- 1Department of Physics, Lab of 2D Materials and Quantum Devices, School of Physics, Hefei University of Technology, Hefei, China
- 2School of Microelectronics, Hefei University of Technology, Hefei, China
- 3Department of Physics, University of Science and Technology of China, Hefei, China
- 4National Synchrotron Radiation Laboratory, University of Science and Technology of China, Hefei, China
- 5School of Physical Sciences, University of Chinese Academy of Sciences, Beijing, China
In this work, we investigate the influence of electric fields (E-fields) on the room-temperature magnetotransport behavior of an artificial multiferroic heterostructure, a Conetic alloy (Ni77Fe14Cu5Mo4) thin film/Pb(Mg1/3Nb2/3)0.7Ti0.3O3 (011). When the external magnetic field is parallel to the applied current, the switching field increases from 0.8 to 3.3 Oe at 0 and 8 kV/cm, respectively, and the corresponding magnetoresistance (MR) ratio at 20 Oe respectively decreases from 0.14% to 0.03% at 0 and 8 kV/cm. However, when the external magnetic field is perpendicular to the current, the switching field decreases from 10.1 to 1.7 Oe at 0 and 8 kV/cm, and the MR ratio in such a case decreases from −0.001% to −0.10%, respectively. Consequently, under the parallel and perpendicular modes, the tunabilities of the switching field are approximately +313% and −83%, and the MR ratio tunabilities under E-fields are approximately −79% and +9,900%, respectively. Such a large and anisotropic tunability of both the switching field and MR ratio is attributed to the ultrasoft magnetic property of the Conetic alloy thin film and anisotropic in-plane strain-mediated magnetoelectric coupling. However, the anisotropic MR ratio is approximately 0.15% and does not vary with the applied E-fields owing to the intrinsic property of Conetic thin films using transfer and circle transfer curve measurements, rather than the magnetization rotation caused by E-field-induced magnetoelastic anisotropy. This work demonstrates that multiferroic heterostructures with electrically tunable MR show considerable potential in designing energy-efficient electronic and spintronic devices.
Introduction
Writing binary information into magnetic storage devices involves switching the magnetization orientation of magnetic storage units (Brataas et al., 2012). In information storage, electric field (E-field)-controlled magnetism is received widespread attention because it can effectively reduce power consumption during information-writing processes using an E-field strategy (Liu and Sun, 2014; Matsukura et al., 2015; Kumar et al., 2020; Ali et al., 2022). The mechanism by which E-fields regulate magnetism mainly involves five aspects: carrier density (Weisheit et al., 2007), strain (Chen et al., 2019), orbital coupling (Peng et al., 2015), exchange coupling (Wang et al., 2018a), and ion effect (Tan et al., 2019). Among these strategies, strain-driven magnetization switching based on magnetoelectric coupling in composite multiferroic heterostructures is considerably more attractive and has been studied extensively (Motti et al., 2020; Chen et al., 2021; Yang et al., 2021). In such heterostructures, the dynamic strain of the ferroelectric (FE) or piezoelectric component is induced by the converse piezoelectric effect under applied external E-fields and then transferred to the adjacent ferromagnetic layer (Eerenstein et al., 2006). Consequently, the magnetic and electrical properties of composite multiferroic heterostructures, such as coercivity (Tkach et al., 2015), magnetic anisotropy (Wang et al., 2019), magnetization orientation (Wang et al., 2018b), and magnetoresistance (MR), can be controlled using E-fields through the converse magnetostriction effect (Jahjah et al., 2020; Yamada et al., 2021). Recently, Yu et al. (2019) found that the lattice distortion caused by residual compressive strain can induce perpendicular magnetic anisotropy in Co2MnAl films. Zhou et al. (2021) reported an effective in-plane magnetization 90° rotation induced by the piezo-strain effect in the Co2FeAl/Pb(Mg1/3Nb2/3)0.7Ti0.3O3 (PMN–PT) structure. Yamada et al. (2021) observed that the presence of the magnetoelastic effect owing to spin–orbit interactions at the epitaxial interface between Co2FeSi and ferroelectric BaTiO3 (001) layers. These studies show great potential for realizing energy-efficient and compact spintronic, and microwave devices using strain-mediated composite multiferroic heterostructures.
In modern spintronics and microelectronics, the MR effects, such as tunneling MR, anisotropic MR (AMR), and giant MR (GMR), have been widely employed in the field of information storage and sensors (Liu and Sun, 2014; Hu and Nan, 2019; Luo et al., 2020). However, in such devices, magnetization orientation and MR modulation are realized under current-induced magnetic fields using a bulky and energy-consumed electromagnet, which will remarkably limit the development of small and ultralow-power spintronic and electronic devices in the future (Hu and Nan, 2019; Wang et al., 2020; Herrera Diez et al., 2021; Ma et al., 2022). Alternatively, by exploiting composite multiferroic heterostructures, the magnetization and subsequent MR can be controlled using E-fields rather than magnetic fields, facilitating the availability of energy-efficient spintronic and electronic devices. Recently, numerous composite multiferroic heterostructures have been proposed and their MR properties have been studied, particularly in Ni-based multiferroic heterostructures. Nan et al. (2015) reported that combined charge and strain-mediated magnetoelectric coupling in an ultrathin NiFe/PMN–PT multiferroic heterostructure, affording the E-field control of reversible and nonvolatile magnetic switching. Gao et al. (2014) studied the voltage-controlled magnetism of the Ni77Fe23/PMN–PT (011) multiferroic heterostructure through MR measurement and theoretical analysis. Hong et al. (2016) developed an angle-dependent MR measurement method to study the magnetization rotation in the Ni/SiO2/Ti/(011)-PMN–PT multiferroic heterostructures. Subsequently, Yang et al. (2017) then used this method to quantify the rotation angle of magnetic anisotropy under the coaction of in situ E-fields and magnetic fields in the Ni/(011)-PMN–0.3PT multiferroic heterostructures. Xiong et al. (2018) proved that the Ni81Fe19-based multiferroic heterostructure can also achieve a large magnetoelectric coupling at room temperature, yielding a tunability of magnetic anisotropy up to 332 fJ/Vm. Du et al. (2020) observed a large, nonvolatile, and tunable magnetization switching in the Ni80Co20 alloy film/PMN–PT multiferroic heterostructure at room temperature. These great achievements in Ni-based magnetic films/ferroelectrics multiferroic heterostructures would enable a promising application in novel spintronic devices.
Motivated by aforementioned achievements in the Ni-based magnetic alloys for multiferroic heterostructures, we explored a well-known mu-metal series of ultrasoft magnetic material (a typical representative Conetic alloy: Ni77Fe14Cu5Mo4) as a ferromagnetic component to create Conetic alloy thin film/PMN–PT multiferroic heterostructures. The Ni77Fe14Cu5Mo4 alloy exhibit relatively smaller easy-axis (e.a.) coercivity, larger hard-axis magnetic susceptibility and higher attenuation (Choi et al., 2010; Lei et al., 2011). Consequently, they are widely used in many spintronic devices as sensing or composite free layers in AMR sensors for hysteresis-free and linear field responses and magnetic tunnel junctions for large TMR ratio (Egelhoff et al., 2010). Therefore, aiming to develop novel spintronic materials for technical applications, we fabricated Conetic alloy thin films using magnetron sputter technique and studied E-field control of MR and magnetization switching behavior using transfer curve (TC) and circle transfer curve (CTC) measurements in the Ni77Fe14Cu5Mo4/(011)-PMN–PT multiferroic heterostructure. We found that switching fields and the MR ratio were anisotropically controlled by E-fields with a giant tunability. Such a Conetic alloy thin film/PMN–PT multiferroic heterostructure demonstrates strong magnetoelectric coupling, regardless of the weak magnetostriction in the Conetic alloy thin film, showing its potential in ultralow-power spintronic devices.
Experimental Details
We deposited Conetic alloy (Ni77Fe14Cu5Mo4) thin films on the (011)-cut PMN–PT substrates using a custom multi-target high-vacuum magnetron sputtering system (base pressure better than 4.5 × 10–8 Torr) (Egelhoff et al., 2010). The sputter power was set to 15 W. The growth environment comprised a constant Ar pressure of 2.3 mTorr. The film thickness was calibrated to be 10 nm by carefully controlling the deposition time. During the sputtering process, the substrates were rotated at a constant speed to ensure uniformity throughout the sample. Then the Cu pads were deposited ex situ at room temperature using the e-beam method by employing a four-probe shadow mask. For magnetoresistance measurements, the magnetic field scanning rate was 2 s per step for one recording data, while E-fields were still maintained along the thickness direction of the PMN–PT. The minimum resistance of the PMN–PT substrate was 2 G·Ω during the scanning of E-fields so that the leakage current could be ignored during magnetotransport measurements. (Zhang et al., 2011).
Results and Discussion
Device Architecture and Magnetotransport Properties
Figure 1A presents a schematic of the Conetic alloy thin-film/PMN–PT multiferroic heterostructure device used for the measurement. The e.a. was set along the [100] direction of the PMN–PT substrate using magnetic thermal annealing treatment (Yang et al., 2021). MR was measured using a four-probe technique with current I (black solid line) along the [01
where R∥ and R⊥ are the maximal and minimal MR values when the magnetic fields are parallel and perpendicular to I, respectively (Aharoni et al., 2000). Therefore, the AMR ratio measured at the initial state (i.e., unpolarized state) is ∼0.15%, comparable to the value of ∼0.85% for a 10-nm-thick Ni80Fe20 thin film on a (100) p-Si substrate (Kateb and Ingvarsson, 2017) and ∼0.48% for a 20-nm-thick Ni81Fe19/glass thin film (Wang et al., 2013). The relatively slight decrease in the AMR ratio in the Ni77Fe14Cu5Mo4 thin films is considerably attributed to the vacuum pressure, temperature and time in the annealing treatment (Zhao et al., 2013), which will be investigated in the future.
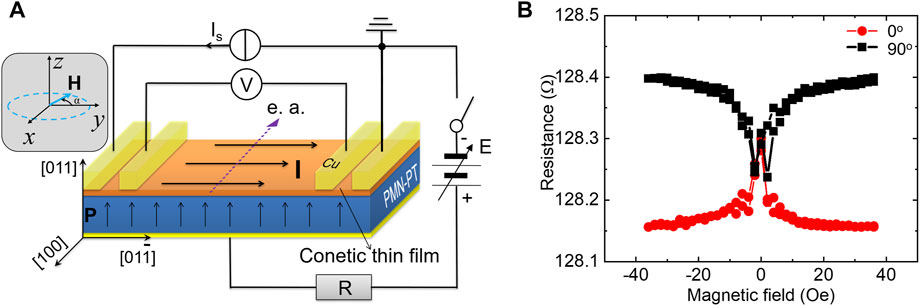
FIGURE 1. (A) Schematic of a Conetic alloy thin film/PMN–PT multiferroic heterostructure. The four-probe MR measurement and in situ E-field application are also shown here. (B) MR as a function of the applied in-plane magnetic field H toward α = 0° and 90°. Herein, α is the angle between the in-plane magnetic field and y-axis (or current I), as illustrated in the upper left corner of Figure 1. Magnetic field H scans back and forth between −36 and +36 Oe. Herein, the ferroelectric substrate PMN-PT was in a unpolarized state.
E-Field Control of Switching Field
To study the influence of E-fields on the magnetotransport characteristics of the Conetic alloy thin film/PMN–PT multiferroic heterostructures, MR was measured along the anisotropic [100] and [01
where Hpara (8 kV/cm) and Hpara (0 kV/cm) are the switching fields at 8 and 0 kV/cm, respectively. In the perpendicular mode (inset of Figure 2C), the MR decreases, showing a negative MR effect with increasing magnetic fields. This result is attributed to the AMR effect (Hong et al., 2016). When increasing the E-field, the amount of resistance change increases, yielding an obvious bimodal hysteresis curve (Figure 2C). Accordingly, the switching field Hperp (the subscript refers to the perpendicular mode) as a function of the E-field is shown in Figure 2D. The switching field decreases from ∼10.1 to 1.7 Oe at 0 and 8 kV/cm, respectively. Consequently, the tunability of the switching field induced by E-fields is up to −83% in the perpendicular mode. These results show that the E-field can considerably modulate the switching field of the Conetic alloy thin film/PMN–PT multiferroic heterostructure. In particular, the switching field shows anisotropic tunability when the magnetic field is along the [100] and [01
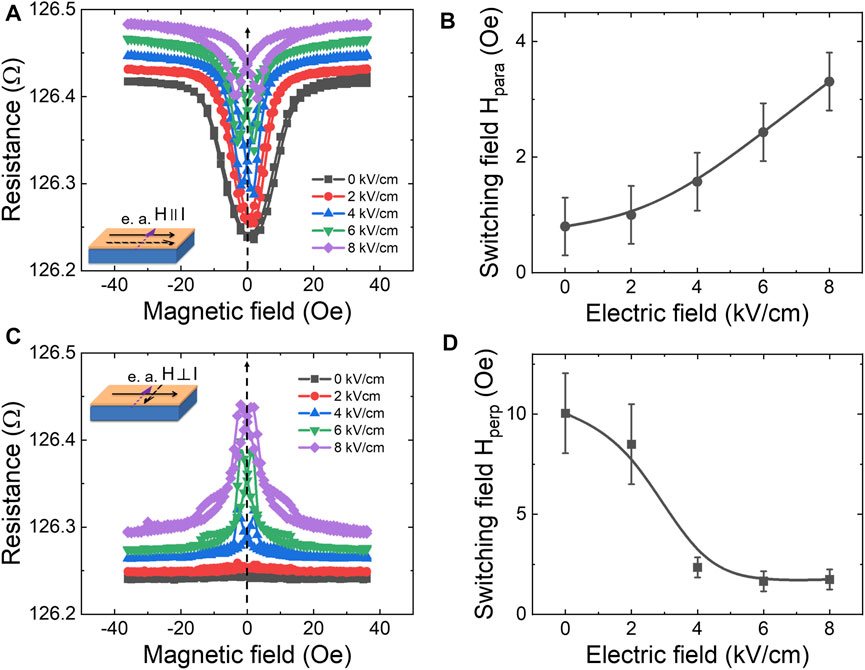
FIGURE 2. (A) and (C) Magnetoresistance in the Conetic alloy thin film/PMN–PT multiferroic heterostructure as a function of the applied magnetic field with different bias E-fields. Magnetic field H (black dotted line) is parallel and perpendicular to current I (black solid line) in the inset of Panels (A,C), respectively. (B) and (D) Dependence of the switching field on the E-fields in the Conetic alloy thin film/PMN–PT multiferroic heterostructure when the magnetic fields are along [01
E-Field Control of Magnetoresistance
We also extract the MR dependence on E-fields using Figures 2A,C. As shown in Figures 3A,B, the parallel MR ratio (i.e., in the parallel mode) is defined as (Zhou et al., 2017)
where
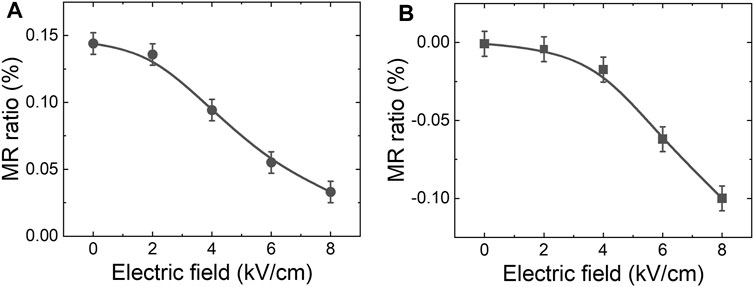
FIGURE 3. (A) and (B) Magnetoresistance ratio under parallel and perpendicular modes, respectively. Herein, magnitude of the magnetic field is set to ∼20 Oe to illustrate the E-field modulation of spin-dependent transport behavior.
As mentioned above, MR ratio under weak magnetic field bias can be remarkably modulated by E-fields. Now we turn to examine the AMR ratio controlled by E-fields under large and even saturated magnetic field bias. Figure 4A shows circle transfer curves (CTCs) under a saturated magnetic field (H = 35 Oe). According to R⊥ and R∥ value as labelled in Figure 4A, we can calculate the AMR ratio using formula (1). The corresponding AMR ratio is summarized in Figure 4B as shown by black line. Additionally, we also check the AMR ratio marked by blue line in Figure 4B using the MR data, which is extracted from TC measurements at 0, 2, 4, 6, and 8 kV/cm as previously shown in Figures 2A,C. On the one hand, these two methods are in good agreement. On the other hand, the AMR ratio barely changes within the tolerance range as the E-field increases. The corresponding average value of the AMR ratio is approximately 0.15%. Currently, the widely accepted physical mechanism is that the AMR effect is an intrinsic material property owing to the anisotropic scattering of the conducted electrons and local d electrons in magnetic alloy (Potter, 1974; Chattopadhyay et al., 2002). Therefore, the strain-mediated magnetoelectric coupling in our multiferroic heterostructure can modulate the switching field and MR behavior at relatively small magnetic field bias, rather than tune the AMR ratio at large and even saturated magnetic field bias, which is also observed in Co/PMN–PT (011) and Ni80Co20/PZN-PT (011) multiferroic heterostructures (Liu et al., 2011; Zhou et al., 2017). It is mentionable that the ferroelectric polarization induced interfacial charge has no effects on the E-field control of switching fields and MR behaviors due to the short charge-screening length of the metallic ferromagnet Conetic films studied here (Hu and Nan, 2019).
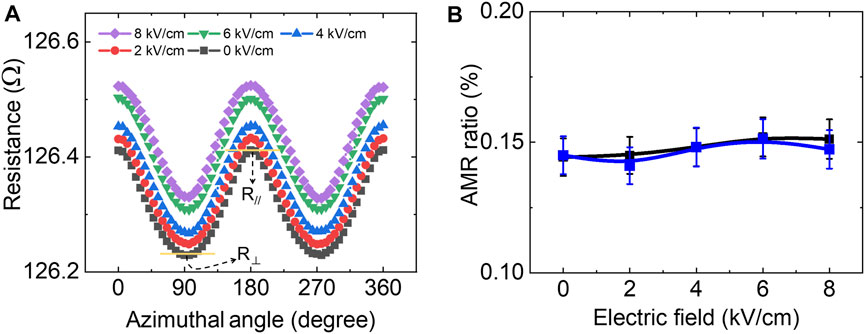
FIGURE 4. (A) Circle transfer curves under different E-field bias. The saturated magnetic field ∼35 Oe was chosen to perform this measurement. R⊥ and R∥ is minimal and maximal magnetoresistance value in the CTCs, respectively. (B) AMR ratio is as a function of the applied electric fields. The black and blue symbols represent the AMR ratio derived by TC and CTC measurements, respectively.
Mechanism of E-Field Control of Switching Field and Magnetoresistance
In order to reveal mechanism of E-field control of switching fields and magnetoresistance behavior (Yang et al., 2021), CTC mappings are performed by scanning magnetic fields from 5 to 35 Oe in a step 5 Oe under E-fields of 0, 2, 4, 6, and 8 kV/cm as shown in Figures 5A–E, respectively. First, considering any of the data plots in Figure 5, the azimuthal position of the maximal value of the MR does not change as marked by dashed lines, either at 0o or at 180o. It is almost the same as the magnetic field rotation direction, strongly indicating ultrasoft magnetic properties of the Conetic thin films (Lei et al., 2011). Second, comparing the CTC mapping results under different E-fields, the applied electric field makes the contour darker, that is, the MR becomes larger, which is also consistent with the TC measurements. Additionally, the position of the maximal magnetoresistance does not move when the electric field is applied, implying that the direction of E-field-induced magnetoelastic anisotropy is exactly along the [01
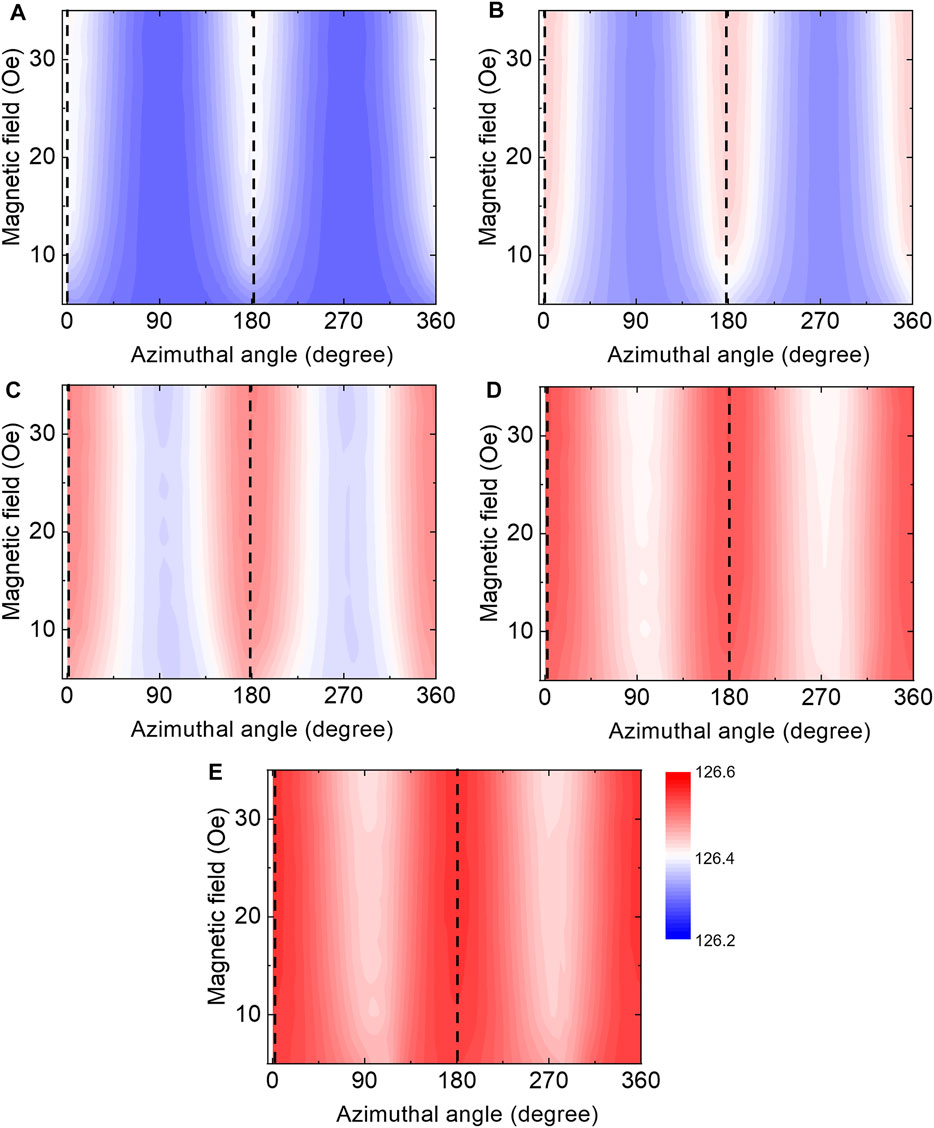
FIGURE 5. (A–E) CTC mappings under different E-field bias, i.e., 0, 2, 4, 6, and 8 kV/cm, respectively. The dashed lines show the angular position of applied magnetic fields with maximum magnetoresistance values. All figures use same contour scale with unit Ω.
According to the above experimental results, we further phenomenologically analyze magnetization rotation through energy competitions among the Zeeman, uniaxial magnetic anisotropic, and magnetoelastic anisotropic energies induced by E-fields in a single-domain mode. As shown in Figures 6A,B, when an E-field is applied to the FE layer, an anisotropic in-plane strain is generated [compressive strain (σx < 0) along the [100] direction and tensile strain (σy > 0) along the [01
where φ represents the angle between the magnetization and strain direction of σx (Figure 6). λs (>0) represents the saturated magnetostriction coefficient of the Conetic alloy thin film (Klokholm and Aboaf, 1981; Egelhoff et al., 2006). To accommodate the E-field-induced magnetoelastic anisotropy energy, the magnetization seeks the lowest energy orientation on the free energy surface (Liu et al., 2010). Qualitatively, the lowest magnetoelastic energy can be obtained as a product of magnetoelastic energy terms λsσx and λsσy. Assuming that the in-plane anisotropic strain in the PMN–PT layer can be completely transferred to the Conetic alloy thin film, the magnetoelastic energy terms λsσx and λsσy under a nonzero unipolar E-field are negative and positive along the [100] and [01
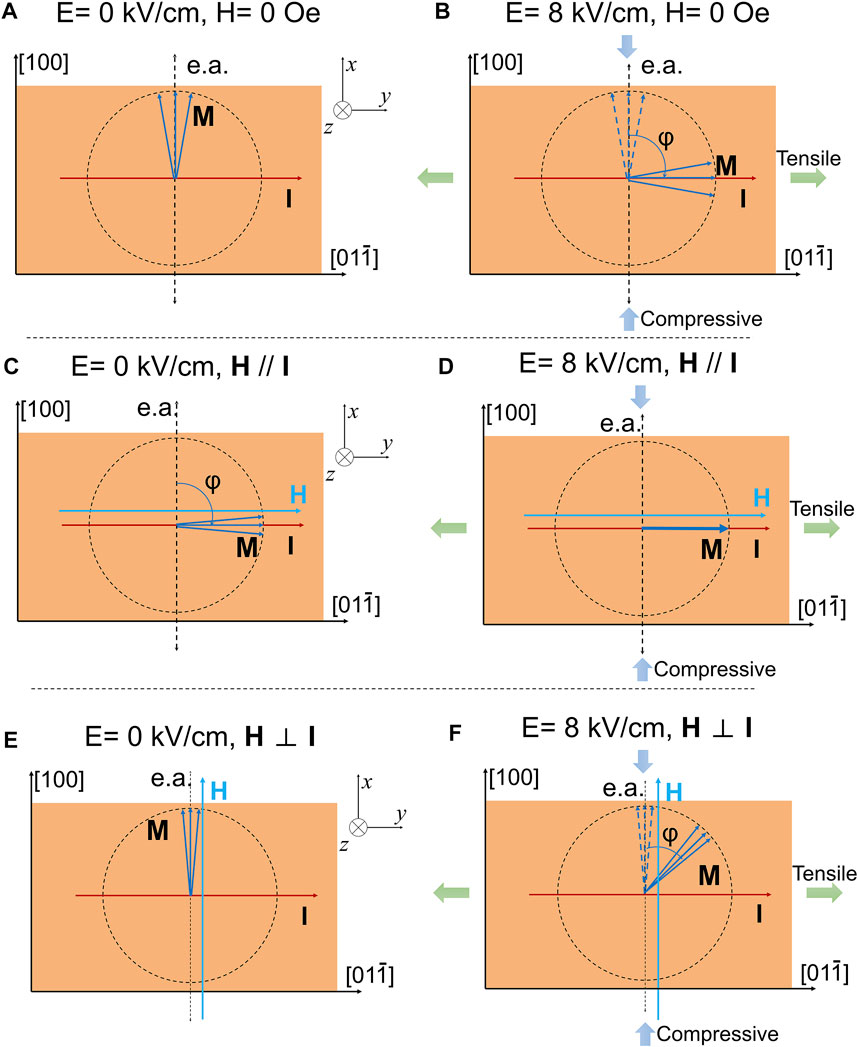
FIGURE 6. (A) Schematic of the initial magnetic state of the Conetic alloy thin film/PMN–PT multiferroic heterostructure. (B) Schematic of magnetization rotation induced by E-field-induced tensile and compressive strains without magnetic field bias. Wide, light blue and green arrows represent in-plane anisotropic compressive and tensile strains, respectively. (C) and (D) Schematics of magnetic states at 0 and 8 kV/cm, respectively, with the magnetic field perpendicular to current (I). (E) and (F) Schematics of magnetic states at 0 and 8 kV/cm, respectively, with the magnetic field parallel to current (I). Light blue, dark blue and dark red arrows represent magnetic field H, magnetization M and applied current I, respectively. The thick, dark blue arrow in Panel (D) means that the magnetization is almost aligned along the [01
Furthermore, in the parallel mode, the applied magnetic field will rotate the magnetization and distribute spatially it along the [01
Conclusion
In conclusion, to develop novel spintronic materials for technical applications, we fabricated a Conetic alloy thin film/PMN–PT multiferroic heterostructure. We observed that the magnetotransport properties are considerably modulated by the E-fields. The tunabilities of the switching field are approximately +313% and −83% under the parallel and perpendicular modes, respectively. Furthermore, the tunability of the MR ratio induced by the E-field is respectively up to approximately −79% and +9,900% under the parallel and perpendicular modes. These results indicate a large and anisotropic tunability of both the switching field and MR ratio because of the change in the magnetization orientation induced by anisotropic in-plane strain-mediated magnetoelectric coupling and ultrasoft magnetic property of the Conetic alloy thin film. However, the AMR ratio is almost unaffected by the E-fields because the AMR effect is an intrinsic property of the Conetic alloy thin film. This work deepens the understanding of the electrical manipulation of magnetic anisotropy in the multiferroic heterostructure. Furthermore, the E-field control of the switching field and MR offers a promising opportunity for further optimizing the Conetic alloy-based spintronic devices in the future.
Data Availability Statement
The original contributions presented in the study are included in the article/Supplementary Material, further inquiries can be directed to the corresponding authors.
Author Contributions
YY and CG conceptualized this work. CaW, WH, ChW, and WG fabricated the samples. CaW, LL, CoW, and YS performed magnetoresistance measurements and collected experimental data. XM, QL, CJ, HZ, and ZL conducted data analysis and discussed with other authors. YY, HZ, and CG looked for funding acquisition for this work. CaW wrote the paper, and all authors revised it.
Funding
This work was mainly funded from the National Natural Science Foundation of China (52072102, 11775224, 12074357, and 11804324), partially supported by the Open Foundation of the University of Science and Technology of China (KF2020002), National Key Research and Development Program of China (2017YFA0205004) and Chinese Academy of Sciences Pioneer Hundred Talents Program.
Conflict of Interest
The authors declare that the research was conducted in the absence of any commercial or financial relationships that could be construed as a potential conflict of interest.
Publisher’s Note
All claims expressed in this article are solely those of the authors and do not necessarily represent those of their affiliated organizations, or those of the publisher, the editors and the reviewers. Any product that may be evaluated in this article, or claim that may be made by its manufacturer, is not guaranteed or endorsed by the publisher.
Acknowledgments
We also gratefully acknowledge the beamlines BL07W, BL11U, and BL12B-α at the National Synchrotron Radiation Laboratory (NSRL) for the device fabrications and property characterizations.
Supplementary Material
The Supplementary Material for this article can be found online at: https://www.frontiersin.org/articles/10.3389/fmats.2022.956349/full#supplementary-material
References
Aharoni, A., Pust, L., and Kief, M. (2000). Comparing Theoretical Demagnetizing Factors with the Observed Saturation Process in Rectangular Shields. J. Appl. Phys. 87, 6564–6566. doi:10.1063/1.372771
Ali, I., Hussan, G., and Ahmed, S. (2022). Fabrication of Self-Healing Hybrid Nanogenerators Based on Polyurethane and ZnO for Harvesting Wind Energy. J. Mat. Sci. Mat. Electron 33, 8048. doi:10.1007/s10854-021-07591-x
Brataas, A., Kent, A. D., and Ohno, H. (2012). Current-induced Torques in Magnetic Materials. Nat. Mater 11, 372–381. doi:10.1038/nmat3311
Chattopadhyay, M. K., Roy, S. B., Chaudhary, S., Jeet Singh, K., and Nigam, A. K. (2002). Magnetic Response ofFe1−xCoxSialloys: A Detailed Study of Magnetization and Magnetoresistance. Phys. Rev. B 66, 174421. doi:10.1103/physrevb.66.174421
Chen, A., Piao, H., Ji, M., Fang, B., Wen, Y., Ma, Y., et al. (2021). Using Dipole Interaction to Achieve Nonvolatile Voltage Control of Magnetism in Multiferroic Heterostructures. Adv. Mat. 23, 2105902. doi:10.1002/adma.202105902
Chen, X., Zhou, X., Cheng, R., Song, C., Zhang, J., Wu, Y., et al. (2019). Electric Field Control of Néel Spin-Orbit Torque in an Antiferromagnet. Nat. Mat. 18, 931–935. doi:10.1038/s41563-019-0424-2
Choi, J.-G., Hwang, D.-G., Rhee, J.-R., and Lee, S.-S. (2010). Comparison of the Soft Magnetic Properties of Permalloy and Conetic Thin Films. J. Magnetism Magnetic Mater. 322, 2191–2194. doi:10.1016/j.jmmm.2010.02.008
Du, W., Liu, M., Su, H., Zhang, H., Liu, B., Meng, H., et al. (2020). Wide Range Voltage-Impulse-Controlled Nonvolatile Magnetic Memory in Magnetoelectric Heterostructure. Appl. Phys. Lett. 117, 222401. doi:10.1063/5.0033319
Eerenstein, W., Mathur, N. D., and Scott, J. F. (2006). Multiferroic and Magnetoelectric Materials. Nature 442, 759–765. doi:10.1038/nature05023
Egelhoff, W. F., Höink, V. E., Lau, J. W., Shen, W. F., Schrag, B. D., and Xiao, G. (2010). Magnetic Tunnel Junctions with Large Tunneling Magnetoresistance and Small Saturation Fields. J. Appl. Phys. 107, 09C705. doi:10.1063/1.3358609
Egelhoff, W. F., McMichael, R. D., Dennis, C. L., Stiles, M. D., Johnson, F., Shapiro, A. J., et al. (2006). Soft Magnetic Layers for Low-Field-Detection Magnetic Sensors. Thin Solid Films 505, 90–92. doi:10.1016/j.tsf.2005.10.014
Gao, Y., Hu, J., Shu, L., and Nan, C. W. (2014). Strain-mediated Voltage Control of Magnetism in Multiferroic Ni77Fe23/Pb(Mg1/3Nb2/3)0.7Ti0.3O3 Heterostructure. Appl. Phys. Lett. 104, 142908. doi:10.1063/1.4870975
García, N., Hao, C., Yonghua, L., Muñoz, M., Chen, Y., Cui, Z., et al. (2006). Is There Ballistic Transport in Metallic Nano-Objects? Ballistic versus Diffusive Contributions. Appl. Phys. Lett. 89, 083112. doi:10.1088/0953-8984/19/1/016212
Guo, Q., Xu, X., Wang, F., Lu, Y., Chen, J., Wu, Y., et al. (2018). In-plane Electric Field Controlled Ferromagnetism and Anisotropic Magnetoresistance in an LSMO/PMN-PT Heterostructure. Nanotechnology 29, 224003. doi:10.1088/1361-6528/aab5fb
Herrera Diez, L., Kruk, R., Leistner, K., and Sort, J. (2021). Magnetoelectric Materials, Phenomena, and Devices. Apl. Mater. 9, 050401. doi:10.1063/5.0053631
Hong, B., Yang, Y., Zhao, J., Hu, K., Peng, J., Zhang, H., et al. (2016). Quantifying Electric-Field Control of Magnetization Rotation in Ni/SiO2/Ti/(011)-PMN-PT Multiferroic Heterostructures via Anisotropic Magnetoresistance Measurements. Mater. Lett. 169, 110–113. doi:10.1016/j.matlet.2016.01.109
Hu, J.-M., and Nan, C.-W. (2019). Opportunities and Challenges for Magnetoelectric Devices. Apl. Mater. 7, 080905. doi:10.1063/1.5112089
Jahjah, W., Jay, J.-Ph., Le Grand, Y., Fessant, A., Prinsloo, A. R. E., Sheppard, C. J., et al. (2020). Electrical Manipulation of Magnetic Anisotropy in a Fe81Ga19/Pb (Mg1/3Nb2/3)O3-Pb (ZrxTi1 -x)O3 Magnetoelectric Multiferroic Composite. Phys. Rev. Appl. 13, 034015. doi:10.1103/PhysRevApplied.13.034015
Kateb, M., and Ingvarsson, S. (2017). “SAS 2017 - 2017 IEEE Sensors Applications Symposium,” in 2017 IEEE Sensors Applications Symposium (SAS), 2017.3.13-2017.3.15 (Glassboro, NJ, USA: IEEE), 1–5. doi:10.1109/SAS.2017.7894043
Klokholm, E., and Aboaf, J. A. (1981). The Saturation Magnetostriction of Permalloy Films. J. Appl. Phys. 52, 2474–2476. doi:10.1063/1.328971
Krzyk, S., Schmidsfeld, A., Kläui, M., and Rüdiger, U. (2010). Magnetotransport Effects of Ultrathin Ni80Fe20films Probedin Situ. New J. Phys. 12, 013001. doi:10.1088/1367-2630/12/1/013001
Kumar, M., Shankar, S., Kumar, A., Anshul, A., Jayasimhadri, M., and Thakur, O. P. (2020). Progress in Multiferroic and Magnetoelectric Materials: Applications, Opportunities and Challenges. J. Mat. Sci. Mat. Electron 31, 19487–19510. doi:10.1007/s10854-020-04574-2
Lei, Z. Q., Li, G. J., Egelhoff, W. F., Lai, P. T., and Pong, P. W. T. (2011). Magnetic Tunnel Junction Sensors with Conetic Alloy. IEEE Trans. Magn. 47, 714–717. doi:10.1109/tmag.2010.2104136
Liu, M., Li, S., Obi, O., Lou, J., Rand, S., and Sun, N. X. (2011). Electric Field Modulation of Magnetoresistance in Multiferroic Heterostructures for Ultralow Power Electronics. Appl. Phys. Lett. 98, 222509. doi:10.1063/1.3597796
Liu, M., Obi, O., Cai, Z., Lou, J., Yang, G., Ziemer, K. S., et al. (2010). Electrical Tuning of Magnetism in Fe3O4/PZN-PT Multiferroic Heterostructures Derived by Reactive Magnetron Sputtering. J. Appl. Phys. 107, 073916. doi:10.1063/1.3354104
Liu, M., and Sun, N. X. (2014). Voltage Control of Magnetism in Multiferroic Heterostructures. Phil. Trans. R. Soc. A 372, 20120439. doi:10.1098/rsta.2012.0439
Luo, J., Zhang, Y., Ou-Yang, J., and Yang, X. (2020). Electric Field-Controlled Anisotropic Magnetoresistance Effect with Four-Fold-Symmetric Magnetic Anisotropy. Ceram. Int. 46, 13732–13736. doi:10.1016/j.ceramint.2020.02.161
Ma, M., Tao, Z., Ren, X., Cao, H., Yin, X., Han, Y., et al. (2022). Electric Field Manipulation of Transport Properties for Ultra-thin Fe70Ga30 Films on BaZr0.2Ti0.8O3-0.5Ba0.7Ca0.3TiO3 Films. J. Mat. Sci. Mat. Electron 33, 7995–8002. doi:10.1007/s10854-022-07950-2
Matsukura, F., Tokura, Y., and Ohno, H. (2015). Control of Magnetism by Electric Fields. Nat. Nanotech 10, 209–220. doi:10.1038/nnano.2015.22
Motti, F., Vinai, G., Bonanni, V., Polewczyk, V., Mantegazza, P., Forrest, T., et al. (2020). Interplay between Morphology and Magnetoelectric Coupling in Fe/PMN-PT Multiferroic Heterostructures Studied by Microscopy Techniques. Phys. Rev. Mater. 4, 114418. doi:10.1103/physrevmaterials.4.114418
Nan, T., Zhou, Z., Liu, M., Yang, X., Gao, Y., Assaf, B. A., et al. (2015). Quantification of Strain and Charge Co-mediated Magnetoelectric Coupling on Ultra-thin Permalloy/PMN-PT Interface. Sci. Rep. 4, 3688. doi:10.1038/srep03688
Peng, J., Song, C., Li, F., Cui, B., Mao, H., Wang, Y., et al. (2015). Charge Transfer and Orbital Reconstruction in Strain-Engineered (La,Sr)MnO3/LaNiO3 Heterostructures. ACS Appl. Mat. Interfaces 7, 17700–17706. doi:10.1021/acsami.5b04994
Potter, R. I. (1974). Magnetoresistance Anisotropy in Ferromagnetic NiCu Alloys. Phys. Rev. B 10, 4626–4636. doi:10.1103/physrevb.10.4626
Rüffer, D., Slot, M., Huber, R., Schwarze, T., Heimbach, F., Tütüncüoglu, G., et al. (2014). Anisotropic Magnetoresistance of Individual CoFeB and Ni Nanotubes with Values of up to 1.4% at Room Temperature. Apl. Mater 2, 076112. doi:10.1063/1.4891276
Tan, A. J., Huang, M., Avci, C. O., Büttner, F., Mann, M., Hu, W., et al. (2019). Magneto-ionic Control of Magnetism Using a Solid-State Proton Pump. Nat. Mater 18, 35–41. doi:10.1038/s41563-018-0211-5
Tkach, A., Kehlberger, A., Büttner, F., Jakob, G., Eisebitt, S., and Kläui, M. (2015). Electric Field Modification of Magnetotransport in Ni Thin Films on (011) PMN-PT Piezosubstrates. Appl. Phys. Lett. 106, 062404. doi:10.1063/1.4907775
Wang, C., Pu, J., Hu, Z., Su, W., Guan, M., Peng, B., et al. (2019). Electric Field Tuning of Anisotropic Magnetoresistance in Ni-Co/PMN-PT Multiferroic Heterostructure. IEEE Trans. Magn. 55, 1–3. doi:10.1109/tmag.2018.2865250
Wang, J., Huang, Q. K., Lu, S. Y., Tian, Y. F., Chen, Y. X., Bai, L. H., et al. (2018). Reversible Electrical-Field Control of Magnetization and Anomalous Hall Effect in Co/PMN-PT Hybrid Heterostructures. Appl. Phys. Lett. 112, 152904. doi:10.1063/1.5022381
Wang, S., Gao, T., Wang, C., and He, J. (2013). Studies of Anisotropic Magnetoresistance and Magnetic Property of Ni81Fe19 Ultra-thin Films with the Lower Base Vacuum. J. Alloys Compd. 554, 405–407. doi:10.1016/j.jallcom.2012.12.004
Wang, X., Yang, Q., Wang, L., Zhou, Z., Min, T., Liu, M., et al. (2018). E-Field Control of the RKKY Interaction in FeCoB/Ru/FeCoB/PMN-PT (011) Multiferroic Heterostructures. Adv. Mat. 30, 1803612. doi:10.1002/adma.201803612
Wang, Y., Sun, J., Shimada, T., Hirakata, H., Kitamura, T., and Wang, J. (2020). Ferroelectric Control of Magnetic Skyrmions in Multiferroic Heterostructures. Phys. Rev. B 102, 014440. doi:10.1103/physrevb.102.014440
Weisheit, M., Fähler, S., Marty, A., Souche, Y., Poinsignon, C., and Givord, D. (2007). Electric Field-Induced Modification of Magnetism in Thin-Film Ferromagnets. Science 315, 349–351. doi:10.1126/science.1136629
Xiong, R., Zhang, W., Fang, B., Li, G., Li, Z., Zeng, Z., et al. (2018). Ferromagnetic Resonance Manipulation by Electric Fields in Ni81Fe19/Bi3.15Nd0.85Ti2.99Mn0.01O12 Multiferroic Heterostructures. Appl. Phys. Lett. 113, 172407. doi:10.1063/1.5047066
Yamada, S., Teramoto, Y., Matsumi, D., Kepaptsoglou, D., Azaceta, I., Murata, T., et al. (2021). Electric Field Tunable Anisotropic Magnetoresistance Effect in an Epitaxial Co2 Fe Si/Ba TiO3 Interfacial Multiferroic System. Phys. Rev. Mat. 5, 014412. doi:10.1103/physrevmaterials.5.014412
Yang, Y., Huang, H., Luo, Z., Gao, C., Li, X., and Tao, C. F. (2017). Electric-field Control of Magnetic Anisotropy Rotation in Multiferroic Ni/(011)-Pb(Mg2/3Nb1/3)0.7Ti0.3O3 Heterostructures. J. Appl. Phys. 122, 134105. doi:10.1063/1.4990874
Yang, Y., Luo, Z., Wang, S., Huang, W., Wang, G., Wang, C., et al. (2021). Electric-field-assisted Non-volatile Magnetic Switching in a Magnetoelectronic Hybrid Structure. iScience 24, 102734. doi:10.1016/j.isci.2021.102734
Yu, Z.-F., Lu, J., Wang, H.-L., Zhao, X.-P., Wei, D.-H., Ma, J.-L., et al. (2019). Tunable Perpendicular Magnetic Anisotropy in Off-Stoichiometric Full-Heusler Alloy Co2MnAl*. Chin. Phys. Lett. 36, 067502. doi:10.1088/0256-307x/36/6/067502
Zhang, S., Zhao, Y. G., Li, P. S., Yang, J. J., Rizwan, S., Zhang, J. X., et al. (2012). Electric-Field Control of Nonvolatile Magnetization inCo40Fe40B20/Pb(Mg1/3Nb2/3)0.7Ti0.3O3Structure at Room Temperature. Phys. Rev. Lett. 108, 137203. doi:10.1103/physrevlett.108.137203
Zhang, S., Zhao, Y., Xiao, X., Wu, Y., Rizwan, S., Yang, L., et al. (2015). Giant Electrical Modulation of Magnetization in Co40Fe40B20/Pb(Mg1/3Nb2/3)0.7Ti0.3O3(011) Heterostructure. Sci. Rep. 4, 3727. doi:10.1038/srep03727
Zhang, W., Hao, Q., and Xiao, G. (2011). Low-frequency Noise in Serial Arrays of MgO-Based Magnetic Tunnel Junctions. Phys. Rev. B 84, 094446. doi:10.1103/physrevb.84.094446
Zhao, C.-J., Ding, L., HuangFu, J.-S., Zhang, J.-Y., and Yu, G.-H. (2013). Research Progress in Anisotropic Magnetoresistance. Rare Mater. 32, 213–224. doi:10.1007/s12598-013-0090-5
Zhou, C., Zhu, D., Liu, F., Feng, C., Zhang, M., Ding, L., et al. (2021). Electric-field-induced In-Plane Effective 90° Magnetization Rotation in Co2FeAl/PMN-PT Structure*. Chin. Phys. B 30, 057504. doi:10.1088/1674-1056/abd7de
Keywords: multiferroic, conetic alloy, ferroelectric, magnetoresistance, magnetoelectric coupling, strain
Citation: Wang C, Huang W, Wang C, Li L, Wei C, Sun Y, Ge W, Mao X, Li Q, Yang Y, Jia C, Zhang H, Luo Z and Gao C (2022) Electric-Field Control of Magnetoresistance Behavior in a Conetic Alloy Thin Film/Pb(Mg1/3Nb2/3)0.7Ti0.3O3 Multiferroic Heterostructure. Front. Mater. 9:956349. doi: 10.3389/fmats.2022.956349
Received: 30 May 2022; Accepted: 15 June 2022;
Published: 07 July 2022.
Edited by:
Aitian Chen, King Abdullah University of Science and Technology, Saudi ArabiaReviewed by:
Cai Zhou, Wuhan Textile University, ChinaZiyao Zhou, Argonne National Laboratory (DOE), United States
Copyright © 2022 Wang, Huang, Wang, Li, Wei, Sun, Ge, Mao, Li, Yang, Jia, Zhang, Luo and Gao. This is an open-access article distributed under the terms of the Creative Commons Attribution License (CC BY). The use, distribution or reproduction in other forums is permitted, provided the original author(s) and the copyright owner(s) are credited and that the original publication in this journal is cited, in accordance with accepted academic practice. No use, distribution or reproduction is permitted which does not comply with these terms.
*Correspondence: Yuanjun Yang, yangyuanjun@hfut.edu.cn; Chen Gao, gaochen@ucas.ac.cn