma et al., 2018).
Hydrogels possess good biocompatibility, biodegradability, injectability, and the ability to load growth factors, meaning they have the potential to repair bone defects (Fang et al., 2022). Some new injectable hydrogels prepared through chemical reactions have been applied to bone tumor treatment. They not only effectively ablate bone tumor cells via photothermal effects but also support the attachment, proliferation, and osteogenic differentiation of bone MSCs. In addition, they can be used for more accurate targeted delivery of anticancer drugs and fixation of bone defect tissue (Yu and Ding, 2008; Luo S. et al., 2019). Although hydrogels are still in development, the prospect of multifunctional hydrogel-based materials has been strongly demonstrated in anticancer treatment and bone repair.
To engineer the ideal biomaterial scaffold, osteoinductive bioactive components, such as growth factors and progenitor cells, should be successfully recruited for bone regeneration (Qi et al., 2021). The essential stem cell sources provide higher proliferative ability and allow the differentiation of various cell types from a single cell (Vats et al., 2002). MSCs, endothelial progenitor cells (EPCs), and induced pluripotent stem cells (iPSCs) are included in bone progenitor cells. MSCs are currently more suitable for tissue repair since they can differentiate into bone and connective tissues (Vats et al., 2002). The incorporation of growth factors into biomaterial scaffolds can promote cell growth and differentiation for the normal healing response. Growth factors for improving osteogenesis and angiogenesis include vascular endothelial growth factors (VEGF), fibroblast growth factors (FGFs), and bone morphogenic proteins (BMPs) (Brunello et al., 2020; Qi et al., 2021).
The development and application of 3D printing technology represents a huge opportunity for bone-tissue engineering. Over the years, this technology has quickly evolved into advanced methods suitable for the fabrication of novel, geometrically intricate, biomimetic biomaterial scaffolds (Nikolova and Chavali, 2019). Moreover, 3D printing technology can be used to design and manufacture living tissue-like structures with properties similar to those of natural bone tissue (Parisi et al., 2018). Additionally, computer-assisted design technologies can be used to generate 3D models that fully resemble native tissue to better mimic cellular interactions and processes (Vats et al., 2002; Koons et al., 2020). Overall, 3D printing is regarded as a revolutionary and powerful tool that has been successfully employed in medicine, especially in the field of tissue engineering. However, it still faces great challenges, and solving these challenges will require multidisciplinary collaboration on the creation of advanced techniques for analysis and quantification.
As one of the widely investigated applications for nanotechnology, nanocarrier-based drug delivery systems offer promise for the treatment of cancers (Bae et al., 2011). Nanomaterials can be used as carriers to encapsulate chemotherapeutic agents to prolong drug circulation time and protect them from rapid clearance (Muntimadugu et al., 2017). Targeting the delivery of drugs to the bone could achieve a high local concentration, enhance therapeutic efficiency, and reduce systemic toxicity (Bae et al., 2011; Lungu et al., 2019). The successful exploration of drug delivery systems will provide new methods for ideal bone tumor therapy, which encourages the development of targeted therapies. Nanocarriers developed for bone tumor mainly include liposomes, polymeric nanoparticles, metallic nanoparticles, carbon-based nanomaterials, and calcium phosphate carriers (Wang et al., 2020). Table 2 summarizes the application of antineoplastic drugs and nanocarriers in osteosarcoma drug delivery systems.
Studies on hyperthermia therapy, such as biomaterials with good photothermal and magnetothermal properties, have demonstrated encouraging outcomes in bone tumor treatment. Photothermal therapy (PTT) employs near-infrared (NIR) laser photoabsorbers to convert light energy into heat energy to ablate cancer cells (Zou et al., 2016; de Oliveira et al., 2021). Photothermal agents could be used as NIR absorbents and enhancers to increase the efficiency of localized light-based heating (Li et al., 2020a). To date, various types of photothermal agents using biomaterials, such as metal nanomaterials, carbon-based nanoparticles, and biomaterial scaffolds, have been designed and developed for bone tumor treatment (Zou et al., 2016). Photodynamic therapy (PDT) can also selectively induce the death of tumor cells, which is based on the localized generation of oxidative stress, preserving normal tissues (Sibata et al., 2000). It has been proved that giant cell tumors, chondrosarcoma, and osteosarcoma are susceptible to in vitro PDT (Hourigan et al., 1993). Magnetic hyperthermia (MHT) is a non-invasive method for bone tumor ablation, and it is based on heat generation by magnetic materials (Sedighi et al., 2021). MHT could achieve localized tumor heating and induces the apoptosis/necrosis of cells by the transformation of electromagnetic energy under an alternating magnetic field (Fatima et al., 2021).
With the development of material science, biomaterials have attracted increasing attention due to their specific biological properties, excellent tumor specificity, and high drug-loading capacity. One of the major advantages of biomaterials is how precisely they can be controlled with specific structures according to individual defect conditions. Biomaterial scaffolds largely mimic native tissue, with excellent biosafety and minimal biological immune reactions due to the combination of biomaterials and bioactive components, allowing them to be used safely in patients. In recent decades, researchers have attempted to design functional biomaterials for simultaneously killing bone tumor cells and repairing bone defects induced by surgical resection. In addition, multifunctional biomaterial designs enabling bone regeneration, chemotherapy drug delivery, and the anticancer effects of hyperthermia therapy have been constructed. Thus, supplementary or alternative methods based on biomaterials are expected to become integrated bone tumor therapy strategies (Figure 2).
FIGURE 2. Biomaterial-based bone tumor and bone repair scheme. The biomaterial-based strategy (including targeted chemotherapy, photothermal therapy, photodynamic therapy, and magnetic hyperthermia) demonstrates a high anticancer effect in the early stage. Additionally, biomaterials with great osteoinductivity support the attachment, proliferation, and differentiation of mesenchymal stem cells in bone tissue repair.
Although many studies have shown that hyperthermia could effectively result in irreversible cell death and tumor destruction by generating high temperatures, it is difficult to avoid damage to normal tissue and other side effects, such as local immune-inflammatory response. Recently, more efficient drug delivery systems incorporating bioactive substances (antibiotics or antineoplastic drugs) have been designed through biomaterial modification, allowing drugs to be released in situ (Andronescu et al., 2013). However, the precise control of drug distribution in space and time is a challenge still faced by drug delivery systems, so further studies are needed. Last but not least, it should be kept in mind that multiple functions of biomaterials are based on animal model studies. We believe that through the interdisciplinary collaboration of experts in the fields of clinical medicine, material science, and nanotechnology, more detailed studies and comprehensive explorations can successfully identify treatments with higher efficiency to aid in the fight against bone tumors.
This review summarizes the very latest developments in the biomaterial-based strategy for bone tumor treatment, with a focus on innovative scaffold design. It also discusses nanomaterials that can help deliver drugs or provide hyperthermia therapy to kill bone tumor cells. In the future, the biomaterial-based strategy may offer unprecedented opportunities for clinical bone tumor therapy and bone regeneration while reducing treatment periods and ultimately improving the prognosis of patients.
YZ reviewed the literature, and wrote the manuscript. YW and XQ wrote and revised the manuscript. TL and YW assisted in drawing. MW designed and revised the manuscript. All authors contributed to the article and approved the submitted version.
This work was supported by Grants from the Natural Science Foundation of Heilongjiang Province (No. LH2019H039), and the Haiyan Foundation of Harbin Medical University Cancer Hospital (No. JJZD2020-09).
We thank LetPub (www.letpub.com) for its linguistic assistance during the preparation of this manuscript.
The authors declare that the research was conducted in the absence of any commercial or financial relationships that could be construed as a potential conflict of interest.
All claims expressed in this article are solely those of the authors and do not necessarily represent those of their affiliated organizations, or those of the publisher, the editors and the reviewers. Any product that may be evaluated in this article, or claim that may be made by its manufacturer, is not guaranteed or endorsed by the publisher.
Ambrosio, L., Raucci, M. G., Vadala, G., Ambrosio, L., Papalia, R., and Denaro, V. (2021). Innovative biomaterials for the treatment of bone cancer. Int. J. Mol. Sci. 22 (15), 8214. doi:10.3390/ijms22158214
Andronescu, E., Ficai, A., Albu, M. G., Mitran, V., Sonmez, M., Ficai, D., et al. (2013). Collagen-hydroxyapatite/cisplatin drug delivery systems for locoregional treatment of bone cancer. Technol. Cancer Res. Treat. 12 (4), 275–284. doi:10.7785/tcrt.2012.500331
Badila, A. E., Radulescu, D. M., Niculescu, A. G., Grumezescu, A. M., Radulescu, M., and Radulescu, A. R. (2021). Recent advances in the treatment of bone metastases and primary bone tumors: An up-to-date review. Cancers (Basel) 13 (16), 4229. doi:10.3390/cancers13164229
Bae, K. H., Chung, H. J., and Park, T. G. (2011). Nanomaterials for cancer therapy and imaging. Mol. Cells 31 (4), 295–302. doi:10.1007/s10059-011-0051-5
Bharadwaz, A., and Jayasuriya, A. C. (2020). Recent trends in the application of widely used natural and synthetic polymer nanocomposites in bone tissue regeneration. Mater. Sci. Eng. C 110, 110698. doi:10.1016/j.msec.2020.110698
Bose, S., Roy, M., and Bandyopadhyay, A. (2012). Recent advances in bone tissue engineering scaffolds. Trends Biotechnol. 30 (10), 546–554. doi:10.1016/j.tibtech.2012.07.005
Brunello, G., Panda, S., Schiavon, L., Sivolella, S., Biasetto, L., and Del Fabbro, M. (2020). The impact of bioceramic scaffolds on bone regeneration in preclinical in vivo studies: A systematic review. Mater. (Basel) 13 (7), 1500. doi:10.3390/ma13071500
Caliskan, Y., Dalgic, A. D., Gerekci, S., Gulec, E. A., Tezcaner, A., Ozen, C., et al. (2019). A new therapeutic combination for osteosarcoma: Gemcitabine and Clofazimine co-loaded liposomal formulation. Int. J. Pharm. X. 557, 97–104. doi:10.1016/j.ijpharm.2018.12.041
Chen, H., and Yao, Y. (2022). Progress of biomaterials for bone tumor therapy. J. Biomater. Appl. 36 (6), 945–955. doi:10.1177/08853282211035236
Dang, W., Li, T., Li, B., Ma, H., Zhai, D., Wang, X., et al. (2018). A bifunctional scaffold with CuFeSe2 nanocrystals for tumor therapy and bone reconstruction. Biomaterials 160, 92–106. doi:10.1016/j.biomaterials.2017.11.020
de Oliveira, S. A., Borges, R., Dos Santos Rosa, D., de Souza, A. C. S., Seabra, A. B., Baino, F., et al. (2021). Strategies for cancer treatment based on photonic nanomedicine. Mater. (Basel) 14 (6), 1435. doi:10.3390/ma14061435
Dong, S., Chen, Y., Yu, L., Lin, K., and Wang, X. (2019). Magnetic hyperthermia-synergistic H2O2 self-sufficient catalytic suppression of osteosarcoma with enhanced bone-RegenerationBioactivity by 3D-printing composite scaffolds. Adv. Funct. Mat. 30 (4), 1907071. doi:10.1002/adfm.201907071
Dorozhkin, S. V. (2013). A detailed history of calcium orthophosphates from 1770s till 1950. Mater. Sci. Eng. C 33 (6), 3085–3110. doi:10.1016/j.msec.2013.04.002
Du, Y., Guo, J. L., Wang, J., Mikos, A. G., and Zhang, S. (2019). Hierarchically designed bone scaffolds: From internal cues to external stimuli. Biomaterials 218, 119334. doi:10.1016/j.biomaterials.2019.119334
Fang, H., Zhu, D., Yang, Q., Chen, Y., Zhang, C., Gao, J., et al. (2022). Emerging zero-dimensional to four-dimensional biomaterials for bone regeneration. J. Nanobiotechnol. 20 (1), 26. doi:10.1186/s12951-021-01228-1
Fang, Z., Sun, Y., Xiao, H., Li, P., Liu, M., Ding, F., et al. (2017). Targeted osteosarcoma chemotherapy using RGD peptide-installed doxorubicin-loaded biodegradable polymeric micelle. Biomed. Pharmacother. 85, 160–168. doi:10.1016/j.biopha.2016.11.132
Fatima, H., Charinpanitkul, T., and Kim, K. S. (2021). Fundamentals to apply magnetic nanoparticles for hyperthermia therapy. Nanomater. (Basel) 11 (5), 1203. doi:10.3390/nano11051203
Filippi, M., Born, G., Chaaban, M., and Scherberich, A. (2020). Natural polymeric scaffolds inBone regeneration. Front. Bioeng. Biotechnol. 8, 474. doi:10.3389/fbioe.2020.00474
Geetha Bai, R., Muthoosamy, K., Manickam, S., and Hilal-Alnaqbi, A. (2019). Graphene-based 3D scaffolds in tissue engineering: Fabrication, applications, and future scope in liver tissue engineering. Int. J. Nanomedicine 14, 5753–5783. doi:10.2147/ijn.S192779
Giannoudis, P. V., Dinopoulos, H., and Tsiridis, E. (2005). Bone substitutes: An update. Injury 36 (3), S20–S27. doi:10.1016/j.injury.2005.07.029
Gonzalez Diaz, E. C., Sinha, S., Avedian, R. S., and Yang, F. (2019). Tissue-engineered 3D models for elucidating primary and metastatic bone cancer progression. Acta Biomater. 99, 18–32. doi:10.1016/j.actbio.2019.08.020
Guerrieri, A. N., Montesi, M., Sprio, S., Laranga, R., Mercatali, L., Tampieri, A., et al. (2020). Innovative options for bone metastasis treatment: An extensive analysis on biomaterials-based strategies for orthopedic surgeons. Front. Bioeng. Biotechnol. 8, 589964. doi:10.3389/fbioe.2020.589964
Hartono, S. B., Yu, M., Gu, W., Yang, J., Strounina, E., Wang, X., et al. (2014). Synthesis of multi-functional large pore mesoporous silica nanoparticles as gene carriers. Nanotechnology 25 (5), 055701. doi:10.1088/0957-4484/25/5/055701
Hourigan, A. J., Kells, A. F., and Schwartz, H. S. (1993). In vitro photodynamic therapy of musculoskeletal neoplasms. J. Orthop. Res. 11 (5), 633–637. doi:10.1002/jor.1100110504
Huang, S., Wang, B., Zhang, X., Lu, F., Wang, Z., Tian, S., et al. (2020). High-purity weight-bearing magnesium screw: Translational application in the healing of femoral neck fracture. Biomaterials 238, 119829. doi:10.1016/j.biomaterials.2020.119829
Irmak, G., Öztürk, M. G., and Gümüşderelioğlu, M. (2020). Salinomycin encapsulated PLGA nanoparticles eliminate osteosarcoma cells via inducing/inhibiting multiple signaling pathways: Comparison with free salinomycin. J. Drug Deliv. Sci. Technol. 58, 101834. doi:10.1016/j.jddst.2020.101834
Jiang, W., Rixiati, Y., Zhao, B., Li, Y., Tang, C., and Liu, J. (2020). Incidence, prevalence, and outcomes of systemic malignancy with bone metastases. J. Orthop. Surg. Hong. Kong) 28 (2), 230949902091598. doi:10.1177/2309499020915989
Koons, G. L., Diba, M., and Mikos, A. G. (2020). Materials design for bone-tissue engineering. Nat. Rev. Mat. 5 (8), 584–603. doi:10.1038/s41578-020-0204-2
Li, D., Nie, W., Chen, L., McCoul, D., Liu, D., Zhang, X., et al. (2018). Self-assembled hydroxyapatite-graphene scaffold for photothermal cancer therapy and bone regeneration. J. Biomed. Nanotechnol. 14 (12), 2003–2017. doi:10.1166/jbn.2018.2646
Li, L., Luo, C., Song, Z., Reyes-Vargas, E., Clayton, F., Huang, J., et al. (2018). Association of anti-HER2 antibody with graphene oxide for curative treatment of osteosarcoma. Nanomedicine Nanotechnol. Biol. Med. 14 (2), 581–593. doi:10.1016/j.nano.2017.11.001
Li, S., Xiong, Y., and Zhang, X. (2017). Poloxamer surface modified trimethyl chitosan nanoparticles for the effective delivery of methotrexate in osteosarcoma. Biomed. Pharmacother. 90, 872–879. doi:10.1016/j.biopha.2017.04.004
Li, X., Park, E. Y., Kang, Y., Kwon, N., Yang, M., Lee, S., et al. (2020a). Supramolecular phthalocyanine assemblies for improved photoacoustic imaging and photothermal therapy. Angew. Chem. Int. Ed. 59 (22), 8630–8634. doi:10.1002/anie.201916147
Li, X., Wang, L., Wang, L., Yu, J., Lu, G., Zhao, W., et al. (2020b). Overcoming therapeutic failure in osteosarcoma via Apatinib-encapsulated hydrophobic poly(ester amide) nanoparticles. Biomater. Sci. 8 (21), 5888–5899. doi:10.1039/d0bm01296c
Liao, J., Han, R., Wu, Y., and Qian, Z. (2021). Review of a new bone tumor therapy strategy based on bifunctional biomaterials. Bone Res. 9 (1), 18. doi:10.1038/s41413-021-00139-z
Liu, Y., Zhang, X., Liu, Z., Wang, L., Luo, L., Wang, M., et al. (2017). Gold nanoshell-based betulinic acid liposomes for synergistic chemo-photothermal therapy. Nanomedicine Nanotechnol. Biol. Med. 13 (6), 1891–1900. doi:10.1016/j.nano.2017.03.012
Long, J., Zhang, W., Chen, Y., Teng, B., Liu, B., Li, H., et al. (2021). Multifunctional magnesium incorporated scaffolds by 3D-Printing for comprehensive postsurgical management of osteosarcoma. Biomaterials 275, 120950. doi:10.1016/j.biomaterials.2021.120950
Low, S. A., Yang, J., and Kopecek, J. (2014). Bone-targeted acid-sensitive doxorubicin conjugate micelles as potential osteosarcoma therapeutics. Bioconjug. Chem. 25 (11), 2012–2020. doi:10.1021/bc500392x
Lu, J. W., Yang, F., Ke, Q. F., Xie, X. T., and Guo, Y. P. (2018). Magnetic nanoparticles modified-porous scaffolds for bone regeneration and photothermal therapy against tumors. Nanomedicine Nanotechnol. Biol. Med. 14 (3), 811–822. doi:10.1016/j.nano.2017.12.025
Lungu, , Grumezescu, A. M., Volceanov, A., and Andronescu, E. (2019). Nanobiomaterials used in cancer therapy: An up-to-date overview. Molecules 24 (19), 3547. doi:10.3390/molecules24193547
Luo, S., Wu, J., Jia, Z., Tang, P., Sheng, J., Xie, C., et al. (2019). An injectable, bifunctional hydrogel with photothermal effects for tumor therapy and bone regeneration. Macromol. Biosci. 19 (9), e1900047. doi:10.1002/mabi.201900047
Luo, Y., Wei, X., Wan, Y., Lin, X., Wang, Z., and Huang, P. (2019). 3D printing of hydrogel scaffolds for future application in photothermal therapy of breast cancer and tissue repair. Acta Biomater. 92, 37–47. doi:10.1016/j.actbio.2019.05.039
Ma, H., Li, T., Huan, Z., Zhang, M., Yang, Z., Wang, J., et al. (2018). 3D printing of high-strength bioscaffolds for the synergistic treatment of bone cancer. NPG Asia Mat. 10 (4), 31–44. doi:10.1038/s41427-018-0015-8
Ma, H., Ma, Z., Chen, Q., Li, W., Liu, X., Ma, X., et al. (2020). Bifunctional, copper-doped, mesoporous silica nanosphere-modified, bioceramic scaffolds for bone tumor therapy. Front. Chem. 8, 610232. doi:10.3389/fchem.2020.610232
Marques, C., Ferreira, J. M., Andronescu, E., Ficai, D., Sonmez, M., and Ficai, A. (2014). Multifunctional materials for bone cancer treatment. Int. J. Nanomedicine 9, 2713–2725. doi:10.2147/IJN.S55943
Martella, E., Ferroni, C., Guerrini, A., Ballestri, M., Columbaro, M., Santi, S., et al. (2018). Functionalized keratin as nanotechnology-based drug delivery system for the pharmacological treatment of osteosarcoma. Int. J. Mol. Sci. 19 (11), 3670. doi:10.3390/ijms19113670
Miwa, S., Yamamoto, N., and Tsuchiya, H. (2021). Bone and soft tissue tumors: New treatment approaches. Cancers (Basel) 13 (8), 1832. doi:10.3390/cancers13081832
Muntimadugu, E., Kommineni, N., and Khan, W. (2017). Exploring the potential of nanotherapeutics in targeting tumor microenvironment for cancer therapy. Pharmacol. Res. 126, 109–122. doi:10.1016/j.phrs.2017.05.010
Nikolova, M. P., and Chavali, M. S. (2019). Recent advances in biomaterials for 3D scaffolds: A review. Bioact. Mat. 4, 271–292. doi:10.1016/j.bioactmat.2019.10.005
Parisi, L., Toffoli, A., Ghiacci, G., and Macaluso, G. M. (2018). Tailoring the interface of biomaterials to design effective scaffolds. J. Funct. Biomater. 9 (3), 50. doi:10.3390/jfb9030050
Peric Kacarevic, Z., Rider, P., Alkildani, S., Retnasingh, S., Pejakic, M., Schnettler, R., et al. (2020). An introduction to bone tissue engineering. Int. J. Artif. Organs 43 (2), 69–86. doi:10.1177/0391398819876286
Popescu, R. C., Andronescu, E., Vasile, B. S., Trusca, R., Boldeiu, A., Mogoanta, L., et al. (2017). Fabrication and cytotoxicity of gemcitabine-functionalized magnetite nanoparticles. Molecules 22 (7), 1080. doi:10.3390/molecules22071080
Qi, J., Yu, T., Hu, B., Wu, H., and Ouyang, H. (2021). Current biomaterial-based bone TissueEngineering and translational medicine. Int. J. Mol. Sci. 22 (19), 10233. doi:10.3390/ijms221910233
Quadros, M., Momin, M., and Verma, G. (2021). Design strategies and evolving role of biomaterial assisted treatment of osteosarcoma. Mater. Sci. Eng. C 121, 111875. doi:10.1016/j.msec.2021.111875
Sarkar, N., and Bose, S. (2020). Controlled release of soy isoflavones from multifunctional 3D printed bone tissue engineering scaffolds. Acta Biomater. 114, 407–420. doi:10.1016/j.actbio.2020.07.006
Sedighi, O., Alaghmandfard, A., Montazerian, M., and Baino, F. (2021). A critical review of bioceramics for magnetic hyperthermia. J. Am. Ceram. Soc. 105, 1723–1747. doi:10.1111/jace.17861
Sibata, C. H., Colussi, V. C., Oleinick, N. L., and Kinsella, T. J. (2000). Photodynamic therapy: A new concept in medical treatment. Braz. J. Med. Biol. Res. 33 (8), 869–880. doi:10.1590/s0100-879x2000000800002
Suksiriworapong, J., Taresco, V., Ivanov, D. P., Styliari, I. D., Sakchaisri, K., Junyaprasert, V. B., et al. (2018). Synthesis and properties of a biodegradable polymer-drug conjugate: Methotrexate-poly(glycerol adipate). Colloids Surfaces B Biointerfaces 167, 115–125. doi:10.1016/j.colsurfb.2018.03.048
Tan, B., Tang, Q., Zhong, Y., Wei, Y., He, L., Wu, Y., et al. (2021). Biomaterial-based strategies for maxillofacial tumour therapy and bone defect regeneration. Int. J. Oral Sci. 13 (1), 9. doi:10.1038/s41368-021-00113-9
Tang, G., Liu, Z., Liu, Y., Yu, J., Wang, X., Tan, Z., et al. (2021). Recent trends in the development of bone regenerative biomaterials. Front. Cell Dev. Biol. 9, 665813. doi:10.3389/fcell.2021.665813
Tevlin, R., McArdle, A., Atashroo, D., Walmsley, G. G., Senarath-Yapa, K., Zielins, E. R., et al. (2014). Biomaterials for craniofacial bone engineering. J. Dent. Res. 93 (12), 1187–1195. doi:10.1177/0022034514547271
Turnbull, G., Clarke, J., Picard, F., Riches, P., Jia, L., Han, F., et al. (2018). 3D bioactive composite scaffolds for bone tissue engineering. Bioact. Mat. 3 (3), 278–314. doi:10.1016/j.bioactmat.2017.10.001
Vats, A., Tolley, N. S., Polak, J. M., and Buttery, L. D. (2002). Stem cells: Sources and applications. Clin. Otolaryngol. 27 (4), 227–232. doi:10.1046/j.1365-2273.2002.00579.x
Wang, S. Y., Hu, H. Z., Qing, X. C., Zhang, Z. C., and Shao, Z. W. (2020). Recent advances of drug delivery nanocarriers in osteosarcoma treatment. J. Cancer 11 (1), 69–82. doi:10.7150/jca.36588
Wu, H., Luo, Y., Xu, D., Ke, X., and Ci, T. (2020). Low molecular weight heparin modified bone targeting liposomes for orthotopic osteosarcoma and breast cancer bone metastatic tumors. Int. J. Biol. Macromol. 164, 2583–2597. doi:10.1016/j.ijbiomac.2020.08.068
Wu, V. M., Mickens, J., and Uskokovic, V. (2017). Bisphosphonate-functionalized hydroxyapatite nanoparticles for the delivery of the bromodomain inhibitor JQ1 in the treatment of osteosarcoma. ACS Appl. Mat. Interfaces 9 (31), 25887–25904. doi:10.1021/acsami.7b08108
Xi, Y., Jiang, T., Yu, Y., Yu, J., Xue, M., Xu, N., et al. (2019). Dual targeting curcumin loaded alendronate-hyaluronan- octadecanoic acid micelles for improving osteosarcoma therapy. Int. J. Nanomedicine 14, 6425–6437. doi:10.2147/IJN.S211981
Yin, J., Pan, S., Guo, X., Gao, Y., Zhu, D., Yang, Q., et al. (2021). Nb2C MXene-functionalized scaffolds enables osteosarcoma phototherapy and angiogenesis/osteogenesis of bone defects. Nanomicro. Lett. 13 (1), 30. doi:10.1007/s40820-020-00547-6
Yin, X., Chi, Y., Guo, C., Feng, S., Liu, J., Sun, K., et al. (2017). Chitooligosaccharides modified reduction-sensitive liposomes: Enhanced cytoplasmic drug delivery and osteosarcomas-tumor inhibition in animal models. Pharm. Res. 34 (10), 2172–2184. doi:10.1007/s11095-017-2225-0
Yu, K., Liang, B., Zheng, Y., Exner, A., Kolios, M., Xu, T., et al. (2019). PMMA-Fe3O4 for internal mechanical support and magnetic thermal ablation of bone tumors. Theranostics 9 (14), 4192–4207. doi:10.7150/thno.34157
Yu, L., and Ding, J. (2008). Injectable hydrogels as unique biomedical materials. Chem. Soc. Rev. 37 (8), 1473–1481. doi:10.1039/b713009k
Zhang, W., Gu, J., Li, K., Zhao, J., Ma, H., Wu, C., et al. (2019). A hydrogenated black TiO2 coating with excellent effects for photothermal therapy of bone tumor and bone regeneration. Mater. Sci. Eng. C 102, 458–470. doi:10.1016/j.msec.2019.04.025
Zhang, Y., Wang, F., Li, M., Yu, Z., Qi, R., Ding, J., et al. (2018). Self-stabilized hyaluronate nanogel for intracellular codelivery of doxorubicin and cisplatin to osteosarcoma. Adv. Sci. (Weinh). 5 (5), 1700821. doi:10.1002/advs.201700821
Zhao, P.-P., Ge, Y.-W., Liu, X.-L., Ke, Q.-F., Zhang, J.-W., Zhu, Z.-A., et al. (2020). Ordered arrangement of hydrated GdPO4 nanorods in magnetic chitosan matrix promotes tumor photothermal therapy and bone regeneration against breast cancer bone metastases. Chem. Eng. J. 381, 122694. doi:10.1016/j.cej.2019.122694
Keywords: bone tumor, biomaterial, bone regeneration, drug delivery, hyperthermia therapy
Citation: Zhang Y, Wu Y, Qiao X, Lin T, Wang Y and Wang M (2022) Biomaterial-based strategy for bone tumor therapy and bone defect regeneration: An innovative application option. Front. Mater. 9:990931. doi: 10.3389/fmats.2022.990931
Received: 11 July 2022; Accepted: 17 August 2022;
Published: 07 September 2022.
Edited by:
Lia Rimondini, University of Eastern Piedmont, ItalyReviewed by:
Ksenia Menshikh, Università del Piemonte Orientale, ItalyCopyright © 2022 Zhang, Wu, Qiao, Lin, Wang and Wang. This is an open-access article distributed under the terms of the Creative Commons Attribution License (CC BY). The use, distribution or reproduction in other forums is permitted, provided the original author(s) and the copyright owner(s) are credited and that the original publication in this journal is cited, in accordance with accepted academic practice. No use, distribution or reproduction is permitted which does not comply with these terms.
*Correspondence: Meng Wang, d2FuZ21lbmdAaHJibXUuZWR1LmNu
†These authors have contributed equally to this work
Disclaimer: All claims expressed in this article are solely those of the authors and do not necessarily represent those of their affiliated organizations, or those of the publisher, the editors and the reviewers. Any product that may be evaluated in this article or claim that may be made by its manufacturer is not guaranteed or endorsed by the publisher.
Research integrity at Frontiers
Learn more about the work of our research integrity team to safeguard the quality of each article we publish.