- Faculty of Engineering, Horus University-Egypt, Damietta, Egypt
Plastic wastes (PW) create chronic environmental problems mainly because it is not biodegradable. The utilization of PW as a fractional substitution of fine aggregate in concrete production is a sustainable application. However, the smooth surface of plastic weakens the cement-plastic bond and consequently lessens the mechanical properties of concrete. This research improves the cement-plastic bond by increasing the hydrophilicity and coarseness of polyethylene terephthalate (PET) through chemical treatment with 5wt% polystyrene (P.S.) and 5wt% PKHH-phenoxy resin. Twenty eight mixtures with different w/c ratio; 0.4, 0.45, 0.5, and 0.6 and different percentage of sand substitution; 5%, 20%, and 50% are cast. Tests are conducted to determine the behavior of fresh and hardened concrete in terms of workability, water absorption, density, compressive strength, flexural strength, splitting tensile strength, and durability. Results show that the proposed chemical treatment rises the cement-plastic bond. The compressive, splitting tensile, and flexural strength were improved by 45%, 35%, and 6% respectively compared to corresponding untreated plastic recycled concrete. The highest mechanical properties are achieved for mixes with 5% plastic. Nevertheless, a 20% replacement of sand with treated plastic can be used effectively to produce structural concrete.
1 Introduction
In the preceding 50 years, worldwide plastic production had amplified extensively (Gu and Ozbakkaloglu, 2016), more than 400 billion tons of plastics are annually produced (Wong et al., 2015; Ferdous et al., 2021). Figure 1 shows the universal PW origination between 1950 and 2015 (Rajasekaran and Maji, 2018). If present production and waste controlling policies remain unchanged, nearly 12, 000, 000,000 tons of PW will be in landfills by 2050 (Ohemeng and Ekolu, 2019). Waste recycling is essential, it services lessen energy production and help in enduring natural supplies (Ohemeng and Ekolu, 2019).
Concrete, being a common construction material, consumes natural resources enormously. The rate of consumption of natural recourses is rising due to the recent development in urban areas (Ismail and AL- Hashmi, 2008). Consequently, discovering new alternatives is crucial to arise sustainability in the construction industry. Researchers studied the usage of wastes as replacements for concrete constituents (Albano et al., 2009), (Khalil and Mahdi, 2020). Reutilizing PW, especially resin-based types and PET, is a feasible substitute for natural sand. Hence it has twofold benefits; decreasing landfills and preserving natural sand. It is considered a cost-effective prospect (Abdel Moti and Mustafa, 2019), (Arivalagan, 2020) especially since the impurity of PW would not affect the concrete properties.
The fresh and hardened properties of concrete containing plastic materials are examined by numerous scientists (Foti, 2011; Amalu et al., 2019; Faraj et al., 2019; Lokeshwari et al., 2019; Adnan and Dawood, 2020).
The compressive strength of recycled plastic concrete is affected by the percentage of substitution (SPA), w/c ratio, and the shape and size of particles (Gu and Ozbakkaloglu, 2016). Results indicate that compressive strength declines as SPA increases (Saikia and De Brito, 2014; Batayneh et al., 2016; Spiesz et al., 2016). This was attributed to different factors; inhabitation of cement hydration due to the hydrophobic properties of PW; the elevated penetrability and air-content of PW concrete; and low cement-plastic bond (Sharma and Bansal, 2016). Batayneh et al. Batayneh et al. (2016) experimented a 23% and 72% reduction in the compressive strengths of concrete at 5 and 20 percent replacement of sand with PW respectively. This reduction percentage is higher than that stated by Almeshal et al. Almeshal et al. (2020). They claimed that compressive strength at 28 days decreased by 15.08 % and 12.72% for 5% and 10% substitution of fine aggregate respectively. This was in agreement with Hannawi et al. Hannawi et al. (2010) and Arivalagan, S. Arivalagan (2020), they both attributed this reduction to the weak bond between cement and PW.
The influence of PW on flexural strength is similar to its effect on compressive strength. Saikia and de Brito Saikia and De Brito (2014) claimed that flexural strength declines with the incorporation of PET in concrete. Likewise, Aciu et al. Aciu et al. (2018), stated that flexural strength declined by 20%, 23%, and 54% at 25%, 50%, and 100% replacement of fine aggregate Arivalagan, S. Arivalagan (2020) declared that 20% replacement of fine aggregate, reduced the flexural strength by 30%. This is nearly the same as what Jaivignesh, B., and Sofi, A. Jaivignesh and Sofi (2017) achieved, their investigations confirmed that flexural Strength decreased by 17.77, 25.1, and 33.82% for 10, 15, and 20 of plastic waste replacement respectively at 28 days. Almeshal et al. Almeshal et al. (2020) claimed a higher reduction rate of 2.4–84.2% for 10–50% replacement. On the contrary, Hannawi et al. Hannawi et al. (2010) stated that no noteworthy variations in the flexural strength with 10% substitution of fine aggregates with PET competed with the control mix. The minor changes in flexural strength were accredited to the elastic behavior of the PW (Hannawi et al., 2010).
Albano et al. Albano et al. (2009) observed that the enclosure of PET in concrete decreased the splitting tensile strength owing to the increase in the porosity. Abdel Moti, H. M., and Mustafa, M. A. Abdel Moti and Mustafa (2019)
Stated that the splitting tensile strength was reduced by 10.15% and 14.03% for the 5% and 10% respectively compared to the control mix. This was attributed to the weak bond between the cement paste and the plastic surface. Arivalagan, S. Arivalagan (2020) stated that the maximum splitting tensile strength at 28 days was 5.5 MPa for 20% replacement but decrease to 3.75 at 30% replacement. Due to weaker bonding between PW and cement particles. This is in agreement with Jaivignesh, B., and Sofi, A Jaivignesh and Sofi (2017), who indicated that splitting tensile strength decreased by 19.8, 14.35, and 24.25% for 10, 15, and 20% of plastic waste replacement respectively at 28.
In studying the consequence of PW on water absorption of concrete, Albano et al. Albano et al. (2009) revealed that the size and content of PET particles imitate the water absorption due to the porosity of PET. However, Frigione Frigione (2010) revealed that concrete enclosing minor percentages of PET have an insignificant effect on water absorption. The dry density of recycled plastic concrete tends to be lower than the reference mixes. The lower weight of concrete mixes containing 5% and 10% PP pellets is due to the fact that sand density is lower that plastic pellets by 69.5 (Abdel Moti and Mustafa, 2019).
Chloride permeability as an essential property of concrete was also studied. Kou et al. Kou et al. (2009) performed the rapid chloride permeability test for concrete with PET at replacement ratios of 5, 15, 30, and 45. The results indicated that increasing PET content decreases the chloride permeability. Chloride penetration declined by 36.2% to the reference concrete at 45% replacement, this was accredited to the impermeable PET which prevents the immigration of the chloride ions (Kou et al., 2009). As foreseen from the literature review the main obstacle to using PW as a substitution for sand is poor mechanical properties due to the low cement-plastic bond. It should be noted that all the previous studies did not expose the plastic particles to any chemical treatment.
2 Research significance
While the use of PET waste as a partial replacement of fine aggregates had previously been investigated, the results showed poor mechanical properties. The main purpose of this paper is to overcome the weak-cement bond associated with using PET as a replacement for fine aggregate. This is done by modifying low-energy surfaces of PET through blending with polystyrene resin (PS Styron 663) and phenoxy resin (PKHH). This chemical treatment increases the roughness at the surface of PET and increases the bond between cement paste and PET. Hence, improves the mechanical properties of plastic recycled concrete.
3 Experimental methodology
In an attempt to improve the mechanical properties of recycled plastic concrete, this paper investigates the consequence of using chemically treated PET. Mixes with different w/c ratio; 0.4, 0.45, 0.5, 0.6 are used. For each w/c ratio three substitution percentages by weight of fine aggregate; 5%, 20%, and 50% are investigated. Compressive strength, flexural strength, splitting tensile strength, and chloride permeability for each mix are tested, then the results are compared to corresponding mixes with untreated plastic particles to assess the effectiveness of the proposed chemical treatment. Furthermore, all results are compared to control mixes with 0% plastic content.
4 Experimental work
4.1 Mix design
Mixtures are intended to reach a strength of 35 MPa at 28 days to ensure the applicability of producing structural concrete rather than lightweight concrete. A total of twenty-eight mixes are prepared, Table 1 presents the concrete mixtures used in this study. To achieve a uniform concrete mix, all mixtures are tested using the slump test in accordance with ASTM C31 (American Society for Testing and Materials, 2019), the slump test results ranged from 30 mm to 120 mm. Mixtures are then cast in standard molds required for each test. The concrete mixtures are poured into the mold in three layers followed by compaction in between those layers to assure well compaction, thus avoiding any deficiencies such as honeycombs and air traps.
The Cement used throughout the study is Type I Portland cement, Table 2 displays the physical properties of cement. The maximum nominal size of the crushed limestone aggregate used is 20 mm following ASTM C33 (ASTM, 2010). Table 3 shows the properties of plastic, coarse and fine aggregate Figure 2 displays the grading of fine aggregate and plastic utilized. After 1 day of casting, the specimens are demolded and moist cured till the day of testing following ASTM C192 (ASTM, 2013a).
4.2 Plastic preparation and chemical treatment
Polyethylene terephthalate (PET) bottles are washed and cut to small particles close to natural sand gradation by a shredder machine. The machine consists of a hopper with dimensions of 116 * 45 cm as input for plastic bottles, and a rotating shaft consisting of nine moving blades and two installed for cutting plastic bottles, in addition to a filter in the bottom to sieve the plastic parts to the required gradation. The grading of PET particles is adjusted to imitate the grading of natural sand in accordance with ASTM C 136 (American Society for Testing and Materials, 2014). Figure 2 represents the grading for the sand, the untreated plastic, and the chemically treated plastic. The chemical treatment tends to increase the coarseness of the plastic particles. However, it is still in the accepted grading limits for fine aggregate. It is recommended for future studies that the plastic particles should be cut into smaller sizes to overcome the coarseness caused by the chemical treatment.
The low surface energy of PET lessens its dyeability, wettability, and adhesion to other surfaces. To improve the cement-PET bond, the blending of PET waste with 5% wt. PS Styron 663 with a density of 1.04 g/ml and 5% PKHH phenoxy resin with Mw of 52 kDa are made using solids incorporation of the three components. PS Styron is an additive-free resin with excellent strength, clarity, and high heat resistance. Phenoxy resins are apolyhydroxyether having terminal alpha-glycolgroups which are tough and ductile thermoplastic materials having high cohesive strength and good impact resistance. The blending technique is used due to its easiness, reproducibility, and commercial accessibility of anticipated additives. This treatment had been chosen based on work done by Ahmad Rezaei Kolahchi, Abdellah Ajji, and Pierre. J. Carreau AhmadKolahchi et al. (2015). They postulated that mixing of 5wt% PKHH phenoxy-resin in the mixture of PET-5wt%PS improved the viscosity of the medium causing a lessening of the PS droplet size and preferred the immigration of the droplets to the film-surface layer resulting in creating roughness at the surface of PET as shown in Figure 3.
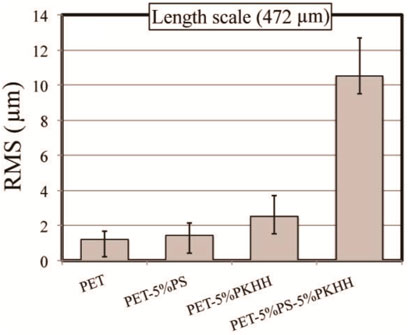
FIGURE 3. RMS surface-roughness for the PET., PET.-5wt%PS, PET-5wt%PKHH, and PET- 5wt%PS-5wt%PKHH films surfaces in 472 μm length scale (AhmadKolahchi et al., 2015).
4.3 Tests on hardened concrete
The consequence of the replacement of fine aggregate with PET with different percentages is assessed by determining water absorption, compressive, flexural, and tensile strength, and chloride permeability.
4.3.1 Water-absorption test
The specimens required for water-absorption analysis are demolded and immersed in water for 24 h before being tested according to ASTM C642 (ASTM, 2013b).
4.3.2 Compressive strength test
Six cubes of side length 150 mm, the uncertainty of reading dimensions = ± 0.05 mm, were prepared from each concrete mixture for investigation of compressive strength. The concrete specimens were cured under conditions as ASTM 192 (ASTM, 2013a) and were examined using a universal Test Machine at 28 days. The test system needs rounding to the closest 0.1N/mm, expanded insecurity at the level of confidence of 1.21 N/mm2.
4.3.3 Splitting tensile test
150 × 300 mm cylindrical specimens were utilized to detect the tensile strength. Specimens were cast and then cured in water at room temperature for 28 days. After this phase, three specimens per mixture were examined for tensile strength following ASTM C496/C496M (ASTM, 2011), and the mean was recorded.
4.3.4 Flexural strength
Flexural strength was examined using a 100 × 100 × 500 mm specimen with a central load. Thirty beams were molded and then cured in water for 28 days. For individual mixture, 3beams were loaded to failure following ASTM C78/C78M (ASTM, 2018), and the mean strength was recorded in each case.
4.3.5 Chloride permeability
The RCPT was in agreement with ASTM C 1202 (ASTM 1202) by observing the quantity of electrical current that passes through a sample 50 mm thick by 100 mm in diameter in 6 hours.
5 Results and discussion
5.1 Workability
A slump values of 40 mm, 60 mm, 93 mm, and 123 mm were obtained for the control concrete mixes A0, B0, C0, and D0 respectively. The concrete mixes with a 5% PET replacement ratio yielded a slump nearly similar to that of the control concrete mixes. As the content of untreated PET increased to 20% and 50%, the slump value reduced by nearly 4% and 10%, respectively. Although the workability of concrete decreased gradually with the increment of PET, the reduction was insignificant relative to the reference concrete dissimilar to the previous studies (Choi et al., 2005; Ismail and AL Hashmi, 2007; Rahmani et al., 2013), which reported a considerable reduction in concrete even with a small percentage replacement. This could be attributed to the smooth surface of PET, which aids fine aggregate dispersion in the mixture. However, on comparing slump loss for chemically treated mixes, the reduction was 12.5%, 16.7%, 7.6%, and 18.7% for mixes AT20, BT20, CT20, and DT20 respectively. Furthermore, the reduction was 50% for all mixes with 50% substitution. The chemical treatment has a significant effect on slump loss. This is accredited to the roughness and irregular shape of the chemically treated PET. Although the results are in agreement with the outcomes of previous studies (Bhogayata et al., 2012; Saxena et al., 2016), it is recommended to use a superplasticizer to overcome the reduction in concrete workability, especially with a high percentage of reduction.
5.2 Water-absorption
The outcomes of water absorption tests show that water absorption was directly proportional to the rise in PET percentages. Although the highest water absorption was 5.7%, it is obvious that the incorporation of untreated plastic particles increases the water absorption compared to their corresponding mixes with chemically treated plastic. The increase in water absorption reaches more than 300% for mixes with 50% untreated plastic particles. This contradicts Ohemeng and Ekolu Ohemeng and Ekolu (2019) who stated that absorption of mortar containing 20% of LDPE as substitution of the fine aggregate increased the water absorption by 3.14%. These differences may be attributed to the difference in the type of plastic waste used in the two studies. Figure 4 shows the water absorption for different mixes.
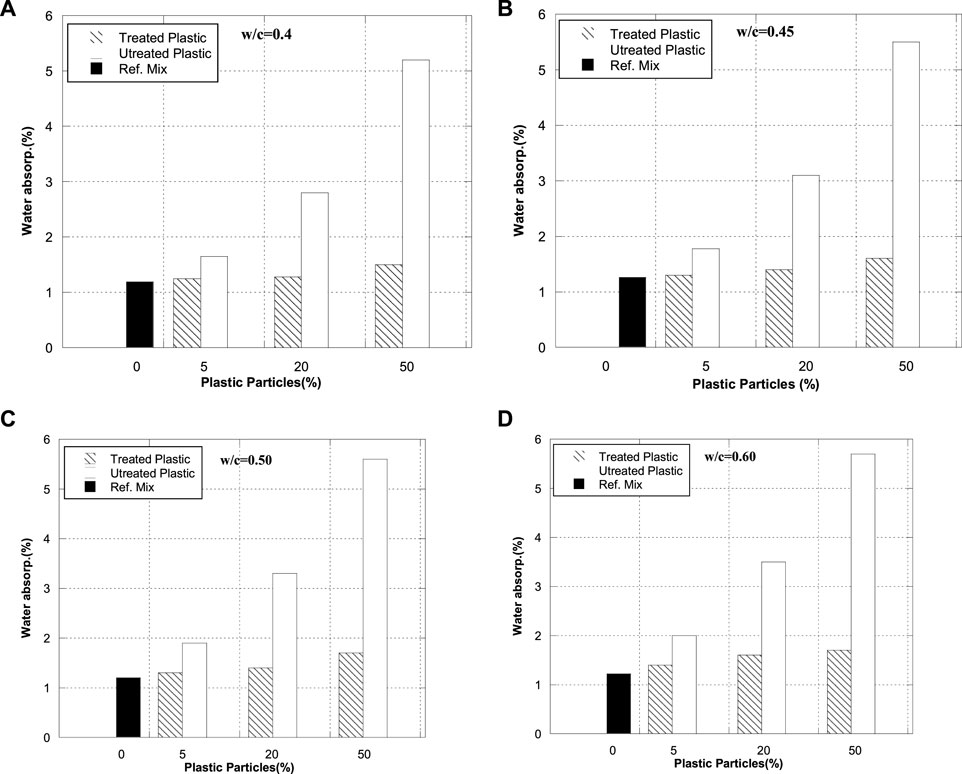
FIGURE 4. Water absorption % for different mixes (A) w/c = 0.40, (B) w/c = 0.45, (C) w/c = 0.50, and (D) w/c = 0.60.
5.3 Density
The dry density measurement results declared that the density of concrete decreased with the increase in PET, treated and untreated, content in concrete. The density for concrete with 5% and 20% replacement ratios were nearly 2,350 kg/m3, and 2,200 kg/m3, respectively, for different w/c ratios which were comparatively close to that of the control sample (2,400 kg/m3). However, the density for the mixes with 50% PET content reached 1800 kg/m3, representing a substantial drop of 25% relative to that of the control sample. These findings are supported by other studies (Choi et al., 2005; Ismail and AL Hashmi, 2007; Rahmani et al., 2013; Almeshal et al., 2020).
5.4 Compressive strength
Figure 5 represents the results of the compressive strength test. For all mixtures, results declared that the greater the percentage of the replacement, the lower the compressive strength. The greatest reduction was for mixes with w/c equals 0.6 and untreated plastic particles. The reduction is 3%, 30%, and 45% for mixes with 5%, 20%, and 50% replacement, respectively, compared to the control mix. In comparing mixes with chemically treated PET to untreated ones, it is evident that the chemical treatment improves the compressive strength for mixes with different w/c and at different percentages of replacement. The enhancement is attributed to the rough surface obtained through the chemical treatment. The surface roughness increases the interbond between cement and PET particles. The compressive strength for mixes with treated PET is higher than the results obtained by Akinyele and Ajede Akinyele and Ajede (2018) who reported that the drop in the strengths of the concrete with 5, 10, 15, and 20% PW were 0.5%, 16%, 33.5%, and 53% respectively. In addition, the results are higher than that obtained by Thorneycroft et al. Thorneycroft et al. (2018) who investigated the compressive strength of concrete with chemically-treated plastic with sodium hypochlorite. They postulated that the compressive strength declined by 40% at 50% substitution. The previous comparison confirmed the appropriacy of the chosen treatment compared to sodium hypochlorite examined by Thorneycroft et al. Thorneycroft et al. (2018).
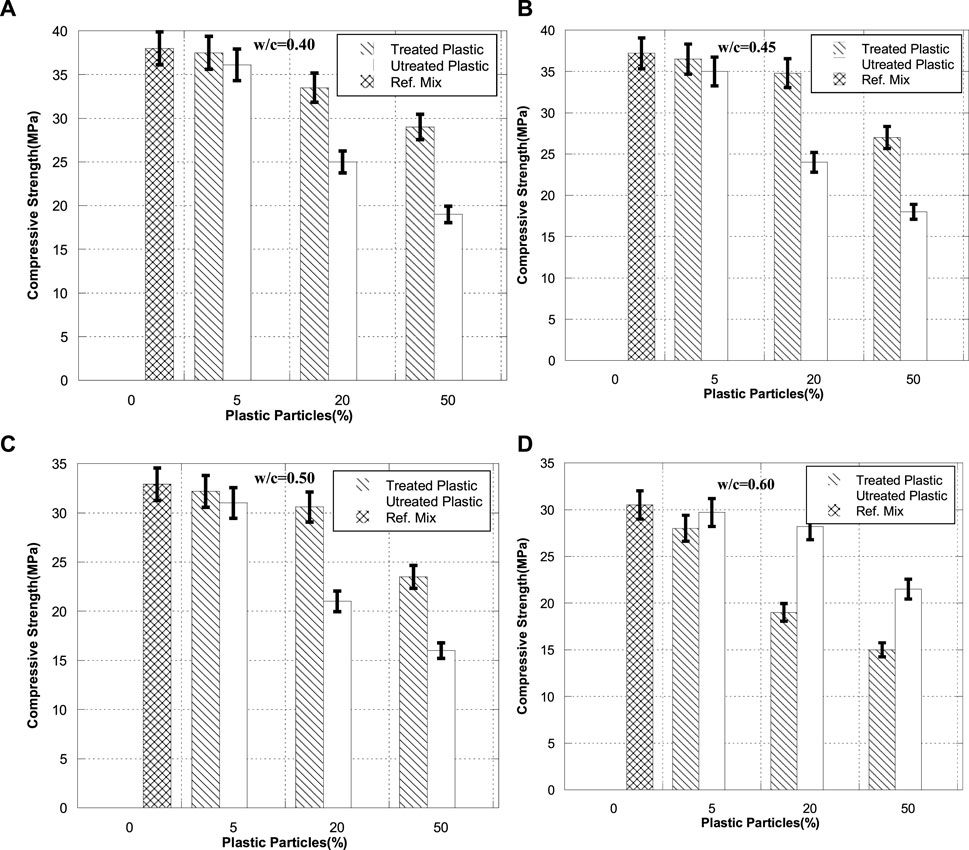
FIGURE 5. Compressive Strength for different mixes (A) w/c = 0.40, (B) w/c = 0.45, (C) w/c = 0.50, and (D) w/c = 0.60.
5.5 Splitting tensile strength
Figure 6 illustrates the results of the splitting tensile strength test. The addition of 5% PET has a nearly negligible effect on tensile strength for all mixes, and the performance began to decline with the increase of PET in the mixture. The specimen with a 20% substitution rate revealed a minor reduction of 3% compared to that of the reference concrete. The explanation for these observations is that the flexibility of PET, as well as chemical treatment provided enhanced adhesion between aggregates and cement matrix. However, the reduction in tensile strength was more distinct when the percentage of PET increased to 50%.
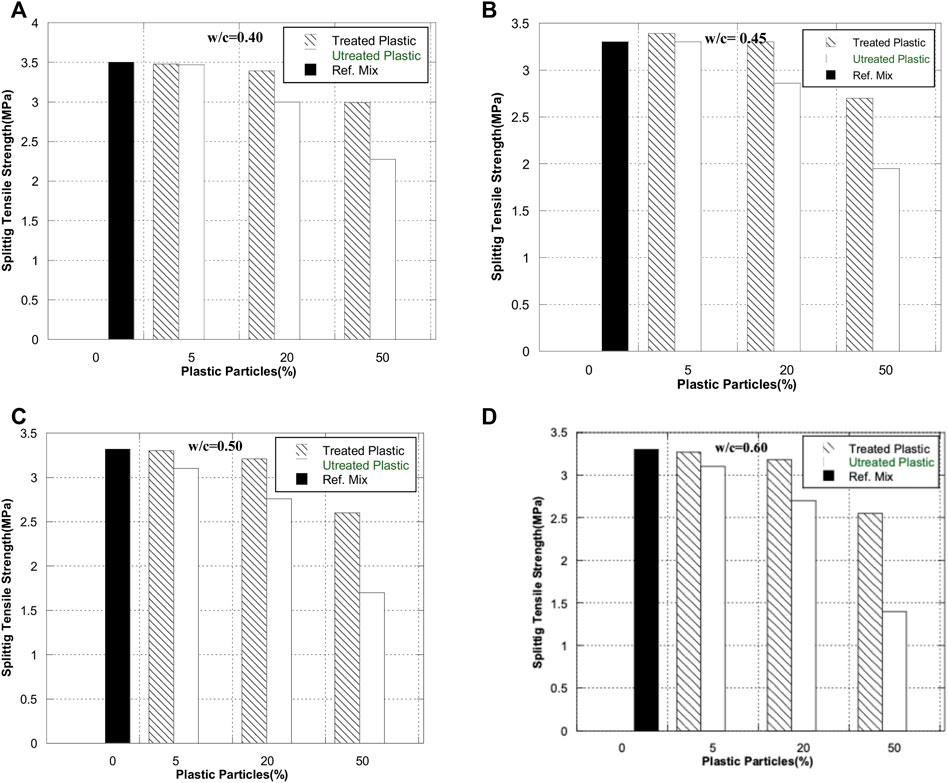
FIGURE 6. Splitting Tensile Strength for different mixes (A) w/c = 0.40, (B) w/c = 0.45, (C) w/c = 0.50, and (D) w/c = 0.60.
The highest reduction, 23%, occurred in the mix with w/c equals 0.6 compared to the control mix. The decrease in splitting tensile strength beyond the 20% replacement ratio is due to the isolation of the PET particles from the cement after attaining ultimate strength as a result of a larger percentage of PET. Reaching higher tensile strength than other studies (Saikia and De Brito, 2014; Akinyele and Ajede, 2018; Almeshal et al., 2020) is attributed to the improvement in bond strength between the cement and PET particles. In comparing the mixes with untreated plastic to mixes with treated plastic, it is observed that the chemical treatment enhanced the splitting tensile strength by 2%, 15%, and 36% at 5%, 20%, and 50% replacement respectively.
It should also be noted that different failure modes occurred during tensile strength tests since samples did not split into two sections. This may be accredited to that the PET behaved as a bridge between the two split pieces. This is in covenant with Babu and Babu Babu and Babu (2003) and Tang et al. Tang et al. (2008) who observed that the breakdown mode of the tensile strength of concrete containing PW did not reveal brittle failure as typically demonstrated by conventional concrete.
5.6 Flexural strength
The flexural strength test results are shown in Figure 7. The test results revealed that the specimen with 5% PET achieved the optimal flexural strength of 4.37, 4.35, 4.05, and 3.96 Mpa for w/c 0.4, 0.45, 0.5, and 0.6 respectively with nearly no difference from the reference concrete. Despite that flexural strength declined as the amount of PET in the mixture increased, the specimen with a 20% replacement ratio still had a negligible impact on flexural strength, demonstrating a 2.4% decrease over the reference samples. Dissimilarity, the flexural strength of the specimen with a 50% replacement ratio declined by 10% compared to that of the control samples. The insignificant decrease in flexural strength of the specimens with 5% and 20% replacement rates compared to the control sample may be attributed to the flexibility of the PET particles. The decrease in flexural strength for the specimen with a 50% substitution rate is attributed to the collecting of PET particles in the mixture resulting in weak areas in the concrete. These results are in agreement with those of Rahmani et al. Rahmani et al. (2013) and Azhdarpour et al. Azhdarpour et al. (2016). It should be noted that the results of the mixes with the untreated plastic were lower than their corresponding mixes with treated plastic by 5% and 7% for 20% and 50% replacement respectively. As for the failure pattern of the beams investigated for flexure strength, it is evident that conventional concrete split in half after reaching ultimate strength due to its brittle nature. In contrast, the specimens incorporating PET are more ductile, with only minor cracks in the specimens with 5% and 20% replacement ratios, and a marginally larger crack in the specimen with a 50% replacement ratio.
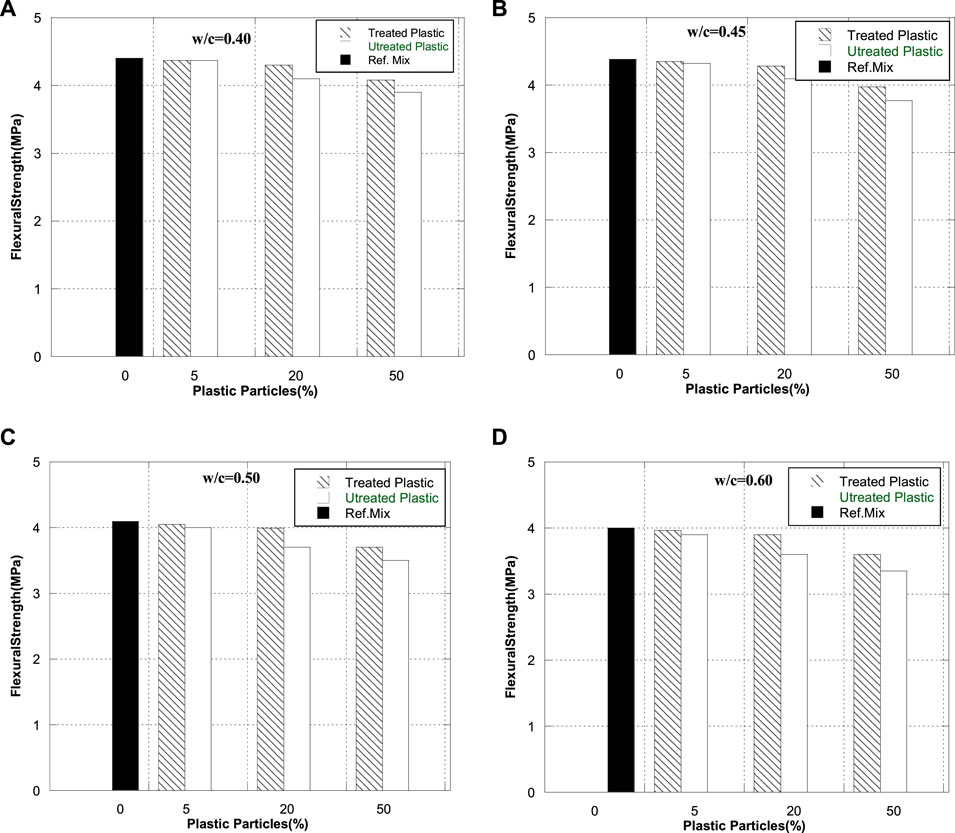
FIGURE 7. Flexural Strength for different mixes (A) w/c = 0.40, (B) w/c = 0.45, (C) w/c = 0.50, and (D) w/c = 0.60.
5.7 Chloride permeability
The RCPT values of concrete specimens are given in Table 4. It can be realized that the substitution of fine-aggregate with PET slightly increases the penetration of chloride ion for all mixtures despite the w/c.
6 Conclusion
This paper investigates the effect of chemical treatment of plastic particles on improving the properties of the produced concrete. Thus, increasing the possibility of utilizing recycled plastic as a substitution for sand in structural concrete. The following points were concluded:
• Particles with Rough surfaces created sufficient bond strength with cementitious matrix.
• Treatment of plastic-particles with 5wt% PKHH phenoxy resin and 5wt%PS Styron improves the bond strength and mechanical properties of concrete.
• The chemical treatment increases the compressive strength by 4%, 40%, 46% for mixes with 5%, 20%5, and 50% replacement of sand.
• The chemical treatment increases the splitting tensile strength by 2%, 15%, 35% for mixes with 5%, 20%5, and 50% replacement of sand.
• The chemical treatment increases the flexural strength by 1.5%, 4.5%, 6% for mixes with 5%, 20%5, and 50% replacement of sand.
• The replacement of fine aggregate with 20% plastic produces a structural concrete with 32 Mpa compressive strength.
• The chloride permeability of concrete is not affected by the treated plastic-substitution despite the replacement percentages.
• The replacement of natural sand by 20% is both efficient and cost-effective for producing sustainable structural concrete.
Data availability statement
The original contributions presented in the study are included in the article/supplementary material, further inquiries can be directed to the corresponding author.
Author contributions
The author confirms being the sole contributor of this work and has approved it for publication.
Funding
This research did not receive any specific grant from funding agencies in the public, commercial, or not-for-profit sectors.
Conflict of interest
The author declares that the research was conducted in the absence of any commercial or financial relationships that could be construed as a potential conflict of interest.
Publisher’s note
All claims expressed in this article are solely those of the authors and do not necessarily represent those of their affiliated organizations, or those of the publisher, the editors and the reviewers. Any product that may be evaluated in this article, or claim that may be made by its manufacturer, is not guaranteed or endorsed by the publisher.
References
Abdel Moti, H. M., and Mustafa, M. A. (2019). Use of polypropylene waste plastic pellets as partial replacement for fine aggregate in concrete. Univ. of Khartoum Eng. J. 9 (1), 37–43.
Aciu, C., Ilutiu-Varvara, D. A., Manea, D. L., Orban, Y. A., and Babota, F. (2018). Recycling of plastic waste materials in the composition of ecological mortars. Procedia Manuf. 22, 274–279. doi:10.1016/j.promfg.2018.03.042
Adnan, H. M., and Dawood, A. O. (2020). Strength behavior of reinforced concrete beam using re-cycle of PET wastes as synthetic fibers. Case Stud. Constr. Mater. 13, e00367. doi:10.1016/j.cscm.2020.e00367
AhmadKolahchi, R., Abdellah, A., and Carreau, P. J. (2015). Improvement of PET surface hydrophilicity and roughness through blending. AIP Conf. Proc., 1–4. doi:10.1063/1.4918391
Akinyele, J. O., and Ajede, A. (2018). The use of granulated plastic waste in structural concrete. Afr. J. Sci. Technol. Innovation Dev. 10 (2), 169–175. doi:10.1080/20421338.2017.1414111
Albano, C., Camacho, N., Hernández, M., Matheus, A., and Gutiérrez, A. (2009). Influence of content and particle size of waste pet bottles on concrete behavior at different w/c ratios. Waste Manag. 29, 2707–2716. doi:10.1016/j.wasman.2009.05.007
Almeshal, I., Tayeh, B. A., Alyousef, R., Alabduljabbar, H., and Mohamed, A. M. (2020). Eco-friendly concrete containing recycled plastic as partial replacement for sand. J. Mater. Res. Technol. 9 (3), 4631–4643. doi:10.1016/j.jmrt.2020.02.090
Amalu, A. A., Hashim, M., Rejith, K. U., and Vijitha, V, R. (2019). Use of waste plastic as fine aggregate substitute. Int. J. Sci. Eng. Res. 7 (4), 172–177.
American Society for Testing and Materials (2014). ASTM C136/C136M - 14 standard test method for sieve analysis of fine and coarse aggregates. West Conshohocken, PA: ASTM International. doi:10.1520/C0136
American Society for Testing and Materials (2019). ASTM C31-19_Standard practice for making and curing concrete test specimens in the field. West Conshohocken, PA: ASTM International. doi:10.1520/C0031
Arivalagan, S. (2020). Experimental study on the properties of green concrete by replacement of E-plastic waste as aggregate. Procedia Comput. Sci. 172, 985–990. doi:10.1016/j.procs.2020.05.145
ASTM 1202. “Astm C 1202-97: standard test method for electrical indication of concrete’s ability to resist chloride ion penetration,” in Annual book of ASTM standards. 0402, 639–644.
ASTM (2011). ASTM C 496/C 496M: Standard test method for splitting tensile strength of cylindrical concrete specimens. West Conshohocken, PA: ASTM International.
ASTM (2013a). ASTM C192: Standard practice for making and curing concrete test specimens in the laboratory. West Conshohocken, PA: ASTM International.
ASTM (2010). ASTM C33: Standard specification for concrete aggregates. West Conshohocken: ASTM International.
ASTM (2013b). ASTM C642: Standard test method for density, absorption, and voids in hardened concrete. West Conshohocken, PA: ASTM International.
ASTM (2018). ASTM C78: Standard test method for flexural strength of concrete (using simple beam with third-point loading). West Conshohocken, PA: ASTM International.
Azhdarpour, A. M., Nikoudel, M. R., and Taheri, M. (2016). The effect of using polyethylene terephthalate particles on physical and strength-related properties of concrete; a laboratory evaluation. Constr. Build. Mat. 109, 55–62. doi:10.1016/j.conbuildmat.2016.01.056
Babu, K. G., and Babu, D. S. (2003). Behaviour of lightweight expanded polystyrene concrete containing silica fume. Cem. Concr. Res. 33 (5), 755–762. doi:10.1016/S0008-8846(02)01055-4
Batayneh, M., Marie, I., and Asi, I. (2016). Use of selected waste materials in concrete mixes. Waste Manag. 27 (12), 1870–1876. doi:10.1016/j.wasman.2006.07.026
Bhogayata, A., Shah, K. D., and Vyas, B. A. (2012). Performance of concrete by using non-recyclable plastic waste as concrete constituent. Int. J. Eng. Res. Technol. 1 (4), 13–22. doi:10.31026/j.eng.2021.12.0227(12)
Choi, Y. W., Moon, D. J., Chung, J. S., and Cho, S. K. (2005). Effects of waste PET bottles aggregate on the properties of concrete. Cem. Concr. Res. 35 (4), 776–781. doi:10.1016/j.cemconres.2004.05.014
Faraj, R. H., Sherwani, A. F. H., and Daraei, A. (2019). Mechanical, fracture and durability properties of self-compacting high strength concrete containing recycled polypropylene plastic particles. J. Build. Eng. 25, 100808. doi:10.1016/j.jobe.2019.100808
Ferdous, W., Manalo, A., Siddique, R., Mendis, P., Zhuge, Y., Wong, H. S., et al. (2021). Recycling of landfill wastes(tyres, plasticsandglass) in construction–A review on global waste generation, performance, application and future opportunities. Resour. Conservation Recycl. 173, 105745. doi:10.1016/j.resconrec.2021.105745
Foti, D. (2011). Preliminary analysis of concrete reinforced with waste bottles PET fibers. Constr. Build. Mat. 25 (4), 1906–1915. doi:10.1016/j.conbuildmat.2010.11.066
Frigione, M. (2010). Recycling of PET bottles as fine aggregate in concrete. Waste Manag. 30 (6), 1101–1106. doi:10.1016/j.wasman.2010.01.030
Gu, L., and Ozbakkaloglu, T. (2016). Use of recycled plastics in concrete: a critical review. Waste Manag. 51, 19–42. doi:10.1016/j.wasman.2016.03.005
Hannawi, K., Kamali-Bernard, S., and Prince, W. (2010). Physical and mechanical properties of mortars containing PET and PC waste aggregates. Waste Manag. 30 (11), 2312–2320. doi:10.1016/j.wasman.2010.03.028
Ismail, Z. Z., and Al Hashmi, E. A. (2007). Use of waste plastic in concrete mixture as aggregate replacement. Waste Manag. 28, 2041–2047. doi:10.1016/j.wasman.2007.08.023
Ismail, Z. Z., and Al-Hashmi, E. A. (2008). Use of waste plastic in concrete mixture as aggregate replacement. Waste Manag. 28 (11), 2041–2047. doi:10.1016/j.wasman.2007.08.023
Jaivignesh, B., and Sofi, A. (2017). Study on mechanical properties of concrete using plastic waste as an aggregate. IOP Conf. Ser. Earth Environ. Sci. 80 (1), 012016. doi:10.1088/1755-1315/80/1/012016
Khalil, W. I., and Mahdi, H. M. (2020). Some properties of sustainable concrete with mixed plastic waste aggregate. IOP Conf. Ser. Mat. Sci. Eng. 737 (No. 1), 012073. doi:10.1088/1757-899x/737/1/012073
Kou, S. C., Lee, G., Poon, C. S., and Lai, W. L. (2009). Properties of lightweight aggregate concrete prepared with PVC granules derived from scraped PVC pipes. Waste Manag. 29 (2), 621–628. doi:10.1016/j.wasman.2008.06.014
Lokeshwari, M., Ostwal, N., Nipun, K. H., Saxena, P., and Pranay, P. (2019). Utilization of waste plastic as partial replacement of fine and coarse aggregates in concrete blocks. Int. Res. J. Eng. Technol. (IRJET), 1–5. doi:10.1109/ICEECCOT.2017.8284686
Ohemeng, E. A., and Ekolu, S. O. (2019). Strength prediction model for cement mortar made with waste LDPE plastic as fine aggregate. J. Sustain. Cement-Based Mater. 8 (4), 228–243. doi:10.1080/21650373.2019.1625826
Rahmani, E., Dehestani, M., Beygi, M. H. A., Allahyari, H., and Nikbin, I. M. (2013). On the mechanical properties of concrete containing waste PET particles. Constr. Build. Mat. 47, 1302–1308. doi:10.1016/j.conbuildmat.2013.06.041
Rajasekaran, D., and Maji, P. K. (2018). Recycling of plastic wastes with poly (ethylene-co-methacrylic acid) copolymer as compatibilizer and their conversion into high-end product. Waste Manag. 74, 135–143. doi:10.1016/j.wasman.2018.01.018
Saikia, N., and De Brito, J. (2014). Mechanical properties and abrasion behaviour of concrete containing shredded PET bottle waste as a partial substitution of natural aggregate. Constr. Build. Mater. 52, 236–244. doi:10.1016/j.conbuildmat.2013.11.049
Saxena, R., Jain, A., and Agrawal, Y. (2016). Utilization of waste plastic in concrete towards sustainable development: a review. J. Eng. Res. Appl. 6 (12), 88–100. doi:10.1007/398_2015_5009
Sharma, R., and Bansal, P. P. (2016). Use of different forms of waste plastic in concrete - a review. J. Clean. Prod. 112, 473–482. doi:10.1016/j.jclepro.2015.08.042
Spiesz, P., Rouvas, S., and Brouwers, H. J. H. (2016). Utilization of waste glass in translucent and photocatalytic concrete. Constr. Build. Mater. 128, 436–448. doi:10.1016/j.conbuildmat.2016.10.063
Tang, W. C., Lo, Y., and Nadeem, A. (2008). Mechanical and drying shrinkage properties of structural-graded polystyrene aggregate concrete. Cem. Concr. Compos. 30 (5), 403–409. doi:10.1016/j.cemconcomp.2008.01.002
Thorneycroft, J., Orr, J., Savoikar, P., and Ball, R. J. (2018). Performance of structural concrete with recycled plastic waste as a partial replacement for sand. Constr. Build. Mater., 63–69. doi:10.1016/j.conbuildmat.2017.11.127
Keywords: material properties, stress, sustainable materials, waste reduction, adhesion, composites, plastic recycling-aggregate replacement
Citation: El-Nadoury WW (2022) Chemically treated plastic replacing fine aggregate in structural concrete. Front. Mater. 9:948117. doi: 10.3389/fmats.2022.948117
Received: 19 May 2022; Accepted: 01 July 2022;
Published: 22 July 2022.
Edited by:
Patricia Krawczak, IMT Nord Europe, Institut Mines-Télécom, FranceReviewed by:
Ailar Hajimohammadi, University of New South Wales, AustraliaGideon Bamigboye, Covenant University, Nigeria
Copyright © 2022 El-Nadoury. This is an open-access article distributed under the terms of the Creative Commons Attribution License (CC BY). The use, distribution or reproduction in other forums is permitted, provided the original author(s) and the copyright owner(s) are credited and that the original publication in this journal is cited, in accordance with accepted academic practice. No use, distribution or reproduction is permitted which does not comply with these terms.
*Correspondence: Wegdan W. El-Nadoury, wegdanelnadoury@gmail.com, welnadowry@horus.edu.eg